ABSTRACT
We examine the pyrometamorphic changes and resulting crustal contamination that occurred when fragments of Otago Schist were entrained and pyrometamorphosed in Dunedin Volcanic Group (DVG) basanite. Otago Schist greenschist facies quartz-albite laminae were replaced by fine-grained quartz grains (inverted from β-quartz or tridymite) with orthopyroxene coronas and dacitic-rhyolitic glass. Former muscovite + chlorite + epidote ± garnet segregations were converted to plagioclase (An30-80), olivine, spinel, cordierite, ilmenite, pigeonite and trachytic to trachy-andesitic glass. Minerals largely formed by mineral replacement and then by crystal nucleation from a quenched liquid as protolith minerals passed their liquidi, with the latter process generating swallow-tailed, skeletal, dendritic and ladder-structured crystal morphologies. Orthopyroxene-, olivine- and plagioclase-liquid geothermometry indicates xenoliths reached at least 940°C. Despite the molten state of the xenoliths, discernible chemical and isotopic (Sr, Nd) contamination of the basanite was inhibited by its enriched trace element budget. The only evidence for crustal contamination comes from basanite Pb isotopes, which have less radiogenic compositions within several cm of the xenoliths. The subtle variation in DVG Sr and Nd isotopes may therefore be due to different mantle source components rather than crustal contamination by the underlying Otago Schist.
Introduction
Rocks struck by lightning, affected by coal combustion or transported as xenoliths in mafic magmas can experience extreme temperatures (T) at low pressure (P) that lead to the pyrometamorphic formation of new phases and textures characteristic of the sanidinite facies (e.g. Graham et al. Citation1988; Dempster et al. Citation1999; Del Moro et al. Citation2011; Grapes Citation2011; McGee et al. Citation2015). However, as pyrometamorphism is almost instantaneous on a geological timescale, chemical equilibration across a pyrometamorphic rock mass is inhibited. In this study, we assess the effect of pyrometamorphism on element mobility in quartzofeldspathic Otago Schist xenoliths entrained in basanite from the Dunedin Volcanic Group in Otago, New Zealand (), and we examine whether this process could account for the subtle but significant range of Sr-Nd-Pb isotopes seen in Dunedin Volcanic Group basaltic rocks.
Figure 1. Metamorphic grades within the Otago Schist, with the studied sample site (Ram Rock) located in the highest-grade belt of upper greenschist facies. Diagram is modified from Wellnitz et al. (Citation2019).
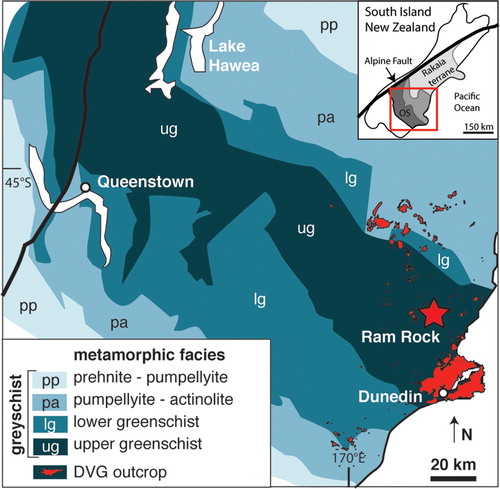
Geological setting
The Oligocene-Miocene Dunedin Volcanic Group (DVG) occurs in the South Island of New Zealand, which is part of the Zealandia continent. Zealandia is a Late Cretaceous rift fragment of the long-lived Australia-Antarctica-Gondwana subduction margin that is composed of an amalgamation of narrow Cambrian to Cretaceous tectonostratigraphic terranes (Landis and Coombs Citation1967; Mortimer Citation2004). The largest exposed terrane is the Rakaia Terrane, which is a large block of mainly quartzofeldspathic rocks extending ∼300 km from south of Dunedin to north of Christchurch (Mortimer Citation2004) (). The contact with the adjacent Caples Terrane is obscured beneath a Jurassic-Cretaceous regionally metamorphosed belt known as the Otago Schist (Mortimer and Roser Citation1992). The suture between the Caples and Rakaia terranes has been overprinted by the Otago Schist, which has converted Al-poor mudstone and greywacke into pelite and psammite, respectively (Turnbull et al. Citation2001). Meta-volcanic (greenschist), chert, and ultramafic rock are rare components (Craw Citation1984; Mortimer Citation1993). Regional metamorphism of the Otago Schist ranges from prehnite-pumpellyite on the northern and southern flanks to greenschist facies garnet-albite zone in the centre of the belt () (Graham and Mortimer Citation1992; Mortimer Citation2000). Peak regional P–T conditions of the exposed rocks are estimated to be 400°C and 0.8 GPa (Mortimer Citation2000) and were attained during Jurassic and Early Cretaceous times (Adams and Graham Citation1997; Little et al. Citation1999; Gray and Foster Citation2004), although the base of the Otago Schist has been affected by a high-temperature Late Cretaceous granulite facies metamorphic event (Jacob et al. Citation2017).
The DVG is an ∼7800 km2 Oligocene-Miocene (25–9 Ma) intraplate field centred around Dunedin and erupted through the Otago Schist (). It mostly comprises small volume alkaline basanites, but compositions extend from basanite to trachytic and phonolitic compositions at evolved centres such as the main Dunedin Volcano edifice (Coombs et al. Citation1986; Price et al. Citation2003; Coombs et al. Citation2008; Scott et al. Citation2020). The trace element and Sr-Nd-Pb isotopic properties have been summarised in Scott et al. (Citation2020), who used their own data and that from Price et al. (Citation2003), Hoernle et al. (Citation2006), Sprung et al. (Citation2007) and Timm et al. (Citation2010) to show that the analysed volcanic rocks generally have isotopic compositions that are very similar to metasomatised lithospheric mantle peridotite xenoliths (Scott, Hodgkinson, et al. Citation2014; Scott, Waight, et al. Citation2014; McCoy-West et al. Citation2016; Dalton et al. Citation2017). However, the DVG isotopic data do have a range of values with the 87Sr/86Sr of some components extending towards slightly more radiogenic values and 143Nd/144Nd extending towards less radiogenic values than the known mantle rocks (e.g. Price and Compston Citation1973; Scott et al. Citation2020). These more evolved compositions could be due to either the magmas having been derived from (or interacted with) a mantle source that is not represented in the lithospheric mantle peridotite xenolith suites, or explained by the magmas having been crustally contaminated by Otago Schist. Therefore, the relationship of pyrometamorphosed Otago Schist xenoliths to their DVG magmas is important because it provides an opportunity to examine the effects of crustal contamination on a primitive magma.
Methods
Since pyrometamorphic rocks typically cool rapidly and grain sizes are extremely fine-grained (e.g. Grapes Citation2011), a scanning electron microscope equipped with an electron dispersal spectrometer (EDS) and an electron backscatter diffraction detector (EBSD) was utilised to characterise the mineralogy, textures, and mineral chemistries. The major element geochemistries of minerals were obtained using a Zeiss Sigma VP FEG Scanning Electron Microscope (SEM) fitted with a HKL INCA Premium Synergy Integrated EDS/EBSD at the Otago Centre for Electron Microscopy at the University of Otago. Operating conditions for EDS were an accelerating voltage of 15 kV, an aperture of 120 µm, and a working distance of 8.5 mm. The electron beam intensity was calibrated using a pure cobalt standard and minerals were standardised using several Smithsonian microbeam standards (Jarosewich et al. Citation1980). EDS data collection, analysis and standardisation were carried out on AZtec software by Oxford Instruments. EBSD operating conditions were an accelerating voltage of 30 kV, an aperture of 300 µm, a working distance of 30 mm with the stage tilted at 70° and the camera at a distance of 207 mm. Maps were collected using a 2—5 μm step size. EBSD data collection was carried out on AZtec software with data analysis via the HKL Channel 5 tango map program, both by Oxford Instruments.
