ABSTRACT
Many volcanoes are buried within Te Riu-a-Māui/Zealandia sedimentary basins. Based on a large collection of seismic reflection and drillhole data, this paper outlines the morphology, location and age of over 400 volcanoes buried and preserved in offshore New Zealand basins. We discuss the relationships of these volcanoes to the timing and locations of the main tectonic events that shaped Zealandia in the last 105 Myr. Late Cretaceous volcanism mainly formed large (>20 km3) composite cones and volcanic complexes that erupted along rift faults related to the break-up of Zealandia from south-eastern Gondwana. Cenozoic intraplate volcanic activity was widespread, typically forming clusters of scattered small-volume (<1 km3) craters and cones. In contrast, large Cenozoic volcanic complexes, composite cones and shield volcanoes erupted along linear arcs associated with subduction along the Pacific-Australian plate boundary. In detail, the presence of pre-existing structures and types of host rocks also influence the passage of magma in the shallow (<5 km) crust and the location of eruptive centres, regardless the tectonic setting of eruption. Insights from this work demonstrate the value of characterising volcanoes buried in sedimentary basins for improved understanding of the interplay between volcanism and plate tectonics in Zealandia and further afield.
Introduction
Over the last 150 years, geological mapping and studies of igneous rocks from onshore New Zealand, New Caledonia and several surrounding small islands have provided an enormous amount of information about volcanic activity in Te Riu-a-Māui/Zealandia region (e.g. Hochstetter Citation1867; Marshall Citation1932; Mortimer and Scott Citation2020). More recently, onshore information has been supplemented by oceanographic data from dredging the sea floor above submarine seamounts, ridges and plateaus across Zealandia (e.g. Timm et al. Citation2010; Bache, Sutherland, et al. Citation2014; Hoernle et al. Citation2020). These complementary datasets are the basis for current geological models describing the syn-and post Gondwana break-up volcanism in Zealandia (e.g. Hoernle et al. Citation2006; Mortimer et al. Citation2018; Van der Meer et al. Citation2018). However, to date, few studies have had incorporated detailed information from volcanoes buried within Zealandia sedimentary basins (e.g. Herzer Citation1995; Uruski Citation2019; Barrier Citation2019).
From coastal exposures, geologists have long suspected that large eroded volcanoes could extend beneath the New Zealand continental shelf and possibly beyond. It was not until the 1960s that igneous rocks were first documented within sedimentary basins in offshore New Zealand. Initially, recognition of these buried igneous systems was inferred by remote sensing techniques such as seismic reflection, gravimetric and magnetometric surveys acquired during petroleum exploration campaigns (e.g. Hatherton Citation1968; Knox Citation1982; Hayward Citation1987). Oil and gas discoveries within the onshore Taranaki Basin stimulated further offshore exploration, including the acquisition of new seismic surveys and drillholes (). Many of these offshore drillholes encountered volcanic and plutonic rocks of various ages confirming an igneous origin of the anomalies recognised from geophysical data (e.g. Milne Citation1975; Field et al. Citation1989; Bergman et al. Citation1992).
Figure 1. Map showing the seismic dataset, main regional physiography, sedimentary basins, and location of offshore volcanoes presented in this study. Basins highlighted in darker yellow are discussed in this paper. Numbers and abbreviations are: (1) Vulcan-Romney volcanic zone; (2) Tikati volcanic complex; (3) Matuku intrusions and small vents; (4) Kaiwero volcanic field; (5) Northland-Mohakatino volcanic belts; (6) Aotea volcanic complex; (7) West Ngatutura volcanic field; (8) Sloop volcanic complex; (9) Barque volcanic complex; (10) Tapuku East volcanic complex; (11) East Waiareka-Deborah volcanic field; (12) Papatowai volcanic field; (13) Maahunui volcanic field; (14) Waka intrusions and small vents; (15) Toroa volcanic field; (TVZ) Taupo Volcanic Zone; (T. Kings R.) Three Kings Ridge. Volcanoes numbered in grey circles are only shown in the supplementary material of this paper. Background image shows a Digital Terrain Model from NIWA (New Zealand Institute of Water and Atmosphere).
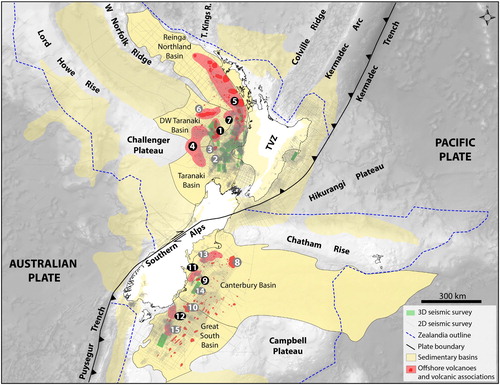
In the past few decades, an increasing quality and coverage of seismic surveys onshore and offshore New Zealand have enabled us to apply a systematic methodology for establishing the locations, timing and morphologies of a variety of igneous-dominated structures belonging to diverse magmatic systems (i.e. rift, intraplate, and subduction-related; ). This paper builds on and extends existing knowledge mainly from Field et al. (Citation1989), Herzer (Citation1995), King and Thrasher (Citation1996), Giba et al. (Citation2013), Seebeck et al. (Citation2014), Bischoff (Citation2019), Barrier (Citation2019) and Uruski (Citation2019), proposing a systematic classification of the best understood examples of volcanoes buried offshore New Zealand. We focus mainly on describing volcanoes buried in the Reinga-Northland, Taranaki, Deepwater Taranaki, Canterbury and Great South basins, where seismic reflection and drillhole data are present in great quantity (). Additional volcanoes are likely buried in other parts of Zealandia (e.g. Norfolk Ridge, Lord Howe Rise, and west of the Puysegur Trench), but because of limited seismic and drillhole coverage, these areas were excluded from this study. and show the nomenclature and main references for the volcanoes presented here. Additional information is available in Supplementary Materials 1–9.
Figure 2. Simplified Late Cretaceous-Cenozoic volcano-stratigraphic framework and main tectonic events offshore New Zealand. Symbols in black indicate the volcanic type and are: composite cone (CC), volcanic complex (VC), volcanic field (VF), caldera (Ca), shield (Sh), intrusions and small-volume vents (IV). Colours in the bars correspond to the active phase of the volcanoes and their tectonic setting of eruptions and are: syn-rift (dark green), immediate post-rift (green), scattered intraplate (blue), subduction-related (red). Numbers in red are the estimated volume of erupted magma in km3. Vertical lines represent uncertainties in the age of the volcanoes.
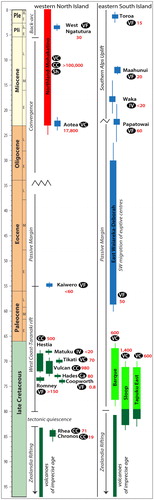
Table 1. Nomenclature and main references of the volcanoes buried in the Taranaki, Deepwater Taranaki and Reinga-Northland basins.
Table 2. Nomenclature and references of the volcanoes buried in the Canterbury and Great South basins.
The results of this study may have an application to those undertaking detailed investigation of volcanoes or volcanic fields buried in sedimentary basins, for improved understanding of regional volcanism across Zealandia and Gondwana, and for development of models relating volcanism and tectonics.
Geological Background
Zealandia is a region of 4.9 million km2 largely (ca 95%) submerged in the Pacific Ocean and Tasman Sea (Mortimer and Campbell Citation2017). It consists of Cambrian-Early Cretaceous basement overlain by Late Cretaceous-Cenozoic sedimentary and igneous rocks (Laird and Bradshaw Citation2004; Mortimer et al. Citation2018). For most of the Mesozoic, Zealandia formed the active convergent south-eastern margin of the Gondwana supercontinent, which separated from Australia and Antarctica in the Late Cretaceous (e.g. Laird Citation1993; Sutherland et al. Citation2001; Mortimer Citation2004). An initial phase of crustal extension (ca 105–83 Ma) leading to continental break-up gave rise to several rift basins throughout Zealandia. These basins were subsequently filled with Late Cretaceous and younger strata up to 10 km thick. In and around the New Zealand landmass, these basins were affected by Eocene to Recent plate boundary deformation associated with the Hikurangi and Puysegur subduction zones, and dextral strike-slip transpression along the Alpine Fault (e.g. Walcott Citation1978; King et al. Citation1999; Nicol et al. Citation2007; and ).
Most sedimentary basins within Zealandia have experienced recurrent and widespread igneous activity (e.g. Field et al. Citation1989; King and Thrasher Citation1996; Sahoo et al. Citation2015). Late Cretaceous-Cenozoic magmatism formed numerous volcanic zones, complexes, fields and centres. These volcanoes are part of the Zealandia Megasequence and are grouped into the Rūaumoko Volcanic Region, broadly classified as having an intraplate (Horomaka Supersuite) or a subduction-related (Whakaari Supersuite) origin (Mortimer et al. Citation2014).
Intraplate volcanoes are predominantly basaltic to trachytic and phonolitic in composition, typically forming clusters of scattered small-volume volcanoes (<1 km3), and less common large polygenetic volcanic systems not associated with plate boundaries or hotspot tracks. These include those of Waiareka-Deborah and Auckland volcanic fields (e.g. Coombs et al. Citation1986; Kereszturi and Németh Citation2016; Hopkins et al. Citation2020), as well as the large volcanic complexes that shaped the Otago and Banks peninsulas (e.g. Sewell Citation1988; Weaver and Smith Citation1989; Scott et al. Citation2020). In contrast, the convergent Australian-Pacific plate boundary formed linear, mainly calc-alkaline basalt, andesite, dacite and rhyolite-dominated volcanic arcs extending from oceanic crust north of Zealandia onto continental crust towards the North Island of New Zealand (e.g. Cole and Lewis Citation1981; King Citation2000; Sutherland et al. Citation2017). The arcs broadly show the migration of eruptive centres to the SE, formerly extending along the Three Kings Ridge to Northland region (ca 25–17 Ma), and from the Lau-Colville Ridge across Coromandel Peninsula to the Mohakatino belt (ca 16–4 Ma). The youngest modern arc (ca 2–0 Ma) extends southwards from the Tonga-Kermadec trend into the Bay of Plenty and Taupo Volcanic Zone (e.g. Herzer Citation1995; Mortimer et al. Citation2010; Seebeck et al. Citation2014; and ).
Data and methods
Data used in this paper has been mainly sourced from the 2018 New Zealand Petroleum and Minerals Exploration Data Pack, which includes a large collection of reports, maps, drillhole records, 2D and 3D seismic surveys. In this study, buried volcanoes were identified using a combination of seismic reflection and drillhole data interpretation. The spacing of the seismic lines is typically <2 km, with 3D datasets having line spacing of ≤12.5 m () and vertical resolution is usually a few tens of metres. Drillhole data commonly include wireline-logs together with lithological, geochemical, geochronological, and biostratigraphic information from cutting samples and sometimes from drill cores.
The age of the volcanic rocks in the subsurface is rarely determined by radiometric dating and is primarily achieved by mapping chronostratigraphic surfaces that correlate seismic anomalies possibly of igneous origin with biostratigraphic markers identified in the drillholes. Chronostratigraphic mapping follows seismic and sequence stratigraphic principles such as stratal reflection relationships and depositional trends within seismic facies (e.g. Mitchum and Vail Citation1977; Hunt and Tucker Citation1992; Catuneanu Citation2006), giving time resolution in the order of few (<5 Myr), similar to the precision of radiometric ages. Interpretation of the environment in which the eruptions occurred was determined by plotting the location of volcanoes on paleogeographic maps of similar age (Arnot et al. Citation2016), and/or by calibration with paleoenvironmental data obtained from microfossils from drillholes across the studied areas.
Detailed characterisation of the buried volcanoes is based on criteria such as the geometry, internal and external configuration of seismic reflections, deformation of enclosing strata, and stratal relationships within the seismic images (Mitchum and Vail Citation1977), together with analysis of the volcanic and sub-volcanic architecture (e.g. Bischoff et al. Citation2017, Bischoff, Nicol, Cole, et al. Citation2019, Bischoff, Nicol, Barrier, et al. Citation2019, Bischoff, Rossetti, et al. Citation2019). Optimal characterisation can be obtained by applying a range of techniques such as seismic attribute analysis, opacity rendering of the seismic signal, decomposition and merging of an array of frequencies of the seismic wavelength spectrum, and 3D mapping of geobodies with the similar seismic response (e.g. Chopra and Marfurt Citation2005; Planke et al. Citation2017; Marfurt Citation2018).
