ABSTRACT
Cenozoic geological evolution of New Zealand centres around the formation of Zealandia, a new continent that became detached from the eastern margin of Gondwana around 105 Ma. Spreading opened the Tasman Sea leaving a fragment of continental lithosphere, largely submerged, in the SW Pacific. Throughout the Cenozoic history, volcanism became an integral part of Zealandia. Continental lithosphere provided the basement for the volcanism, both onshore and offshore. Monogenetic volcanism was common throughout the Cenozoic. The availability of water was ubiquitous through surface water bodies (oceans and lakes) and various other terrestrial hydrous systems provided by the humid temperate climate of Zealandia. Hydrovolcanism, both explosive and non-explosive, has played a significant role in Zealandia's volcanic history resulting in volcano mega- architecture involving edifice geology and volcanic hazards. However, hydrovolcanism has commonly been overlooked in Zealandia's monogenetic volcanism context. Cenozoic monogenetic fields of Zealandia provide a unique laboratory and comparative analogy for other volcanic fields on Earth that are associated with low-lying terrestrial settings or shallow marine environments in a humid temperate climate.
Introduction
Hydrovolcanism refers to a process where magma and water or water saturated sediments interact either explosively or non-explosively during a volcanic event (Sheridan and Wohletz Citation1983). Recognition of hydrovolcanism is significant as it can be used as a proxy to determine the eruptive environment the magma entered (e.g. deep to shallow subaqueous, subaerial) (Gutmann Citation2002; Risso et al. Citation2008; Graettinger et al. Citation2013; Németh and Kósik Citation2020). In this regard, hydrovolcanism contains a signature of the environmental conditions at the time of magma emplacement. It thereby links either the broader environmental conditions of a region where the magmatism and volcanism took place or the environment created by the magmatic process itself; for instance upper conduit, vent or crater processes (Németh and White Citation2003; Kereszturi et al. Citation2011, Citation2014; Kereszturi and Németh Citation2011; Ross et al. Citation2011; Németh and Kósik Citation2020). Hydrovolcanism or hydromagmatism are commonly used as synonyms for phreatomagmatism (Valentine and White Citation2012; Németh and Kósik Citation2020). In recent years, however, phreatomagmatism has become associated with magma – water interaction driven volcanic eruptions such as those that typically occur in subaerial conditions and driven by Molten Fuel-Coolant Interactions (MFCI) (Lorenz Citation1987; White Citation1991; Németh and Kósik Citation2020). Hydrovolcanism can take place in any type of volcanic setting including subaqueous (e.g. shallow marine, deep marine, sub-lacustrine, subglacial) and subaerial (e.g. coastal plains, on flank of central volcanoes, along fluvial valleys, intramountain basins) environments and associated with small-volume or more complex, large volcanoes such as stratovolcanoes, composite volcanoes or calderas (Self Citation1983; Walker Citation1993; Wilson Citation1994; Giordano Citation1998; Muraviev et al. Citation1998; De Rita et al. Citation2002; Geshi et al. Citation2003; Mastin et al. Citation2004; Darragh et al. Citation2006; Edgar et al. Citation2007; Németh and Cronin Citation2008; Wong and Larsen Citation2010; Nagaoka and Okuno Citation2011; Swanson et al. Citation2012; Van Eaton et al. Citation2012). In recent years the recognition of hydrovolcanism in any volcanic system has evolved hugely. These include direct observations through experiments designed to understand cratering processes (Valentine et al. Citation2015; Graettinger et al. Citation2016; Macorps et al. Citation2016; Graettinger and Valentine Citation2017; Sonder et al. Citation2018) to field descriptions of the resulting magmatic-to-volcanic systems (Leat and Thompson Citation1988; Heiken et al. Citation2000; Valentine et al. Citation2017b; Graettinger Citation2018; Pedrazzi et al. Citation2018; Németh and Kósik Citation2020). Phreatomagmatism plays an important role of the style of volcanism of small-volume, dispersed volcanic centres, commonly associated with volcanic fields generated by monogenetic volcanism (Németh and White Citation2003; Seghedi et al. Citation2007; Wijbrans et al. Citation2007; Németh et al. Citation2010; Kereszturi et al. Citation2011; Kshirsagar et al. Citation2011; Mattsson and Tripoli Citation2011; Stárková et al. Citation2011; Buechner and Tietz Citation2012; Herrero-Hernandez et al. Citation2012; Ramon Avellan et al. Citation2012; Valentine Citation2012; van Otterloo et al. Citation2013; van Otterloo and Cas Citation2013; Valentine et al. Citation2017a). Monogenetic volcanism in this context defines volcanism that involves small-volumes of magma producing relatively simple volcanic edifices in a short time (Németh and Kereszturi Citation2015; Smith and Németh Citation2017). The significance of hydrovolcanism in the context of monogenetic volcanic field evolution is that the external water can influence the magma fragmentation in the case of explosive processes, hence the resulting eruptive products and volcanic macroforms (a term referring to a volcanic edifice regardless of the geo-environment – subaqueous vs subaerial) can be greatly affected (Kereszturi et al. Citation2011; Németh et al. Citation2012b; Agustin-Flores et al. Citation2014). In the past decade numerous case studies have used New Zealand examples to demonstrate the link between volcanic eruption style, the role of water in explosive volcanism and the interaction between the environment and the volcanism (Németh et al. Citation2012b; Kereszturi et al. Citation2014). As monogenetic volcanism involves a small magma volume in a single or a few magma batches, the presence of external water can dominate or influence the volcanic eruptions significantly and that can be recorded in the eruptive products (Agustin-Flores et al. Citation2014; Németh and Kereszturi Citation2015; Smith and Németh Citation2017). This approach has recently been used to define various volcano types in the context of monogenetic volcanism, and the effect of external water has been utilised as a measure of the influence the environment has on the resulting volcanic macroform and the facies architecture of the deposits. Using various configurations of the volume of the interacting magma and the influence of the environment (external water) a framework classifying various small-volume volcanic landforms has been developed (Smith and Németh Citation2017). This approach has been validated and proven its usefulness in regions where volcanism (magmatism) takes place in a geo-environment ranging from humid climatic conditions, to those located near sea level or entirely subaqueous. Throughout the Cenozoic, suitable conditions existed for preserving dispersed volcanic deposits in the New Zealand region. Consequently, there are excellent examples of hydrovolcanism ranging from fully subaqueous, emergent styles to subaerial phreatomagmatism. However, vegetation cover or incomplete exposure commonly hinder assessment of key sites, many of which are Quaternary and geologically young. In addition, offshore examples are commonly known only from indirect methods (e.g. geophysical measurements) and direct observation or sampling is limited. In this paper we provide an overview of various monogenetic volcanic fields in New Zealand and the evidence used to establish the hydrovolcanic origin of a volcanic succession. The paper will primarily focus on volcanic megaforms and textures (macro and micro) traditionally used to identify hydrovolcanism across various Cenozoic monogenetic volcanic fields in Zealandia.
Geological context of hydrovolcanism of monogenetic volcanic fields of Zealandia
New Zealand is located in an active plate margin defined by two opposing subduction fronts and associated volcanic arcs separated by the 400+ km long dextral strike-slip Alpine Fault (Cole and Lewis Citation1981; Brothers Citation1984, Citation1985; Ballance et al. Citation1985; Gaina et al. Citation1998; King Citation2000; Nicol et al. Citation2007) (A). The continental crustal fragments comprising New Zealand were separated from Gondwana in the late Cretaceous, after 105 Ma (Laird Citation1993; Sutherland et al. Citation2001; Laird and Bradshaw Citation2004; Nicol et al. Citation2007). The continental mass commenced drifting during active Tasman Sea rifting that started at c. 105 Ma, which was followed by breakup and seafloor spreading in the Tasman Sea from 83 Ma (Sutherland et al. Citation2001, Citation2017; Laird and Bradshaw Citation2004). In addition, in the Taranaki and West Coast, a second, later rift event occurred. These tectonic events culminated in the initiation of the convergence associated with the Hikurangi and Puysegur subduction zones and eventually lead to form the present-day Zealandia, including the current landmass of New Zealand (Sutherland et al. Citation2001; Segev et al. Citation2012; Mortimer et al. Citation2014). Dispersed volcanism has commonly occurred since the Late Cretaceous, but most of the rift related volcanoes are buried within sedimentary basins (Bischoff et al. Citation2020). These well-preserved Cenozoic volcanic successions tend to be restricted to distinct regions at different times (Timm et al. Citation2010) (). Most of the intraplate volcanism was basaltic (Timm et al. Citation2010).
Figure 1. Topographic and tectonic settings of the broader environment of New Zealand (Zealandia) with respect to the locations of Cenozoic monogenetic volcanism; Auckland Volcanic Field (AVF) (inset A), South Auckland Volcanic Field (SAVF) and Waikato coastline (W) (inset B), Taupo Volcanic Zone (TVZ) (inset C) and Central and East Otago with Waipiata Volcanic Field (WVF) and Dunedin Volcanic Complex (inset D). Bathymetric information of offshore Zealandia was derived from the 250 m resolution gridded bathymetric data (2016), National Institute of Water and Atmospheric Research (NIWA) (Mitchell et al. Citation2012). Tectonic and structural information from Mortimer et al. (Citation2017). Regional maps of the TVZ, South Auckland and Waikato region and the central Otago region were plotted to the 8 m NZ DEM (LINZ – Land Information New Zealand Citation2012), whereas the Auckland Volcanic Field was mapped on a 1 m LiDAR DEM (LINZ – Land Information New Zealand Citation2013).
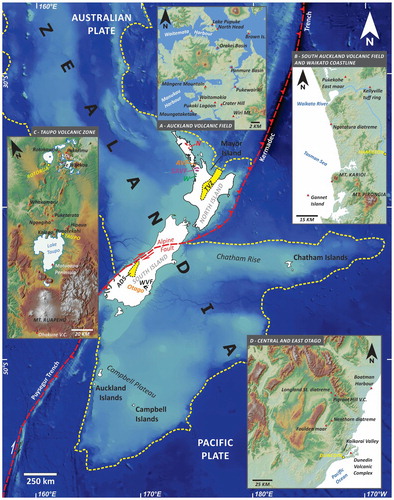
Some small volume, monogenetic felsic volcanoes also occurred close to plate boundaries; the most recent of these are associated with rifting in the Taupo Volcanic Zone (Wilson et al. Citation1995; Wilson and Rowland Citation2016; Kósik et al. Citation2018, Citation2019, Citation2020) (). Within the Taupo Volcanic Zone basaltic as well as more silicic, dacitic to rhyolitic volcanism, commonly resulted in the formation of small-volume, and likely short-lived, lava dome eruptions (Rooney and Deering Citation2014; Kósik et al. Citation2016; Wilson and Rowland Citation2016; Kósik et al. Citation2019). The explosive silicic volcanism commonly associated with Taupo also generated numerous silicic tuff rings that filled with lava domes (Brooker et al. Citation1993; Kósik et al. Citation2019).
Today New Zealand is part of a recently defined continent Zealandia [https://www.gns.cri.nz/Home/News-and-Events/What-s-new/The-origin-and-meaning-of-the-name-Te-Riu-a-Maui-Zealandia]. This continental mass, which is largely submerged today, fulfils the geophysical criteria for a continent. However, not all geoscientists agree with this interpretation and strong debate continues (Dowding and Ebach Citation2017). The stratigraphic framework of Zealandia can be grouped into two stratigraphical mega-units (Austral Superprovince and Zealandia Megasequence); the older Austral Megaunit is related to the geological evolution of the eastern Gondwana margin and includes the Eastern and Western terrain slices, defined as ‘provinces’ (Mortimer et al. Citation2014). The younger Zealandia Megasequence consists of the late Cretaceous to Cenozoic succession including the early Cenozoic terrestrial sedimentary units that infilled the rift basins created during the earlier rifting phase (Mortimer et al. Citation2014). These units were gradually covered by marine deposits as water depth increased in reponse to the gradual submergence of the continent through the early part of the Cenozoic (Mortimer et al. Citation2014). A large portion of Zealandia was submerged by late Oligocene coincident with the onset of extensive subaqueous volcanism forming shallow marine plateaus. Volcanism in a largely marine setting meant subaqueous to emergent volcanic successions inter-fingered with marine sedimentary rocks (Stilwell and Consoli Citation2012; Thompson et al. Citation2014).
Intraplate volcanism of various styles has taken place in Zealandia periodically throughout the Cenozoic. With the development of the modern New Zealand plate boundary in the early Miocene reuplift led to an archipelago developing into an ever-increasing landmass with shallowing of the marine conditions in the surrounding offshore regions. The resulting geo-environment included abundant Miocene to Pliocene volcanic centres dispersed from the Chatham Rise and Campbell Plateau to the present day Otago region in the South Island (Gamble et al. Citation1986; Cas Citation1989; Briggs and McDonough Citation1990; Kear Citation1994; Hoernle et al. Citation2006; Timm et al. Citation2010; Scott et al. Citation2013; Scott and Turnbull Citation2019) (). Many of these are well preserved. Direct sea floor dredging and geophysical surveys confirms the presence of dispersed volcanism to the east of the plate boundary in the south where a large number of mafic volcanoes occur offshore, on land (Timm et al. Citation2010) and out into the deep-submarine farther offshore (Uruski Citation2020). In eastern Otago, Paleogene submarine to emergent volcanism occurred in a shallow continental shelf setting, producing Surtseyan volcanic mounds and tuff cones the deposits of which are exposed along the present day coastline, (Price and Coombs Citation1975; Coombs et al. Citation1986, Citation2008; Cas et al. Citation1989; Németh et al. Citation2003; Price et al. Citation2003; Sorrentino et al. Citation2011; Fox et al. Citation2015; Moorhouse et al. Citation2015; Moorhouse and White Citation2016; Jones et al. Citation2017; McLeod and White Citation2018; Scott et al. Citation2020). The most extensive group of volcanoes formed during the Cenozoic in the Otago region are the continental alkaline basaltic volcanoes centred on the Dunedin area. Known as the Dunedin Volcanic Group (Scott et al. Citation2020) it includes, the Waipiata Volcanic Field (WVF) and the Dunedin Volcanic Complex (DVC) (Coombs et al. Citation1986). In recent work (Scott et al. Citation2020) it is proposed that the Waipiata Volcanics/Waipiata Volcanic Field be replaced with Dunedin Volcanic Group, since it is actually not easy to subdivide Waipiata from the Dunedin Volcano as they overlap in age, chemistry, isotopic properties and were only distinguished by Coombs et al. (Citation1986) as ‘where the main flows end’, which is ambiguous.
There are several onshore Cenozoic volcanic fields in the southern part of the South Island. One of the largest and best preserved is the Miocene Waipiata Volcanic Field (WVF), an eroded intracontinental volcanic field (Németh and White Citation2003) adjacent to the Dunedin Volcano (or Dunedin Volcanic Complex after Coombs et al. Citation1986).
The pre-volcanic Cenozoic units consist of non-marine and marine clastic sediments deposited on an early Cretaceous erosional surface cut into schist basement (Bishop and Turnbull Citation1996) and successively overlain by thick Oligocene transgressive deposits (Bishop and Turnbull Citation1996). These marine units consisting of generally fine-grained glauconitic sandstones and siltstones are well preserved in the northern part of the Waipiata Volcanic Field (Németh and White Citation2003). The contacts of the volcanic conduits exposed today display cross-cutting relationships with the pre-volcanic marine units. The area re-emerged in the early Miocene in response to transpressional tectonics associated with the inception of the Alpine Fault and marked by the first non-marine (terrestrial fluvio-lacustrine) deposits (Mortimer et al. Citation2014). This geological setting for intraplate volcanism in the South Island is the perfect geo-environment in which to have rising magma interact with external water, either en-route at shallow crustal level or with surface waters such as rivers, lakes or shallow marine bays. The known pre-volcanic lithologies, sand, silt or gravelly beds, are good aquifers and are, in places, closely associated with carbonate rocks, some with extensive fracture networks. Vent alignments have also been recognised suggesting the presence of strong structural control on the distribution of volcanism. This structural fabric likely manifested in the formation of elongated fluvial networks providing broad water-rich environments. Later on, farther north intraplate volcanism developed west of the plate boundary forming a laterally extensive zone of dispersed volcanic fields including the monogenetic volcanic fields from south Waikato region through to Northland (Briggs and McDonough Citation1990) (A). This volcanism developed in a low-lying terrestrial to shallow subaqueous environment forming volcanoes typical of emergent Surtseyan style to more terrestrial type of volcanoes including tuff rings, maars and scoria cones commonly associated with extensive outpourings of lava (Goles et al. Citation1996; Briggs et al. Citation1997; Cook et al. Citation2003, Citation2005; Smith and Briggs Citation2008; Ilanko et al. Citation2009; Gibson et al. Citation2010).