Whole rock geochemistry was measured by ALS Brisbane. Major elements were measured on a dissolved lithium boron fused disk by inductively coupled plasma (ICP) atomic emission spectrometry, and trace elements were measured by ICP-mass spectrometry.
Strontium and neodymium isotopes were measured using a Nu Instruments NuPlasma HR MC-ICP-MS at the Department of Geological Sciences, University of Cape Town, South Africa. Whole rock sample powders were digested over a 48-h period in a 1:1 concentrated HF-HNO3 solution at 140°C before being dried down and converted to nitrate. Element separation was carried out using column chromatography following the methods described in Pin et al. (Citation2014). Two columns were used for elution of digested rock powders, with a Sr Spec resin for extraction of Sr and Pb, and a TRU spec resin for REE separation. A sequential elution of the REE using a Ln Spec resin separated Nd from Sm (Pin et al. Citation2014). Strontium was analysed as a 200 ppb 0.2% HNO3 solution. The 87Sr/86Sr data was corrected for mass interference of 87Rb using the 85Rb signal and the naturally occurring 87Rb/85Rb. Mass fractionation on the ICP-MS was corrected using an 86Sr/88Sr value of 0.1194 and the exponential law. Data was normalised to the NIST SRM987 reference material with an 87Sr/86Sr value of 0.710255 (McArthur Citation1994). Neodymium was analysed as a 50 ppb 2% HNO3 solution on a Nu Instruments DSN-100 desolvating nebuliser. Data was corrected for Sm and Ce interferences using the measured signals of 147Sm and 140Ce and known isotope ratios of Sm and Ce. Correction for instrumental mass fractionation was carried out using a 146Nd/144Nd value of 0.7219 and the exponential law. Normalisation of the corrected 143Nd/144Nd data was carried out using the JNdi-1 reference standard, which has a value of 0.512115 (Tanaka et al. Citation2000). Pb isotopes were analysed as 50 ppb 2% HNO3 solutions using Nu Instruments DSN-100 desolvating nebuliser. A NIST SRM997 Tl standard was added to all standards and samples at a ∼10:1 Pb:Tl ratio. NIST SRM981 was analysed as reference standard, with 206Pb/204Pb, 207Pb/204Pb, 206Pb/204Pb results normalised to values of 15.4963, 16.9405 and 36.7219 (Galer and Abouchami Citation1998). All Pb isotope data were corrected for Hg interference and instrumental mass fractionation using the exponential law and a 205Tl/203Tl value of 2.3889 (after Woodhead Citation2002; Baker et al. Citation2004).
Ram Rock basanite
The intermittently quarried Ram Rock basanite at 45°56′68″S, 170°50′07″E () occurs over an area of ∼0.08 km2 and has a K-Ar age of 16.4 ± 0.7 (Coombs et al. Citation2008). The fan-like columnar jointing (A) and lower elevation compared to nearby basanitic flows are interpreted to mean it is a plug. The basanite contains an array of mantle and crustal xenoliths that range up to 15 cm in diameter (B). The crustal xenoliths form the basis of the paper; for detailed information on mantle xenoliths from this location and elsewhere in Otago, the reader is directed to Dalton et al. (Citation2017), Li et al. (Citation2018), Liu et al. (Citation2015), McCoy-West et al. (Citation2013, Citation2015, Citation2016), Scott, Hodgkinson, et al. (Citation2014), Scott, Waight, et al. (Citation2014), Scott, Brenna, et al. (Citation2016), Scott, Liu, et al. (Citation2016), Scott et al. (Citation2019), and Wang et al. (Citation2016). The fine-grained dark grey basanite contains olivine and augite phenocrysts set in a groundmass of plagioclase, augite and ilmenite. Olivine phenocrysts are commonly 0.2–0.4 mm in size, although larger (∼1 mm) phenocrysts are present. Large olivine phenocrysts have higher Mg contents in the cores (Mg# 83 – Mg# 71, where Mg# = 100*Mg/(Mg + Fe2+)) than the rims (). The smaller phenocrysts have Mg# = 66–72.
Figure 2. A, Ram Rock is a columnar-jointed basanite plug that hosts crust and mantle xenoliths. B, This schist xenolith in the basanite displays dark mafic domains and yellow felsic domains. Purple layers are cordierite. C, Crustal xenolith in outcrop of the basanite. Coin is 26.5 mm. D, Sample of the regional Otago Schist collected from near to the basanite outcrop. E, Outcrop of basanite with a small peridotite xenolith and small crustal xenolith. Coin is 26.5 mm.
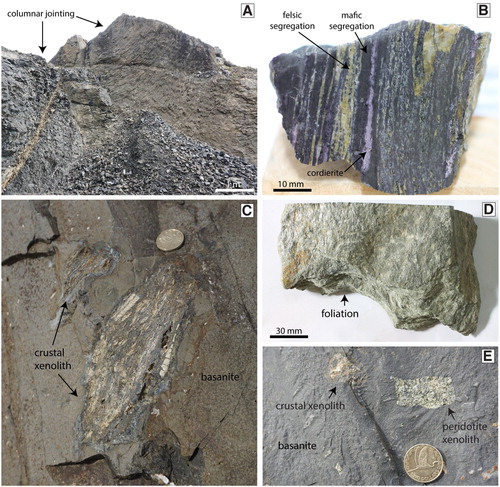
Table 1. Representative analyses of mineral geochemistry from Otago Schist and crustal xenolith samples.
A whole rock analysis was reported by Scott et al. (Citation2020) and two new basanite analyses are reported here (). The two new samples were obtained from within 1.1 to 2 cm (3C) and 0.1 to 1 cm (sample 2B) from a schist xenolith (1A). The three basanite samples show virtually no chemical variation ( and ) except for Rb – but the sample closest to the schist (highest Rb) has the least similar Rb abundance. With the exception of SiO2, Th and U, the basanites tend to have greater element abundances than the schist (; ). The three basanite samples have 87Sr/86Sr(16 Ma) values of 0.70285 to 0.70308 and the same 143Nd/144Nd(16 Ma) (0.51292) (). 206Pb/204Pb values vary from 19.559 to 19.831, 207Pb/204Pb values are 15.644 to 15.656 and 208Pb/204Pb values of 39.376 to 39.243 ().
Figure 3. Total alkali versus silica (TAS) classification diagram of Le Maitre et al. (Citation2005). The host lava plots as a basanite (Reay et al. Citation1991; Scott et al. Citation2020; this paper). Glass compositions from the different segregations of the xenoliths plots in three clusters that display little overlap. Glasses within the felsic domains have sub-alkaline compositions while glasses from the mafic domains and xenolith-basalt interface display alkaline compositions. The Dunedin Volcanic Group field is based on compiled data from Price et al. (Citation2003), Hoernle et al. (Citation2006), Sprung et al. (Citation2007), Timm et al. (Citation2010) and Scott et al. (Citation2020).
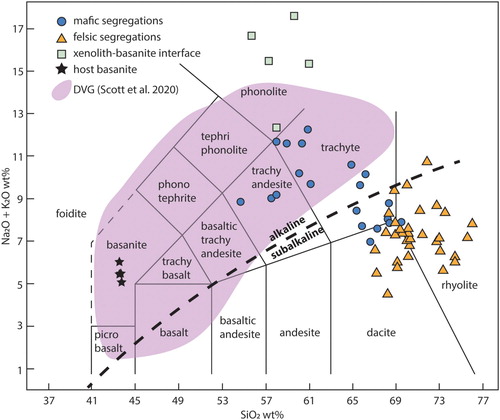
Figure 4. Multi-element plot of measured Ram Rock basanite and an Otago Schist xenolith with two samples of basanite at varying distances from an Otago Schist xenolith.