The height of the buried volcanoes is estimated from their present-day morphology (i.e. after erosion and compaction during burial) by recording the distance between the pre- and post-eruptive surfaces in two-way-travel-time (TWT) in seconds and multiplying it by the estimated acoustic velocity of the volcanic rocks within the seismic anomaly. Massive and unaltered basalts typically have acoustic velocities between 5000 and 7000 m/s, while velocities of non-welded stratified pyroclastic rocks are commonly around 3000 m/s (e.g. Planke et al. Citation2000; Holford et al. Citation2012; Klarner and Klarner Citation2012). These values were calibrated with data available in the literature (e.g. Heap and Kennedy Citation2016; Millett et al. Citation2016; Cant et al. Citation2018), and when possible, with velocities of volcanic rocks recorded in wireline-logs from drillholes penetrating the buried volcanoes (e.g. Milne Citation1975; Field et al. Citation1989; Rad Citation2015). Using our analysis, each buried volcanic edifice was approximated as a cone, a trapezoidal prism, or as a spherical cap to roughly estimate the volume of erupted material. As explained in the literature (e.g. Magee et al. Citation2019; Reynolds et al. Citation2016; Bischoff, Nicol, Barrier, et al. Citation2019), these estimations are only approximate, but variations in the acoustic velocities of volcanic rocks do not affect the first-order interpretations of volcano morphologies.
Northern Zealandia
Vulcan–Romney volcanic zone – Late Cretaceous
Vulcan–Romney volcanic zone comprises seven large Late Cretaceous volcanoes, as well as several small vents and numerous intrusive bodies presently buried beneath ca 4000 m of sedimentary strata in the Deepwater Taranaki Basin (). This group of volcanoes has been named in petroleum reports and the literature after gods and goddesses of Greek mythology, as well as after sheep breeds common in New Zealand. We follow this nomenclature where possible, and extend it to describe volcanoes not previously named ().
Figure 3. (a) Regional map of the post-eruptive surface of the Vulcan-Romney volcanic zone (ca 68 Ma). Numbers are: (1) Chronos composite volcano; (2) Rhea composite volcano; (3) Coopworth volcanoes; (4) Romney volcanic field; (5) Hades caldera; (6) Vulcan composite volcano; and (7) Hestia composite volcano. (b) 2D cross-section across the Romney volcanic field. Vertical black arrows indicate the location of eruptive vents. Blue lines correspond to fault planes and red lines to the location of possible conduits. (c) Plan view map of Coopworth volcanoes and Romney volcanic field. Dots are located at the position of eruptive vents. Dashed black lines mark the terminal front of lava-flow deposits and the boundaries of the volcanic fields. (d) Detailed structural map of the post-eruptive surface of the Hades caldera at ca 74 Ma.
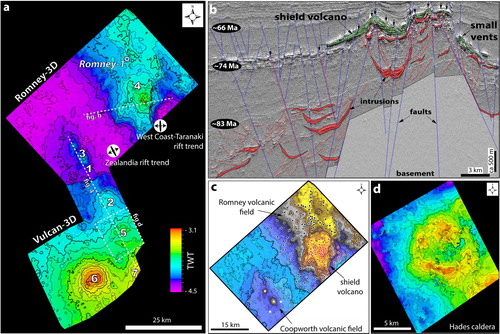
Late Cretaceous volcanic activity in the Deepwater Taranaki Basin was initially inferred by the interpretation of seismic reflection lines (McAlpine Citation1999; Uruski and Warburton Citation2010). In 2014, the Romney-1 drillhole penetrated a sequence of volcanic rocks interbedded with coal measures of Haumurian age (83.6–66 Ma), as well as sandstones and mudstones of the Rakopi Formation, confirming a volcanic origin of the anomalies observed in seismic imagery. These volcanic rocks are overlain by shallow marine sandstones and siltstones of the latest Cretaceous North Cape Formation, indicating that eruptions occurred near paleo-shoreline (Schiøler et al. Citation2014; Uruski Citation2019). Drill cuttings were initially identified as moderately- to highly-altered tuffs and epiclastic rocks of basaltic composition (Rad Citation2015), but our petrographic, SEM and EDS analysis indicates that both mafic and felsic (possibly alkaline) rocks are present in the Romney-1 drillhole (Supplementary Material 1).
Vulcan-Romney volcanoes erupted in two phases (Zhu et al. Citation2017). Eruptions in the Chronos volcano mark the onset of volcanic activity in the area at ca 85 Ma. The present-day morphology of this volcano is semi-conical, 4.5 km wide across the base of the cone and ca 900 m high. Rhea erupted shortly after Chronos, and also forms a semi-conical edifice at least 7 km in width and ca 1400 m high ( and ). The large volume of each edifice (>20 km3) suggests that they were constructed by multiple eruptive cycles, rather than a single volcanic episode. Both volcanoes are aligned in an NW orientation parallel with the contemporaneous trend of the Zealandia Rift (Strogen et al. Citation2017).
Figure 4. Uninterpreted (a) and interpreted (b) 2D seismic section showing the morphology and stratigraphic relationships of some of the large volcanoes of the Vulcan-Romney volcanic zone. Po is the post-eruptive surface of each volcano, while Pr is the pre-eruptive surface, these surfaces are the upper and lower bounds of each coloured polygon. Numbers indicate the stratigraphic order relative to the onset of volcanic activity in each volcano. Note that some volcanoes interfinger (e.g. Vulcan and Hestia) indicating that volcanic activity was contemporaneous, while in others (i.e. Coopworth and Hades) the Pr and Po amalgamate with increasing distance from the eruptive centre, indicating that eruptions were relatively short-lived compared to the rate of sedimentary and volcanic processes that control the local basin infill. Blue lines correspond to fault planes and red lines indicate possible magma conduits. The location of this line is shown in .
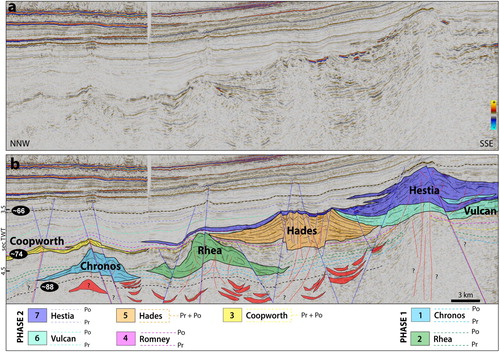
The onset of the second eruptive phase is marked by eruptions in the Coopworth volcanoes at ca 74 Ma ( and ), which comprise two main small-volume cones and minor scattered vents. The northern volcano is smaller (1000 m across and 160 m high) than the southern (1800 m across and 200 m high). The small relief edifices are associated with large peripheral aprons, suggesting a morphology observed in fields of scattered spatter cones and extensive lava fields, a characteristic observed in basaltic monogenetic volcanic fields (Németh Citation2010).
Romney volcanic field erupted shortly after Coopworth volcanoes and comprises a large shield volcano, as well as numerous (possibly hundreds) scattered small-volume vents and several shallow intrusions typically of saucer-shaped morphology ((c) and ). The large shield volcano is 15 km in width and ca 400 m high as preserved in the basin. Its flanks contain many parasitic vents preferentially orientated parallel with normal faults ((b)), which globally is commonly observed in volcanoes associated with rifting and extension (e.g. Galland et al. Citation2014; Burchardt et al. Citation2018; Vries and Vries Citation2018). The extremities of the shield volcano show high-amplitude reflections with ‘toe-like’ terminations ((c)), a morphology typically associated with both Pahoehoe and a′ā lava flows sourced in Hawaiian eruptions (Gregg Citation2017). In contrast to the shield volcano, the scattered small-volume vents tend to show cone-type morphology with extensive flat-lying aprons of high-amplitude reflections that possibly correspond to spatter cones and related lava fields (). These vents are preferentially aligned with NW-SE to NE-SW normal faults ((c)). In the western part of the Romney field, there is a narrow NE trending structure that resembles a fissure vent ((a)), which is also located along a normal fault, reinforcing the interpretation of volcanic activity simultaneously with extension, as commonly described in rift volcanoes (Sigmundsson et al. Citation2018; Neal et al. Citation2019).
Figure 5. (a) Plan view map of the Romney volcanic field revealed by spectral decomposition at the 4100 ms time-slice. (b) Opacity rendering of the Romney-3D showing the morphology of a double-summit shield-like volcano composed by several eruptive vents. (c) Time-slice section at 4200 milliseconds coupled with a 2D cross-section across the shield volcano shown in (b). Note the ‘bird-foot’ morphology highlighting the termination of lava flows, while the concentric structures mark the location of conduits. S1 and S2 are mapped summits of the shield volcanoes. WCTRT is the West Coast-Taranaki rift trend. Note the structural control of faults in the location of eruptive vents.
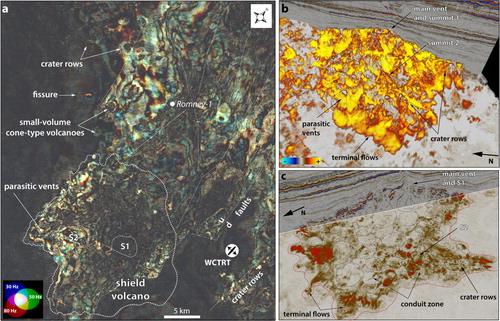
Volcanic activity in Hades caldera occurred simultaneously or immediately after eruptions in Romney volcanic field, at around 73 Ma. Hades has a semi-circular structure 10 km across and ca 800 m high with a central depression 3.5 km wide and 1 km deep bounded by ring faults ((d) and ). Its bedded circular flanks suggest that material was spread relatively uniformly and deposited in tabular layers around the vent, as is common with high energy pyroclastic eruptions (Kereszturi and Németh Citation2013), while a central depression and ring faults are frequently observed in explosive and non-explosive caldera volcanoes (Cole et al. Citation2005; Martí et al. Citation2008). The moderate amplitude and bedded reflections on Hades flanks suggest deposition of fragmented material more likely sourced by explosive eruptions.
Vulcan and Hestia are two large long-lived composite volcanoes that interfinger with each other and with basin strata deposited over at least 8 Myr ((a)). Vulcan is 25 km in width and ca 1500 high, while Hestia is 22 km wide and ca 1000 m high. Volcanic activity started in Vulcan at ca 74 Ma and ended while Hestia was still active, which is confirmed by the western flank of Hestia overlapping the eastern flank of Vulcan (). Eruptions ceased in Hestia at around 68 Ma, marking the end of volcanism in the Vulcan-Romney volcanic zone. The magma composition and eruptive mechanisms that constructed Vulcan and Hestia remain poorly calibrated in absence of drillholes, but possibly include pyroclastic and lava-flow deposits and volcanogenic sedimentary rocks, as common of large long-lived composite volcanoes (Manville et al. Citation2009; de Silva and Lindsay Citation2015). Both volcanoes are located along N-S trending faults parallel with the trend of the West Coast-Taranaki rift (Strogen et al. Citation2017).
Kaiwero volcanic field – Eocene
The Kaiwero volcanic field is a cluster of >50 scattered Eocene small-volume volcanoes and several shallow (<1 km) intrusions currently buried by 300–1500 m of sedimentary strata offshore western North Island, New Zealand (). The name is derived from the Māori for the large submarine plateau (Challenger Plateau) adjacent to the area where the volcanoes are located ( and ).
Figure 6. (a) Map showing the distribution of volcanoes in the Kaiwero volcanic field and available 2D seismic grid. The coloured bars show the location and present burial depth of the volcanoes in TWT. The area highlighted in pink corresponds to the limit of occurrence of intrusive and extrusive bodies of Eocene age. (b) 2D seismic section (line MER10-004) showing a small cone-type volcano located at the tip of a saucer-shaped intrusion. Both the pre-eruptive surface and forced folding above the intrusions indicate an Eocene age. Red lines represent the location of possible magma conduits. (c) 2D seismic section (line DTB01-20) showing a small cone-type volcano and an intrusion, both of Eocene age. Numbers are: (1) intrusions; (2) jacked-up dome; (3) conduits; (4) seismic artefact (loss of reflectivity); (5) cone-type volcano.