Buried late Miocene volcanoes are inferred to exist east of Dunedin and the Campbell Plateau (Adams Citation1981; Gamble et al. Citation1986; Gamble and Thomson Citation1990; Hoernle et al. Citation2006). Large shield volcanoes similar to the Dunedin Volcano exist in the south at the Auckland Islands (Carnley and Ross volcanoes) (Gamble and Adams Citation1985; Gamble et al. Citation1986) and Campbell Island (Morris Citation1984). Intraplate volcanism is reported from the Antipodes Islands (Scott et al. Citation2013) and the Chatham Islands (Eocene - Oligocene/40 - 35 Ma and Miocene – Pliocene/6 - 2.6 Ma) (Hoernle et al. Citation2006). Recent work on the intraplate volcanoes of the South Island, Chatham Rise and Campbell Plateau suggests volcanism occurred intermittently over a large area throughout the Cenozoic, with no recognisable lineament array (Hoernle et al. Citation2006; Timm et al. Citation2010) or melting of metasomatised lithospheric mantle (Panter et al. Citation2006; van der Meer et al. Citation2017).
Currently there are two concurrent models to explain the origin of Zealandia intra-plate volcanism. Some authors relate it to the presence of a mantle plume that initiated during the final stage of eastern Gondwana breakup (Panter et al. Citation2006; van der Meer et al. Citation2017) while others ascribe volcanism to asthenospheric upwelling induced by removal of parts of the subcontinental lithosphere throughout the Cenozoic (Hoernle et al. Citation2006; Timm et al. Citation2010).
Scales of observation used to recognise hydrovolcanism in the New Zealand geological record
The determination of whether or not magma fragmentation was influenced or directly triggered by magma and water interaction is challenging as there are no tools available to directly test it. Evidence of the action of hydrovolcanism during magma fragmentation is usually indirect often from a common set of micro- and macro-textural features consistent with magma and water interaction including micro and macro textural features of on the chilled margins of pyroclasts, presence of accretionary lapilli and the dominance of pyroclastic density current deposits rich in accidental lithic clasts derived from conduit walls at the crater rim (Németh and Kósik Citation2020). The problem is perfectly highlighted in a recent review pointing it out that ‘absence of evidence is not evidence of absence’ (White and Valentine Citation2016). This statement highlights the problem that even if we cannot see direct evidence that hydromagmatic processes took place during a volcanic eruption that is not evidence to say it didn't happen (White and Valentine Citation2016). In this paper we will restrict ourselves to a list ‘common wisdoms’ used to identify features generated by hydrovolcanism-associated with eruptions of small volume volcanoes in the New Zealand context. There are at least three levels of scale of observations used in New Zealand volcanology to establish magma-water influenced eruption styles in the Cenozoic. These are based on landscape level recognition of volcanic features and macroforms that can be linked to hydrovolcanism, outcrop scale lithofacies and facies relationships in both a vertical and lateral sense, and microtextural works focusing on juvenile pyroclast textures that can be associated with MFCI processes. Unfortunately none of the above mentioned observations can provide unique and decisive arguments to establish hydrovolcanic origin of an eruption sequence of a volcano, volcanic erosion remnant or volcanic successions in the geological record (Lorenz Citation1987; Graettinger et al. Citation2015; White and Valentine Citation2016; Amin and Valentine Citation2017; Sonder et al. Citation2018; Németh and Kósik Citation2020). Recent research is heavily focused on finding a link between eruption styles and resultant volcanic macroforms and deposits. Here we provide some common methods applied in New Zealand in recent years to establish phreatomagmatic origin of volcanoes and volcanic successions.
Volcanic macroform-scale evidence
The macroforms of small volume volcanoes such as maar craters (A), tuff rings (B) or tuff cones (C) are commonly used to infer hydrovolcanic processes responsible for the growth of those macroforms (Morrissey et al. Citation2000; Brand and Brož Citation2015; Brož and Németh Citation2015; De Hon Citation2015; de Silva and Lindsay Citation2015; Valentine and Connor Citation2015; White et al. Citation2015). Recent research, however, has demonstrated that the process of cratering, for instance the formation of a maar, should be looked at as process creating the volcanic macroform that may or may not be linked to an explosive magma – water interaction hence hydrovolcanism, or more specifically to phreatomagmatism (Graettinger et al. Citation2015, Citation2016; Graettinger and Valentine Citation2017). Here we use maar as a volcanic landform that consists of a flat floored crater cut into the syn-volcanic landscape (even if it is rugged) creating a ‘hole-in-the-ground’ morphology (Christenson et al. Citation2015; Graettinger Citation2018; Németh and Kósik Citation2020), without linking it directly to explosive phreatomagmatic eruptions. Some reports suggest that dry maar craters may form in response to a gas-rich magma subterranean explosion (Mattsson Citation2012; Berghuijs and Mattsson Citation2013; Balashova et al. Citation2016). However, the preserved tephra rings that surround a maar crater show many microtextural features indicative of magma and water interaction, hence phreatomagmatism (Graettinger Citation2018; Ort et al. Citation2018).
Figure 2. A – Pukekohe East maar (PEM) from the air (South Auckland Volcanic Field), Note the flat crater floor and the smooth surface of the tephra ring surround the maar crater; B – Overview of the Motukorea/Brown Island (Auckland Volcanic Field) complex tuff ring (tr). Note the scoria cone (sc) complex filling the crater of the tuff ring.; C – Overview of the Maungauika/North Head tuff cone (Auckland Volcanic Field) from the east. The core of the tuff cone (tc) is preserved, its outer edifice has been shoaled away. The centre of the tuff cone is filled with a scoria cone (sc); D – Overview or the preserved part of the Foulden Maar (Waipiata Volcanic Field). The image was taken from the north. Small quarry operation of diatomite is in the middle of the depression (red arrow). In the left side of the view an erosional remnant of a scoria cone (Bald Hill) sitting on the tuff ring.
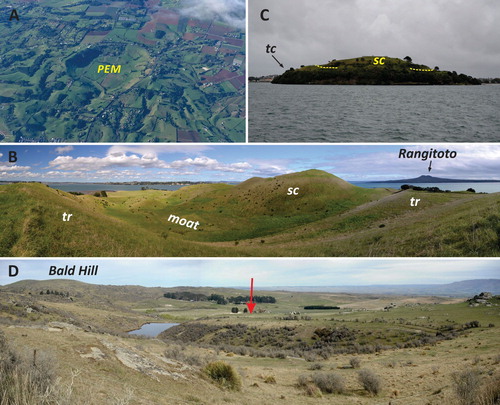
Erosional remnants of scoria cones, tuff rings and maars of the Waipiata Volcanic Field (WVF) form a characteristic landscape comprising volcanic buttes and knobs (D). Cenozoic sedimentary units are mainly preserved along the northern margin of the WVF and not in the central, uplifted fault/fold block areas due to the presence of thick lava flows (Bishop and Turnbull Citation1996). The only direct evidence of their previous existence or absence comes from studying the pyroclastic deposits preserved in various volcanic pipes, diatremes, in volcanic fields like the Waipiata Volcanic Field (Németh and White Citation2003).
The WVF is an example of an eroded monogenetic volcanic field where shallow surface water and multiple groundwater tables facilitate water-influenced small-volume volcanism including explosive phreatomagmatic eruptions (Németh and White Citation2003). This field includes erosional remnants of at least 55 volcanoes in an area recently revised to 7800 km2 (Scott et al. Citation2020). The original small-volume volcanoes, and their associated lava fields, are preserved in the various vent-filling, intra-crater and proximal pyroclastic successions within tuff rings and suspected maar craters (Németh Citation2003).
Eroded volcanic edifices are also common in younger volcanic regions such as the Taupo Volcanic Zone (C). Here entire small-volume volcanoes are commonly covered by thick, younger ignimbrite or reworked volcaniclastic successions. These have been exposed by river downcutting or quarrying. A section of a suspected diatreme is exposed in the interior of the K-trig basalt quarry near Taupo (C) (Brown et al. Citation1994), where a dish-like feature composed of accidental-lithic-rich basaltic tuff overlying coarse breccia infilling a conduit is exhumed (A). Similarly, a previously unknown volcanic centre, Te Hukui diatreme, has been discovered near Taupo. This basaltic lapilli tuff and tuff succession forms an odd hill-side associated with a younger silicic tuff ring and maar chain and is interpreted as a remnant of a diatreme (Kósik et al. Citation2017) (B). In both cases the pyroclastic rocks of the preserved successions are dominated by glassy juvenile lapilli and ash particles hosted in a fine-grained accidental-lithic rich matrix, suggesting intensive excavation of country rocks most probably by explosive magma-water interaction (C & D).
Figure 3. A – A small diatreme is exhumed as the base of the Punatekahi volcanic complex (Taupo Volcanic Zone). Note the v-shaped architecture of pyroclastic rocks in the base of the section (white dashed lines), marking an exposed diatreme (d).; B – Te Hukui Basalt formation exposing typical phreatomagmatic pyroclastic successions in a well-defined small area inferred to be a diatreme near the Puketerata Volcanic Complex, Taupo Volcanic Zone (note people for scale); C – pyroclastic rocks recovered from the Punatekahi diatreme is composed of glassy juvenile ash and lapilli and abundant accidental lithic clasts forming a bedded, cross-bedded succession inferred to accumulated by base surges; D – Accretionary lapilli-bearing basaltic lapilli tuff and tuff succession from the middle section of the Te Hukui diatreme.
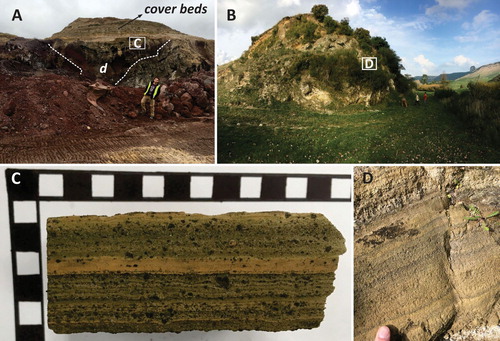
A basaltic diatreme, Ngatatura diatreme (Heming Citation1980), exposed on the Waikato coastline, (A) has been K-Ar dated at 1.83–1.54 Ma (Briggs et al. Citation1989; Briggs et al. Citation1994, Citation1997). However, new K-Ar dating reporting ages of 3.34 ± 0.06–1 Ma are well outside of the commonly accepted Ngatatura ages and similar to those known from Okete Formation and Alexandra Volcanics (Van Niekerk Citation2016). The diatreme itself is dominated by pyroclastic breccias (B) and cannot be linked to any surface expression of a volcano nearby (van Niekerk et al. Citation2015b). A recent study has revealed the rock defined as Ngatatura diatreme is part of a complex volcano with a history of multiple events potentially spanning a few hundreds of thousands of years and contributing to a record of dramatic changes in the coastal region where near-sea level, coastal conditions have dominated and controlled small volume volcanism over time (van Niekerk et al. Citation2014, Citation2015a). As a result, the Ngatatura diatreme and enclosing deposits reveal a history of shallow, subaqueous volcanism that produced hyaloclastite (C) and pillow lavas subsequent to the diatreme formation (Van Niekerk Citation2016).
Figure 4. A – Overview of the Ngatatura diatreme (d) from the NW located along the Waikato coastline (D). Note the steep diatreme wall (white dashed lines) that slightly enlarged upsection. The cliff face is about 60 m high; B – Close up view of the pyroclastic breccia of the Ngatatura diatreme rich in angular glassy juvenile pyroclasts (j) and variable rounded sedimentary (s) rocks from the pre-volcanic deposits; C – Over the Ngatatura diatreme shallow marine sediments (ms) intercalated with a hyaloclastite breccia (hy) suggesting a younger eruptive phase in the same area where the diatreme formed previously.
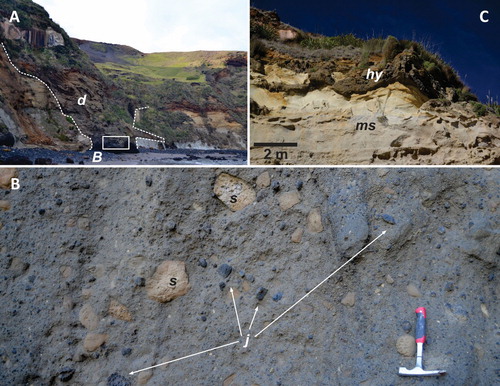
Evidence of magma-water interaction-driven explosive eruptions that can be likened to Surtseyan style eruptions (e.g. explosion taking place within a water body, while the eruption cloud pierces the water surface) are abundant in New Zealand. Along the Waikato Coastline, near Ngatatura diatreme, a complete half section of a tuff cone is exposed with its monotonous pyroclastic succession including abundant dunes and soft sediment deformation (A). In the offshore Gannet Island/Karewa a half section of a nephelinitic tuff ring has been reported as a hydrovolcanic edifice (Briggs et al. Citation1997). Similar half sections are known from East Otago, such as those on the Boatmans Harbour in Oamaru (B), or in Cape Young at Chatham Islands (C) and interpreted to be result of shallow subaqueous eruptions that gradually built emergent hydrovolcanic edifices through Surtseyan style eruptions (Cas et al. Citation1989; Maicher Citation2003; Corcoran and Moore Citation2008; Sorrentino et al. Citation2011; Stilwell and Consoli Citation2012; Sorrentino et al. Citation2014; Agustin-Flores et al. Citation2015; Moorhouse et al. Citation2015). Another interesting example documented for its characteristic facies architecture, is Northhead in the Auckland Volcanic Field which is defined as a Surtseyan volcano formed in very shallow water (30 metres maximum) (Agustin-Flores et al. Citation2015) (D). Kaiapo volcano, a basaltic tuff cone in the Taupo Volcanic Zone, has been reconstructed on the basis of characteristic blocky pyroclasts (B) in a 500 m long, albeit dissected exposure consisting of a 50 m thick monotonous, palagonite tuff succession (Brown et al. Citation1994) (A) exposed along the Kaiapo Fault near Taupo. Not far from Kaiapo, poorly exposed basaltic tuffs occur in shoreline cliffs at Acacia Bay, Lake Taupo. These are interpreted as an erosional remnant of a phreatomagmatic tuff ring that formed in subaerial conditions (Wilson and Smith Citation1985). These tuff ring deposits are dominated by pumiceous lake sediments mixed with 15–20 volume % juvenile glassy basaltic ash and lapilli deposited by pyroclastic fall and surge (Wilson and Smith Citation1985). None of these volcanoes are well preserved nor do they show their original landform geometry. Their hydromagmatic origin and potential Surtseyan type edifices are inferred on the basis of their pyroclastic texture and facies architecture as outlined in the next section. Ohakune Volcanic Complex, on the southern Ruapehu ring-plain, is a small volcanic field composed of at least four volcanic craters. One of them is being actively quarried and since the first documentation of this complex tuff ring and multiple scoria cones (Houghton and Hackett Citation1984), new exposures provide insight into the volcano's evolution (Kósik et al. Citation2016). During its emplacement this scoria cone was influenced by shallow water entering the erupting vents that changed their locations as the edifice grew. However, a complete ‘wet’ eruption history is not preserved. This is a common scenario in large scoria cones with initial phreatomagmatic tuff ring basement (Murcia et al. Citation2015). The tuff ring at Ohakune shows evidence of magmatic fragmentation generating lava spatter dominated dark horizons (C) that are commonly interbedded with fine ash often accompanied by dewatering pipes and plastering effects (D).