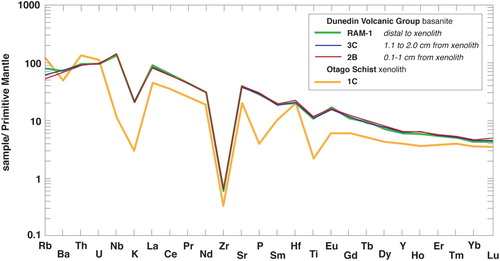
Table 2. Whole rock geochemistry and Sr-Nd-Pb isotopic analyses for Ram Rock basanite samples and an Otago Schist xenolith.
Otago Schist
As a reference frame for the pyrometamorphosed xenoliths described below, samples of un-pyrometamorphosed quartzofeldspathic Otago Schist from near to the basanite have been inspected. The rocks are pale green-grey, have a pervasive foliation and prominent millimetre-scale segregations common to the Otago Schist (C). The segregations comprise alternating quartz-albite and phyllosilicate-rich layers (A). The quartz-albite layers are typically 0.5–2 mm wide and separate 1–3 mm wide microlithons that are dominated by white mica, chlorite and epidote that are sometimes accompanied by small garnet grains (∼0.1 mm). The albite is end-member albite (Ab100An0Or0) () . The white mica is muscovite and contains 14.5 wt% K2O, ∼3.4 wt% MgO and ∼2.5 wt% FeO. Epidote has ∼9 wt% Fe2O3 and 22.7 wt% CaO. Chlorite is rich in Fe (FeO ∼27 wt%) compared to Mg (MgO ∼12 wt%). Garnet grains have ragged margins and are zoned, with a core to rim variation of Alm24-33Grs34-38Sps39-27Andr2-2.
Figure 5. A, Photomicrograph in plane polarised light (PPL) of a garnet-bearing sample of Otago Schist collected near Ram Rock. B, Photomicrograph in PPL of a felsic domain in one of the collected Ram Rock crustal xenoliths. Quartz has lobate boundaries and a dark corona. Glass is heterogeneous in colour, ranging from colourless to a dark brown. C, Backscattered electron (BSE) image of skeletal orthopyroxene grains within glass in a felsic layer. D, Photomicrograph in PPL of a mafic domain in one of the collected Ram Rock crustal xenoliths. The mafic segregations are heterogeneous with a variety of minerals that are too small for optical identification. Glass varies from a medium brown to a dark brown/black colour. E, BSE image of plagioclase grains with a rectangular prismatic crystal shape. This morphology of plagioclase is always found within glass in the mafic layers. F, Inverted BSE image of a zoned anhedral plagioclase grain. Zoned plagioclase grains in the studied xenoliths consistently have a Ca-rich core and Na-rich rim. mus = muscovite, chl = chlorite, grt = garnet, qtz = quartz, opx = orthopyroxene, plag = plagioclase, dv glass = devitrified glass.
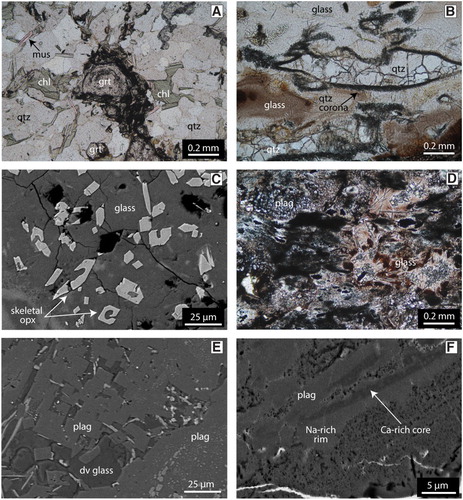
Pyrometamorphic Otago Schist xenoliths
Like the in-situ outcrop schist, the Otago Schist xenoliths in the Ram Rock plug display prominent segregation layering, except that the former white quartz-albite layers are now yellow-white and the former mica-rich brown bands are now dark grey (B). Bands and lenses composed of light purple cordierite occur in several samples (B). Calculation of the effective bulk composition of the major domains using mineral modes and mineral chemistries indicates that the yellow-white segregations are very felsic (SiO2 > 80 wt%), whereas the dark domains are very mafic (SiO2 < 40 wt%).
The felsic domains comprise lobate polycrystalline quartz grains within a colourless to pale brown glass (B). Quartz grains are 1–20 mm and are surrounded by a thin rim composed of very fine-grained (<50 µm) needles of orthopyroxene. Dauphine twins were identified in the quartz grains using EBSD analysis; these twins are related to each other through 180° rotations of the c-axis (Tullis Citation1970). Orthopyroxene grains can also be found within glass and commonly display skeletal rims or swallow tail crystal terminations (C). The orthopyroxene grains are enstatite, varying from 20 to 30 wt% FeO and 13–20 wt% MgO, with pigeonite only identified in a large symplectite (). The glass is silica and aluminium-rich with 4–5 wt% FeO, 5–7 wt% K2O and ∼3 wt% Na2O () and has a dacite to rhyolitic composition when plotted on a TAS diagram ().
Table 3. Representative geochemical analyses of glasses within the crustal xenoliths. Glass was measured in both the felsic and mafic domains and from the basanite-xenolith interface.
The mafic segregations have more variable compositions compared to the felsic segregations. Their mineral assemblages comprise combinations of plagioclase, cordierite, olivine, spinel, ilmenite and glass (D). Plagioclase is present as euhedral laths, prismatic grains or anhedral grains that vary from 10–50 µm (E,F). Feldspar compositions vary from anorthite to oligoclase and anorthoclase, with most grains either being calcic (Ab15-25An70-80Or5) or sodic (Ab50-60An40-50Or2-15) plagioclase (; ). Normal zoning and replacement features of Ca-rich plagioclase are present (E,F). The purple colouration noted in some hand samples (B) is made up of fine 10–20 µm prismatic grains of cordierite within glass (A). Cordierite grains contain 7–9 wt% FeO and 7.5–8.5 wt% MgO and have a 38–40 mol% sekaninaite (Fe2Al3(AlSi5O18)) component (). Olivine occurs mainly in the form of very fine (1–5 µm) symplectic intergrowths with spinel (B). Stunning cruciform olivine grains, ∼4 µm in size, are common (C). The symplectic olivine grains have a composition of Fo45-53Fa47-65Tp1, whereas the cruciform grains have higher iron contents (Fo31Fa68Tp1; ). The intergrown spinel is hercynite and has 22–40 wt% FeO and 7–14 wt% MgO, with V2O3 reaching up to 1.6 wt%. One very large symplectite (∼150 µm) contains anorthite, spinel, olivine, and ilmenite surrounded by pigeonite (D). Ilmenite occurs in a lobate morphology and is surrounded by glass. Ilmenite often contains minor MgO (∼2 wt%) and up to 2.6 wt% V2O3. An acicular mineral is present within all samples but remains unidentified due to the fine grainsize; this mineral often has a dendritic morphology and may be mullite (E). Glass in the mafic section is trachyandesite to trachydacite () and the totals slightly lower than 100% indicate that it is hydrous ().
Figure 6. Ternary feldspar diagram showing the wide variety of plagioclase compositions in the crustal xenoliths in comparison to the pure albite of the Otago Schist protolith. Plagioclase with a prismatic morphology tends to have an andesine composition while anhedral plagioclase is comparatively enriched in Ca. Considerable overlap in the plagioclase chemistry is present between the different plagioclase morphologies. Feldspar isotherms are from Benisek et al. (Citation2010).