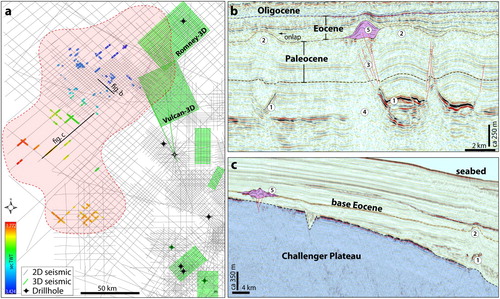
Volcanism occurred over an area of ca 17,000 km2, extending from the NE boundary of the Challenger Plateau towards the SW Deepwater Taranaki Basin. Volcanic rocks of Late Eocene age are reported in western Challenger Plateau (Nelson et al. Citation1986; Uruski Citation2019), and several drillholes in the western Taranaki Basin (e.g. Amokura-1, Pateke-1 and Kiwi-1) penetrate a unit rick in bentonite clays at the top of the Turi Formation, referred to in petroleum exploration reports as ‘the ash unit’.
The volcanoes erupted onto a low-gradient submarine sea floor dipping N to NE, in paleo-water-depths ranging from 50 to 1500 m (). Most volcanoes are cone-type, <1 km wide and <200 m high. Some edifices appear to be formed by overlapped cones up to 5 km wide and ca 300 high. A single small (<1 km across) crater-type volcano was observed in the central part of the field (line DTB01-31). The volcanoes are often located above the tips of saucer-shaped intrusions or associated with high-amplitude reflections that cross-cut the Cretaceous to Paleocene sedimentary sequence.
The association of vents with large aprons of high-amplitude reflections suggests eruptions of low viscosity lavas, which together with the internal mound and rubble morphology of the vents, may indicate that in some cases they correspond to submarine pillow mounds. The volcanoes that show bedded flanks may have been formed by submarine pyroclastic eruptions (e.g. submarine Strombolian and eruption-fed density currents), which is consistent with the widespread occurrence of the ‘ash unit' in drillholes over 100 km to the SE. The scattered distribution of small-volume volcanoes gives the Kaiwero volcanic field a characteristic observed in monogenetic volcanic fields (Cas et al. Citation1989; Németh Citation2010; Kereszturi and Németh Citation2013). This volcanic field does not show a relationship with plate boundaries or pre-existing crustal structures and it is likely that to have an intraplate origin consistent with the Horomaka Supersuite (Mortimer et al. Citation2014).
Northland-Mohakatino volcanic belts – Early Miocene to Recent
The volcanoes of the Northland-Mohakatino volcanic belts have been identified by geophysical tomography since the 1960s (Hatherton Citation1968; ). Several drillholes (beginning with e.g. Mangaa-1 in 1968, and including Kora-1–4, Albacore-1, and Tangaroa-1) drilled into volcanic rocks of Miocene age, confirming a volcanic origin of the geophysical anomalies. Volcanoes in these belts are considered to be of subduction-related origin (Whakaari Supersuite; Mortimer et al. Citation2018).
These volcanoes form a geographically continuous chain of more than 70 large basaltic-andesitic calc-alkaline edifices (Bergman et al. Citation1992), hundreds of parasitic and satellite vents, as well as numerous intrusive bodies ( and ). The age of the volcanoes range from Early Miocene (ca 25 Ma) in the north, to the active Mount Taranaki in the south, forming a roughly north–south trending belt oblique to the modern trend of the Taupo Rift and to the strike of the subducting Pacific plate. Most volcanoes are deeply buried (>1000 m) offshore of the west coast of the North Island and correlate in age and lithologies with volcanic rocks outcropping in the Northland and Coromandel Peninsulas, and with modern volcanoes of the Taranaki Peninsula and Taupo Volcanic Zone (e.g. Herzer Citation1995; Hayward et al. Citation2001; Seebeck et al. Citation2014).
Figure 7. Simplified map showing the distribution of Miocene-Quaternary subduction-related volcanoes onshore and offshore the North Island of New Zealand. Coloured polygons show the location and age of volcanoes mapped in seismic data, while dots correspond to dredged samples of volcanic origin or the location active volcanoes shown in the Global Volcanism Programme webmap. White dashed lines show the trends of subduction-related volcanoes by age groups. Note a progressive younging in the age of the volcanoes from NW to SE. Aotea volcanic complex has a representative dredge sample comprising a basalt with an Ar/Ar date of 22.5 Ma (Mortimer et al. Citation2018), indicative of intraplate origin. See discussion and Supplementary Material 5 for more details. Mapping of the offshore volcanoes was combined with information from Herzer (Citation1995), Hayward et al. (Citation2001), Mortimer et al. (Citation2010), Giba et al. (Citation2013), Seebeck et al. (Citation2014) and GNS Geological Map (Heron Citation2014). Background image shows a bathymetric map from NIWA (New Zealand Institute of Water and Atmosphere).
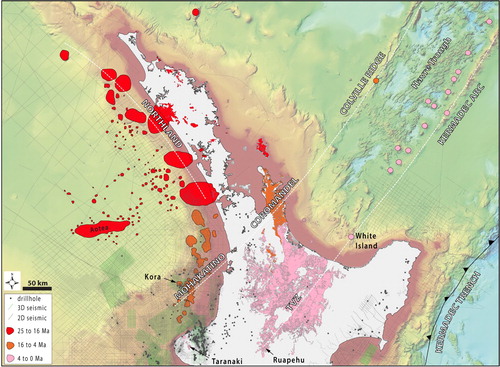
The morphologies of volcanic edifices include large stratocones (e.g. Kora), shields (e.g. Waipoua), and volcanic complexes with several overlapping edifices (e.g. within the Parihaka and Nimitz 3D seismic volumes). Many of these volcanoes were long-lived systems active for >8 Myr (e.g. Kora, Manukau, Te Kumi; Giba et al. Citation2013). Typically, in the north, the volcanoes are wider and show low-angle flanks (e.g. Manukau, 75 km wide and ca 1600 m high), while smaller and steeper volcanoes predominantly occur in the south (e.g. Kora, 15 km wide and ca 1200 m high), which could reflect changes in the geometry of the subducting slab (Seebeck et al. Citation2014). Most volcanoes have erupted in a submarine setting, comprising a complex variety of lithofacies such as tuffs to tuff-breccias, and pillow-lavas commonly interbedded with volcanogenic sandstones and conglomerates (Bergman et al. Citation1992; Bear and Cas Citation2013; Shumaker et al. Citation2018; (c,d)).
Figure 8. Oblique 3D seismic visualisation and volcanogenic rocks of the Kora Volcano, a long-lived Miocene stratocone of the Mohakatino belt. (a and b) Main chronostratigraphic surfaces rock units and structures coupled with geobody mapping of Kora plumbing system. Note that intrusions were preferentially emplaced into pre-existing Cretaceous-Paleocene grabens and along normal faults. (c) Photograph of a core sample from the drillhole Kora-1a at the depth of 1783 m showing a moderately sorted lapilli-tuff. (d) A polymictic volcanic conglomerate from 1908 m in Kora-1a. The location of the Kora Volcano is shown in . Modified from Bischoff et al. (Citation2017) and Bischoff and Nicol (Citation2019).
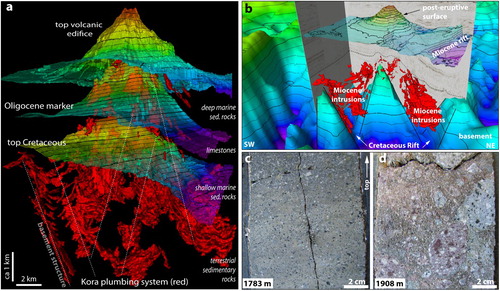
The plumbing systems of the Northland-Mohakatino belts emplaced hundreds of km3 of magma into pre-existing Cretaceous grabens and associated normal fault zones, which forms a large batholithic mass of igneous rocks N-S trending and extending semi-continuously for >500 km (). Observations in the Kora and Nimitz 3D datasets indicate that most of these intrusions occur in association with large polygenetic volcanoes. The intrusions show seismically detectable sheet-like shapes such as dikes and sills, and relatively small (<7 km across) swarms of hybrid bodies with a variety of geometries such as saucer- and stock-like morphologies that cross-cut each other and the sedimentary host rocks (e.g. Infante-Paez and Marfurt Citation2017; Morley Citation2018; Kumar et al. Citation2018). In detail, these bodies more commonly occur as interconnected networks of magmatic intrusions rather than forming large plutonic rock masses. Dikes and sills of polygenetic volcanoes usually form along, or branching from, pre-existing Cretaceous faults, commonly converging towards a relatively stationary central vent, while small parasitic/satellite volcanoes are typically, but not exclusively, located above the tips of peripheral shallow (<2 km) saucer-shaped intrusions ((a,b); Bischoff et al. Citation2017).
West Ngatutura volcanic field – Pliocene
The West Ngatutura volcanic field is a Pliocene cluster of at least 40 scattered small-volume cone-type volcanoes varying in size from <1 km across and <400 m high, distributed over an area of ca 2100 km2. Presently, these volcanoes are buried by 400–800 m of sedimentary strata offshore of the Waikato and Auckland coasts ( and ). We refer to this cluster of volcanoes as the western equivalent of the onshore Ngatutura volcanic field ().
Figure 9. (a) Map showing the location of the Pliocene West Ngatutura volcanic field, regional fault structures and possibly correlative onshore volcanic fields. The coloured bar shows the isochron map between the pre- and post-eruptive surfaces, revealing the location and morphology of the volcanoes. Onshore volcanic rocks are from GNS Geological Map (Heron Citation2014) and faults are from Giba et al. (Citation2013). (b) Interpreted 2D seismic section (line OA07-025) showing the morphology of some volcanoes and intrusions in the West Ngatutura volcanic field. Numbers are: (1) feeder dikes; (2) saucer-shaped intrusion; (3) jacked-up dome; (4) seismic artefact (multiple); (5) seismic artefact (hyperbola); (6) seismic artefact (pull-up velocity); (7) small-volume cone-type volcano; (8) lava-flows; (9) vents.
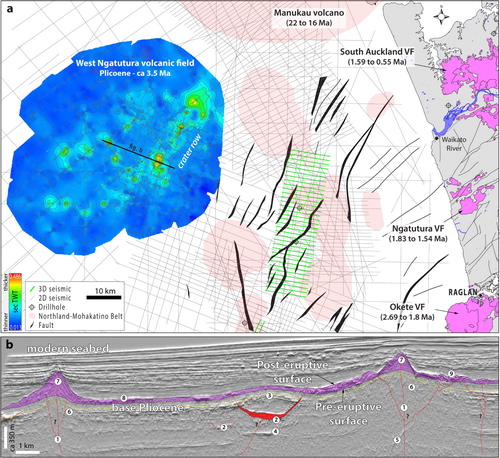
The volcanoes erupted within the lower part of the Pliocene sequence of the Taranaki Basin (ca 3.5 Ma) in paleo-water-depths around 2000 m. There is no evidence of volcanic rocks of this age from the nearest drillholes. However, mound- and cone seismic anomalies are underlain by pull-up of seismic velocities artefacts, indicating that rocks within the anomalies have a much higher acoustic impedance than surrounding sedimentary strata, supporting the interpretation of an igneous origin (Uruski Citation2019; (b)). This interpretation is reinforced by numerous high-amplitude reflections cross-cutting host strata located below the pre-eruptive surface of these volcanoes, as is typical of igneous intrusions. In addition, doming of reflections overlying cone-type volcanoes is often observed, where volcanic rocks have compacted less than surrounding sedimentary strata (e.g. Planke et al. Citation1999; Holford et al. Citation2012; Bischoff et al. Citation2017).
The random distribution of the volcanoes is typical of the intraplate Horomaka Supersuite (Mortimer et al. Citation2014). However, a NE alignment of vents is observed in the east part of the field, which is located above a Cretaceous fault of same direction ((b)), and parallel with the concurrent opening of the Taranaki Northern Graben (King and Thrasher Citation1996), situated 20 km east of the field. Such alignment of vents is common for the Neogene intraplate volcanoes erupted in the North Island (Le Corvec et al. Citation2013).
Other volcanoes buried in the northern Zealandia
Other Late Cretaceous (and possible older) volcanoes identified offshore the North Island are Tikati volcanic complex (Uruski Citation2019; Supplementary Material 3), and intrusions and small vents in the area of the Matuku-3D dataset (Supplementary Material 4). The north-western limit of the Deepwater Taranaki Basin contains a large (ca 100 km) W–E elongated seamount oblique to the NW orientation of the New Caledonia Trough (Aotea Seamount, Brodie Citation1965). Its morphology in seismic imagery shows a main volcanic body with multiple edifices and parasitic and satellite vents, consistent with it being a volcanic complex (Supplementary Material 5). The volcanoes of Aotea erupted immediately above the base of the Miocene chronostratigraphic surface indicating an age of ca 23 Ma, which is supported by Ar–Ar dating of ca 22.5 Ma, while a dredge sample returned an ultra-alkaline chemical signature, suggesting an intraplate origin (Mortimer et al. Citation2018).