Figure 5. A – Half section of a Surtseyan tuff cone along the Waikato coastline, just south of Karioi Mountain. Note the strong erosional features, u-shaped channels (u), and the dune-bedded pyroclastic succession typical for an emergent volcano, commonly defined as a result of Surtseyan style eruptions (hammer is for scale); B – Various distal beds of eruption fed density currents at Boatman Harbour in Oamaru as part of a complex emergent volcano. Soft sediment deformation (ss) features are apparent; C – Half section of a Surtseyan volcano with thick pyroclastic succession (pyx) at Cape Yoiung, Chatham Island intruded by feeder dykes with peperitic margin (d), section is about 70 m high; D – Lower sequence of the pyroclastic succession of the Maungaika/North Head Surtseyan volcano at the Auckland Volcanic Field.
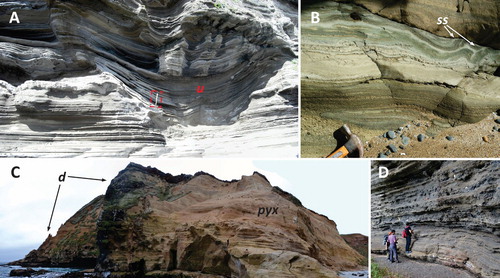
Figure 6. A – Overview of the half section pyroclastic succession of an emergent volcano (Kaiapo) near the city of Taupo, Taupo Volcanic Zone (hammer is for scale). Arrows indicate coarse-grained juvenile pyroclast-rich layers; B – Lapilli tuff from the middle section of the pyroclastic succession of the Kaiapo volcano. Larger angular juvenile, low-vesicularity pyroclasts arrowed with white. Some orange-brown coloured pyroclasts are juvenile palagonitized pyroclasts (yellow arrows); C – Pyroclastic succession of the Ohakune Volcanic Complex exposes alternating magmatic explosive (m) and phreatomagmatic explosive (ph) pyroclastic deposits (Taupo Volcanic Zone); D – Fine grained tuff of phreatomagmatic origin at Ohakune Volcanic Complex basal section with vertical pipes (arrows).
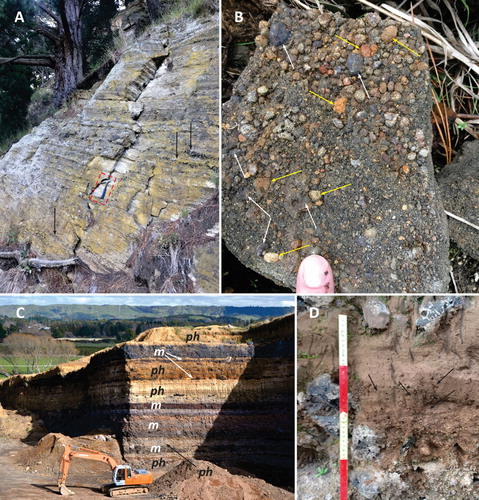
Outcrop-scale evidence
At outcrop scale pyroclastic deposits that can be inferred to have originated from pyroclastic density currents (PDC) include base surges which are considered indirect indicators for phreatomagmatism (Valentine et al. Citation2017b). The recognition of abundant PDC deposits is another indirect line of evidence for hydrovolcanic explosive eruptions. PDC-generation is a common process documented with violent, explosive hydrovolcanic eruptions in young sites or directly observed and recorded from known eruptions (Self et al. Citation1980; Geshi et al. Citation2003; Doronzo et al. Citation2017; Ort et al. Citation2018; Sweeney et al. Citation2018). However, in recent explosive eruptions identifying the pyroclast textures that may reflect magma-water explosive interaction is compounded by the difficulty identifying juvenile pyroclasts (Pardo et al. Citation2014).
In the WVF, preserved and exposed pyroclastic successions along with the common volcanic landform elements were used to group volcanic rocks to specific vent types (Németh and White Citation2003). Detailed facies architecture of the preserved volcanic erosion remnants and the type of volcanic successions preserved in association with the vents were classified into 3 types (Németh and White Citation2003). Type 1 vents are inferred to be the remnants of scoria cones and are dominated by scoriaceous juvenile lapilli (Németh and White Citation2003). Occasionally small outcrops of chaotic lapilli tuffs with glassy juvenile pyroclasts and accidental lithics are preserved along dykes indicating that while Type 1 vents likely represent former scoria cones, we cannot rule out that phreatomagmatism was, at times, involved in the eruptions during edifice growth (Németh and White Citation2003). Recent studies in young Quaternary volcanic fields elsewhere have found similar features on scoria cones and interpreted them as showing the scoria cones had a short phreatomagmatic phase during their initial eruption phase (Murcia et al. Citation2015). Vent successions dominated by pyroclastic infill are classified as Type 2 vents and are inferred to have been the substructures of phreatomagmatic tuff ring and/or maar volcanoes. Typical pyroclastic facies are non-volcanic-lithic rich, grain- or matrix-supported, massive tuff breccias and lapilli tuffs that consist predominantly of accidental lithic blocks and lapilli (A). These facies are often interbedded with unsorted, matrix-supported, diffusely stratified tuff breccia and lapilli tuff beds. Tuff breccias and lapilli tuff rocks with abundant accidental lithic content are volumetrically dominant in these vent remnants and are interpreted to be vent-filling pyroclastic units formed by phreatomagmatic explosive eruptions. Thinly bedded, locally scour-fill, cross stratified, accidental lithic-rich lapilli tuff and tuff beds form decimetre scale domains with uniform bedding dipping toward the centre of the vent. These domains are enclosed within other pyroclastic rocks and indicate syn-eruptive collapse and/or subsidence; these are commonly claimed processes in the formation of maar-diatremes (Lorenz Citation1986, Citation1987, Citation2007; White Citation1991; Suhr et al. Citation2004; Suhr et al. Citation2006; Lorenz and Kurszlaukis Citation2007; Valentine et al. Citation2015; Kurszlaukis and Lorenz Citation2016; Lorenz et al. Citation2017). The sedimentary textures of the facies suggest deposition from base surges. Many of the pyroclastic rocks recovered from Type 2 vents show evidence of sudden cooling of pyroclasts at every scale suggesting that they formed due to explosive magma – water interaction. The low vesicularity and the blocky shape of the fine lapilli to ash fractions also support fragmentation driven by external forces. Type 3 vent complexes are groups of closely spaced or overlapping vents, many share similar pyroclastic textures to those preserved in Type 2 vents suggesting phreatomagmatic fragmentation. Many of the Type 3 vents are associated with thick and extensive lava flow fields that commonly show intra-crater facies characteristics. Type 3 vents, such along the Pigroot Hill along the Palmerston – Kyeburn SH85, are interpreted to be the remnants of volcanoes comprising adjoining to coalescing maars and tuff rings with explosive and effusive magmatic products (B). These volcanic centres often had simultaneous eruptions from more than one of their vents, producing intercalated, mostly proximal beds of explosive and effusive products. Hence, a Type 3 vent complex is a group of coalesced Type 2 and/or Type 1 vents. Type 3 vent complexes locally include deposits formed on the paleo-ground surface adjacent to the volcano and thus represent the best preserved, least eroded volcanic remnants in the field. Some of these (for instance tuff units characterised by dune-bedding and/or accretionary lapilli and/or vesiculated tuff) are typical of medial to distal deposits of phreatomagmatic tuff rings. Different types of scoriaceous, structureless to weakly bedded, tuff breccia, lapilli tuff, and tuff beds are preserved in thick piles (>10 m) as capping units, indicating a ‘dry’ explosive magmatic phase preceded or accompanied the effusion of the lava. Clastogenic lava flows are commonly preserved in dish-like structures. Spatter-rich pyroclastic beds are intercalated with thinly bedded sideromelane-rich pyroclastic beds, suggesting simultaneous deposition from magmatic and phreatomagmatic activity at closely spaced vents (Houghton and Hackett Citation1984; Houghton and Schmincke Citation1986; Houghton et al. Citation1996; Kósik et al. Citation2016). The pyroclastic deposits of most of the Waipiata vents record initial explosive phreatomagmatic activity fuelled by groundwater, followed by Strombolian-style eruptions (Németh et al. Citation2012a).
Figure 7. A – Pyroclastic breccia rich in accidental lithic fragments from an exhumed diatreme near Longland Station, Swinburn Plateau (Waipiata Volcanic Field). View is about 20 cm across; B – Base surge units of the Pigroot Hill Volcanic Complex along the Red Cutting Summit (Waipiata Volcanic Field). Camera bag is for scale (12 cm long); C – Ballistic bomb (arrow shows impact direction) causing impact sag on the phreatomagmatic pyroclastic successions of Motukorea/Browns Island (Auckland Volcanic Field). Pen is 14 cm long; D – Cauliflower-shaped bombs (arrows show impact directions) from the basal section of the Orakei Basin maar (Auckland Volcanic Field). Arrows point to the impact direction (50c coin is for scale).
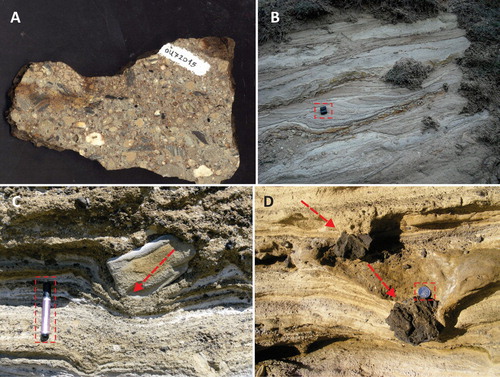
Younger monogenetic fields such as those located in part of the South Auckland, Auckland and Northland Volcanic Fields contain several well-preserved but normally covered and poorly exposed volcanic landforms typical for phreatomagmatic explosive eruptions. These include shallow maars and tuff rings of unusual scoria cones with intermittent phreatomagmatic explosive phases (Hopkins et al. Citation2020). Younger Cenozoic volcanic fields, mostly in the northern part of the present-day North Island provide opportunity for more detailed geological work to establish the important role of hydrovolcanism and phreatomagmatism in the formation of their volcanoes (Kereszturi et al. Citation2014). The Quaternary Auckland Volcanic Field is considered to be active and studies to date recognise that explosive phreatomagmatic eruption styles have occurred in nearly all of its known vents (Kereszturi et al. Citation2014). This has formed a strong basis for current volcanic hazard studies as Auckland is New Zealand's largest city and the economic powerhouse of New Zealand which is located directly over of this active volcanic field (Lindsay et al. Citation2011; Kereszturi et al. Citation2013, Citation2014; Le Corvec et al. Citation2013; Blake et al. Citation2017; Leonard et al. Citation2017; Hayes et al. Citation2018; Hopkins et al. Citation2020). Pyroclastic successions from at least 45 of the currently known 55 vents starts with a typical lapilli tuff and tuff deposits considered to be the product of explosive magma – water interaction (Kereszturi et al. Citation2014). In some cases, such as the Brown Island (Motukorea), Orakei Basin, Waitomokia or Mangataketake the initial pyroclastic successions are typically rich in accidental lithic bombs and blocks from the country rock immediately underlying the region (C & D). The initial pyroclastic units consist of plastically deformed, fine-grained tuff beds consistent with deposition in a water-saturated environment. These are ballistic bombs which impacted the underlying pyroclast horizons and are commonly large accidental lithics. In addition, complex cauliflower textured bombs, that commonly enclose xenoliths of sedimentary country rock are also more common in the basal part of the pyroclastic succession, however, more work is required to understand this relationship. The basal layers are generally less than a metre thick and change up-section into alternating coarse-fine-coarse lapilli tuff and tuff beds rich in lapilli strings, scour fill, low angle cross stratification or dune bedding all indicating transportation involving water; base surges are most likely to be responsible for their development (A & B).
Figure 8. A – Dune-bedded base surge deposits in the mid-section of the Motukorea/Browns Island tuff ring (Auckland Volcanic Field). Arrow shows transport direction, hammer is for scale; B – Climbing dunes of a base surge succession in the upper section of the tuff ring of the Lake Pupuke maar pyroclastic deposits, Auckland Volcanic Field (Arrow shows transport direction); C – Weathered, palagonitized dune bedded phreatomagmatic tuff from a tuff ring of Pukewairiki/Highbrook Park (Auckland Volcanic Field) (Arrow shows transport direction); D – Weathered accretionary lapilli-rich phreatomagmatic tuff from the southern crater rim of Pukekohe East maar (South Auckland Volcanic Field)
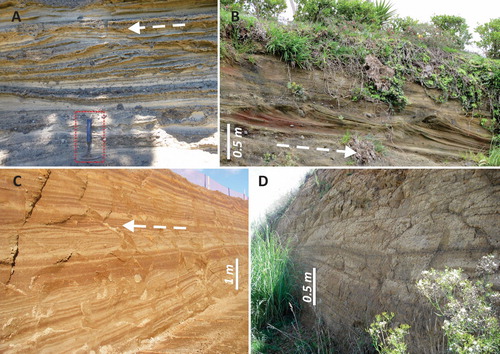
In well-preserved sections accretionary lapilli beds are recognised and their sedimentology records the involvement of magma-water interaction. In the Auckland and South Auckland Volcanic Fields, fine tuff beds are commonly, almost exclusively, composed of accretionary lapilli. These units display thin, parallel bedding dominating the upper part of the pyroclastic successions. In some cases, despite the young ages of most of the Auckland volcanoes, the tephra rings are covered and poorly exposed. Once exposed, the pyroclastic units weather quickly and intensely. However, on freshly exposed surfaces, most common in the Auckland and South Auckland regions, weathering enhances the texture of dune-bedded tuff successions (C & D). Weathering of tuffs can make it difficult to establish the eruption styles responsible for the formation of the pyroclastic successions.
The upper sections of the exposed pyroclastic units of Auckland's volcanoes are composed of fine-grained matrix-rich tuff with common clusters of ballistic impact bombs. These beds are almost exclusively composed of fine-grained matrix derived from the underlying fine-grained siliciclastic sediments. From the Auckland and South Auckland region, where thick (10 m +) pyroclastic sections are preserved and accessible, the main part of the succession clearly shows repetitive fine- and coarse-grained pyroclastic bed alterations without significant visible juvenile and accidental lithic content variations. Most of the sections have some characteristically different types of pyroclastic interbedding such as thick scoriaceous units (e.g. Brown Island/Motukorea) (A). The top sections of all the known volcanoes across Auckland and South Auckland have a magmatic cap. This gradually develops from the underlying tuff and lapilli tuff dominated successions leaving a transitional zone only a few metres thick (B & C). In places the magmatic caps culminate in a coherent lava succession with a clastogenic character in the base (D). Across the Auckland and South Auckland region to date no volcano where the magmatic cap is covered by a new pyroclastic succession similar in texture, componentry and appearance to those forming the basal successions of the same volcanoes have been observed.
Figure 9. A – Nearly a metre thick scoriaceous bed (s) in the middle of the phreatomagmatic succession of Motukorea/Browns Island tuff ring indicates intermittent changes in the eruption style during the edifice growth of the tuff ring (Auckland Volcanic Field). Note the normal fault that dissect the section (arrows); B – The Motukorea/Browns Island tuff ring is capped by a thick scoriaceous lapilli and agglomeratic unit (white dashed line) indicating the complete eruption style changes to magmatic explosive in the end of the volcanic activity. Note the scoria cone (sc) in the background filling the crater of the tuff ring (tr); C – Well-exposed section at Te Manurewa o Tamapahore/Wiri Mt tuff ring (Auckland Volcanic Field) showing a capping magmatic succession (m) over the phreatomagmatic pyroclastic units (ph); D – Complex magmatic capping unit at Te Manurewa o Tamapahore/Wiri Mt showing gradual eruption style changes and the growth of a complex lava spatter and scoria cone intercalated with clastogenic lava flows (s1-s2-s3) and the entire section covered by lava flows (lf).