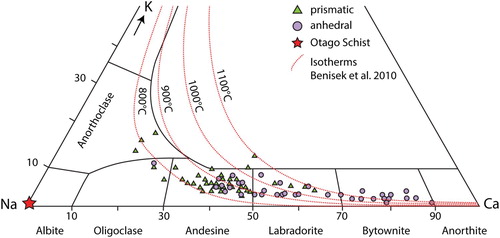
Figure 7. A, BSE image of cordierite in a mafic segregation with angular grain boundaries. Cordierite is typically found with a rectangular to square crystal morphology and hosted in glass. B, BSE image of an olivine-spinel symplectite. C, BSE image of cruciform and laddered olivine grains within devitrified glass. D, BSE image of a large symplectite composed of intergrown anorthite, ilmenite, olivine, and spinel with a rim of larger subhedral pigeonite grains. E, BSE image of an unknown mineral within the xenoliths. This mineral has an acicular morphology and can also be found as dendrites. F, BSE image of acicular SiO2 grains that have been identified at the xenolith-basanite interface. crd = cordierite, ol = olivine, spl = spinel, dv glass = devitrified glass, pig = pigeonite, ilm = ilmenite, an = anorthite, pl = plagioclase, un-id = unidentified mineral, qtz = quartz, cpx = clinopyroxene.
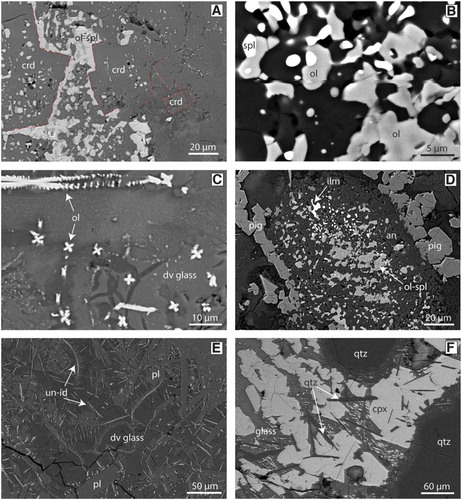
Discussion
Geothermometry
Since most of the pyrometamorphic minerals appear to have formed from precipitation from a liquid, the chemistries of minerals co-existing with glass can be used to estimate mineral-liquid equilibration conditions. Selected mineral-glass pairs occur within 50 µm of one another and a P = 1 bar is applied to reflect the shallow crystallisation. Using 1 bar or 1 kbar makes very little difference to the results of calculations; for example, the difference is only 31°C for the olivine-liquid geothermometer of Beattie (Citation1993), which has an endemic standard error associated with the equation of 53°C. The error used for the average calculated temperatures is 2SD to reflect the small population sizes used for each geothermometer. Orthopyroxene-liquid thermometry (Beattie Citation1993, equation 11; Putirka Citation2008, equation 28a) is based on Mg-Fe-Mn exchange. Euhedral orthopyroxene crystals within the felsic segregations are set within a glass and over half of the samples are in equilibrium with this glass when plotted on a Rhodes diagram (Roeder and Emslie Citation1970). The pairs that display equilibrium give averages of 1086 ± 73°C and 940 ± 112°C for the two calibrations, respectively (). The Beattie equation gives higher temperatures, but does not consider the presence of H2O within the liquid, which may cause overestimation. Plagioclase-liquid geothermometry (Putirka Citation2005, equation A; Putirka Citation2008, equation 24a) was used on coexisting phases within the mafic domains and yielded averages of 978 ± 96°C and 942 ± 111°C (). Since the mafic domains also contain olivine and glass, four olivine-liquid geothermometers were used; equation 10 from Beattie (Citation1993), a H2O corrected version of the same equation, and equations 2 and 4 from Putirka et al. (Citation2007). Olivine-glass pairs were plotted on a Rhodes diagram to evaluate equilibrium, and those satisfying equilibrium criteria yielded 1044 ± 86°C (for both Beattie equations) and 856 ± 167°C and 987 ± 112°C (Putirka equations), respectively ().
Table 4. Average values and the standard deviation for geothermometry of selected liquid-mineral pairs.
Reactions of minerals
Two stages of mineral reaction have likely occurred in the felsic and mafic domains in the xenoliths following conversion from older greenschist facies assemblages. The first is prograde heating, where the Otago Schist greenschist facies minerals reacted to form new phases such as the olivine-spinel symplectites in the mafic domains or plagioclase co-existing with melt in the felsic domains (E, 7B). The occurrence of olivine, orthopyroxene, cordierite, pigeonite with glass indicates formation at sanidinite facies conditions. The second stage of mineral reactions occurred as the xenoliths began to cool and new minerals nucleated from the liquid that is estimated via orthopyroxene-, olivine- and plagioclase-melt equilibria to have been >940°C (). Examples of this are the cruciform olivine in the mafic layers and the skeletal quench-textured orthopyroxene grains in the felsic layers (C, 7C). The bimodal composition of plagioclase may represent these two reaction stages. On heating, plagioclase melts through dissolution of pre-existing grains and growth of new plagioclase is progressively enriched in the anorthite component (Grapes Citation2011). Ca-rich plagioclase grains in the studied xenoliths are anhedral and likely formed in the first stage of mineral reactions. The evidence for the this may be the calcic cores and sodic rims (F). This indicates Ca-rich grains were overgrown by Na-rich plagioclase, and the Na-rich grains have tabular textures and are set within glass and probably crystallised directly from the melt (E).
One of the main phases that does not appear to have precipitated from melt is quartz (B). Electron backscatter diffraction analysis indicates that these grains are α-quartz, which is the low-T polymorph; however, the presence of a high frequency of dauphine twins (relative frequency of misorientation angles of 0.26; ) compared to the representative Otago Schist samples (relative frequency of 0.18; ) indicates that the grains in the pyrometamorphic rock have inverted from β-quartz (or higher) during cooling (Barber and Wenk Citation1991). The presence of needles of quartz surrounding sub-spherical quartz grains (F) is similar to textures that have been interpreted as a result of partial melting of β-quartz and precipitation of tridymite (Clark and Peacor Citation1992; Holness et al. Citation2005; Grapes Citation2011), which occurs at > 870°C (Klein and Philpotts Citation2013).
Figure 8. Distribution of misorientation angles of analysed quartz in an Otago Schist sample (A) and a crustal xenolith (B). The peak of ∼60° represents the dauphine twins. A higher relative frequency of dauphine twins is present in the crustal xenolith (0.26) in comparison to the Otago Schist (0.18).
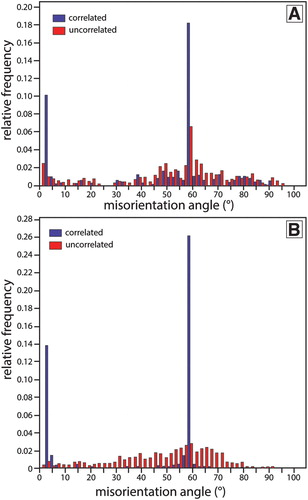
The Otago Schist in the area is phyllosilicate-rich, yet neither chlorite nor mica were identified in the xenoliths (). Published occurrences of thermal decomposition in pyrometamorphic rocks has predicted that breakdown of intermediate Mg-Fe chlorite – similar to the chlorite present in the Otago schist – occurs at T > 650°C and leads to the formation of olivine, orthopyroxene, cordierite, spinel, magnetite (McOnie et al. Citation1975; Worden et al. Citation1987). Magnetite and corundum are absent in the studied xenoliths, but common olivine-spinel symplectites found within the mafic domains could be the result of chlorite breakdown (B, 9A). Prismatic cordierite grains within glass are often associated with these symplectites. Muscovite breakdown occurs via dehydroxylation, which converts the hydroxyl groups into H2O, lowering the solidus within the xenoliths and resulting in melting at T > 900°C (Guggenheim et al. Citation1987; Gridi-Bennadji et al. Citation2008). Studies on high temperature muscovite breakdown show the formation of mullite or corundum, often with sanidine (Guggenheim et al. Citation1987; Rodriguez-Navarro et al. Citation2003; Gridi-Bennadji et al. Citation2008; Grapes Citation2011). The sanidine component is often incorporated within the melt, as K+ ions are highly mobile (Devineau et al. Citation2006; Grapes Citation2011). Corundum and mullite are both absent in the xenoliths, although acicular grains that are too fine-grained for SEM analysis do resemble mullite (E). However, the bulk rock composition may not allow for formation of these minerals as spinel is a common Al-phase instead.