Southern Zealandia
Late Cretaceous volcanoes
Numerous Late Cretaceous volcanic centres are mapped in areas of coeval continental rifting in the Canterbury and Great South basins (Field et al. Citation1989; Bischoff et al. Citation2016; Barrier Citation2019; ). Many of these volcanoes occur immediately above the basement of the host basin, which, due to the similarity in the seismic signal of volcanic and basement rocks, makes their characterisation difficult (Barrier Citation2019). Similar seismic facies are identified SE of Banks Peninsula (e.g. line IP256-98-001) where volcanic rocks of the Mount Somers Volcanic Group were intersected by the Leeston-1 and JD George-1 drillholes proving a Late Cretaceous volcanic origin for these seismic facies in at least some cases. Large Late Cretaceous volcanic complexes within the Canterbury and Great South basins are typically imaged with good resolution in seismic data. Examples of these volcanic complexes are Sloop (Supplementary Material 6), Barque (), and Tapuku East (Supplementary Material 7).
Figure 10. (a) Spectral decomposition of the Endurance-3D seismic cube with a depth-slice window at 3000 m. (b) 2D seismic section across the Late Cretaceous volcanoes of the Barque volcanic complex. This volcanic complex comprises two large edifices (ca 17 km across and ca 1000 m high each), as well as several parasitic and satellite vents and numerous intrusive bodies (Beggs Citation2016). Note that most eruptive centers are located at, or aligned with, NE-SW trending normal faults, which are parallel to the trend of extension of the Zealandia rift in the area (Barrier Citation2019). Figures 1 and 10 show the location of the volcanic complex.
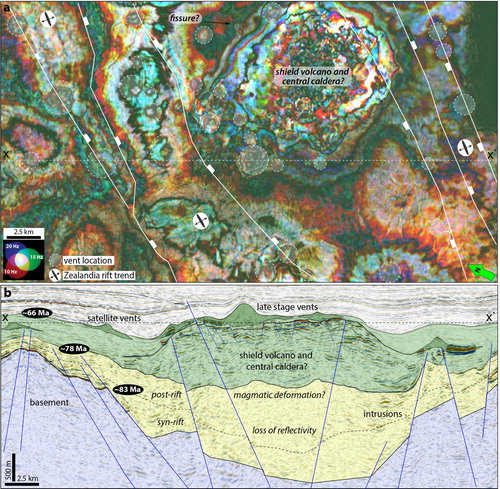
Table 3. Main characteristics of the volcanoes buried in the Taranaki, Deepwater Taranaki and Reinga-Northland basins.
Table 4. Main characteristics of the volcanoes buried in the buried in the Canterbury and Great South basins.
East Waiareka-Deborah volcanic field – Paleocene to Oligocene
A cluster of >50 randomly distributed small-volume edifices is buried by up to 3 km within Paleocene-Oligocene strata of the Canterbury Basin, covering an area of ca 3,500 km2 (). These edifices have been interpreted as an extension of the volcanoes of the Waiareka-Deborah field, onshore in the region of Oamaru (e.g. Field et al. Citation1989; Barrier et al. Citation2017; Fensom Citation2020). There is presently no evidence of Paleocene volcanism in inland eastern Otago, thus, we refer to the offshore volcanoes as the East Waiareka-Deborah volcanic field to differentiate them from the onshore counterpart ().
Figure 11. (a) Map showing the location and age of volcanoes onshore and offshore eastern South Island (Horomaka Supersuite). Onshore volcanic rocks are from GNS Geological Map (Heron Citation2014). (b,c) 2D sections showing the age and morphology of small-volume cone-type volcanoes buried in the Canterbury Basin. Numbers are: (1) intrusions; (2) conduits. (d) An outcrop within the Kakanui Head, a volcano of the Waiareka-Deborah volcanic field showing an angular contact between amalgamated and poorly-sorted pyroclastic beds inward-dipping towards the central crater of the edifice, while well-sorted and tabular beds dip outward.
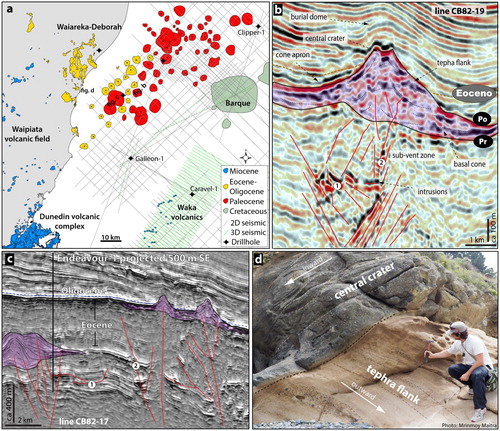
Some volcanoes in this field were intersected by the Endeavour-1, Cutter-1, Galleon-1, Clipper-1, Oamaru-1 and Oamaru-2 drillholes (Field et al. Citation1989), confirming an igneous origin for the seismic anomalies. Volcanic rocks recovered from these drillholes comprise basaltic tuffs up to 124 m thick interbedded with marine mudstones, sandstones and limestones, indicating that eruptions occurred in a shallow (<500 m) marine environment (Schiøler et al. Citation2011), which is in accordance with interpretations of onshore volcanics (Coombs et al. Citation1986; Corcoran and Moore Citation2008). Samples from the Endeavour-1 drillhole contain glassy basaltic tuffs eventually altered to palagonite (Wilding and Sweetman Citation1971), a characteristic of the rocks exposed onshore (Cas et al. Citation1989; Moorhouse et al. Citation2015; Fensom Citation2020).
The volcanoes are typically <1 km wide and <400 m high. The flanks of well-preserved cones usually show bedded seismic facies that dip outward from a central zone of chaotic and/or inward-dipping reflections of moderate amplitude ((b,c)), suggesting accumulation of pyroclastic material (Bischoff, Nicol, Barrier, et al. Citation2019). This interpretation is reinforced by the tuffaceous facies described in the drillholes (Field et al. Citation1989), and by the comparable morphology of volcanoes in seismic imagery and onshore exposures ((d); Fensom Citation2020). Some minor high-amplitude reflections located next to the vents may indicate the presence of pillow-lava deposits.
Disrupted seismic facies commonly occur below the vents and likely indicate the presence of magma conduits ((b,c)). Large (>3 km) intrusive bodies are rarely observed below this volcanic field. This contrasts with other monogenetic volcanic fields presented in this study, suggesting that the East Waiareka-Deborah volcanoes are mainly fed directly from a magma source at least deeper than the seismic resolution of our dataset (i.e. >5 km), which is in agreement with petrological models for the volcanoes onshore (Coombs et al. Citation1986). Based on the random distribution of the volcanoes, distance from plate boundaries and correlation with the onshore counterparts, the East Waiareka-Deborah volcanic field likely has an intraplate origin (Horomaka Supersuite).
Papatowai volcanic field – Oligocene or Early Miocene
The Papatowai volcanic field comprises at least 30 scattered small-volume cone-type volcanoes located offshore of the Clutha district, South Island, New Zealand (). We refer to this cluster of volcanoes using the name of the town and river adjacent to the area where they are located (). There is no physical evidence of volcanic rocks in this area, however, the presence of pull-up velocity artefacts underlying the volcanoes, as well as high-amplitude reflections with saucer-shaped geometry cross-cutting host pre-eruptive strata indicate a volcanic origin (). In addition, the interpretation of aeromagnetic data suggests the presence of Cenozoic volcanic rocks in the region (Waight et al. Citation2019).
Figure 12. (a) TWT map on the proxy post-eruptive surface of small-volume cone-type volcanoes of the Papatowai volcanic field. (b and c) 2D sections (lines OMV08-042 and Husky-H-104) showing the morphology of some of the volcanoes and part of their plumbing systems. Numbers are: (1) feeder dikes, (2) intrusion; (3) jacked-up dome; (4) seismic artefact (pull-up velocity); (5) pillow mounds? (6) small-volume cone-type volcano; (7) pre-eruptive surface; (8) post-eruptive surface.
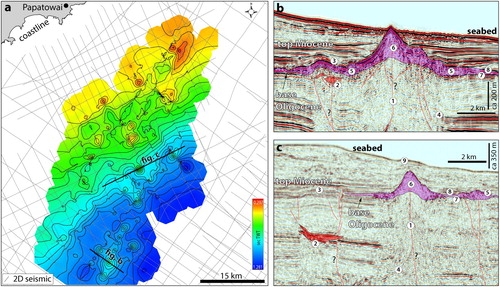
Volcanism in this field occurred over an area of ca 1600 km2. The volcanoes erupted in paleo-water-depths <500 m. It is not possible to precisely determine the age of these volcanoes based exclusively on seismic lines because they are buried within a thin condensed section of sedimentary rocks of Oligocene to Early Miocene age, with epoch boundaries below the seismic resolution of the dataset at that location.
As is the case in the East Waiareka-Deborah and Kaiwero volcanic fields, the flanks of the Papatowai cones usually show bedded seismic facies outward dipping from a central zone of chaotic and/or inward-dipping reflections of mod-amplitude ((b,c)), suggesting pyroclastic eruptions. In addition, most cones have peripheral high-amplitude reflections, which could correspond to pillow-lavas or other forms of dense submarine lava-flow deposits. The Papatowai volcanoes likely have an intraplate origin and belong to the Horomaka Supersuite.
Other volcanoes buried in the southern Zealandia
Offshore southeast of Banks Peninsula, the Maahunui volcanic field comprises a cluster of Middle Miocene volcanoes and shallow (<1 km) intrusions currently buried by ca 1000 m of sedimentary strata in the offshore Canterbury Basin (Supplementary Material 8; Bischoff, Nicol, Cole, et al. Citation2019, Bischoff, Nicol, Barrier, et al. Citation2019, Bischoff, Rossetti, et al. Citation2019). The Waka-3D seismic survey located offshore Dunedin contains several large (ca 5 km across) saucer-shaped intrusions, mainly aligned with pre-existing Cretaceous faults (Blanke Citation2012; ). Above these intrusions, a number of volcanic vents are located onto the Miocene paleo-sea floor ( and ), having been erupted at a water depth of >500 m. The lateral extent of this volcanic field is unclear due to the limited resolution of the seismic data outside the area of the 3D survey, but they correlate in age and morphology with monogenetic vents erupted in the vicinity of the Dunedin volcano onshore (; Scott et al. Citation2020). Another field of scattered small-volume volcanoes and shallow intrusions probably of Pleistocene age occurs ca 100 km SE of Stewart Island, near the location of the Toroa-1 drillhole (Supplementary Material 9; and ). Maahunui, Waka and Toroa volcanic fields are all likely intraplate in origin (Horomaka Supersuite).
Figure 13. Examples of intrusions and vents of the Miocene Waka volcanics. (a) Amplitude opacity rendered seismic volume (Waka-3D) revealing a number of intrusions emplaced into Paleocene sedimentary strata and vents erupted onto the Miocene paleo-sea-bed. Note the spatial relationship between the intrusive bodies and vents. (b) Geobody extraction of a saucer-shaped intrusion showing its geometry, relationship with enclosing strata, conduits, and co-genetic eruptive vents. Modified from Bischoff and Nicol (Citation2019).
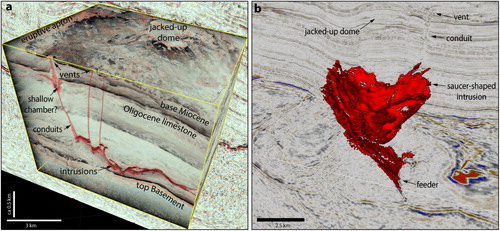
Discussion
Insights from volcanoes buried in sedimentary basins can help to fill important gaps in understanding patterns of volcanism across Zealandia. However, vast areas are still poorly imaged (e.g. West Coast, Aotea, Bellona, Emerald, Northeast Slope basins, and towards east of the Canterbury and Great South basins), or only covered by sparse 2D seismic grids that cannot give insights in the same resolution as 3D datasets (e.g. Reinga-Northland Basin).