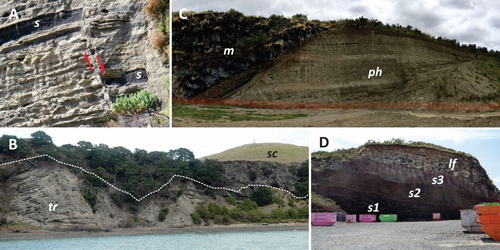
Micro-scale evidence
Undoubtfully the strongest supporting evidence for phreatomagmatic fragmentation are microtextures preserved in the pyroclasts. Juvenile pyroclast vesicularity, vesicle shape and bubble density are the strongest evidence supporting explosive magma-water interaction. The textural characteristics, such as microtextures in fine grained pyroclasts can be used as strong evidence of rapid cooling and brittle fragmentation (Zimanowski et al. Citation1997a, Citation1997b; Buettner et al. Citation1999, Citation2002). These clasts are typically a few tens of microns across and are inferred to have been derived from the interaction zone where the MFCI took place. Such clasts are commonly referred to as active particles from the MFCI perspective and supports explosive magma-water interaction during eruptions (Heiken Citation1972, Citation1974; Aramaki et al. Citation1986; Zimanowski et al. Citation1997b; Buettner et al. Citation1999, Citation2002; Morrissey et al. Citation2000; Mastin Citation2007; Németh Citation2010; Németh and Cronin Citation2011; Duerig and Zimanowski Citation2012; Jordan et al. Citation2014). The MFCI processes are associated with the finest fraction of juvenile pyroclasts inferred to be particles derived from the interaction zone between molten fuel (magma) and external coolant (water or water saturated sediment) (Zimanowski et al. Citation1997b) (0A & B). Textural and sedimentary indicators, such as ash aggregates, accretionary lapilli or vesicular tuffs, are thought to form during phreatomagmatic explosive eruptions (C). Their presence and abundance in a tephra layer, however, indicates moist conditions in the transporting agent (either fall or PDC) suggesting presence of water which transforms to steam in the heat of the eruption and then forms ash aggregates. Similarly, the presence of abundant cauliflower shape lapilli and bombs in the pyroclastic deposits supports the presence of water prior to the fragmentation of magma forming rapidly cooled and contracted pyroclasts (Lorenz Citation1986, Citation1987) (D). Most of the rocks of the facies contain sideromelane with various degrees of palagonite around the rim, low vesicularity and microlite content all indicative for fast cooling rate of the pyroclasts due to hydrovoclanism (Heiken and Wohletz Citation1986) (A). Most of the pyroclastic products of hydrovolcanic eruptions contain a small proportion of opaque volcanic glass called tachylite that cooled slower than sideromelane glass, hence indicating variable chilling and cooling conditions in a complex eruption cloud (B). The vent remnants are commonly topped with pyroclastic units rich in lava spatter. These are inferred to indicate exhaustion of the water supply to the explosion sites and hence cessation of phreatomagmatic fragmentation. A sudden increase in mantle-derived xenoliths in the top of the pyroclastic succession prior to the appearance of the magmatic capping units is documented in the WVF suggesting relatively rapid upward movement of the magma in the later stages of the eruptions. Many of the pyroclastic successions of the Cenozoic monogenetic volcanic fields across New Zealand are dominated by pyroclastic rocks rich in accidental lithic fragments (A). On micro-scale tuffs are commonly high in minerals or rock fragments typically derived from the underlying country rocks (Németh et al. Citation2007; Hencz et al. Citation2017) (B). Large juvenile lapilli or bombs often enclose blocky accidental lithic fragments that were entrained from the conduit wall as observed from the Waipiata Volcanic Field, South Auckland and Auckland Volcanic Fields (C). In special cases exotic mineral phases such as glauconite (e.g. in diatremes of the Waipiata Volcanic Fields) have been used to infer that glauconitic sandstone cover must have been widespread in areas in the past and been ripped from the conduit wall during the later phase of phreatomagmatic volcanism (Németh Citation2001) (D).
Figure 10. A – SEM image of an interactive particle, a glassy pyroclasts with fractured texture and blocky, non-vesicular appearance indicating MFCI process to be responsible for its formation; B – Close up view of a glassy pyroclasts from Orakei Basin maar showing surface textural features (arrows) suggest brittle fragmentation caused by magma-water explosive interaction; C – Thin section view (plane parallel light) of an accretionary lapilli (ac) from a fine grained tuff of the Crater Hill tuff ring succession. The image is about 1 mm across; D – A peperitic domain as a lapilli in the basal tuff ring sequence of the Mangere Mountain (Auckland Volcanic Field)
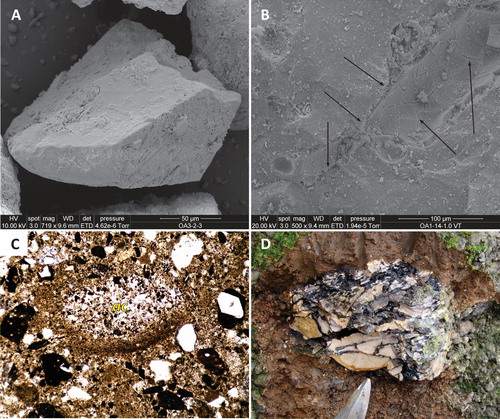
Figure 11. A – Slightly palagonitized sideromelane pyroclast in thin section (plane parallel light) from the ejecta ring of the Pukaki Lagoon maar, Auckland Volcanic Field. Note that the pyroclasts has low vesicularity, it is blocky and has abundant microlites. The core of the pyroclasts (bounded by white dashed line) is still fresh while the outer part (o) became dark yellow indicating gradual palagonitization. The image is about 0.5 mm across; B – Tachylite (black) and sideromelane (yellow) glass in thin section (plane paralell light) from the tuff ring of Crater Hill (Auckland Volcanic Field). Note the palagonitization on the sideromelane glass shard in form of colour changes as well as the thin rim around large pyroclasts forming armoured pyroclasts (arrows). The image is about 2 mm across.
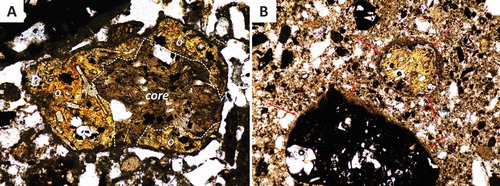
Figure 12. A – Typical proximal pyroclastic succession of a phreatomagmatic volcano in the Auckland Volcanic Field. The image was taken from the Waitomokia/Mt Gabriel tuff ring basal section. It is rich in shallow excavated accidental lithic clasts commonly forming distinct bomb horizons (arrows) that can be interpreted as ballistic curtain deposits; B – A typical sieved sample from the fine tuff beds of the Panmure Basin maar ejecta ring succession. The image shows the 125–250 μm size fractions. The grains are mineral phases (mostly quartz) derived from various siliciclastic country rocks that have been ‘recycled’ by the phreatomagmatic explosions and crater excavation. Note the dark volcanic glass shards; C – Sedimentary rock fragment (s) in a cored basaltic bomb from the basal succession of the ejecta ring of the Orakei Basin maar (Auckland Volcanic Field); D – Thin section image of a pyroclastic rock from the Nenthorn Diatreme (Waipiata Volcanic Field). The samples contain abundant glauconite (g) from pre-volcanic marine sediments that were eroded away in the region. The identification of such grains used as proof to state that marine sedimentary cover existed in the region in the time the maar-diatreme formed.
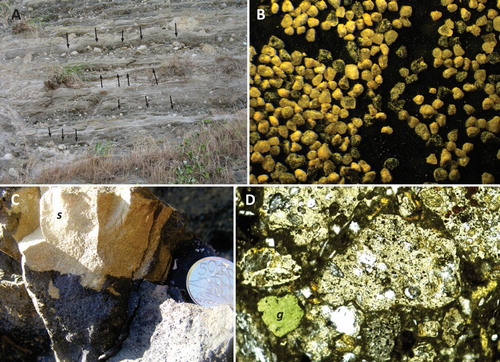
Evidence of hydrovolcanism associated with small volume silicic volcanism along the Taupo Volcanic Zone
The volcanic geology of the Taupo Volcanic Zone (TVZ) indicates that phreatomagmatic, or in broader sense hydrovolcanic activity, were involved in silicic, small to medium-sized eruptions since the 25.4 ka Oruanui caldera-forming eruption of Taupo volcano (Wilson Citation2001). In contrast, there are only a few known silicic examples, where magma and water interaction was proven prior to the Oruanui eruption for the onshore part of TVZ, even though the paleoenvironmental settings were very similar to the present day (Kósik et al. Citation2018). A lake side outcrop on Motuoapa Peninsula, southeast shore of Lake Taupo, exposes more than 100 m of coarse PDC and fall dominated pyroclastic deposits (A & B) that formed about 80 ka (Kósik Citation2018; Kósik et al. Citation2020). The architecture of the remnant pyroclastic cone and sedimentological features of the pyroclastic deposits indicate magma-water interaction occurred during explosive phases of activity. The deposits include accidental lithic rich lapilli tuffs resulting from PDC transportation, and the low vesicularity and micro-texture of juvenile fragments such as micro-fracturing, pitting on glass shard surfaces or fine dust adhering on fine ash grains (Kósik Citation2018). Paleoenvironmental reconstruction indicates an extensive lacustrine environment existed here between 200–25 ka relating to the ancient Lake Huka (Manville Citation2001). This is in keeping with the inferred subaqueous to emergent nature of Motuoapa activity. Tuff/pumice cone edifices similar to Motuoapa were also reported from the Haroharo Dome Complex in the Okataina Volcanic Centre such as Pukerimu tuff cone (Nairn Citation2002) and from the peralkaline Mayor Island volcano located offshore in the Bay of Plenty (Houghton and Wilson Citation1986).
Figure 13. A – View of the exposed, more than 100 m thick pyroclastic succession of Motuoapa Peninsula from Lake Taupo; B – Coarse PDC and fall-dominated sequence of the middle upper succession of Motuoapa pyroclastic deposits; C – Larger dome of Puketerata Volcanic Complex, which surrounded by an atypical steep-sided tuff ring; D – Wet surge-dominated cohesive layers with a bomb sag of large rhyolitic clast and thin better sorted shower-bedded layers at the base of the Puketerata sequence; E – Chain of maar craters relating to the NE section of the Puketerata fissure eruption. The arrow indicates the location of the Te Hukui diatreme (B); F – Block-and-ash-flow deposits found near the top of the western side of the ejecta ring that surrounds the larger dome.
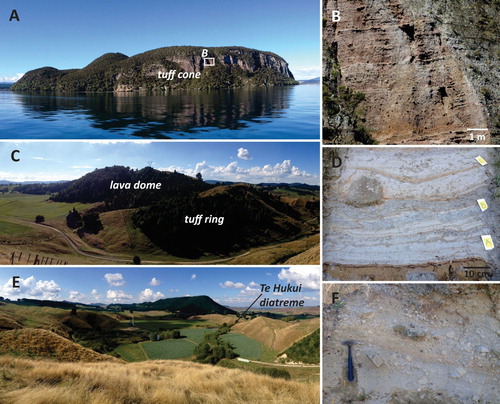
Post-Oruanui activity of Taupo volcano was mostly characterised by small-volume (<0.5km3) sub-Plinian to Plinian rhyolitic eruptions that occurred through Lake Taupo, where some degree of magma-water interaction was present (Wilson Citation1993). It is the same for the young activity of Okataina, where the initial phases of eruption were usually phreatomagmatic. Their activity was usually characterised by fissure-fed eruptions lasting several months to years (Smith and Houghton Citation1995; Cole et al. Citation2010; Houghton et al. Citation2010), where the erupted volumes of single eruptive episodes ranged between 4–17 km3 and were almost equally shared by effusive dome-forming and sub-Plinian to Plinian explosive activity (Kósik Citation2018). Phreatomagmatic activity was frequently present during the activity of Okataina Volcanic Centre in the past 45 ka indicated by the deposits of wet pyroclastic surges often containing accretionary lapilli, although their products are volumetrically insignificant.
Outside of the two most active areas of the silicic volcanism-dominated central TVZ (Okataina and Taupo), only a few eruptions were phreatomagmatic. Knowledge about the explosive activity of Ngangiho and Hipaua domes is quite restricted, however, the Puketarata (or Puketerata) Volcanic Complex is extensively studied (Brooker et al. Citation1993; Kósik et al. Citation2019), due to the early recognition of a dome surrounded by a well-preserved tuff ring (C) (Kósik et al. Citation2019). Sedimentological observations reveal that initial activity produced fine-grained cohesive surge deposits (D) along a 2.5 km long fissure vent. The southwest part of the fissure later produced two lava domes and a composite ejecta ring, whereas the northeast part exhibits the architecture of embryonic activity with a chain of maar craters (E). Similar structures with deep excavation are preserved at Okataina such as the Te Whekau crater (Nairn Citation2002) and at the Whakamaru dome at North Western Dome Complex of Whakamaru caldera (Leonard Citation2003). At Puketarata, the micro textural observations indicate that fracturing of groundmass glass post-dated the vesiculation and was most likely driven by contraction associated to fast cooling, melt quenching and glass transition, which was estimated to have happened about 620 m below the surface (Kósik et al. Citation2019). Fragmentation of quenched silicic magma allows the acceleration of pre-mixing with groundwater, essential for MFCI sensu stricto phreatomagmatic eruption (Austin-Erickson et al. Citation2008). The later stages of activity were dominated by effusive activity, which was frequently interrupted by explosive phases. The juvenile fragments of the fall deposits from these destructive events exhibit low vesicularity (ranges between 0% and 45% with an average 25%), indicating the explosive activity was triggered externally. Destruction of the lava dome usually triggered short-running block-and-ash flows that were mostly deposited in the vicinity of dome (F).
Discussion
Hydromagmatism as outlined in the previous sections is an important geological process recognised in various scales of preserved volcanic successions across Zealandia. Hydromagmatism in the eruption styles of many monogenetic volcanoes of Zealandia is a direct consequence of the availability of surface water, abundant ground water, and a general wet climate through the Cenozoic.
At macro, meso and micro-scale there are numerous features commonly associated with magma and water interaction (or water influence upon rising magma), but none of them alone are diagnostic. The micro and macro textural features (juvenile pyroclast vesicularity, shape and pyroclastic deposit/rock componentry alongside with various bedding features such as cross bedding, dune bedding or ballistic curtain deposits), together with the 3D facies architecture and the geological context, provide evidence that hydrovolcanic processes have taken place.
Through most of the Cenozoic the microcontinent of Zealandia experienced a warm – temperate climate with humid conditions providing abundant surface waters and water-rich subsurface conditions (Buening et al. Citation1998; Nelson and Cooke Citation2001; Lindqvist and Lee Citation2009; Land et al. Citation2010; Mildenhall et al. Citation2014; Prebble et al. Citation2017). While there is no complete climate record through the Cenozoic to link precisely the type of volcanism with the climatic conditions at the time, we have a fairly good climate and paleohydrogeology record for the Miocene when a large number of volcanic fields formed, mostly onshore in the proto-South Island and Chatham Rise regions (Field et al. Citation1989; Schiøler et al. Citation2011, Citation2014; Arnot and Bland Citation2016). Based on the terrestrial fossil record during the Miocene, New Zealand experienced significant shifts in average annual temperature but stayed within the conditions facilitating hydrovolcanism. Well-preserved floral assemblages recovered from the Dunedin region revealed that during the mid/late Miocene climate, during the Dunedin volcano episode, was warm-temperate to subtropical (mean annual temperature [MAT]: 17–19 degrees C) at Double Hill, similar to modern coastal Queensland, and cool- to warm-temperate (MAT: similar to 12–14 degrees C) at Kaikorai Valley, similar to modern northern New Zealand (Reichgelt et al. Citation2016). These conditions provide the perfect hydrogeological setting that can heavily influence small-volume, monogenetic volcanism, and provide the emergence of abundant volcanoes formed by hydrovolcanic processes.
Farther inland, in the area of the WVF for instance, paleobotany and paleoclimatic research has revealed a diverse floral assemblage of sub-tropical species in a water-rich environment that likely affected the style of volcanism in that area to be more hydrovolcanic (Lindqvist and Lee Citation2009; Kaulfuss et al. Citation2014; Mildenhall et al. Citation2014; Fox et al. Citation2015; Kaulfuss and Dlussky Citation2015; Lee et al. Citation2016; Jones et al. Citation2017).