Figure 9. A, Schematic diagram of the mineralogical and textural changes that the regionally metamorphosed Otago Schist underwent during pyrometamorphism. Not to scale. B, P-T-t diagram of regional metamorphism of the Otago Schist around 160–140 Ma, followed by the pyrometamorphic event at 16 Ma (Coombs et al. Citation2008). Pressure and temperature range of Otago Schist metamorphism from Mortimer (Citation2000). Entrainment of the crustal xenoliths occurred at a shallow depth, with peak metamorphism reached quickly before the xenoliths were erupted and cooled.
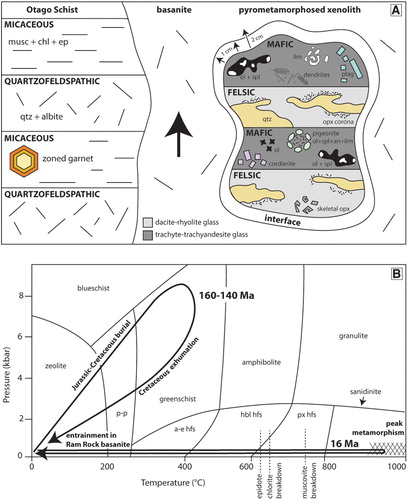
While epidote is present in greenschist facies Otago Schist, all epidote has been destroyed in the xenoliths and no obvious replacement textures are evident (A). Experimental data on the breakdown of epidote at T > 625°C indicates anorthite is a product, as indicated by increasing Ca content of plagioclase (Apted and Liou Citation1983). Anorthite, cordierite, and H2O are the likely products of epidote breakdown when the Otago Schist epidote is plotted on an ACF (alkali-calcium-iron) diagram (from Eskola Citation1939 in Grapes Citation2011). Cordierite and anorthite-rich feldspar are common in the xenoliths.
Garnet is a minor component within the Otago Schist. A rare symplectite anorthite, olivine, spinel, and ilmenite in one pyrometamorphosed xenolith is very similar to the breakdown products of garnet documented in pyroxene hornfels facies mafic rocks (D, 9A) (Scott et al. Citation2013). The analysed symplectite has a bulk major element composition similar to Otago Schist garnet with the exception of Mn (). Misch and Onyeagocha (Citation1976) documented manganese mobility in non-isochemical pyrometamorphic garnet breakdown and suggest that Mn could be mobilised and then hosted in orthopyroxene, olivine, spinel, cordierite and ilmenite. Titanium and Mg were added during symplectite formation, most likely from the surrounding melt. A pigeonite corona surrounds the symplectite, and this may reflect a reaction front and signify interaction with the surrounding melt or minerals.
Table 5. Comparison of major element mineral chemistry of garnets from the analysed Otago Schist of a large anorthite-ilmenite-olivine-spinel-pigeonite symplectite in one of the crustal xenoliths.
Model for the evolution of the xenoliths
The cellular mineral growth textures, particularly skeletal and swallow tail orthopyroxene grains, dendritic morphologies, and cruciform and ladder olivine (C, 7C, 7E), have been documented in minerals that crystallise rapidly by quenching of melts (Bryan Citation1972). Common quench textures include spherulites (Swanson Citation1977), dendritic mineral shapes (Evans et al. Citation1994), skeletal grains, swallow-tails and mineral chains (Bryan Citation1972; Nabelek et al. Citation1978). These textures have been documented in minerals including magnetite (Long and Wood Citation1986), olivine (Bryan Citation1972; Tegner et al. Citation1993), and plagioclase (Bryan Citation1972). The presence of these quench textures in the Ram Rock Otago Schist xenoliths leads to the question of how they formed within a basanite that itself does not display evidence of quenching.
The morphology of crystals is a function of the growth rate and the mobility of crystal-forming components in the melt, measured as the diffusion coefficient (Kirkpatrick Citation1975). Crystal growth theory states that skeletal grains or dendritic morphologies form where compositional gradients and diffusion near the crystal-melt interface impact the crystal growth, causing the crystal to break up into ‘cells’ (Kirkpatrick Citation1975). Large undercooling typically results in spherulitic crystal growth, then dendritic, followed by skeletal grains to finally form tabular grains as the degree of undercooling decreases (Lofgren Citation1974; Swanson Citation1977). Experimental work has shown that diffusion-controlled growth results in the formation of swallow-tail morphology in plagioclase crystals (Nabelek et al. Citation1978). Diffusion controlled growth becomes the dominant form over interface-controlled growth as supersaturation increases.
Due to the different chemistry and mineralogy of the schist xenoliths compared to the basanite, the thermal properties and viscosity of the schist xenoliths will vary from that of the basanite. The mode of crystal formation in the xenoliths must have been different than in the magma, as shown by the variety of mineral textures present (C–F, 7A–F). Limited chemical exchange between the mafic and felsic domains suggests that element mobility was extremely restricted. The xenolith quench textures may therefore have formed due to a dominance of diffusion-controlled growth and the limited mobility of elements. Nucleation dynamics were also different in the xenolith in comparison to the basanite, with a finer grain size and a higher number of crystals. The higher number of crystals will have limited the size of crystal growth during the short time of entrainment.
Crustal contamination
Although the textures show that Otago Schist xenoliths were almost entirely composed of liquids whilst in the host magma, the whole rock trace element data show little evidence for contamination of the surrounding basanite (). Simple assimilation (A) – percentage of melt remaining (F) – crystallisation (C) models, assuming trace element fractionation of only olivine and clinopyroxene, using crystal-liquid partition coefficients for Sr, Nd and Pb from the GERM database and a r (mass of assimilation compared to mass of crystallisation) of 0.2, also indicate that the basanite Sr and Nd isotopes display negligible isotopic change adjacent to the xenolith. This is because the Otago Schist has roughly half the Sr (845 ppm) and Nd (25.7 ppm) of the basanite (A; ) and so, for example, changing the Sr isotope ratio from ∼0.7029 to ∼0.7035 in the basanite via crustal contamination would require the isotopically evolved component to have crystallised from a liquid with F of ∼0.1 (e.g. 90% already crystallised) (A). This is unrealistic because for values of F > 0.5, the remaining melt would no longer be basaltic. In contrast to Sr and Nd, however, the average Pb concentration for DVG basanite (3.7 ± 5 ppm; Hoernle et al. Citation2006; Timm et al. Citation2010) is lower than the Otago Schist (18 ± 4 ppm; Scanlan et al. Citation2018; Scott et al. Citation2020). This means that basanite Pb isotopes should be more susceptible than Sr and Nd to modification by small amounts of Otago Schist contamination. B,C shows that the basanite 206Pb/204Pb, 207Pb/204Pb and 208Pb/204Pb isotope ratios plot close to or along the AFC arrays, and that this contamination can be achieved before significant volumes of melt crystallised. The Pb analyses therefore show that there are haloes of crustally contaminated basanite extending several cm from the xenoliths, but the enriched trace element character of the basanite in most other elements obscures this contamination. Furthermore, since mantle xenolith-bearing alkali basalts are estimated to rise through the crust at rates of 0.01–10 m/s (Spera Citation1984; Peslier and Luhr Citation2006), the process of the Ram Rock basanite ascending rapidly, entraining and then rapidly quenching them should have also inhibited xenolith-magma interaction.
Figure 10. A, Plot of 143Nd/144Nd versus 87Sr/86Sr shows little isotopic change in the basanite samples within several cm from a crustal xenolith (2B, 3C). B, 207Pb/204Pb versus 206Pb/204Pb reveals variation in the basanite adjacent to the crustal xenolith, with the highest isotopic change occurring in the sample taken from closest to the xenolith. C, A similar trend to 10B is visible on a plot of 208Pb/204Pb against 206Pb/204Pb. The arrays represent the modelled fraction of melt remaining as the isotopic compositions evolve. The DVG basalts are from Scott et al. (Citation2020).