Regions of high erosion or those affected by tectonic processes associated with the Hikurangi and Puysegur subduction zones, and with uplift of the Southern Alps (e.g. Ballance Citation1993; Lebrun et al. Citation2003; Stagpoole and Nicol et al. 2007) generally have poor scope for burial and preservation of volcanoes. The following sections discuss the first-order regional features in areas where preservation was enhanced by low deformation, little or no erosion, and the presence of seismic reflection data is sufficient to image volcanoes and volcanic fields ( and ).
Figure 14. Simplified geological evolution of the New Zealand region showing four main tectonic-magmatic phases. (a) The first phase (ca 100–85 Ma) is characterised by widespread intracontinental break-up (Zealandia rifts) and simultaneous volcanism broadly associated with lithosphere thinning and rifting. (b) The second phase (ca 85–66 Ma) corresponds to the separation of Zealandia from eastern Australia and Antarctica, and volcanism in western New Zealand coeval with the West Coast-Taranaki rift, and volcanic activity immediately after cessation of rifting in southern Zealandia. (c) During the third phase (ca 66–30 Ma), diffuse intraplate volcanism mainly formed fields of small-volume volcanoes. (d) The fourth phase (ca 30–0 Ma) is characterised by both subduction-related volcanism and semi-continuous diffuse intraplate magmatic activity. Maps are compiled and updated from Herzer (Citation1995), Hayward et al. (Citation2001), Mortimer et al. (Citation2010), Giba et al. (Citation2013), Seebeck et al. (Citation2014), Bache, Mortimer, et al. (Citation2014), Mortimer et al. (Citation2018), Bischoff, Nicol, Barrier, et al. (Citation2019), Waight et al. (Citation2019), Uruski (Citation2019), Barrier (Citation2019), Global Volcanism Programme, and GNS Geological Map (Heron Citation2014).
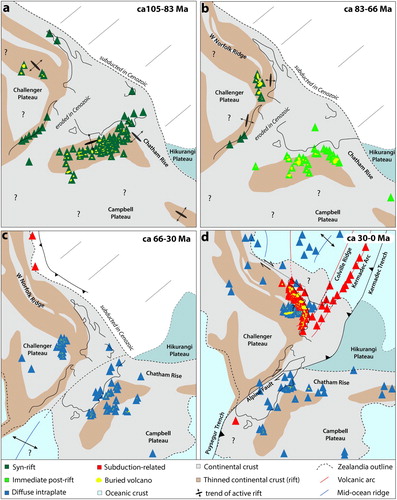
Late Cretaceous syn- and immediate post-rift volcanism
Late Cretaceous volcanic activity typically formed large (20–1400 km3) composite cones and volcanic complexes that were active for ca 1–15 Myr, and less commonly fields of scattered small-volume (<1 km3) volcanoes erupted into subaerial to shallow marine environments (). These volcanoes primarily erupted under the influence of a tensional crustal stress field (i.e. σ1 vertical, σ2 and σ3 horizontal) associated with the break-up and separation of Zealandia from eastern Gondwana ( and (a,b)). For example, in the Taranaki and Deepwater Taranaki basins, two eruptive phases of volcanism occurred in Late Cretaceous and were at least in part concurrent with two rifting events (i.e. Zealandia and West Coast-Taranaki rifts; Strogen et al. Citation2017). During the first eruptive phase, ca 85–84 Ma and possibly older, Chronos, Rhea and at least two additional unnamed volcanoes (Uruski Citation2019) erupted along the NW-SE rift system of the New Caledonia Trough ( and (a)). The precise timing of cessation of this eruptive phase is difficult to determine based on the available data, but it was contemporaneous with, or followed by, deposition of the lowermost Taranaki Delta around 83 Ma (Schiøler et al. Citation2014). In northern Zealandia, the first rift phase shut down at ca 83 Ma, perhaps due to the onset of seafloor spreading in the Tasman Sea and the associated cessation of normal faulting in the interior of Zealandia (Gaina et al. Citation1998; Strogen et al. Citation2017; Barrier, Nicol, et al. Citation2020). Relaxation or reduction of the tensional stresses across Zealandia with the advent of sea floor spreading may have caused the cessation of this first eruptive phase.
The second eruptive phase (ca 74–68 Ma) formed a broad N-S alignment of volcanoes along the extension of the West Coast-Taranaki rift ( and (b)). These include Coopworth, Romney, Hades, Vulcan, Hestia ( and ), Tikati (Supplementary Material 3), and volcanoes within the Matuku-3D dataset (Supplementary Material 4). We observe that many, if not most, volcanoes of the second eruptive phase are typically located along concurrent N-S trending normal faults (). This is also documented in Zhu et al. (Citation2017) and Uruski (Citation2019) and suggests that the second eruptive phase is linked with extension concurrent with West Coast-Taranaki rifting.
Uruski (Citation2019) argues that crustal extension does not control the location of volcanoes in Deepwater Taranaki Basin, since many volcanoes erupted above local basement highs with magma migrating through thicker crust. However, the locus of eruptions along continental rifts, such as those of Late Cretaceous age in Zealandia, is thought to be controlled by the interplay between the rate of extension of the crust and the presence of pre-existing structures (Corti Citation2009; Vries and Vries Citation2018). Rifts associated with high rates of extension tend to form volcanoes at the centres of the rift axis, where intense crustal thinning occurs (e.g. Afar Rift; Illsley-Kemp et al. Citation2017), while rifts with low extension rates typically have volcanoes located along the rift margins and on local basement highs (e.g. Limagne Rift; Vries and Vries Citation2018). Volcanoes erupted at both rift axis and margins are buried in offshore New Zealand. We infer that the locations of these volcanoes probably reflect the interplay of tectonic stress during volcanic activity and the presence of pre-existing crustal structures ((a)).
A relationship between extension and the location of eruptive centres is also observed in southern Zealandia. In the Canterbury Basin, 70% of the syn-rift volcanoes are within 5 km of a rift fault (Barrier Citation2019). This author also suggest that elongated features formed along rift faults may correspond to volcanoes erupted in fissures, typical of extension-related volcanism elsewhere (e.g. Corti Citation2009; Sigmundsson et al. Citation2018; Neal et al. Citation2019). Large (>500 km3) polygenetic volcanoes erupted post Zealandia break-up (e.g. Barque, Sloop and Tapuku) are also aligned with both NE-SW and NW-SE trending rift faults ((b); Barrier Citation2019; ). Similarly, volcanoes of Late Cretaceous age in the Great South Basin follow the NE-SW trend of rifting ((a,b)). In addition, areas of high-positive magnetic anomalies across Zealandia are interpreted to be caused by Late Cretaceous mafic rift-related igneous rocks (Waight et al. Citation2019; Mortimer and Scott Citation2020).
Collectively, evidence compiled from previous work and new data presented in this paper indicates that during the separation of Zealandia from south-eastern Gondwana, Late Cretaceous magmatism and continental rifting were closely linked in time and space ((a,b)). In a tectonic sense, we consider that these rift-related volcanoes should be distinguished from the intraplate Horomaka Supersuite (Mortimer et al. Citation2014). We propose here a new stratigraphic unit, Te Kiekie Supersuite, to describe Late Cretaceous volcanoes that form within coeval intracontinental rifting of Zealandia. Te Kiekie is the Māori name for the Mount Somers, inland Canterbury Basin, which represents volcanic activity contemporaneous with Zealandia rifting (e.g. Laird and Bradshaw Citation2004; Mortimer et al. Citation2014; Van der Meer et al. Citation2018). This classification could open up scope for future research for identifying petrological changes and differences between Late Cretaceous and Cenozoic intraplate volcanoes of the Horomaka Supersuite.
Cenozoic diffuse intraplate volcanism
Offshore buried volcanoes erupted throughout the Cenozoic and remote from the Australian-Pacific plate boundary (>200 km) predominately form clusters of scattered small-volume (<1 km3) vents (), as characteristic of monogenetic volcanic fields (Németh and Kereszturi Citation2015). The random distribution and absence of obvious structural control on the location of these buried volcanoes suggest that they have an intraplate origin, which is supported by geochemistry studies on their onshore counterpart volcanic fields, such as the Auckland and Waiareka-Deborah (Scott et al. Citation2020; Hopkins et al. Citation2020).
In detail, alignment of vents and intrusions does occur, but at a much more local scale than volcanoes of Late Cretaceous age ((a) and ). These alignments are observed in three Neogene volcanic fields (West Ngatutura, Waka and Maahunui) that erupted a large distance (<300 km) from the primary plate boundary structure (, and (c,d)). In all three cases, the vents and intrusions are aligned parallel to the trend of pre-existing faults, which is commonly also observed for Neogene monogenetic volcanic fields in the North Island (Le Corvec et al. Citation2013). In contrast, during a period of tectonic quiescence across most New Zealand in the Paleogene, neither onshore nor offshore monogenetic fields show any clear relationship with pre-existing crustal structures.
After ca 40 Myr of predominantly monogenetic small-volume intraplate volcanic activity in Zealandia, the large polygenetic volcanoes of Banks Peninsula, Otago Peninsula, and Aotea Seamount erupted concurrently with Neogene tectonic activity in the Hikurangi and Puysegur subduction zones and the transpressional Alpine Fault. Banks Peninsula erupted at the intersection of WNW-ESE and NE-SW trending basement structures of Cretaceous age (i.e. Chatham Rise and Endeavour High; Field et al. Citation1989; Barrier et al. Citation2020). Eruptive centres formed along normal faults of similar orientation, presumably due to reactivation concurrently with volcanic activity (Hampton and Cole Citation2009). Otago Peninsula erupted near the limit of the Rakaia and Caples terranes, and is also aligned with the Titri fault and Taieri graben (Coombs et al. Citation1960; Deckert et al. Citation2002). Aotea volcanic complex erupted onto the West Norfolk Ridge, with normal faults along the boundary with the New Caledonia Trough (Supplementary Material 5).
The influence of tectonics on fault displacement is reported to extend far from plate boundaries. For example, in the intra-cratonic Paraná Basin, southern Brazil, extension and reactivation of normal faults along the boundaries of crustal blocks are linked with the timing of terrain accretion on the western Gondwana plate boundary, which was located at least 1500 km from the basin (Milani and Ramos Citation1998; Holz et al. Citation2006). There is a general agreement that magma erupted remote from plate boundaries have to rise through thicker (>10 km) crust, facilitated mostly by the existence of an extensional stress field in the lithosphere, and by the interaction of magma with pre-existing crustal structures (e.g. Shaw Citation1980; Le Corvec et al. Citation2013; Vries and Vries Citation2018).
The spatial associations between major structures and Neogene volcanoes across Zealandia may suggest that the stress field imposed at plate boundaries could be at least in part responsible for fault reactivation, extension and eruptions in an intraplate setting some distance from the primary plate boundary structures.
Cenozoic subduction-related volcanism
Zealandia arc-related volcanoes mainly comprise large deep-submarine to subaerial composite, shield and caldera volcanoes (Ballance et al. Citation1985; Cole Citation1986; King and Thrasher Citation1996; ). The relative locations of the arcs are complex and have been interpreted to reflect progressive rollback and/or steepening of the slab and southward migration of crustal extension of the upper plate during the Miocene to Recent (Kamp Citation1984; Mortimer et al. Citation2010; Giba et al. Citation2010; (d)). Volcanoes associated with subduction along the Hikurangi margin define a broad NE–SW trending zone that was oriented approximately parallel to the strike of the subducting Pacific plate since at least from the last 16 Ma (Seebeck et al. Citation2014). Until about 12 Ma, significant volcanism did not extend south of about 38o S latitude, migrating ca 60 km further south during 12–8 Ma, with voluminous eruptions then in the area of the Parihaka-3D survey up to about 4 Ma (Hayward et al. Citation2001; Giba et al. Citation2013; ). After 4 Ma, volcanism migrated further SE to its present location at the Taupo-Kermadec-Tonga trend (Cole and Lewis Citation1981; ).
The active volcanoes of the Central North Island are now situated about 80–100 km above the Pacific plate subducting slab, with magma interpreted to be produced by slab dehydration and partial melting of the asthenosphere (Seebeck et al. Citation2014; Illsley-Kemp et al. Citation2019; ). Southwest of the Central North Island and south of the Mt Taranaki, seismicity indicates that the subducting slab reaches depths of >100 km, however, there is no evidence of volcanic activity in seismic reflection surveys and drillholes on this area (). A similar southward termination also occurred for the Miocene Mohakatino volcanoes, although this termination was northwest of the contemporary termination of the Central North Island volcanoes ((d)).