During the Cenozoic, at the maximum submergence of Zealandia, the regions with the thickest continental crust, were covered by a shelf-like, shallow sea into which small volumes of mafic magma were erupted and formed small-volume shallow marine to emergent volcanoes such as those in the Chatham Islands’ Red Bluff Tuff (Sorrentino et al. Citation2011; Stilwell and Consoli Citation2012) or the erosional remnant of East Otago's Surtseyan tuff cones and subaqueous pyroclastic mounds (Cas et al. Citation1989; Moorhouse et al. Citation2015).
Using the modern analogy, Cenozoic monogenetic volcanism in the Zealandia context shows similarities to other well-studied volcanic regions formed in or near sea level coastal plains and shallow shelfs showing a complete spectrum from shallow marine, emergent to terrestrial volcanism that is influenced heavily by external water (Németh et al. Citation2010). Cenozoic monogenetic volcanism in Zealandia shows strong physical volcanology similarities to volcanic regions formed in the Miocene to Pleistocene time in the Pannonian Basin in Central Europe where the geodynamics change from a subduction-dominated, narrow marine basin to a broad coastal region that includes intracontinental mafic volcanism through a near-plate boundary setting in low-lying, well-drained fluvio-lacustrine terrestrial environment (Wijbrans et al. Citation2007; Lexa et al. Citation2010; Kereszturi et al. Citation2011; Kovács et al. Citation2020).
Analogies have recently been used for identifying analogous volcanic fields where the eruption scenarios of individual volcano growth can be viewed as similar to those in the most recent monogenetic volcanic fields in the northern part of the North Island of New Zealand. Comparison of the New Zealand's monogenetic volcanic fields and their volcanic eruption styles with those in Jeju Island, Korea (Brenna et al. Citation2010, Citation2011; Sohn et al. Citation2012), in western Saudi Arabia (Moufti et al. Citation2015), Japan (Geshi et al. Citation2011; Geshi et al. Citation2019) or Argentina (Risso et al. Citation2008) provides vital insight to the full spectrum of eruption styles that a typical low-lying, well-drained mafic-dominated volcanic system can produce. Terrestrial hydrovolcanic edifices, particularly those where the original volcanic landforms are still recognisable are good sites that can be used to understand cratering (e.g. maar-diatreme growth) processes, or pyroclastic density current transportation and deposition. So far, little research have been conducted to define the relative ratios of various lithofacies preserved in a tuff ring succession surrounding a broad crater (e.g. maar) to help understand the relative explosion depth, the estimated explosion energy release and the processes of crater growth (Graettinger et al. Citation2015, Citation2016; Valentine et al. Citation2015; Macorps et al. Citation2016; Graettinger and Valentine Citation2017; Graettinger Citation2018; Sonder et al. Citation2018). Most tuff ring successions of the youngest hydrovolcanic edifices formed by phreatomagmatic explosive eruptions resemble natural examples abundant in bedded, dune-bedded and stratified tuffs and lapilli tuffs. Most of the New Zealand examples have initial tuff breccia horizons (Németh et al. Citation2010, Citation2012b). In this respect the New Zealand examples resemble the facies architecture similar of Ubehebe maars (California) (Champion et al. Citation2018), Zuni Salt Lake maar (Utah) (Shoemaker Citation1957; Onken and Forman Citation2017), Tihany maars (Hungary) (Németh et al. Citation2001) or Suwolbong tuff ring (Jeju Island, Korea) (Sohn and Chough Citation1989). Similar facies architecture is recorded in large scale experiments where the explosion locus was deeper than the optimal scaled depth (Valentine et al. Citation2015; Graettinger and Valentine Citation2017). No such systematic study has been undertaken on the tephra rings of New Zealand phreatomagmatic volcanoes. This could be a new and valuable direction for future research because of its relevance to understanding the volcanic hazard such eruptions can pose.
Conclusion
In this overview we provide an up-to-date critical review of hydrovolcanism and its relevance to the volcanic evolution of Zealandia during the Cenozoic. The region has been geographically isolated during these 65 million years and was comprised of a gradually submerging continental landmass that at maximum submergence consisted of an archipelago of island refugia, surrounded by a massive water body and located mostly in a temperate climatic zone. Abundant surface water fed the resultant hydrovolcanism of the monogenetic volcanic field evolution. In near-sea level coastal regions, maars, tuff rings and associated scoria cones formed as ascending dykes interacted with the available water. In contrast emergent to fully subaqueous volcanism dominated those offshore regions, where magma found its way to the surface. If we are looking in a broader Zealandia context and adding the buried or offshore volcanic remnants in the argument, we can say that magma-water influenced volcanism is widespread in the region (Herzer Citation1995; Bischoff et al. Citation2019a, Citation2019b, Citation2019c). As increasing new data become available from various sedimentary basins worldwide, we can see that in a low-lying coastal environment typical hydrovolcanic landforms can grow, quickly be buried and preserved in a shallow subaqueous sedimentary basin succession along with subaqueous hydromagmatic macroforms developed over the lifespan of the volcanic fields in such geo-environments (Panisova et al. Citation2018). Hence New Zealand provides a natural laboratory for studying the full spectrum of eruption styles, from fully subaqueous to terrestrial, in time and space. However, the preservation potential of many of New Zealand's Cenozoic monogenetic volcanoes on land is not great due intense weathering and erosion that accompanies the same environmental conditions. In contrast hydrovolcanic macroforms in offshore regions are commonly preserved as volcanic mounds in the sedimentary record of the post-Cretaceous rock record across Zealandia (Bischoff et al. Citation2020). In pre-Pliocene volcanoes, commonly only the core of the crater or upper conduit zones are preserved as exposed diatremes or core of emergent to fully subaqueous volcanoes. There are a few exceptions such as the Oamaru Surtseyan volcanoes, which still have recognisable crater and near-vent pyroclastic successions exposed in the present day landscape (Moorhouse et al. Citation2015). In addition, young volcanic landforms are commonly covered by vegetation or are undergoing deep chemical weathering of juvenile pyroclasts thus reducing their usefulness as global reference volcanoes. In this respect we conclude that while the hydrovolcanic landforms in New Zealand are important researching them needs to be undertaken in a global perspective using overseas analogues if we are to better understand the geological information they hold.
Acknowledgements
We grateful for the invitation of the NZJGG to contribute to the IAVCEI 2022 special issue to provide an overview of Cenozoic hydrovolcanism in the Zealandia context. We thankful for all the suggestions and ideas colleagues provided in the past decades to be able to provide a concise overview on this topic. Many thanks for Julie Palmer (Massey University) for reading the original draft and make good suggestions on presentation style and context. The excellent formal Journal reviews by Rosie Cole, Alan Bischoff and an Anonymous reviewer as well as the editorial work of James Scott are greatly appreciated.
Disclosure statement
No potential conflict of interest was reported by the author(s).
Data availability statement
Data sharing is not applicable to this article as no new data were created or analyzed in this study.
References
- Adams CJ. 1981. Migration of late Cenozoic volcanism in the South Island of New Zealand. Nature. 294:153–155.
- Agustin-Flores J, Németh K, Cronin SJ, Lindsay JM, Kereszturi G, Brand BD, Smith IEM. 2014. Phreatomagmatic eruptions through unconsolidated coastal plain sequences, Maungataketake, Auckland Volcanic Field (New Zealand). Journal of Volcanology and Geothermal Research. 276:46–63.
- Agustin-Flores J, Németh K, Cronin SJ, Lindsay JM, Kereszturi G. 2015. Construction of the North Head (Maungauika) tuff cone: a product of Surtseyan volcanism, rare in the Auckland Volcanic Field, New Zealand. Bulletin of Volcanology. 77(2). Article number: 11.
- Amin J, Valentine GA. 2017. Compound maar crater and co-eruptive scoria cone in the Lunar Crater Volcanic Field (Nevada, USA). Journal of Volcanology and Geothermal Research. 339:41–51.
- Aramaki S, Hayakawa Y, Fujii T, Nakamura K, Fukuoka T. 1986. The October 1983 eruption of Miyakejima Volcano. Journal of Volcanology and Geothermal Research. 29(1-4):203–229.
- Arnot MJ, Bland KJ. 2016. Atlas of petroleum prospectivity, Northwest Province: ArcGIS geodatabase and technical report. GNS Science Data Series 23b. GNS Science Data Series 23b. 34 p. + 1 ArcGIS geodatabase+5 ArcGIS projects. https://data.gns.cri.nz/pbe/#!#HTML:Content%2Foutputs%2FAPP%2FReport%2Fnavigation%2Fintro.html.
- Austin-Erickson A, Buettner R, Dellino P, Ort MH, Zimanowski B. 2008. Phreatomagmatic explosions of rhyolitic magma: experimental and field evidence. Journal of Geophysical Research-Solid Earth. 113(B11). Article Number: B11201.
- Balashova A, Mattsson HB, Hirt AM, Almqvist BSG. 2016. The Lake Natron Footprint Tuff (northern Tanzania): volcanic source, depositional processes and age constraints from field relations. Journal of Quaternary Science. 31(5):526–537.
- Ballance PF, Hayward BW, Brook FJ. 1985. Subduction regression of volcanism in New Zealand. Nature. 313(6005):820–820.
- Berghuijs JF, Mattsson HB. 2013. Magma ascent, fragmentation and depositional characteristics of “dry” maar volcanoes: similarities with vent-facies kimberlite deposits. Journal of Volcanology and Geothermal Research. 252:53–72.
- Bischoff A, Barrier A, Beggs M, Nicol A, Cole J, Sahoo T. 2020. Magmatic and tectonic interactions revealed by buried volcanoes in Te Riu-a-Māui/Zealandia sedimentary basins. New Zealand Journal of Geology and Geophysics, in review.
- Bischoff A, Nicol A, Barrier A, Wang H. 2019a. Paleogeography and volcanic morphology reconstruction of a buried monogenetic volcanic field (part 2). Bulletin of Volcanology. 81(9). Article number: 57.
- Bischoff A, Nicol A, Cole J, Gravley D. 2019b. Stratigraphy of architectural elements of a buried monogenetic volcanic system. Open Geosciences. 11(1):581–616.
- Bischoff A, Rossetti M, Nicol A, Kennedy B. 2019c. Seismic reflection and petrographic interpretation of a buried monogenetic volcanic Field (part 1). Bulletin of Volcanology. 81(9). Article number: 56.
- Bishop DG, Turnbull IM. 1996. Geology of the Dunedin area: scale 1:250,000. Lower Hutt: Institute of Geological & Nuclear Sciences. Institute of Geological & Nuclear Sciences 1:250,000 geological map 21. 52 p. + 1 folded map.
- Blake DM, Deligne NI, Wilson TM, Lindsay JM, Woods R. 2017. Investigating the consequences of urban volcanism using a scenario approach II: insights into transportation network damage and functionality. Journal of Volcanology and Geothermal Research. 340:92–116.
- Brand BD, Brož P. 2015. Tuff cone. In: Hargitai H, Kereszturi Á, editors. Encyclopedia of planetary landforms. New York, NY: Springer; p. 2197–2204.
- Brenna M, Cronin SJ, Németh K, Smith IEM, Sohn YK. 2011. The influence of magma plumbing complexity on monogenetic eruptions, Jeju Island, Korea. Terra Nova. 23(2):70–75.
- Brenna M, Cronin SJ, Smith IEM, Sohn YK, Németh K. 2010. Mechanisms driving polymagmatic activity at a monogenetic volcano, Udo, Jeju Island, South Korea. Contributions to Mineralogy and Petrology. 160(6):931–950.
- Briggs RM, Itaya T, Lowe DJ, Keane AJ. 1989. Ages of the Pliocene Alexandra and Ngatutura Volcanics, Western North-Island, New Zealand, and some geological implications. New Zealand Journal of Geology and Geophysics. 32(4):417–427.
- Briggs RM, McDonough WF. 1990. Contemporaneous convergent margin and intraplate magmatism, North Island, New Zealand. Journal of Petrology. 31(4):813–951.
- Briggs RM, Okada T, Itaya T, Shibuya H, Smith IEM. 1994. K-Ar Ages, paleomagnetism, and geochemistry of the South Auckland Volcanic Field, North-Island, New-Zealand. New Zealand Journal of Geology and Geophysics. 37(2):143–153.
- Briggs RM, Rosenberg MD, deLange PJ, Itaya T, King PR, Price RC. 1997. Geology and geochemistry of Gannet (Karewa) island, Tasman sea: a rift-related nephelinitic tuff ring. New Zealand Journal of Geology and Geophysics. 40(3):263–273.
- Brooker MR, Houghton BF, Wilson CJN, Gamble JA. 1993. Pyroclastic phases of a rhyolitic dome-building eruption - Puketarata tuff ring, Taupo Volcanic Zone, New Zealand. Bulletin of Volcanology. 55(6):395–406.
- Brothers RN. 1984. Subduction regression and oceanward migration of volcanism, North Island, New Zealand. Nature. 309(5970):698–700.
- Brothers RN. 1985. Subduction regression of volcanism in New Zealand - Reply. Nature. 313(6005):820–821.
- Brown SJA, Smith RT, Cole JW, Houghton BF. 1994. Compositional and textural characteristics of the strombolian and surtseyan K-Trig basalts, Taupo volcanic centre, New Zealand; implications for eruption dynamics. New Zealand Journal of Geology and Geophysics. 37(1):113–126.
- Brož P, Németh K. 2015. Tuff ring. In: Hargitai H, Kereszturi Á, editors. Encyclopedia of planetary landforms. New York, NY: Springer; p. 2204–2210.
- Buechner J, Tietz O. 2012. Reconstruction of the Landeskrone Scoria Cone in the Lusatian Volcanic Field, Eastern Germany - long-term degradation of volcanic edifices and implications for landscape evolution. Geomorphology. 151:175–187.
- Buening N, Carlson SJ, Spero HJ, Lee DE. 1998. Evidence for the Early Oligocene formation of a proto-Subtropical Convergence from oxygen isotope records of New Zealand Paleogene brachiopods. Palaeogeography Palaeoclimatology Palaeoecology. 138(1-4):43–68.
- Buettner R, Dellino P, La Volpe L, Lorenz V, Zimanowski B. 2002. Thermohydraulic explosions in phreatomagmatic eruptions as evidenced by the comparison between pyroclasts and products from Molten Fuel Coolant Interaction experiments. Journal of Geophysical Research. 107(B11):14–14.
- Buettner R, Dellino P, Zimanowski B. 1999. Identifying magma-water interaction from the surface features of ash particles. Nature. 401(6754):688–690.
- Cas RAF. 1989. Intraplate volcanism in eastern Australia and New Zealand. In: Johnson RW, Knutson J, Taylor SR, editors. Cambridge, United Kingdom (GBR). Cambridge: Cambridge Univ. Press.
- Cas R, Ca, L, RE F. 1989. A monogenetic, Surtla-type, Surtseyan volcano from Eocene-Oligocene Waiareka-Deborah volcanics, Otago, New Zealand: a model. Bulletin of Volcanology. 51:281–298.
- Champion DE, Cyr A, Fierstein J, Hildreth W. 2018. Monogenetic origin of Ubehebe Crater maar volcano, Death Valley, California: Paleomagnetic and stratigraphic evidence. Journal of Volcanology and Geothermal Research. 354:67–73.
- Christenson B, Németh K, Rouwet D, Tassi F, Vandemeulebrouck J, Varekamp JC. 2015. Volcanic lakes. In: Rouwet D, Christenson B, Tassi F, Vandemeulebrouck J, editors. Volcanic lakes. Berlin - Heidelberg: Springer-Verlag; p. 1–20.
- Cole JW, Lewis KB. 1981. Evolution of the Taupo-Hikurangi subduction system. Tectonophysics. 72(1-2):1–21.
- Cole JW, Spinks KD, Deering CD, Nairn IA, Leonard GS. 2010. Volcanic and structural evolution of the Okataina Volcanic Centre; dominantly silicic volcanism associated with the Taupo Rift, New Zealand. Journal of Volcanology and Geothermal Research. 190(1-2):123–135.