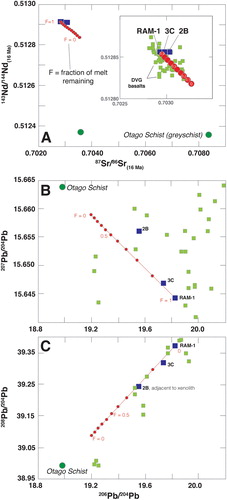
The implication of the AFC modelling is that the isotopic spread that occurs in the DVG basalts (), especially in the Sr and Nd isotopes, is unable to be explained alone by crustal contamination by Otago Schist greyschist. Exchanging the contaminant of the basalts with greenschist, which is the next major component of the Otago Schist, also fails to explain the variable compositions of some DVG basalts since the greenschists have unradiogenic 87Sr/86Sr (∼0.70498), radiogenic 143Nd/144Nd (0.51270) and unradiogenic Pb (206Pb/204Pb = 18.769; 207Pb/204Pb = 15.627; 208Pb/204Pb = 38.676) (Scanlan Citation2018). This means that the isotopic variation in the DVG basalts is either due to post-crystallisation processes (e.g. by filling of voids by crustally Sr-enriched fluids) or that mantle source to the magmas was subtly heterogeneous. We prefer the latter explanation, as many of the isotopically investigated basalts do not have large amounts of alteration.
Conclusions
Quartzofeldspathic schist xenoliths entrained within a Miocene Dunedin Volcanic Group basanite magma display pyrometamorphic phase assemblages that are constrained by the pre-existing metamorphic segregations in their Otago Schist protolith. Mineral-melt equilibria and feldspar compositions indicate that temperatures achieved during pyrometamorphism were >940°C. The effective reacting domains were either extremely felsic or extremely mafic, with rhyolite-dacite glass in the felsic layers and the trachyte-trachyandesite glasses in the mafic layers having formed as different minerals passed their thermal maximums and then dissolved other components. A combination of the elevated trace element composition of the basanite compared to the crustal xenoliths, coupled with rapid quenching of the partially melted xenoliths, inhibited chemical exchange. The host basanite largely retains a primitive Sr and Nd isotopic composition but Pb isotopes reveal evidence for crustal contamination within several cm of the Otago Schist xenoliths. Nonetheless, crustal contamination cannot account for the range in Sr, Nd and Pb isotopes in the Dunedin Volcanic Group basalts, which means that this volcanic province is probably derived from several subtly different isotopic reservoirs.
Acknowledgments
We thank Brent Pooley for making the polished sections and Kat Lilly and Marianne Negrini for SEM assistance with analyses. Dave Prior helped identification and interpretation of the dauphine twins. We thank Alex McCoy-West and Christian Timm for their insightful reviews.
Disclosure statement
No potential conflict of interest was reported by the author(s).
Data availability statement
The authors confirm that the data supporting the findings of this study are available within the article.
References
- Adams CJ, Graham IJ. 1997. Age of metamorphism of Otago Schist in eastern Otago and determination of protoliths from initial strontium isotope characteristics. New Zealand Journal of Geology and Geophysics. 40(3):275–286. doi: 10.1080/00288306.1997.9514760
- Apted MJ, Liou JG. 1983. Phase relations among greenschist, epidote-amphibolite, and amphibolite in a basaltic system. American Journal of Science. 283(A):328–354.
- Baker J, Peate D, Waight T, Meyzen C. 2004. Pb isotopic analysis of standards and samples using a 207Pb–204Pb double spike and thallium to correct for mass bias with a double-focusing MC-ICP-MS. Chemical Geology. 211(3-4):275–303. doi: 10.1016/j.chemgeo.2004.06.030
- Barber DJ, Wenk HR. 1991. Dauphiné twinning in deformed quartzites: implications of an in situ TEM study of the α-β phase transformation. Physics and Chemistry of Minerals. 17(6):492–502. doi: 10.1007/BF00202229
- Beattie P. 1993. Olivine-melt and orthopyroxene-melt equilibria. Contributions to Mineralogy and Petrology. 115(1):103–111. doi: 10.1007/BF00712982
- Benisek A, Dachs E, Kroll H. 2010. A ternary feldspar-mixing model based on calorimetric data: development and application. Contributions to Mineralogy and Petrology. 160(3):327–337. doi: 10.1007/s00410-009-0480-8
- Bryan WB. 1972. Morphology of quench crystals in submarine basalts. Journal of Geophysical Research. 77(29):5812–5819. doi: 10.1029/JB077i029p05812
- Clark BH, Peacor DR. 1992. Pyrometamorphism and partial melting of shales during combustion metamorphism: mineralogical, textural, and chemical effects. Contributions to Mineralogy and Petrology. 112(4):558–568. doi: 10.1007/BF00310784
- Coombs DS, Adams CJ, Roser BP, Reay A. 2008. Geochronology and geochemistry of the Dunedin Volcanic Group, eastern Otago, New Zealand. New Zealand Journal of Geology and Geophysics. 51(3):195–218. doi: 10.1080/00288300809509860
- Coombs DS, Cas RA, Kawachi Y, Landis CA, McDonough WF, Reay A. 1986. Cenozoic volcanism in north, east and central Otago. Royal Society of New Zealand Bulletin. 23:278–312.
- Craw D. 1984. Lithologic variations in Otago Schist, Mt Aspiring area, northwest Otago, New Zealand. New Zealand Journal of Geology and Geophysics. 27(2):151—166. doi: 10.1080/00288306.1984.10422524
- Dalton HB, Scott JM, Liu J, Waight TE, Pearson DG, Brenna M, Le Roux P, Palin JM. 2017. Diffusion-zoned pyroxenes in an isotopically heterogeneous mantle lithosphere beneath the Dunedin Volcanic Group, New Zealand, and their implications for intraplate alkaline magma sources. Lithosphere. 9(3):463–475. doi: 10.1130/L631.1
- Del Moro S, Renzulli A, Tribaudino M. 2011. Pyrometamorphic processes at the magma–hydrothermal system interface of active volcanoes: evidence from buchite ejecta of Stromboli (Aeolian Islands, Italy). Journal of Petrology. 52(3):541–564. doi: 10.1093/petrology/egq090
- Dempster TJ, Preston RJ, Bell BR. 1999. The origin of Proterozoic massif-type anorthosites: evidence from interactions between crustal xenoliths and basaltic magma. Journal of the Geological Society. 156(1):41–46. doi: 10.1144/gsjgs.156.1.0041
- Devineau K, Devouard B, Villieras F, Faure F, Devidal JL, Kohler A. 2006. Evolution of product phase assemblages during thermal decomposition of muscovite under strong disequilibrium conditions. American Mineralogist. 91(2-3):413–424. doi: 10.2138/am.2006.1933
- Eskola P. 1939. Die metamorphen Gesteine. In: Barth TFW, Correns CW, Eskola P, editors. Die Entstehung der Gesteine. Berlin Heidelberg: Springer; p. 263–407.
- Evans NJ, Shahinpoor M, Ahrens TJ. 1994. Hypervelocity impact: ejecta velocity, angle, and composition. Geological Society of America. Special Paper. 293:93–101. doi: 10.1130/SPE293-p93
- Galer SJG, Abouchami W. 1998. Practical application of lead triple spiking for correction of instrumental mass discrimination. Mineralogical Magazine. 62:491–492. doi: 10.1180/minmag.1998.62A.1.260
- Graham IJ, Grapes RH, Kifle K. 1988. Buchitic metagreywacke xenoliths from Mount Ngauruhoe, Taupo Volcanic Zone, New Zealand. Journal of Volcanology and Geothermal Research. 35(3):205–216. doi: 10.1016/0377-0273(88)90017-0
- Graham IJ, Mortimer N. 1992. Terrane characterisation and timing of metamorphism in the Otago Schist, New Zealand, using Rb–Sr and K–Ar geochronology. New Zealand Journal of Geology and Geophysics. 35(4):391–401. doi: 10.1080/00288306.1992.9514534
- Grapes R. 2011. Pyrometamorphism. 2nd ed. Berlin Heidelberg: Springer-Verlag.