During both time intervals volcanism was absent from regions primarily accommodating crustal contraction, where near-horizontal σ1 stress may generally increase fault-normal stresses and reduce fracture apertures, both of which would act to reduce the utility of crustal weakness in the ascent of magma. In areas where crustal extension rate is sufficiently low that it is not manifest as normal faulting, the rise of magma through the crust may be facilitated by pre-existing Cretaceous faults (Giba et al. Citation2013). Conversely, volcanism is also absent in some areas where normal faulting and crustal extension has occurred. At the present time, the southwards transition from crustal tension to crustal compression in the southern Taranaki Basin occurs midway between North and South Islands (e.g. Nicol et al. Citation2007; Rajabi et al. Citation2016; Massiot et al. Citation2019), and yet there is no volcanism south of Mt Taranaki. The absence of volcanism in this case may reflect the presence of thickened continental crust due to late Cenozoic thrusting and shortening, and this thickening may inhibit the upward flow of magma to the ground surface. Collectively, this evidences suggest that the interplay of stress fields, pre-existing structures and crustal thickness may play important role on the location of the Cenozoic subduction-related volcanoes of the Norther Zealandia.
Conclusions
Interpretation of a large seismic reflection dataset and petroleum exploration drillhole from offshore New Zealand revealed over 400 Late Cretaceous-Cenozoic volcanoes buried within Te Riu-a-Māui/Zealandia sedimentary basins. In this paper, we propose a systematic classification of the best understood examples of these volcanoes into three large-scale categories (i.e. rift, intraplate, and subduction-related). These categories are sub-divided into informal volcanic zones, fields, complexes, and individual volcanic edifices, according to the location, distribution, age, size and morphology of the volcanoes. Results indicate that the location of eruptive centres was primarily controlled by the stress regime operating at each tectonic setting, with the local influence of pre-existing crustal structures and types of host rocks. Late Cretaceous volcanoes mainly comprise large (>20 km3) long-lived (>1 Myr) composite cones and volcanic complexes that erupted concurrently in time, space and orientation of continental rifts formed during break-up of Zealandia from the south-eastern Gondwana. Cenozoic intraplate volcanism was randomly distributed, and characterised by clusters of scattered small-volume (<1 km3) cones and craters associated with numerous shallow (<2 km) magmatic bodies, typically of saucer-shaped geometry. In detail, only Cenozoic intraplate volcanoes erupted contemporaneously with tectonic activity show a spatial relationship with pre-existing structures, suggesting that fault reactivation and volcanism in an intraplate setting could be influenced by the stress field imposed near (<400 km) plate boundaries. In contrast, subduction-related volcanism has formed a geographically continuous chain of over 70 large strato- and shield volcanoes from Early Miocene in the north to the active Mt Taranaki in the south. These volcanoes are the south-western extremity of multiple volcanic arcs associated with the Kermadec subduction zone, in which southward migration in the locus of eruptions is interpreted to reflect progressive rollback and/or steepening of the subducting Pacific Place beneath the Australian Plate. In detail, the location of arc-related eruptive centers was influenced by the interplay of stress field, thickness of the crust and presence of pre-existing tectonic structures. This paper showcases the utility of seismic reflection for determining the relationship between volcanic and tectonic activity. Studying the volcanoes buried in sedimentary basins can help advance understanding of syn- and post-Gondwana break-up magmatism, with global impacts to comprehend the interplay between volcanism and plate tectonics in Zealandia and further afield.
List of Supplementary Material
Download MS Word (16.4 KB)Suplemmentary Material 09
Download TIFF Image (26.5 MB)Suplemmentary Material 08
Download TIFF Image (29.2 MB)Suplemmentary Material 07
Download TIFF Image (29.6 MB)Suplemmentary Material 06
Download TIFF Image (29.2 MB)Suplemmentary Material 05
Download TIFF Image (22.1 MB)Suplemmentary Material 04
Download TIFF Image (27.7 MB)Suplemmentary Material 03
Download TIFF Image (29.2 MB)Suplemmentary Material 02
Download TIFF Image (32.7 MB)Suplemmentary Material 01
Download TIFF Image (18.2 MB)Acknowledgments
We would like to thank IHS Markit and Schlumberger for providing academic licence to use Kingdom and Petrel software. Thanks for the constructive reviews of Nick Mortimer and an anonymous reviewer, and to Marcos Rossetti for petrographic discussions. We are very grateful for all the support provided by the School of Earth and Environment of the University of Canterbury, particularly to Rob Spiers and John Southward.
Disclosure statement
No potential conflict of interest was reported by the author(s).
Data availability statement
The data that support the findings of this study were provided by the New Zealand Petroleum and Minerals (NZPAM) and are openly available in the 2018 Petroleum Exploration Data Pack and at https://data.nzpam.govt.nz.
Additional information
Funding
References
- Arnot MJ, Bland KJ, et al. (Compilers) 2016. Atlas of petroleum prospectivity, Northwest Province: ArcGIS geodatabase and technical report. GNS Science Data Series 23b 34 p. + 1 ArcGIS geodatabase + 5 ArcGIS projects.
- Bache F, Mortimer N, Sutherland R, Collot J, Rouillard P, Stagpoole V, Nicol A. 2014. Seismic stratigraphic record of transition from Mesozoic subduction to continental breakup in the Zealandia sector of eastern Gondwana. Gondwana Research. 26:1060–1078. doi: 10.1016/j.gr.2013.08.012
- Bache F, Sutherland R, Mortimer N, Browne G, Lawrence MJF, Black J, Flowers M, Rouillard P, Pallentin A, Woelz S, et al. 2014. Tangaroa TAN1312 voyage report: dredging Reinga and Aotea basins to constrain seismic stratigraphy and petroleum systems (DRASP), NW New Zealand. GNS Science Report 2014/05. New Zealand Petroleum Report PR4916. Lower Hutt: GNS Science.
- Ballance PF. 1993. The New Zealand Neogene forearc basins. In: Ballance PF, editor. South Pacific sedimentary basins, sedimentary basins of the World 2. Amsterdam: Elsevier Science; p. 177–193.
- Ballance PF, Hayward BW, Brook FJ. 1985. Subduction regression of volcanism in New Zealand. Nature. 313:820. doi: 10.1038/313820a0
- Barrier A. 2019. Tectonics, sedimentation and magmatism of the Canterbury Basin, New Zealand [Unpublished PhD thesis]. Christchurch: University of Canterbury. p. 232.
- Barrier A, Nicol A, Bischoff AP. 2017. Volcanism occurrences in the Canterbury Basin, New Zealand and implication for petroleum exploration. AAPG GTW Influence of Volcanism and Associated Magmatic Processes on Petroleum Systems Conference; Oamaru, New Zealand.
- Barrier A, Nicol A, Browne GH, Bassett K. 2020. Late Cretaceous coeval multi-directional extension in southeastern Zealandia: Implications for eastern Gondwana breakup. Marine and Petroleum Geology. 118. doi: 10.1016/j.marpetgeo.2020.104383
- Bear AN, Cas RAF. 2013. The complex facies architecture and emplacement sequence of a Miocene submarine mega-pillow lava flow system, Muriwai, North Island, New Zealand. Journal of Volcanology and Geothermal Research. 160(1–2):1–22. doi: 10.1016/j.jvolgeores.2006.09.002
- Beckman DW. 2012. Final interpretation report for Acb11, Offshore Canterbury, New Zealand. Ministry of Economic Development Petroleum Report Series, PR4492, 50 p.
- Beggs M. 2016. Barque prospect, offshore Canterbury basin: Impact of 3d seismic on evaluation of a giant structural prospect. Petroleum New Zealand Conference 2016, Auckland.
- Bergman SC, Talbot JP, Thompson and PR. 1992. The Kora Miocene Submarine Andesite Stratovolcano Hydrocarbon Reservoir, Northern Taranaki Basin, New Zealand. 1991 New Zealand Oil Exploration Conference, p. 178–206.
- Bischoff AP, Nicol A. 2019. Seismic reflection imaging of volcano plumbing systems in sedimentary basins. VI LASI Workshop, Malargüe, Argentina.
- Bischoff A, Nicol A, Cole J, Gravely D. 2019. Stratigraphy of Architectural Elements of a buried monogenetic volcanic system. Open Geosciences. 11(1):581–616. doi:10.1515/geo-2019-0048.
- Bischoff AP. 2019. Architectural elements of buried volcanic systems and their impact on geoenergy resources [Ph.D. Thesis]. New Zealand: Canterbury University. https://ir.canterbury.ac.nz/handle/10092/16730.
- Bischoff AP, Nicol A, Barrier A, Beggs M. 2016. The stratigraphic record of volcanism – examples from New Zealand sedimentary basins. 2016 Geoscience Society of New Zealand Conference, Wanaka, Abstract.
- Bischoff AP, Nicol A, Barrier A, Wang H. 2019. Paleogeography and volcanic morphology Reconstruction of a buried monogenetic volcanic field (part 2). Bull Volcanol. 81:57. Doi:10.1007/s00445-019-1317-6.
- Bischoff AP, Nicol A, Beggs M. 2017. Stratigraphy of architectural elements in a buried volcanic system and implications for hydrocarbon exploration. Interpretation. doi: 10.1190/INT-2016-0201.1
- Bischoff AP, Rossetti M, Nicol A, Kennedy B. 2019. Seismic reflection and petrographic interpretation of a buried monogenetic volcanic field (part 1). Bull Volcanol. 81:56. doi:10.1007/s00445-019-1316-7.
- Blanke SJ. 2012. Saucer sills of the offshore Canterbury Basin: GNS Publication. doi:10.1177/0094306114545742f.
- Brodie JW. 1965. Aotea Seamount, eastern Tasman Sea, New Zealand. Journal of Geology and Geophysics. 8(3):510–517. doi: 10.1080/00288306.1965.10426421.
- Burchardt S, Walter TR, Tuffen H. 2018. Growth of a volcanic edifice through plumbing system processes - volcanic rift zones, magmatic sheet-intrusion swarms and long-lived conduits. In: Steffi Burchardt, editor. Volcanic and igneous plumbing systems. Amsterdam: Elsevier; p. 89–112. doi: 10.1016/B978-0-12-809749-6.00004-2
- Cant JL, Siratovich PA, Cole JW, Villeneuve MC, Kennedy BM. 2018. Matrix permeability of reservoir rocks, Ngatamariki geothermal field, Taupo Volcanic Zone, New ZealandGeotherm Energy, 6:2. doi:10.1186/s40517-017-0088-6.
- Cas RAF, Landis CA, Fordyce RE. 1989. A monogenetic, Surtla-type, Surtseyan volcano from the Eocene-Oligocene Waiareka-Deborah volcanics, Otago. New Zealand: A Model: Bulletin of Volcanology 51(4):281–298. doi: 10.1007/BF01073517
- Catuneanu O. 2006. Principles of sequence Stratigraphy. Changes. 375. doi:10.5860/Choice.44-4462.
- Chopra S, Marfurt KJ. 2005. Seismic Attributes - A Historical Perspective. Geophysics. doi:10.1190/1.2098670.
- Cole J, Lewis KB. 1981. Evolution of the Taupo-Hikurangi subduction system. Tectonophysics. 72:1–21. doi: 10.1016/0040-1951(81)90084-6
- Cole JW. 1986. Distribution and tectonic setting of Late Cenozoic volcanism in New Zealand. Royal Society of New Zealand Bulletin. 23:7–20.
- Cole JW, Milner DM, Spinks KD. 2005. Calderas and caldera structures: a review. Earth Science Reviews. 69:1–26. doi: 10.1016/j.earscirev.2004.06.004
- Coombs DS, Cas RA, Kawachi Y, Landis CA, McDonough WF, Reay A. 1986. Cenozoic volcanism in north, east and central Otago. Smith IEM (ed) Late Cenozoic Volcanism in New Zealand. R Soc New Zeal Bull 23, 278–312.
- Coombs DS, White AJR, Hamilton D, Couper RA. 1960. Age relations of the Dunedin volcanic complex and some paleogeographic implications—part II. New Zealand Journal of Geology and Geophysics. doi: 10.1080/00288306.1960.10420145
- Corcoran PL, Moore L. 2008. Subaqueous eruption and shallow-water reworking of a small-volume Surtseyan edifice at Kakanui, New Zealand. Canadian Journal of Earth Sciences. 45(12):1469–1485. doi: 10.1139/E08-068
- Corti G. 2009. Continental rift evolution: from rift initiation to incipient break-up in the main Ethiopian rift, East Africa. Earth Science Reviews. 96(1–2):1–53. doi:10.1016/j.earscirev.2009.06.005.