- Cook C, Briggs RM, Rosenberg MD, Smith IEM. 2003. Geochemical diversity of juvenile basaltic pyroclasts at Barriball tuff ring, south Auckland, New Zealand. Geological Society of New Zealand Miscellaneous Publication. 116A:33–33.
- Cook C, Briggs RM, Smith IEM, Maas R. 2005. Petrology and geochemistry of intraplate basalts in the South Auckland Volcanic Field, New Zealand: evidence for two coeval magma suites from distinct sources. Journal of Petrology. 46(3):473–503.
- Coombs DS, Adams CJ, Roser BP, Reay A. 2008. Geochronology and geochemistry of the Dunedin Volcanic Group, eastern Otago, New Zealand. New Zealand Journal of Geology and Geophysics. 51(3):195–218.
- Coombs DS, Cas RA, Kawachi Y, Landis CA, McDonough WF, Reay A. 1986. Cenozoic volcanism in North, East and Central Otago. In: Smith I, editor. Cenozoic Volcanism in New Zealand, Roy. Soc. NZ Bull. p. 278–312.
- Corcoran PL, Moore LN. 2008. Subaqueous eruption and shallow-water reworking of a small-volume Surtseyan edifice at Kakanui, New Zealand. Canadian Journal of Earth Sciences. 45(12):1469–1485.
- Darragh M, Cole J, Nairn I, Shane P. 2006. Pyroclastic stratigraphy and eruption dynamics of the 21.9 ka Okareka and 17.6 ka Rerewhakaaitu eruption episodes from Tarawera Volcano, Okataina Volcanic Centre, New Zealand. New Zealand Journal of Geology and Geophysics. 49(3):309–328.
- De Hon R. 2015. Maar. In: Hargitai H, Kereszturi Á, editors. Encyclopedia of planetary landforms. New York (NY): Springer; p. 1295–1299.
- De Rita D, Giordano G, Esposito A, Fabbri M, Rodani S. 2002. Large volume phreatomagmatic ignimbrites from the Colli Albani Volcano (Middle Pleistocene, Italy). Journal of Volcanology and Geothermal Research. 118(1-2):77–98.
- de Silva S, Lindsay JM. 2015. Chapter 15 - Primary volcanic landforms. In: Sigurdsson H, editor. The Encyclopedia of volcanoes. 2nd ed. Amsterdam: Academic Press; p. 273–297.
- Doronzo DM, Dellino P, Sulpizio R, Lucchi F. 2017. Merging field mapping and numerical simulation to interpret the lithofacies variations from unsteady pyroclastic density currents on uneven terrain: the case of La Fossa di Vulcano (Aeolian Islands, Italy). Journal of Volcanology and Geothermal Research. 330:36–42.
- Dowding EM, Ebach MC. 2017. Zealandia is not a continent. Nature. 543(7644):179–179.
- Duerig T, Zimanowski B. 2012. “Breaking news” on the formation of volcanic ash: fracture dynamics in silicate glass. Earth and Planetary Science Letters. 335:1–8.
- Edgar CJ, Wolff JA, Olin PH, Nichols HJ, Pittari A, Cas RAF, Reiners PW, Spell TL, Marti J. 2007. The late quaternary Diego Hernandez Formation, Tenerife: volcanology of a complex cycle of voluminous explosive phonolitic eruptions. Journal of Volcanology and Geothermal Research. 160(1-2):59–85.
- Field BD, Browne GH, Davy BW, Herzer RH, Hoskins RH, Raine JI, Wilson GJ, Sewell RJ, Smale D, Watters WA. 1989. Cretaceous and Cenozoic sedimentary basins and geological evolution of the Canterbury region, South Island, New Zealand. New Zealand Geological Survey Basin Studies [New Zealand Geological Survey, Lower Hutt, New Zealand]. 2:1–94.
- Fox BRS, Wartho J, Wilson GS, Lee DE, Nelson FE, Kaulfuss U. 2015. Long-term evolution of an Oligocene/Miocene maar lake from Otago, New Zealand. Geochemistry Geophysics Geosystems. 16(1):59–76.
- Gaina C, Muller DR, Royer JY, Stock J, Hardebeck J, Symonds P. 1998. The tectonic history of the Tasman Sea: a puzzle with 13 pieces. Journal of Geophysical Research-Solid Earth. 103(B6):12413–12433.
- Gamble JA, Adams AE. 1985. Volcanic geology of Carnley volcano, Auckland Island. New Zealand Journal of Geology and Geophysics. 28:43–54.
- Gamble JA, Morris PA, Adams CJ. 1986. The geology, petrology and geochemistry of Cenozoic volcanic rocks from the Campbell Plateau and Chatham Rise. Bulletin - Royal Society of New Zealand. 23:344–365.
- Gamble JA, Thomson JW. 1990. Volcanoes of the Antarctic Plate and Southern Oceans. Antarctic Research Series. 48:465–467.
- Geshi N, Kim H, Kaetsu D, Young PT. 2003. Phreatomagmatic eruption with caldera collapse during the Miyakejima 2000 eruption, Japan. Abstracts - International Volcanological Congress. 3:48–48.
- Geshi N, Németh K, Noguchi R, Oikawa T. 2019. Shift from magmatic to phreatomagmatic explosions controlled by the lateral evolution of a feeder dike in the Suoana-Kazahaya eruption, Miyakejima Volcano, Japan. Earth and Planetary Science Letters. 511:177–189.
- Geshi N, Németh K, Oikawa T. 2011. Growth of phreatomagmatic explosion craters: a model inferred from Suoana crater in Miyakejima Volcano, Japan. Journal of Volcanology and Geothermal Research. 201(1-4):30–38.
- Gibson A, Briggs RM, Pittari A, Németh K. 2010. Eruption processes of the Kellyville volcanic complex. Geoscience Society of New Zealand Miscellaneous Publication. 129A:105–105.
- Giordano G. 1998. Facies characteristics and magma-water interactions of the white trachytic tuffs (Roccamonfina Volcano, southern Italy). Bulletin of Volcanology. 60(1):10–26.
- Goles GG, Briggs RM, Rosenberg MD. 1996. Late pliocene stratigraphic succession and volcanic evolution of Karioi volcano, western North Island, New Zealand. New Zealand Journal of Geology and Geophysics. 39(2):283–294.
- Graettinger AH. 2018. Trends in maar crater size and shape using the global Maar Volcano Location and Shape (MaarVLS) database. Journal of Volcanology and Geothermal Research. 357:1–13.
- Graettinger AH, Skilling I, McGarvie D, Hoskuldsson A. 2013. Subaqueous basaltic magmatic explosions trigger phreatomagmatism: a case study from Askja, Iceland. Journal of Volcanology and Geothermal Research. 264:17–35.
- Graettinger AH, Valentine GA. 2017. Evidence for the relative depths and energies of phreatomagmatic explosions recorded in tephra rings. Bulletin of Volcanology. 79(12). Article number: 88.
- Graettinger AH, Valentine GA, Sonder I. 2015. Circum-crater variability of deposits from discrete, laterally and vertically migrating volcanic explosions: experimental evidence and field implications. Journal of Volcanology and Geothermal Research. 308:61–69.
- Graettinger AH, Valentine GA, Sonder I. 2016. Recycling in debris-filled volcanic vents. Geology. 44(10):811–814.
- Gutmann JT. 2002. Strombolian and effusive activity as precursors to phreatomagmatism: eruptive sequence at maars of the Pinacate volcanic field, Sonora, Mexico. Journal of Volcanology and Geothermal Research. 113(1-2):345–356.
- Hayes JL, Tsang SW, Fitzgerald RH, Blake DM, Deligne NI, Doherty A, Hopkins JL, Hurst AW, Le Corvec N, Leonard GS, et al. 2018. The DEVORA scenarios; multi-hazard eruption scenarios for the Auckland volcanic field. GNS Science Report: 138.
- Heiken GH. 1972. Morphology and petrography of volcanic ashes. Geological Society of America Bulletin. 83:1961–1988.
- Heiken GH. 1974. An atlas of volcanic ash. Smithsonian Earth Science Contributions. 12:1–101.
- Heiken G, Fisher RV, Negendank J, Brüchmann C. 2000. Water and magma can mix—a history of the concepts of hydrovolcanism (1819–1980). International Maar Conference: Daun, Germany: Terra Nostra; p. 165–189.
- Heiken GH, Wohletz KH. 1986. Volcanic Ash. Berkeley: University of California Press. 246 p.
- Heming RF. 1980. The Ngatatura diatreme. New Zealand Journal of Geology and Geophysics. 23:569–573.
- Hencz M, Karátson D, Németh K, Biró T. 2017. A Badacsony freatomagmás piroklasztitösszlete: következtetések a monogenetikus bazaltvulkáni működés folyamataira és formáira = The phreatomagmatic pyroclastic sequence of the Badacsony Hill: implications for the processes and landforms of monogenetic basaltic volcanism. Földtani közlöny. 147(3):297–310.
- Herrero-Hernandez A, Lopez-Moro FJ, Gomez-Fernandez F, Martin-Serrano A. 2012. Interaction between intra-continental sedimentary basins and small-volume monogenetic volcanism: Argamasilla and Calzada-Moral basins, Campo de Calatrava Volcanic Field, Spain. Journal of Iberian Geology. 38(2):407–428.
- Herzer RH. 1995. Seismic stratigraphy of a buried volcanic arc, Northland, New Zealand and implications for Neogene subduction. Marine and Petroleum Geology. 12(5):511–531.
- Hoernle K, White JDL, van den Bogaard P, Hauff F, Coombs DS, Werner R, Timm C, Garbe-Schonberg D, Reay A, Cooper AF. 2006. Cenozoic intraplate volcanism on New Zealand: upwelling induced by lithospheric removal. Earth and Planetary Science Letters. 248(1-2):350–367.
- Hopkins JL, Smid ER, Eccles JD, Hayes JL, Hayward BW, McGee LE, van Wijk K, Wilson TM, Cronin SJ, Leonard GS, et al. 2020. Auckland Volcanic field magmatism, volcanism, and hazard: a review. New Zealand Journal of Geology and Geophysics. https://doi.org/10.1080/00288306.2020.1736102.
- Houghton BF, Carey RJ, Cashman KV, Wilson CJN, Hobden BJ, Hammer JE. 2010. Diverse patterns of ascent, degassing, and eruption of rhyolite magma during the 1.8 ka Taupo eruption, New Zealand: evidence from clast vesicularity. Journal of Volcanology and Geothermal Research. 195(1):31–47.
- Houghton BF, Hackett WR. 1984. Strombolian and phreatomagmatic deposits of Ohakune Craters, Ruapehu, New Zealand; a complex interaction between external water and rising basaltic magma. Journal of Volcanology and Geothermal Research. 21(3-4):207–231.
- Houghton BF, Schmincke HU. 1986. Mixed deposits of simultaneous strombolian and phreatomagmatic volcanism; Rothenberg Volcano, East Eifel volcanic field. Journal of Volcanology and Geothermal Research. 30(1-2):117–130.
- Houghton BF, Wilson CJN. 1986. A1; Explosive rhyolite volcanism; the case studies of Mayor Island and Taupo volcanoes. Record - New Zealand Geological Survey. 12:33–100.
- Houghton BF, Wilson CJN, Rosenberg MD, Smith IEM, Parker RJ. 1996. Mixed deposits of complex magmatic and phreatomagmatic volcanism: an example from Crater Hill, Auckland, New Zealand. Bulletin of Volcanology. 58(1):59–66.
- Ilanko T, Pittari A, Briggs RM, Németh K. 2009. Pyroclastic successions of a tuff ring in a monogenetic field; Barriball Road tuff ring, south Auckland. Geological Society of New Zealand Miscellaneous Publication. 128A:93–93.
- Jones DA, Wilson GS, Gorman AR, Fox BRS, Lee DE, Kaulfuss U. 2017. A drill-hole calibrated geophysical characterisation of the 23 Ma Foulden Maar stratigraphic sequence, Otago, New Zealand. New Zealand Journal of Geology and Geophysics. 60(4):465–477.
- Jordan SC, Duerig T, Cas RAF, Zimanowski B. 2014. Processes controlling the shape of ash particles: results of statistical IPA. Journal of Volcanology and Geothermal Research. 288:19–27.
- Kaulfuss U, Dlussky GM. 2015. Early Miocene Formicidae (Amblyoponinae, Ectatomminae, ?Dolichoderinae, Formicinae, and Ponerinae) from the Foulden Maar Fossil Lagerstatte, New Zealand, and their biogeographic relevance. Journal of Paleontology. 89(6):1043–1055.
- Kaulfuss U, Harris AC, Conran JG, Lee DE. 2014. An early Miocene ant (subfam. Amblyoponinae) from Foulden Maar: the first fossil Hymenoptera from New Zealand. Alcheringa. 38(4):568–574.
- Kear D. 1994. A Least Complex Dynamic-Model for Late Cenozoic Volcanism in the North-Island, New-Zealand. New Zealand Journal of Geology and Geophysics. 37(2):223–236.
- Kereszturi G, Németh K. 2011. Shallow-seated controls on the evolution of the Upper Pliocene Kopasz-hegy nested monogenetic volcanic chain in the Western Pannonian Basin (Hungary). Geologica Carpathica. 62(6):535–546.
- Kereszturi G, Németh K, Cronin SJ, Agustin-Flores J, Smith IEM, Lindsay J. 2013. A model for calculating eruptive volumes for monogenetic volcanoes - Implication for the Quaternary Auckland Volcanic Field, New Zealand. Journal of Volcanology and Geothermal Research. 266:16–33.
- Kereszturi G, Németh K, Cronin SJ, Procter J, Agustin-Flores J. 2014. Influences on the variability of eruption sequences and style transitions in the Auckland Volcanic Field, New Zealand. Journal of Volcanology and Geothermal Research. 286:101–115.
- Kereszturi G, Németh K, Csillag G, Balogh K, Kovacs J. 2011. The role of external environmental factors in changing eruption styles of monogenetic volcanoes in a Mio/Pleistocene continental volcanic field in western Hungary. Journal of Volcanology and Geothermal Research. 201(1-4):227–240.
- King PR. 2000. Tectonic reconstructions of New Zealand: 40 Ma to the present. New Zealand Journal of Geology and Geophysics. 43(4):611–638.
- Kósik S. 2018. Small-volume volcanism associated with polygenetic volcanoes, Taupo Volcanic Zone, New Zealand: a thesis presented in partial fulfilment of the requirements for the degree of Doctor of Philosophy in Earth Science at Massey University, Palmerston North, New Zealand. Unpublished Doctoral thesis, Massey University.
- Kósik S, Németh K, Danišík M, Procter JN, Schmitt A, Friedrichs B, Stewart RB. 2020. Shallow subaqueous to emergent intra-caldera silicic volcanism of the Motuoapa Peninsula, Taupo Volcanic Zone, New Zealand – new constraints from geologic mapping, sedimentology and zircon geochronology. Journal of Volcanology and Geothermal Research, in review.
- Kósik S, Nemeth K, Gravley D, Procter J. 2018. Hidden by “super-eruptions”: temporal-volumetric characteristics of small-volume volcanism of the Taupo Volcanic Zone, New Zealand. Geoscience Society of New Zealand Miscellaneous Publication. 151A:153.
- Kósik S, Németh K, Kereszturi G, Procter JN, Zellmer GF, Geshi N. 2016. Phreatomagmatic and water-influenced Strombolian eruptions of a small-volume parasitic cone complex on the southern ringplain of Mt. Ruapehu, New Zealand: facies architecture and eruption mechanisms of the Ohakune Volcanic Complex controlled by an unstable fissure eruption. Journal of Volcanology and Geothermal Research. 327:99–115.
- Kósik S, Németh K, Lexa J, Procter JN. 2019. Understanding the evolution of a small-volume silicic fissure eruption: Puketerata Volcanic Complex, Taupo Volcanic Zone, New Zealand. Journal of Volcanology and Geothermal Research. 383:28–46.