- Gray DR, Foster DA. 2004. 40Ar/39Ar thermochronologic constraints on deformation, metamorphism and cooling/exhumation of a Mesozoic accretionary wedge, Otago Schist, New Zealand. Tectonophysics. 385(1-4):181–210. doi: 10.1016/j.tecto.2004.05.001
- Gridi-Bennadji F, Beneu B, Laval JP, Blanchart P. 2008. Structural transformations of muscovite at high temperature by X-ray and neutron diffraction. Applied Clay Science. 38(3-4):259–267. doi: 10.1016/j.clay.2007.03.003
- Guggenheim S, Chang YH, Koster van Gross AF. 1987. Muscovite dehydroxylation; high-temperature studies. American Mineralogist. 72(5-6):537–550.
- Hoernle K, White JV, van den Bogaard P, Hauff F, Coombs DS, Werner R, Timm C, Garbe-Schönberg D, Reay A, Cooper AF. 2006. Cenozoic intraplate volcanism on New Zealand: upwelling induced by lithospheric removal. Earth and Planetary Science Letters. 248(1-2):350–367. doi: 10.1016/j.epsl.2006.06.001
- Holness MB, Dane K, Sides R, Richardson C, Caddick M. 2005. Melting and melt segregation in the aureole of the Glenmore Plug, Ardnamurchan. Journal of Metamorphic Geology. 23(1):29–43. doi: 10.1111/j.1525-1314.2005.00560.x
- Jacob JB, Scott JM, Turnbull RE, Tarling MS, Sagar MW. 2017. High-to ultrahigh-temperature metamorphism in the lower crust: an example resulting from Hikurangi Plateau collision and slab roll-back in New Zealand. Journal of Metamorphic Geology. 35(8):831–853. doi: 10.1111/jmg.12257
- Jarosewich E, Nelen JA, Norberg JA. 1980. Reference samples for electron microprobe analysis. Geostandards Newsletter. 4(1):43–47. doi: 10.1111/j.1751-908X.1980.tb00273.x
- Kirkpatrick RJ. 1975. Crystal growth from the melt: a review. American Mineralogist: Journal of Earth and Planetary Materials. 60(9-10):798–814.
- Klein C, Philpotts AR. 2013. Earth materials: introduction to mineralogy and petrology. New York (NY): Cambridge University Press.
- Landis CA, Coombs DS. 1967. Metamorphic belts and orogenesis in southern New Zealand. Tectonophysics. 4(4-6):501–518. doi: 10.1016/0040-1951(67)90014-5
- Le Maitre RW, Streckeisen A, Zanettin B, Le Bas MJ, Bonin B, Bateman P. 2005. Igneous rocks: a classification and glossary of terms: recommendations of the International Union of Geological Sciences Subcommission on the Systematics of Igneous Rocks. 2nd ed. Cambridge, UK: Cambridge University Press.
- Li P, Scott JM, Liu J, Xia QK. 2018. Lateral H2O variation in the Zealandia lithospheric mantle controls orogen width. Earth and Planetary Science Letters. 502:200–209. doi: 10.1016/j.epsl.2018.09.004
- Little TA, Mortimer N, McWilliams M. 1999. An episodic Cretaceous cooling model for the Otago-MArlborough Schist, New Zealand, based on 40Ar/39Ar white mica ages. New Zealand Journal of Geology and Geophysics. 42(3):305–325. doi: 10.1080/00288306.1999.9514848
- Liu J, Scott JM, Martin CE, Pearson DG. 2015. The longevity of Archean mantle residues in the convecting upper mantle and their role in young continent formation. Earth and Planetary Science Letters. 424:109–118. doi: 10.1016/j.epsl.2015.05.027
- Lofgren G. 1974. An experimental study of plagioclase crystal morphology; isothermal crystallization. American Journal of Science. 274(3):243–273. doi: 10.2475/ajs.274.3.243
- Long PE, Wood BJ. 1986. Structures, textures, and cooling histories of Columbia River basalt flows. Geological Society of America Bulletin. 97(9):1144–1155. doi: 10.1130/0016-7606(1986)97<1144:STACHO>2.0.CO;2
- McArthur JM. 1994. Recent trends in strontium isotope stratigraphy. Terra Nova. 6(4):331–358. doi: 10.1111/j.1365-3121.1994.tb00507.x
- McCoy-West AJ, Bennett VC, Amelin Y. 2016. Rapid Cenozoic ingrowth of isotopic signatures simulating ‘HIMU’ in ancient lithospheric mantle: distinguishing source from process. Geochimica et Cosmochimica Acta. 187:79–101. doi: 10.1016/j.gca.2016.05.013
- McCoy-West AJ, Bennett VC, O’Neill HSC, Hermann J, Puchtel IS. 2015. The interplay between melting, refertilization and carbonatite metasomatism in off-cratonic lithospheric mantle under Zealandia: an integrated major, trace and platinum group element study. Journal of Petrology. 56(3):563–604. doi: 10.1093/petrology/egv011
- McCoy-West AJ, Bennett VC, Puchtel IS, Walker RJ. 2013. Extreme persistence of cratonic lithosphere in the southwest Pacific: Paleoproterozoic Os isotopic signatures in Zealandia. Geology. 41(2):231–234. doi: 10.1130/G33626.1
- McGee LE, McLeod C, Davidson JP. 2015. A spectrum of disequilibrium melting preserved in lava-hosted, partially melted crustal xenoliths from the Wudalianchi volcanic field, NE China. Chemical Geology. 417:184–199. doi: 10.1016/j.chemgeo.2015.09.023
- McOnie AW, Fawcett JJ, James RS. 1975. The stability of intermediate chlorites of the clinochlore-daphnite series at 2 kbar PH2O. American Mineralogist: Journal of Earth and Planetary Materials. 60(11-12):1047–1062.
- Misch P, Onyeagocha AC. 1976. Symplectite breakdown of Ca-rich almandines in upper amphibolite- facies Skagit Gneiss, North Cascades, Washington. Contributions to Mineralogy and Petrology. 54(3):189–224. doi: 10.1007/BF00371006
- Mortimer N. 2000. Metamorphic discontinuities in orogenic belts: example of the garnet–biotite–albite zone in the Otago Schist, New Zealand. International Journal of Earth Sciences. 89(2):295–306. doi: 10.1007/s005310000086
- Mortimer N. 2004. New Zealand's geological foundations. Gondwana Research. 7(1):261–272. doi: 10.1016/S1342-937X(05)70324-5
- Mortimer N. 1993. Jurassic tectonic history of the Otago Schist, New Zealand. Tectonics. 12(1):237–244. doi: 10.1029/92TC01563
- Mortimer N, Roser BP. 1992. Geochemical evidence for the position of the Caples–Torlesse boundary in the Otago Schist, New Zealand. Journal of the Geological Society. 149(6):967–977. doi: 10.1144/gsjgs.149.6.0967
- Nabelek PI, Taylor LA, Lofgren GE. 1978. Nucleation and growth of plagioclase and the development of textures in a high-alumina basaltic melt. In: 9th Lunar and Planetary Science Conference Proceedings, Houston, Texas. p. 725–741.