- de Silva S, Lindsay JM. 2015. Primary volcanic landforms. In: H. Sigurdsson, editor. The Encyclopedia of volcanoes (second Edition). Amsterdam: Academic Press; p. 273–297. doi: 10.1016/B978-0-12-385938-9.00015-8
- Deckert H, Ring U, Mortimer N. 2002. Tectonic Significance of Cretaceous Bivergent extensional Shear zones in the Torlesse Accretionary Wedge, central Otago Schist, New Zealand. New Zealand Journal of Geology and Geophysics. 45:537–547. doi: 10.1080/00288306.2002.9514990
- Fensom J. 2020. Reservoir evaluation of volcanic and associated carbonate rocks: case-study in a basaltic monogenetic volcanic field, Oamaru, New Zealand (Unpublished master’s thesis). Christchurch: University of Canterbury.
- Field BD, Browne GH, Davy BW, Herzer RH, Hoskins RH, Raine JI, Wilson GJ, Sewell RJ, Smale D, Watters WA. 1989. Cretaceous and Cenozoic sedimentary basins and geological evolution of the Canterbury region, south Island, New Zealand. lower Hutt: New Zealand Geological survey. New Zealand Geological Survey Basin Studies. 2:94.
- Gaina C, Müller DR, Royer JY, Stock J, Hardebeck J, Symonds P. 1998. The tectonic history of the Tasman Sea: A puzzle with 13 pieces. Journal of Geophysical Research. 103:12413–12433. doi:10.1029/98jb00386.
- Galland O, Burchardt S, Hallot E, Mourgues R, Bulois C. 2014. Dynamics of dikes versus cone sheets in volcanic systems. Journal of Geophysical Research Solid Earth. 119:6178–6192. doi:10.1002/2014JB011059.
- Giba M, Nicol A, Walsh JJ. 2010. Evolution of faulting and volcanism in a back-arc basin and its implications for subduction processes. Tectonics. 29(4):1–18. doi:10.1029/2009TC002634.
- Giba M, Walsh JJ, Nicol A, Mouslopoulou V, Seebeck H. 2013. Investigation of the spatio-temporal relationship between normal faulting and arc volcanism on million-year time scales. Journal of the Geological Society. 170(6):951–962. doi:10.1144/jgs2012-121.
- Gregg T. 2017. Patterns and processes: subaerial lava flow morphologies: a review. Journal of Volcanology and Geothermal Research. 342. doi:10.1016/j.jvolgeores.2017.04.022.
- Hampton SJ, Cole JM. 2009. Lyttelton volcano, Banks Peninsula, New Zealand: primary volcanic Landforms and eruptive centre Identification. Geomorphology. 104(3–4):284–298. doi:10.1016/j.geomorph.2008.09.005.
- Hatherton T. 1968. A source for volcanic material in the Mohakatino beds. New Zealand Journal of Geology and Geophysics. 11:1207–1210. doi: 10.1080/00288306.1968.10420253
- Hayward BW. 1987. Identity of igneous bodies beneath the continental shelf of west Northland, New Zealand. New Zealand Journal of Geology and Geophysics. 30:93–95. doi: 10.1080/00288306.1987.10422197
- Hayward B, Black P, Smith I, Balance P, Itaya T, Doi M, Takagi M, Bergman S, Adams C, Herzer R, Robertson D. 2001. K-Ar ages of Early Miocene arc-type volcanoes in northern New Zealand. New Zealand Journal of Geology and Geophysics. doi:10.1080/00288306.2001.9514939.
- Heap MJ, Kennedy BM. 2016. Exploring the scale-dependent permeability of fractured andesite. Earth and Planetary Science Letters. doi: 10.1016/j.epsl.2016.05.004
- Heron DW (custodian). 2014. Geological Map of New Zealand 1:250 000. GNS Science Geological Map 1. Lower Hutt, New Zealand. GNS Science.
- Herzer RH. 1995. Seismic Stratigraphy of a buried volcanic Arc, Northland, New Zealand and Implications for Neogene subduction. Marine and Petroleum Geology. 12(5):511–531. Doi:10.1016/0264-8172(95)91506-K.
- Higgs KE, Strogen DP. 2014. A reservoir petrology study of the Cretaceous to Paleogene succession, well Matuku-1, offshore Taranaki Basin, GNS Science Consultancy Report 2014/202. 59 p + Appendices & 1 enclosure.
- Hochstetter F. 1867. New Zealand: its physical geography, geology and natural history. J. G. Cotta. https://collections.tepapa.govt.nz/object/1484337.
- Hoernle K, Timm C, Hauff F, Tappenden V, Werner R, Jolis EM, Mortimer N, Weaver S, Riefstahl F, Gohl K. 2020. Late Cretaceous (99-69 Ma) basaltic intraplate volcanism on and around Zealandia: Tracing upper mantle geodynamics from Hikurangi Plateau collision to Gondwana breakup and beyond. Earth and Planetary Science Letters. 529:115864. doi: 10.1016/j.epsl.2019.115864
- Hoernle K, White JV, Van Den Bogaard P, Hauff F, Coombs D, Werner R, Timm C, Garbe-Schonberg D, Reay A, Cooper A. 2006. Cenozoic intraplate volcanism on New Zealand: Upwelling Induced by Lithospheric Removal. Earth and Planetary Science Letters. 248:350–367. pp. doi: 10.1016/j.epsl.2006.06.001
- Holford SP, Schofield N, MacDonald JD, Duddy IR, Green PF. 2012. Seismic analysis of igneous systems in sedimentary basins and their impacts on Hydrocarbon Prospectivity: examples from the southern Australian margin. APPEA Journal. 52:229–252. doi: 10.1071/AJ11017
- Holz M, Küchle J, Philipp RP, Bischoff AP, Arima N. 2006. Hierarchy of tectonic control on stratigraphic signatures: base-level changes during the Early Permian in the Paraná Basin, southernmost Brazil. Journal of South American Earth Sciences. 22(3–4):185–204. doi:10.1016/j.jsames.2006.09.007.
- Hopkins JL, Smid ER, Eccles JD, Hayes JL, Hayward BW, McGee LE, van Wijk K, Wilson TM, Cronin SJ, Leonard GS, Lindsay JM, Németh K, Smith IEM. 2020. Auckland volcanic field magmatism, volcanism, and hazard: A review, New Zealand. Journal of Geology and Geophysics. doi:10.1080/00288306.2020.1736102.
- Hunt D, Tucker ME. 1992. Stranded parasequences and the forced regressive wedge systems tract: deposition during base-level fall. Sedimentary Geology. 81(1–2):1–9. doi:10.1016/0037-0738(92)90052-S.
- Illsley-Kemp F, Savage MK, Keir D, Hirschberg HP, Bull JM, Gernon TM, et al. 2017. Extension and stress during continental breakup: Seismic anisotropy of the crust in Northern Afar. Earth and Planetary Science Letters. 477:41–51. doi: 10.1016/j.epsl.2017.08.014
- Illsley-Kemp F, Savage M, Wilson C, Bannister S. 2019. Mapping stress and structure from subducting slab to magmatic rift: crustal seismic Anisotropy of the North Island, New Zealand. Geochemistry, Geophysics, Geosystems. doi:10.1029/2019GC008529.
- Infante-Paez L, Marfurt KJ. 2017. Seismic expression and geomorphology of igneous bodies: A Taranaki Basin, New Zealand, case study. Interpretation. 5(3): SK121-SK140, doi:10.1190/INT-2016-0244.1.
- Kamp PJJ. 1984. Neogene and Quaternary extent and geometry of the subducted Pacific plate beneath North Island, New Zealand: implications for Kaikoura tectonics. Tectonophysics. 108:241–266. doi: 10.1016/0040-1951(84)90238-5
- Kereszturi G, Németh C. 2013. Monogenetic basaltic volcanoes: genetic classification, growth, geomorphology and degradation: updates in volcanology. New Advances in Understanding Volcanic Systems. doi: 10.5772/51387
- Kereszturi G, Németh K. 2016. Sedimentology, eruptive mechanism and facies architecture of basaltic scoria cones from the Auckland volcanic field (New Zealand). Journal of Volcanology and Geothermal Research. 324:41–56. doi:10.1016/j.jvolgeores.2016.05.012.
- King PR. 2000. Tectonic reconstructions of New Zealand: 40 Ma to the present. New Zealand Journal of Geology and Geophysics. 43(4):611–638. doi: 10.1080/00288306.2000.9514913
- King PR, Naish TR, Browne GH, Field BD, Edbroke SW. 1999. Cretaceous to recent sedimentary patterns in New Zealand. Institute of Geological and Nuclear Sciences Folio Series. 1:35.
- King PR, Thrasher GP. 1996. Cretaceous Cenozoic geology and petroleum systems of the Taranaki Basin, New Zealand. Vol. 2. Wellington: Institute of Geological & Nuclear Sciences.
- Klarner S, Klarner O. 2012. Identification of paleo-volcanic rocks on seismic data. In: K Németh, editor. Updates in Volcanology-A comprehensive approach to volcanological problems. IntechOpen; p. 181–206.
- Knox GJ. 1982. Taranaki Basin, structural style and tectonic setting. New Zealand Journal of Geology and Geophysics. 25:125–140. doi: 10.1080/00288306.1982.10421405
- Kumar P, Kamaldeen O, Kalachand S. 2018. Sill Cube: An automated approach for the interpretation of magmatic sill complexes on seismic reflection data. Marine and Petroleum Geology. 100. doi:10.1016/j.marpetgeo.2018.10.054.
- Laird MG. 1993. Cretaceous continental rifts: New Zealand region. In: PE Ballance, editor, Sedimentary basins of the world. Amsterdam: South Pacific sedimentary basins Elsevier Sci. Publ. B.V.; p. 37-49.
- Laird MG, Bradshaw JD. 2004. The break-up of a long-term relationship: the Cretaceous separation of New Zealand from Gondwana. Gondwana Research. 7:273–286. doi: 10.1016/S1342-937X(05)70325-7
- Le Corvec N, Bebbington MS, Lindsay JM, McGee LE. 2013. Age, distance, and geochemical evolution within a monogenetic volcanic field: Analyzing patterns in the Auckland volcanic field eruption sequence. Geochemistry Geophysics Geosystems 14:3648–3665. doi:10.1002/ggge.20223.
- Lebrun JF, Lamarche G, Collot JY. 2003. Subduction initiation at a strike-slip plate boundary: The Cenozoic Pacific-Australian plate boundary, south of New Zealand. Journal of Geophysical Research. 108:2453. doi:10.1029/2002JB002041.
- Magee C, Murray H, Christopher JAL, Stephen JM. 2019. Burial-related compaction modifies intrusion-induced forced folds: Implications for reconciling roof uplift mechanisms using seismic reflection data. Front Earth Sci. doi:10.3389/feart.2019.00037.
- Manville V, Nemeth K, Kano K. 2009. Source to sink: A review of three decades of progress in the understanding of volcaniclastic processes, deposits, and hazards. Sedimentary Geology. 136–161. 10.1016/j.sedgeo.2009.04.022.
- Marfurt K. 2018. Seismic attributes as the framework for data integration throughout the oilfield life cycle. Society of Exploration Geophysicists. 508. doi:10.1190/1.9781560803522.
- Marshall P. 1932. Notes on some volcanic rocks of the North Island of New Zealand. New Zealand Journal of Science and Technology B. 13:198–202.
- Martí J, Geyer A, Folch A, Gottsmann J. 2008. A review on collapse caldera modelling. In: J. Gottsmann, J. Martí, editor. Caldera volcanism: analysis, Modelling and response. Amsterdam: Elsevier; p. 233–283.
- Massiot C, Seebeck H, Nicol A, McNamara DD, Lawrence MJ, Griffin AG, Thrasher GP, O'Brien G, Viskovic P. 2019. Effects of regional and local stress variations on slip tendency in the southern Taranaki Basin, New Zealand. Marine and Petroleum Geology. 107:467–483. doi:10.1016/j.marpetgeo.2019.05.030.
- McAlpine A. 1999. Maturation & migration modelling: PEP 38462, Offshore Taranaki, New Zealand. New Zealand Petroleum & Minerals, Ministry of Business, Innovation and Employment. Petroleum Report 2454.