- Kósik S, Németh K, Procter JN, Zellmer GF. 2017. Maar-diatreme volcanism relating to the pyroclastic sequence of a newly discovered high-alumina basalt in the Maroa Volcanic Centre, Taupo Volcanic Zone, New Zealand. Journal of Volcanology and Geothermal Research. 341:363–370.
- Kovács J, Németh K, Szabó P, Kocsis L, Kereszturi G, Újvári G, Vennemann T. 2020. Volcanism and paleoenvironment of the Pula maar complex: a pliocene terrestrial fossil site in Central Europe (Hungary). Palaeogeography Palaeoclimatology Palaeoecology. 537:15.
- Kshirsagar PV, Sheth HC, Shaikh B. 2011. Mafic alkalic magmatism in central Kachchh, India: a monogenetic volcanic field in the northwestern Deccan Traps. Bulletin of Volcanology. 73(5):595–612.
- Kurszlaukis S, Lorenz V. 2016. Differences and similarities between emplacement models of kimberlite and basaltic maar-diatreme volcanoes. Special Publication - Geological Society of London. 446:22.
- Laird MG. 1993. Cretaceous continental rifts: New Zealand region. In: Ballance PE, editor. Sedimentary basins of the world. Amsterdam, The Netherland: Elsevier Science Publisher; p. 37–49.
- Laird MG, Bradshaw JD. 2004. The break-up of a long-term relationship: the Cretaceous separation of New Zealand from Gondwana. Gondwana Research. 7(1):273–286.
- Land M, Wust RAJ, Robert C, Carter RM. 2010. Plio-Pleistocene paleoclimate in the Southwest Pacific as reflected in clay mineralogy and particle size at ODP Site 1119, SE New Zealand. Marine Geology. 274(1-4):165–176.
- Leat PT, Thompson RN. 1988. Miocene hydrovolcanism in NW Colorado, USA, fuelled by explosive mixing of basic magma and wet unconsolidated sediment. Bulletin of Volcanology. 50:229–243.
- Le Corvec N, Bebbington MS, Lindsay JM, McGee LE. 2013. Age, distance, and geochemical evolution within a monogenetic volcanic field: analyzing patterns in the Auckland Volcanic Field eruption sequence. Geochemistry Geophysics Geosystems. 14(9):3648–3665.
- Lee DE, Kaulfuss U, Conran JG, Bannister JM, Lindqvist JK. 2016. Biodiversity and palaeoecology of Foulden Maar: an early Miocene Konservat-Lagerstatte deposit in southern New Zealand. Alcheringa. 40(4):525–541.
- Leonard GS. 2003. The evolution of Maroa Volcanic Centre, Taupo Volcanic Zone, New Zealand [Ph.D. thesis ]: Christchurch, New Zealand, University of Canterbury. p. 332.
- Leonard GS, Calvert AT, Hopkins JL, Wilson CJN, Smid ER, Lindsay JM, Champion DE. 2017. High-precision Ar-40/Ar-39 dating of Quaternary basalts from Auckland Volcanic Field, New Zealand, with implications for eruption rates and paleomagnetic correlations. Journal of Volcanology and Geothermal Research. 343:60–74.
- Lexa J, Seghedi I, Németh K, Szakács A, Koneĉny V, Pécskay Z, Fülöp A, Kovacs M. 2010. Neogene-Quaternary volcanic forms in the Carpathian-Pannonian Region: a review. Central European Journal of Geosciences. 2(3):207–270.
- Lindqvist JK, Lee DE. 2009. High-frequency paleoclimate signals from Foulden Maar, Waipiata Volcanic Field, southern New Zealand: an Early Miocene varved lacustrine diatomite deposit. Sedimentary Geology. 222(1-2):98–110.
- Lindsay JM, Leonard GS, Smid ER, Hayward BW. 2011. Age of the Auckland Volcanic Field: a review of existing data. New Zealand Journal of Geology and Geophysics. 54(4):379–401.
- LINZ. 2012. Land Information New Zealand, NZ 8 m Digital Elevation Model. (data.linz.govt.nz).
- LINZ. 2013. Land Information New Zealand. Auckland LiDAR 1 m DEM. (data.linz.govt.nz).
- Lorenz V. 1986. On the growth of maars and diatremes and its relevance to the formation of tuff rings. Bulletin of Volcanology. 48:265–274.
- Lorenz V. 1987. Phreatomagmatism and its relevance. Chemical Geology. 62(1-2):149–156.
- Lorenz V. 2007. Syn- and posteruptive hazards of maar-diatreme volcanoes. Journal of Volcanology and Geothermal Research. 159(1-3):285–312.
- Lorenz V, Kurszlaukis S. 2007. Root zone processes in the phreatomagmatic pipe emplacement model and consequences for the evolution of maar-diatreme volcanoes. Journal of Volcanology and Geothermal Research. 159(1-3):4–32.
- Lorenz V, Suhr P, Suhr S. 2017. Phreatomagmatic maar-diatreme volcanoes and their incremental growth: a model. In: Németh K, Carrasco-Nuñez G, Aranda-Gomez JJ, Smith IEM, editors. Monogenetic volcanism. Bath, UK: The Geological Society Publishing House. https://pubs.geoscienceworld.org/books/book/2068/Monogenetic-Volcanism.
- Macorps E, Graettinger AH, Valentine GA, Ross P-S, White JDL, Sonder I. 2016. The effects of the host-substrate properties on maar-diatreme volcanoes: experimental evidence. Bulletin of Volcanology. 78(4). Article number: 26.
- Maicher D. 2003. A cluster of Surtseyan volcanoes at Lookout Bluff, North Otago, New Zealand: aspects of edifice spacing and time. In: White JDL, Smellie J, Clague D, editors. Explosive subaqueous volcanism. Geophysical Monograph Series. Vol. 140. Washington, DC: American Geophysical Union; p. 167–178.
- Manville V. 2001. Sedimentology and history of Lake Reporoa: anephemeral supra-ignimbrite lake, Taupo Volcanic Zone, NewZealand. In: White JDL, Riggs NR, editors. Volcanogenic sedi-mentation in lacustrine settings. International Association ofSedimentologists Special Publication; 30: 109–140.
- Mastin LG. 2007. Generation of fine hydromagmatic ash by growth and disintegration of glassy rinds. Journal of Geophysical Research-Solid Earth. 112(B2).
- Mastin LG, Christiansen RL, Thornber C, Lowenstern J, Beeson M. 2004. What makes hydromagmatic eruptions violent? Some insights from the Keanakako’i Ash, Kilauea Volcano, Hawai’i. Journal of Volcanology and Geothermal Research. 137(1-3):15–31.
- Mattsson HB. 2012. Rapid magma ascent and short eruption durations in the Lake Natron-Engaruka monogenetic volcanic field (Tanzania): a case study of the olivine melilititic Pello Hill scoria cone. Journal of Volcanology and Geothermal Research. 247:16–25.
- Mattsson HB, Tripoli BA. 2011. Depositional characteristics and volcanic landforms in the Lake Natron-Engaruka monogenetic field, northern Tanzania. Journal of Volcanology and Geothermal Research. 203(1-2):23–34.
- McLeod OE, White JDL. 2018. Petrogenetic links between the Dunedin Volcano and peripheral volcanics of the Karitane Suite. New Zealand Journal of Geology and Geophysics. 61(4):543–561.
- Mildenhall DC, Kennedy EM, Lee DE, Kaulfuss U, Bannister JM, Fox B, Conran JG. 2014. Palynology of the early Miocene Foulden Maar, Otago, New Zealand: diversity following destruction. Review of Palaeobotany and Palynology. 204:27–42.
- Mitchell JS, Mackay KA, Neil HL, Mackay EJ, Pallentin A. P. N 2012. Undersea New Zealand, 1:5,000,000.
- Moorhouse BL, White JDL. 2016. Interpreting ambiguous bedforms to distinguish subaerial base surge from subaqueous density current deposits. Depositional Record. 2(2):173–195.
- Moorhouse BL, White JDL, Scott JM. 2015. Cape Wanbrow: a stack of Surtseyan-style volcanoes built over millions of years in the Waiareka-Deborah volcanic field, New Zealand. Journal of Volcanology and Geothermal Research. 298:27–46.
- Morris PA. 1984. Petrology of the Campbell Island volcanics, southwest Pacific Ocean. Journal of Volcanology and Geothermal Research. 21:119–148.
- Morrissey MM, Zimanowski B, Wohletz K, Buettner R, Ballard RD. 2000. Phreatomagmatic fragmentation. Sigurdsson H, Houghton BF, McNutt SR, Rymer H, Stix J, editors. San Diego (CA): Academic Press.
- Mortimer N, Campbell HJ, Stagpoole M, Wood RA, Rattenbury MS, Sutherland R, Seton M. 2017. Zealandia: earth’s hidden continent. GSA Today. 27(3):27–35.
- Mortimer N, Rattenbury MS, King PR, Bland KJ, Barrell DJA, Bache F, Begg JG, Campbell HJ, Cox SC, Crampton JS, et al. 2014. High-level stratigraphic scheme for New Zealand rocks. New Zealand Journal of Geology and Geophysics. 57(4):402–419.
- Moufti MR, Németh K, El-Masry N, Qaddah A. 2015. Volcanic Geotopes and their Geosites preserved in an Arid climate related to landscape and climate changes since the Neogene in Northern Saudi Arabia: Harrat Hutaymah (Hai’il Region). Geoheritage. 7(2):103–118.
- Muraviev YD, Fedotov SA, Budnikov VA, Ozerov AY, Maguskin MA, Dvigalo VN, Andreev VI, Ivanov VV, Kartasheva LA, Markov IA. 1998. Volcanic activity in the Karymsky Center in 1996; summit eruption at Karymsky and phreatomagmatic eruption in the Akademii Nauk Caldera. Volcanology and Seismology. 19(5):567–604.
- Murcia H, Németh K, El-Masry NN, Lindsay JM, Moufti MRH, Wameyo P, Cronin SJ, Smith IEM, Kereszturi G. 2015. The Al-Du’aythah volcanic cones, Al-Madinah City: implications for volcanic hazards in northern Harrat Rahat, Kingdom of Saudi Arabia. Bulletin of Volcanology. 77(6).
- Nagaoka S, Okuno M. 2011. Tephrochronology and eruptive history of Kirishima Volcano in southern Japan. Quaternary International. 246(1-2):260–269.
- Nairn IA. 2002. Geology of the Okataina volcanic centre; sheets part U15, part U16, part V15 & part V16. Lower Hutt, New Zealand (NZL), Institute of Geological & Nuclear Sciences, Lower Hutt. Pp. 156-156, 1 sheet.
- Nelson CS, Cooke PJ. 2001. History of oceanic front development in the New Zealand sector of the Southern Ocean during the Cenozoic - a synthesis. New Zealand Journal of Geology and Geophysics. 44(4):535–553.
- Németh K. 2001. Long-term erosion-rate calculation from the Waipiata volcanic field (New Zealand) based on erosion remnants of scoria cones, tuff rings and maars. Geomorphologie. 2001(2):137–152.
- Németh K. 2003. Calculation of long-term erosion in Central Otago, New Zealand, based on erosional remnants of maar/tuff rings. Zeitschrift für Geomorphologie. 47(1):29–49.
- Németh K. 2010. Volcanic glass textures, shape characteristics and compositions of phreatomagmatic rock units from the Western Hungarian monogenetic volcanic fields and their implications for magma fragmentation. Central European Journal of Geosciences. 2(3):399–419.
- Németh K, Agustin-Flores J, Briggs R, Cronin SJ, Kereszturi G, Lindsay JM, Pittari A, Smith IEM. 2012a. Field guide: monogenetic volcanism of the South Auckland and Auckland Volcanic Fields. 4IMC Auckland, New Zealand 20-24 February 2012. Geoscience Society of New Zealand Miscellaneous Publication 131B. [ISBN 978-1-877480-16-4; ISSN Online 2230-4495; ISSN Print 2230-4487], pp. 1–72.
- Németh K, Cronin SJ. 2008. Volcanic craters, pit craters and high-level magma-feeding systems of a mafic island-arc volcano; Ambrym, Vanuatu, South Pacific. Geological Society Special Publications. 302:87–102.
- Németh K, Cronin SJ. 2011. Drivers of explosivity and elevated hazard in basaltic fissure eruptions: the 1913 eruption of Ambrym Volcano, Vanuatu (SW-Pacific). Journal of Volcanology and Geothermal Research. 201(1-4):194–209.
- Németh K, Cronin SJ, Haller MJ, Brenna M, Csillag G. 2010. Modern analogues for Miocene to Pleistocene alkali basaltic phreatomagmatic fields in the Pannonian Basin: “soft-substrate” to “combined” aquifer controlled phreatomagmatism in intraplate volcanic fields. Central European Journal of Geosciences. 2(3):339–361.
- Németh K, Cronin SJ, Smith IEM, Flores JA. 2012b. Amplified hazard of small-volume monogenetic eruptions due to environmental controls, Orakei Basin, Auckland Volcanic Field, New Zealand. Bulletin of Volcanology. 74(9):2121–2137.
- Németh K, Kereszturi G. 2015. Monogenetic volcanism: personal views and discussion. International Journal of Earth Sciences. 104(8):2131–2146.
- Németh K, Kósik S. 2020. Review of explosive hydrovolcanism. Geosciences. 10(2):44.
- Németh K, Martin U, Csillag G. 2007. Pitfalls in erosion level calculation based on remnants of maar and diatreme volcanoes. Geomorphologie-Relief Processus Environnement. 3:225–235.
- Németh K, Martin U, Harangi S. 2001. Miocene phreatomagmatic volcanism at Tihany (Pannonian Basin, Hungary). Journal of Volcanology and Geothermal Research. 111(1-4):111–135.
- Németh K, White JDL. 2003. Reconstructing eruption processes of a Miocene monogenetic volcanic field from vent remnants: Waipiata Volcanic Field, South Island, New Zealand. Journal of Volcanology and Geothermal Research. 124(1-2):1–21.
- Németh K, White JDL, Reay A, Martin U. 2003. Compositional variation during monogenetic volcano growth and its implications for magma supply to continental volcanic fields. Journal of the Geological Society of London. 160(4):523–530.
- Nicol A, Mazengarb C, Chanier F, Rait G, Uruski C, Wallace L. 2007. Tectonic evolution of the active Hikurangi subduction margin, New Zealand, since the Oligocene. Tectonics. 26(4):TC4002.
- Onken J, Forman S. 2017. Terminal Pleistocene to early Holocene volcanic eruptions at Zuni Salt Lake, west-central New Mexico, USA. Bulletin of Volcanology. 79(1). Article number: 10.
- Ort MH, Lefebvre NS, Neal CA, McConnell VS, Wohletz KH. 2018. Linking the Ukinrek 1977 maar-eruption observations to the tephra deposits: new insights into maar depositional processes. Journal of Volcanology and Geothermal Research. 360:36–60.
- Panisova J, Balazs A, Zalai Z, Bielik M, Horvath F, Harangi S, Schmidt S, Goetze H-J. 2018. Intraplate volcanism in the Danube Basin of NW Hungary: 3D geophysical modelling of the Late Miocene Pasztori volcano. International Journal of Earth Sciences. 107(5):1713–1730.
- Panter KS, Blusztajn J, Hart SR, Kyle PR, Esser R, McIntosh WC. 2006. The origin of HIMU in the SW Pacific: evidence from intraplate volcanism in southern New Zealand and subantarctic islands. Journal of Petrology. 47(9):1673–1704.
- Pardo N, Cronin SJ, Németh K, Brenna M, Schipper CI, Breard E, White JDL, Procter J, Stewart B, Agustin-Flores J, et al. 2014. Perils in distinguishing phreatic from phreatomagmatic ash; insights into the eruption mechanisms of the 6 August 2012 Mt. Tongariro eruption, New Zealand. Journal of Volcanology and Geothermal Research. 286:397–414.
- Pedrazzi D, Nemeth K, Adelina G, Alvarez-Valero AM, Aguirre-Diaz G, Bartolini S. 2018. Historic hydrovolcanism at Deception Island (Antarctica): implications for eruption hazards. Bulletin of Volcanology. 80:1.