- Peslier AH, Luhr JF. 2006. Hydrogen loss from olivines in mantle xenoliths from Simcoe (USA) and Mexico: MAfic alkalic magma ascent rates and water budget of the sub-continental lithosphere. Earth and Planetary Science Letters. 242(3-4):302–319. doi: 10.1016/j.epsl.2005.12.019
- Pin C, Gannoun A, Dupont A. 2014. Rapid, simultaneous separation of Sr, Pb, and Nd by extraction chromatography prior to isotope ratios determination by TIMS and MC-ICP-MS. Journal of Analytical Atomic Spectrometry. 29(10):1858–1870. doi: 10.1039/C4JA00169A
- Price RC, Compston W. 1973. The geochemistry of the Dunedin Volcano: strontium isotope chemistry. Contributions to Mineralogy and Petrology. 42(1):55–61. doi: 10.1007/BF00521647
- Price RC, Cooper AF, Woodhead JD, Cartwright I. 2003. Phonolitic diatremes within the Dunedin volcano, south Island, New Zealand. Journal of Petrology. 44(11):2053–2080. doi: 10.1093/petrology/egg070
- Putirka KD. 2005. Igneous thermometers and barometers based on plagioclase+ liquid equilibria: tests of some existing models and new calibrations. American Mineralogist. 90(2-3):336–346. doi: 10.2138/am.2005.1449
- Putirka KD. 2008. Thermometers and barometers for volcanic systems. Reviews in Mineralogy and Geochemistry. 69(1):61–120. doi: 10.2138/rmg.2008.69.3
- Putirka KD, Perfit M, Ryerson FJ, Jackson MG. 2007. Ambient and excess mantle temperatures, olivine thermometry, and active vs. passive upwelling. Chemical Geology. 241(3-4):177–206. doi: 10.1016/j.chemgeo.2007.01.014
- Reay A, McIntosh PE, Gibson IL. 1991. Lherzolite xenolith bearing flows from the East Otago province: crystal fractionation of upper mantle magmas. New Zealand Journal of Geology and Geophysics. 34(3):317–327. doi: 10.1080/00288306.1991.9514469
- Rodriguez-Navarro C, Cultrone G, Sanchez-Navas A, Sebastian E. 2003. TEM study of mullite growth after muscovite breakdown. American Mineralogist. 88(5-6):713–724. doi: 10.2138/am-2003-5-601
- Roeder PL, Emslie R. 1970. Olivine-liquid equilibrium. Contributions to Mineralogy and Petrology. 29(4):275–289. doi: 10.1007/BF00371276
- Scanlan EJ. 2018. Paragenesis and geochemistry of scheelite deposits at Glenorchy, West Otago [master’s thesis]. Dunedin (New Zealand): University of Otago.
- Scanlan EJ, Scott JM, Wilson VJ, Stirling CH, Reid MR, Le Roux PJ. 2018. In situ 87Sr/86Sr of scheelite and calcite reveals proximal and distal fluid-rock interaction during orogenic W-Au mineralization, Otago Schist, New Zealand. Economic Geology. 113(7):1571–1586. doi: 10.5382/econgeo.2018.4603
- Scott JM, Brenna M, Crase JA, Waight TE, van der Meer QHA, Cooper AF, Palin JM, Le Roux P, Münker C. 2016. Peridotitic lithosphere metasomatized by volatile-bearing melts, and its association with intraplate alkaline HIMU-like magmatism. Journal of Petrology. 57(10):2053–2078. doi: 10.1093/petrology/egw069
- Scott JM, Hodgkinson A, Palin JM, Waight TE, Van der Meer QHA, Cooper AF. 2014. Ancient melt depletion overprinted by young carbonatitic metasomatism in the New Zealand lithospheric mantle. Contributions to Mineralogy and Petrology. 167(1):963. doi: 10.1007/s00410-014-0963-0
- Scott JM, Konrad-Schmolke M, O’brien PJ, Günter C. 2013. High-T, low-P formation of rare olivine-bearing symplectites in Variscan eclogite. Journal of Petrology. 54(7):1375–1398. doi: 10.1093/petrology/egt015
- Scott JM, Liu J, Pearson DG, Harris GA, Czertowicz TA, Woodland SJ, Riches AJV, Luth RW. 2019. Continent stabilisation by lateral accretion of subduction zone-processed depleted mantle residues; insights from Zealandia. Earth and Planetary Science Letters. 507:175–186. doi: 10.1016/j.epsl.2018.11.039
- Scott JM, Liu J, Pearson DG, Waight TE. 2016. Mantle depletion and metasomatism recorded in orthopyroxene in highly depleted peridotites. Chemical Geology. 441:280–291. doi: 10.1016/j.chemgeo.2016.08.024
- Scott JM, Pontesilli A, Brenna M, White JDL, Giacalone E, Palin JM, le Roux PJ. 2020. The Dunedin Volcanic Group and a revised model for Zealandia’s alkaline intraplate volcanism. New Zealand Journal of Geology and Geophysics. 1–20.
- Scott JM, Waight TE, Van der Meer QHA, Palin JM, Cooper AF, Münker C. 2014. Metasomatized ancient lithospheric mantle beneath the young Zealandia microcontinent and its role in HIMU-like intraplate magmatism. Geochemistry, Geophysics, Geosystems. 15(9):3477–3501. doi: 10.1002/2014GC005300
- Spera FJ. 1984. Carbon dioxide in petrogenesis III: role of volatiles in the ascent of alkaline magma with special reference to xenolith-bearing mafic lavas. Contributions to Mineralogy and Petrology. 88(3):217–232. doi: 10.1007/BF00380167
- Sprung P, Schuth S, Münker C, Hoke L. 2007. Intraplate volcanism in New Zealand: the role of fossil plume material and variable lithospheric properties. Contributions to Mineralogy and Petrology. 153(6):669–687. doi: 10.1007/s00410-006-0169-1
- Swanson SE. 1977. Relation of nucleation and crystal-growth rate to the development of granitic textures. American Mineralogist. 62(9-10):966–978.
- Tanaka T, Togashi S, Kamioka H, Amakawa H, Kagami H, Hamamoto T, Yuhara M, Orihashi Y, Yoneda S, Shimizu H, Kunimaru T. 2000. JNdi-1: a neodymium isotopic reference in consistency with LaJolla neodymium. Chemical Geology. 168:279–281. doi: 10.1016/S0009-2541(00)00198-4
- Tegner C, Wilson JR, Brooks CK. 1993. Intraplutonic quench zones in the Kap Edvard Holm layered gabbro complex, East Greenland. Journal of Petrology. 34(4):681–710. doi: 10.1093/petrology/34.4.681
- Timm C, Hoernle K, Werner R, Hauff F, van den Bogaard P, White J, Mortimer N, Garbe-Schönberg D. 2010. Temporal and geochemical evolution of the Cenozoic intraplate volcanism of Zealandia. Earth-Science Reviews. 98(1-2):38–64. doi: 10.1016/j.earscirev.2009.10.002
- Tullis J. 1970. Quartz: preferred orientation in rocks produced by dauphiné twinning. Science. 168(3937):1342–1344. doi: 10.1126/science.168.3937.1342
- Turnbull IM, Mortimer N, Craw D. 2001. Textural zones in the Haast Schist – a reappraisal. New Zealand Journal of Geology and Geophysics. 44:171–183. doi: 10.1080/00288306.2001.9514933
- Wang SJ, Teng FZ, Scott JM. 2016. Tracing the origin of continental HIMU-like intraplate volcanism using magnesium isotope systematics. Geochimica et Cosmochimica Acta. 185:78–87. doi: 10.1016/j.gca.2016.01.007
- Wellnitz AK, Scott JM, Martin CE, Palin JM, Stirling CH, Reid MR, Wombacher F, Craw D. 2019. Carbonation reactions and coupled element and isotope redistribution during shallow crustal gold mineralisation, New Zealand. Mineralium Deposita. 54(5):743–760. doi: 10.1007/s00126-018-0838-9
- Woodhead J. 2002. A simple method for obtaining highly accurate Pb isotope data by MC-ICP-MS. Journal of Analytical Atomic Spectrometry. 17(10):1381–1385. doi: 10.1039/b205045e
- Worden RH, Champness PE, Droop GTR. 1987. Transmission electron microscopy of pyrometamorphic breakdown of phengite and chlorite. Mineralogical Magazine. 51(359):107–121. doi: 10.1180/minmag.1987.051.359.10