- Milani E, Ramos V. 1998. Orogenias paleozóicas no domínio sul-ocidental do Gondwana e os ciclos de subsidência da Bacia do Paraná. Revista Brasileira de Geociências. 28:473–484. 10.25249/0375-7536.1998473484.
- Millett J, Wilkins A, Campbell E, Hole M, Taylor R, Healy D, Jerram D, Jolley D, Planke S, Archer S, Blischke A. 2016. The geology of offshore drilling through basalt sequences: understanding operational complications to improve efficiency. doi: 10.1016/j.marpetgeo.2016.08.010
- Milne AD. 1975. Well completion report Resolution, for BP, Shell, Todd Canterbury Service Limited. New Zealand Geological Survey Open-file Petroleum Report No. 648.
- Mitchum RM, Vail PR. 1977. Seismic stratigraphy and global changes of sea level, Part 7 : Seismic stratigraphic interpretation procedure. Seismic stratigraphy: applications to hydrocarbon exploration. AAPG Memoir 26: 135–143.
- Moorhouse BL, White JDL, Scott JM. 2015. Cape Wanbrow: A stack of Surtseyan-style volcanoes built over millions of years in the Waiareka-Deborah volcanic field, New Zealand. Journal of Volcanology and Geothermal Research. doi:10.1016/j.jvolgeores.2015.03.019.
- Morley C. 2018. 3D seismic imaging of the plumbing system of the Kora volcano, Taranaki Basin, New Zealand: The influences of syn-rift structure on shallow igneous intrusion architecture. Geosphere. doi:10.1130/GES01645.1.
- Mortimer N. 2004. New Zealand's geological foundations. Gondwana Research. 7(1):261–272. doi: 10.1016/S1342-937X(05)70324-5
- Mortimer N, Campbell HJ, Tulloch AJ, King PR, Stagpoole VM, Wood RA, Rattenbury MS, Sutherland R, Adams CJ, Collot J, et al. 2017. Zealandia: Earth’s hidden continent. GSA Today. 27:8. doi:10.1130/GSATG321A.1.
- Mortimer N, Gans P, Palin J, Meffre S, Herzer R, Skinner D. 2010. Location and migration of Miocene–quaternary volcanic arcs in the SW Pacific region. Journal of Volcanology and Geothermal Research. 190:1–10. 10.1016/j.jvolgeores.2009.02.017.
- Mortimer N, Gans PB, Meffre S, Martin CE, Seton M, Williams S, Turnbull RE, Quilty PG, Micklethwaite S, Timm C, et al. 2018. Regional volcanism of Northern Zealandia: post-Gondwana break-up magmatism on an extended, submerged continent. London: Geological Society. Special Publications 463, 28 p.
- Mortimer N, Rattenbury MS, King PR, Bland KJ, Barrell DJA, Bache F, Begg JG, et al. 2014. High-level stratigraphic scheme for New Zealand rocks. New Zealand Journal of Geology and Geophysics. 57(4):402–419. doi: 10.1080/00288306.2014.946062
- Mortimer N, Scott JM. 2020. Volcanoes of Zealandia and the Southwest Pacific. New Zealand Journal of Geology and Geophysics. 1–7. https://www.tandfonline.com/doi/full/10.1080/00288306.2020.1713824.
- Neal C, Brantley S, Antolik L, Babb J, Burgess M, Calles K, Cappos M, Chang J, Conway S, Desmither L. 2019. The 2018 rift eruption and summit Collapse of kīlauea volcano. Science. 363:367–374. doi: 10.1126/science.aav7046
- Nelson CS, Briggs RM, Kamp PJJ. 1986. Nature and significance of volcanogenic deposits at the Eocene/Oligocene boundary, Hole 593, Challenger Plateau, Tasman Sea. In: Kennett JP, et al. editors. Initial reports of the deep sea drilling project 90. Washington (DC): U.S. Government Printing Office; p. 1175–1187.
- Németh K, Kereszturi G. 2015. Monogenetic volcanism: personal views and discussion. International Journal of Earth Science (Geol Rundsch). 104:2131. doi:10.1007/s00531-015-1243-6.
- Németh K. 2010. Monogenetic volcanic fields; origin, sedimentary record, and relationship with polygenetic volcanism: Special Paper Geological Society of America. doi:10.1130/2010.2470(04).
- Nicol A, Mazengarb C, Chanier F, Rait G, Uruski C, Wallace L. 2007. Tectonic evolution of the active Hikurangi subduction margin, New Zealand, since the Oligocene. Tectonics. 26:4. doi:10.1029/2006TC002090.
- Planke S, Alvestad E, Eldholm O. 1999. Seismic characteristics of Basaltic extrusive and intrusive rocks. The Leading Edge. 18(3):342. doi:10.1190/1.1438289.
- Planke S, Millett JM, Maharjan D, Jerram DA, Abdelmalak MM, Groth A, Hoffmann J, Berndt C, Myklebust R, et al. 2017. Igneous seismic geomorphology of buried lava fields and coastal escarpments on the vøring volcanic rifted margin. Interpretation. doi: 10.1190/INT-2016-0164.1
- Planke S, Symonds PA, Alvestad E, Skogseid J. 2000. Seismic Volcanoestratigraphy of large-volume Basaltic extrusive complexes on Rifted margins. J Geophys Res. 105(B8):19335. doi:10.1029/1999JB900005.
- Rad F. 2015. Pep 38451 Romney-1 Well Completion Report. NZP&M, Ministry of Business, Innovation & Employment (MBIE), New Zealand. Unpublished Petroleum Report PR4951, 980 p.
- Rajabi M, Ziegler M, Tingay M, Heidbach O, Reynolds S. 2016. Contemporary tectonic stress pattern of the Taranaki Basin. New Zealand Journal of Geology and Geophysics. 121(8):6053–6070. doi:10.1002/2016JB013178.
- Reynolds P, Schofield N, Brown RJ, Holford SP. 2016. The architecture of submarine monogenetic volcanoes - insights from 3D seismic data. Basin Research. 30:437–451. doi:10.1111/bre.12230.
- Sahoo TR, Kroeger KF, Thrasher G, Munday S, Mingard H, Cozens N, Hill M. 2015. Facies distribution and Impact on petroleum migration in the Canterbury Basin, New Zealand. Eastern Australian basins Symposium 2015: Publication of Proceedings. Perth, WA: Petroleum Exploration Society of Australia; p. 187–202.
- Schiøler P, Powell S, Rexilius J. 2014. PEP 38451 biostratigraphy of Romney-1 well (2370–4618 m) Deepwater Taranaki, New Zealand. New Zealand Unpublished Openfile Petroleum Report, PR4951.
- Schiøler P, Raine JI, Griffin A, Hollis CJ, Kulhanek DK, Morgans HEG, Roncaglia L, Strong CP, Uruski C. 2011. Revised biostratigraphy and well correlation, Canterbury Basin, New Zealand. GNS Science Consultancy Report 2011/12. 142 p.
- Scott JM, Pontesilli A, Brenna M, White JDL, Giacalone E, Michael Palin J, le Roux PJ. 2020. The Dunedin Volcanic Group and a revised model for Zealandia's alkaline intraplate volcanism, New Zealand. Journal of Geology and Geophysics. doi:10.1080/00288306.2019.1707695.
- Seebeck H, Nicol A, Giba M, Pettinga J, Walsh JJ. 2014. Geometry of the subducting Pacific plate since 20 Ma, Hikurangi margin, New Zealand. Journal of the Geological Society. 171:131–143. 10.1144/jgs2012-145.
- Sewell RJ. 1988. Late Miocene volcanic Stratigraphy of central Banks Peninsula, Canterbury, New Zealand. New Zealand Journal of Geology and Geophysics. 31(March 2015):41–64. doi:10.1080/00288306.1988.10417809.
- Shaw HR. 1980. Fracture mechanisms of magma transport from the mantle to the surface. In: Hardgraves R.B, editor. Physics of magmatic processes. Princeton, NJ: Princeton University Press; p. 201–264.
- Shumaker L, Sharman G, King P, Graham S. 2018. The source is in the sink: deep-water deposition by a submarine volcanic arc, Taranaki Basin, New Zealand. Sedimentology. 2018:65. doi:10.1111/sed.12475.
- Sigmundsson F, Parks M, Pedersen R, Jónsdóttir K, Ófeigsson BG, Grapenthin R, Dumont S, Einarsson P, Drouin V, Heimisson ER, et al. 2018. Magma Movements in volcanic plumbing systems and their associated ground deformation and seismic patterns. In: Steffi Burchardt, editor. Volcanic and igneous plumbing systems. Amsterdam: Elsevier; p. 285–322. doi:10.1016/B978-0-12-809749-6.00011-X.
- Strogen DP, Seebeck H, Nicol A, King PR. 2017. Two-phase Cretaceous–paleocene rifting in the Taranaki Basin region, New Zealand; implications for Gondwana break-up. Journal of the Geological Society. doi:10.1144/jgs2016-160.
- Sutherland R, Collot J, Bache F, Henrys S, Barker D, Browne GH, Lawrence MJ, Morgans HE, Hollis CJ, Clowes C, et al. 2017. Widespread compression associated with Eocene Tonga-Kermadec subduction initiation. Geology. 45:G38617.1. doi:10.1130/G38617.1.
- Sutherland R, King PR, Wood RA. 2001. Tectonic evolution of Cretaceous rift basins in south-eastern Australia and New Zealand: implications for exploration risk assessment. In: Hill K.C., Bernecker T, editor. Eastern Australasian basins symposium 2001. Melbourne: Petroleum Exploration Society of Australia; p. 3–13.
- Timm C, Hoernle K, Werner R, Hauff F, van den Bogaard P, White J, Mortimer N, Garbe-Schönberg D. 2010. Temporal and geochemical evolution of the Cenozoic intraplate volcanism of Zealandia. doi: 10.1016/j.earscirev.2009.10.002
- Waight TE, Maas R, Palin JM, Sahoo T, Seebeck H, Sagar MW, Barrier A, Turnbull RE. 2019. Reconnaissance basement geology and tectonics of south Zealandia. Tectonics. 38:516–551. doi: 10.1029/2018TC005116
- Uruski CI. 2020. Seismic recognition of igneous rocks of the Deepwater Taranaki Basin, New Zealand, and their distribution, New Zealand. Journal of Geology and Geophysics. 63(2):190–209. doi:10.1080/00288306.2019.1647253.
- Uruski C. 2019. Seismic recognition of igneous rocks of the Deepwater Taranaki Basin, New Zealand, and their distribution. New Zealand Journal of Geology and Geophysics. 1–20.
- Uruski CI, Warburton J. 2010. Seismic interpretation and petroleum prospectivity report; DTB08 2D seismic survey. New Zealand Petroleum & Minerals, Ministry of Business, Innovation and Employment; Petroleum Report 4115.
- Van der Meer Q, Waight TE, Tulloch A, Whitehouse MJ, Andersen T. 2018. Magmatic evolution during the Cretaceous transition from subduction to continental break-up of the Eastern Gondwana margin (New Zealand) documented by in-situ Zircon O–Hf isotopes and bulk-rock Sr–Nd isotopes. Journal of Petrology. 59:849–880. doi: 10.1093/petrology/egy047
- Vries B, Vries M. 2018. Tectonics and volcanic and igneous plumbing systems. In: Burchardt S, editor. Volcanic and igneous plumbing systems. Amsterdam: Elsevier; p. 111–136. doi:10.1016/B978-0-12-809749-6.00007-8.
- Walcott RI. 1978. Present tectonics and late Cenozoic evolution of New Zealand. Geophysical Journal of the Royal Astronomical Society. 52:137–164. doi: 10.1111/j.1365-246X.1978.tb04225.x
- Weaver SD, Smith IEM. 1989. New Zealand intraplate volcanism. In: Johnson RW, editor. Intraplate volcanism in eastern Australia and New Zealand. Cambridge: Cambridge University Press; p. 157–188.
- Wilding A, Sweetman LAD. 1971. PR303 Endeavour-1 well completation report. Offshore Canterbury, New Zealand. Unpublished Open file Petroleum Report, PR303.
- Zhu H, Seebeck H, King P. 2017. Late Cretaceous volcanism in Taranaki Basin: examples from seismic reflection data. AAPG GTW influence of volcanism and associated magmatic processes on petroleum systems conference, Oamaru New Zealand.