- Prebble JG, Reichgelt T, Mildenhall DC, Greenwood DR, Raine JI, Kennedy EM, Seebeck HC. 2017. Terrestrial climate evolution in the Southwest Pacific over the past 30 million years. Earth and Planetary Science Letters. 459:136–144.
- Price RC, Coombs DS. 1975. Phonolitic lava domes and other features of the Dunedin Volcano, East Otago. Journal of the Royal Society of New Zealand. 5(2):133–152.
- Price RC, Cooper AF, Woodhead JD, Cartwright I. 2003. Phonolitic diatremes within the Dunedin Volcano, South Island, New Zealand. Journal of Petrology. 44(11):2053–2080.
- Ramon Avellan D, Luis Macias J, Pardo N, Scolamacchia T, Rodriguez D. 2012. Stratigraphy, geomorphology, geochemistry and hazard implications of the Nejapa Volcanic Field, western Managua, Nicaragua. Journal of Volcanology and Geothermal Research. 213:51–71.
- Reichgelt T, Kennedy EM, Jones WA, Jones DT, Lee DE. 2016. Contrasting palaeoenvironments of the mid/late Miocene Dunedin Volcano, southern New Zealand: climate or topography? Palaeogeography Palaeoclimatology Palaeoecology. 441:696–703.
- Risso C, Németh K, Combina AM, Nullo F, Drosina M. 2008. The role of phreatomagmatism in a Plio-Pleistocene high-density scoria cone field: Llancanelo Volcanic Field (Mendoza), Argentina. Journal of Volcanology and Geothermal. Research. 169(1-2):61–86.
- Rooney TO, Deering CD. 2014. Conditions of melt generation beneath the Taupo Volcanic Zone: the influence of heterogeneous mantle inputs on large-volume silicic systems. Geology. 42(1):3–6.
- Ross PS, Delpit S, Haller MJ, Németh K, Corbella H. 2011. Influence of the substrate on maar-diatreme volcanoes - An example of a mixed setting from the Pali Aike volcanic field, Argentina. Journal of Volcanology and Geothermal Research. 201(1-4):253–271.
- Schiøler P, Powell S, Rexilius J. 2014. PEP 38451 biostratigraphy of Romney-1 well (2370–4618 m) Deepwater Taranaki, New Zealand. New Zealand Unpublished Openfile Petroleum Report, PR4951.
- Schiøler P, Raine JI, Hollis CJ, Kulhanek DK, Morgans HEG, Roncaglia L, Strong CP, Uruski C. 2011. Revised biostratigraphy and well correlation, Canterbury Basin, New Zealand. GNS Science Consultancy Report 2011/12.: 1–142.
- Scott JM, Pontesilli A, Brenna M, White JDL, Giacalone E, Palin JM, le Roux PJ. 2020. The Dunedin Volcanic Group and a revised model for Zealandia’s alkaline intraplate volcanism. New Zealand Journal of Geology and Geophysics. 63(4):510–529.
- Scott JM, Turnbull IM. 2019. Geology of New Zealand’s Sub-Antarctic Islands. New Zealand Journal of Geology and Geophysics. 62(3):291–317.
- Scott JM, Turnbull IM, Auer A, Palin JM. 2013. The sub-Antarctic Antipodes Volcano: a <0.5 Ma HIMU-like Surtseyan volcanic outpost on the edge of the Campbell Plateau, New Zealand. New Zealand Journal of Geology and Geophysics. 56(3):134–153.
- Segev A, Rybakov M, Mortimer N. 2012. A crustal model for Zealandia and Fiji. Geophysical Journal International. 189(3):1277–1292.
- Seghedi I, Szakacs A, Pacheco AH, Matesanz JLB. 2007. Miocene lamproite volcanoes in south-eastern Spain - an association of phreatomagmatic and magmatic products. Journal of Volcanology and Geothermal Research. 159(1-3):210–224.
- Self S. 1983. Large-scale phreatomagmatic silicic volcanism; a case study from New Zealand. Journal of Volcanology and Geothermal Research. 17(1-4):433–469.
- Self S, Kienle J, Huot JP. 1980. Ukinrek Maars, Alaska 2. Deposits and formation of the 1977 craters. Journal of Volcanology and Geothermal Research. 7(1-2):39–65.
- Sheridan MF, Wohletz KH. 1983. Hydrovolcanism - Basic considerations and review. Journal of Volcanology and Geothermal Research. 17(1-4):1–29.
- Shoemaker EM. 1957. Primary structures of maar rims and their bearing on the origin of Kilbourne Hole and Zuni Salt Lake, New Mexico. Geological Society of America Bulletin. 68(12, Part 2):1846–1846.
- Smith IEM, Briggs RM. 2008. Hot spots in the North. Geological Society of New Zealand Miscellaneous Publication. 124:158–161.
- Smith RT, Houghton BF. 1995. Vent migration and changing eruptive style during the 1800a Taupo eruption: new evidence from the Hatepe and Rotongaio phreatoplinian ashes. Bulletin of Volcanology. 57(6):432–439.
- Smith IEM, Németh K. 2017. Source to surface model of monogenetic volcanism: a critical review. In: Németh K, Carrasco-Nuñez G, Aranda-Gomez JJ, Smith IEM, editors. Monogenetic volcanism. Bath (UK): The Geological Society Publishing House; p. 1–28.
- Sohn YK, Chough SK. 1989. Depositional processes of the Suwolbong Tuff Ring, Cheju Island (Korea). Sedimentology. 36(5):837–855.
- Sohn YK, Cronin SJ, Brenna M, Smith IEM, Németh K, White JDL, Murtagh RM, Jeon YM, Kwon CW. 2012. Ilchulbong tuff cone, Jeju Island, Korea, revisited: a compound monogenetic volcano involving multiple magma pulses, shifting vents, and discrete eruptive phases. Geological Society of America Bulletin. 124(3-4):259–274.
- Sonder I, Harp AG, Graettinger AH, Moitra P, Valentine GA, Buettner R, Zimanowski B. 2018. Meter-Scale experiments on magma-water interaction. Journal of Geophysical Research-Solid Earth. 123(12):10597–10615.
- Sorrentino L, Cas RAF, Stilwell JD. 2011. Evolution and facies architecture of Paleogene Surtseyan volcanoes on Chatham Islands, New Zealand, Southwest Pacific Ocean. Journal of Volcanology and Geothermal Research. 202(1-2):1–21.
- Sorrentino L, Stilwell JD, Mays C. 2014. A model of tephra dispersal from an early Palaeogene shallow submarine Surtseyan-style eruption(s), the Red Bluff Tuff Formation, Chatham Island, New Zealand. Sedimentary Geology. 300:86–102.
- Stárková M, Rapprich V, Breitkreuz C. 2011. Variable eruptive styles in an ancient monogenetic volcanic field: examples from the permian levín volcanic field (krkonoše piedmont basin, Bohemian massif). Journal of Geosciences. 56(2):163–180.
- Stilwell JD, Consoli CP. 2012. Tectono-stratigraphic history of the Chatham Islands, SW Pacific-The emergence, flooding and reappearance of eastern ‘Zealandia’. Proceedings of the Geologists Association. 123(1):170–181.
- Suhr P, Goth K, Lorenz V, Suhr S. 2006. Long lasting subsidence and deformation in and above maar-diatreme volcanoes - a never ending story. Zeitschrift der Deutschen Gesellschaft für Geowissenschaften. 157(3):491–511.
- Suhr P, Lorenz V, Goth K. 2004. Subsidence within and above maar-diatreme volcanoes. Abstract Volume of the 2nd International Maar Conference Occasional Papers of the Geological Institute of Hungary. 203; p. 94.
- Sutherland R, Collot J, Bache F, Henrys S, Barker D, Browne GH, Lawrence MJF, Morgans HEG, Hollis CJ, Clowes C, et al. 2017. Widespread compression associated with Eocene Tonga-Kermadec subduction initiation. Geology. 45(4):355–358.
- Sutherland R, King PR, Wood RA. 2001. Tectonic evolution of Cretaceous rift basins in south-eastern Australia and New Zealand: implications for exploration risk assessment. In: Hill KC, Bernecker T, editors. Eastern Australasian Basins Symposium 2001. Melbourne (Australia): Petroleum Exploration Society of Australia; p. 3–13.
- Swanson DA, Zolkos SP, Haravitch B. 2012. Ballistic blocks around Kilauea Caldera; their vent locations and number of eruptions in the late 18th century. Journal of Volcanology and Geothermal Research. 231–232:1–11.
- Sweeney MR, Grosso ZS, Valentine GA. 2018. Topographic controls on a phreatomagmatic maar-diatreme eruption: field and numerical results from the Holocene Dotsero volcano (Colorado, USA). Bulletin of Volcanology. 80:11.
- Thompson NK, Bassett KN, Reid CM. 2014. The effect of volcanism on cool-water carbonate facies during maximum inundation of Zealandia in the Waitaki-Oamaru region. New Zealand Journal of Geology and Geophysics. 57(2):149–169.
- Timm C, Hoernle K, Werner R, Hauff F, van den Bogaard P, White J, Mortimer N, Garbe-Schonberg D. 2010. Temporal and geochemical evolution of the Cenozoic intraplate volcanism of Zealandia. Earth-Science Reviews. 98(1-2):38–64.
- Uruski CI. 2020. Seismic recognition of igneous rocks of the Deepwater Taranaki Basin, New Zealand, and their distribution. New Zealand Journal of Geology and Geophysics. 63(2):190–209.
- Valentine GA. 2012. Shallow plumbing systems for small-volume basaltic volcanoes, 2: evidence from crustal xenoliths at scoria cones and maars. Journal of Volcanology and Geothermal Research. 223:47–63.
- Valentine GA, Connor CB. 2015. Chapter 23 - basaltic volcanic fields. In: Sigurdsson H, editor. The encyclopedia of volcanoes. 2nd ed. Amsterdam: Academic Press; p. 423–439.
- Valentine GA, Cortes JA, Widom E, Smith EI, Rasoazanamparany C, Johnsen R, Briner JP, Harp AG, Turrin B. 2017a. Lunar Crater volcanic field (Reveille and Pancake Ranges, Basin and Range Province, Nevada, USA). Geosphere. 13(2):391–438.
- Valentine GA, Graettinger AH, Macorps E, Ross P-S, White JDL, Doehring E, Sonder I. 2015. Experiments with vertically and laterally migrating subsurface explosions with applications to the geology of phreatomagmatic and hydrothermal explosion craters and diatremes. Bulletin of Volcanology. 77(3). Article number: 15.
- Valentine GA, White JDL. 2012. Revised conceptual model for maar-diatremes: subsurface processes, energetics, and eruptive products. Geology. 40(12):1111–1114.
- Valentine GA, White JDL, Ross P-S, Graettinger AH, Sonder I. 2017b. Updates to Concepts on phreatomagmatic Maar-diatremes and their pyroclastic deposits. Frontiers in Earth Science. 5. https://doi.org/10.3389/feart.2017.00068.
- van der Meer QHA, Waight TE, Scott JM, Munker C. 2017. Variable sources for Cretaceous to recent HIMU and HIMU-like intraplate magmatism in New Zealand. Earth and Planetary Science Letters. 469:27–41.
- Van Eaton AR, Herzog M, Wilson CJN, McGregor J. 2012. Ascent dynamics of large phreatomagmatic eruption clouds; the role of microphysics. Journal of Geophysical Research. 117(B3): 0-Citation B03203.
- Van Niekerk R. 2016. Reconstructing the complex history of a small-volume basaltic volcano (Ngatutura volcanic field, New Zealand): the role of subsurface processes and implications for diatreme formation: a thesis presented in partial fulfilment of the requirements for the degree of Master of Science in Earth Science at Massey University, Palmerston North, New Zealand. Unpublished Masters thesis, Massey University.
- van Niekerk R, Lube G, Németh K. 2015a. New constraints on subsurface processes of monogenetic volcanism; an example from the Ngatutura volcanic field, Waikato, New Zealand. Newsletter - Geoscience Society of New Zealand. 17:8–9.
- van Niekerk R, Lube G, Németh K. 2015b. The role of primary explosive processes on the formation of diatremes; the Ngatutura Diatreme, New Zealand. Geoscience Society of New Zealand Miscellaneous Publication. 143A:136.
- van Niekerk R, Németh K, Lube G. 2014. The roots of a monogenetic volcano; hyaloclastites, dykes and diatremes reveal a complex history of Pliocene volcanism along the Waikato coast, New Zealand. Geoscience Society of New Zealand Miscellaneous Publication. 139A:111–112.
- van Otterloo J, Cas RAF. 2013. Reconstructing the eruption magnitude and energy budgets for the pre-historic eruption of the monogenetic similar to 5 ka Mt. Gambier Volcanic Complex, south-eastern Australia. Bulletin of Volcanology. 75(12). Article number: 769.
- van Otterloo J, Cas RAF, Sheard MJ. 2013. Eruption processes and deposit characteristics at the monogenetic Mt. Gambier Volcanic Complex, SE Australia: implications for alternating magmatic and phreatomagmatic activity. Bulletin of Volcanology. 75(8). Article number: 737.
- Walker GPL. 1993. Basaltic-volcano systems. Geological Society Special Publications. 76:3–38.
- White JDL. 1991. Maar-diatreme phreatomagmatism at Hopi Buttes, Navajo Nation (Arizona), USA. Bulletin of Volcanology. 53:239–258.
- White JDL, Schipper CI, Kano K. 2015. Chapter 31 - submarine explosive eruptions. In: Sigurdsson H, editor. The Encyclopedia of volcanoes. 2nd ed. Amsterdam: Academic Press; p. 553–569.
- White JDL, Valentine GA. 2016. Magmatic versus phreatomagmatic fragmentation: absence of evidence is not evidence of absence. Geosphere. 12(5):1478–1488.
- Wijbrans J, Németh K, Martin U, Balogh K. 2007. Ar-40/Ar-39 geochronology of Neogene phreatomagmatic volcanism in the western Pannonian Basin, Hungary. Journal of Volcanology and Geothermal Research. 164(4):193–204.
- Wilson CJN. 1993. Stratigraphy, chronology, styles and dynamics of Late Quaternary eruptions from Taupo Volcano, New-Zealand. Philosophical Transactions of The Royal Society of London Series A-Mathematical Physical and Engineering Sciences. 343(1668):205–306.
- Wilson CJN. 1994. Ash-fall deposits from large-scale phreatomagmatic volcanism: limitations of available eruption column models. In: Casadevall TJ, editor. Volcanic ash and aviation safety: Proceedings of the first international conference on volcanism and aviation safety (U.S. Geological Survey Bulletin 2047). Wasington, DC: U.S. Geological Survey; p. 93–99.
- Wilson CJN. 2001. The 26.5 ka Oruanui eruption, New Zealand: an introduction and overview. Journal of Volcanology and Geothermal Research. 112(1-4):133–174.
- Wilson CJN, Houghton BF, McWilliams MO, Lanphere MA, Weaver SD, Briggs RM. 1995. Volcanic and structural evolution of Taupo Volcanic Zone, New-Zealand - A review. Journal of Volcanology and Geothermal Research. 68(1-3):1–28.
- Wilson CJN, Rowland JV. 2016. The volcanic, magmatic and tectonic setting of the Taupo Volcanic Zone, New Zealand, reviewed from a geothermal perspective. Geothermics. 59:168–187.
- Wilson CJN, Smith IEM. 1985. A basaltic phreatomagmatic eruptive centre at Acacia Bay, Taupo volcanic centre. Journal of the Royal Society of New Zealand. 15(3):329–337.
- Wong LJ, Larsen JF. 2010. The Middle Scoria sequence: a Holocene violent strombolian, subplinian and phreatomagmatic eruption of Okmok volcano, Alaska. Bulletin of Volcanology. 72(1):17–31.
- Zimanowski B, Buettner R, Lorenz V. 1997a. Premixing of magma and water in MFCI experiments. Bulletin of Volcanology. 58(6):491–495.
- Zimanowski B, Buettner R, Lorenz V, Haefele H-G. 1997b. Fragmentation of basaltic melt in the course of explosive volcanism. Journal of Geophysical Research. 102(B1):803–814.