ABSTRACT
Monogenetic volcanism in the Maniototo Basin in the Dunedin Volcanic Group was unusual because the eroded lavas, plugs and dikes comprise spatially and temporally restricted silica-saturated and silica-undersaturated magmas. 40Ar/39Ar dating coupled with existing data and field relationships indicate that volcanism was focused at ∼11 Ma. Major and trace element data show the volcanic rocks to have mafic compositions and little evidence for fractionation and/or crustal contamination, but to have been variably altered. The volcanic rocks comprise an alkaline group and an alkaline to subalkaline transitional group, with one intermediate unit. With the exception of one flow, the transitional group has more radiogenic 87Sr/86Sr and less radiogenic 143Nd/144Nd, 206Pb/204Pb and 208Pb/204Pb than the alkaline group, as well as smaller Ti and K primitive mantle-normalised anomalies. Overall, the different magma compositions cannot be related to varying degrees of melting of equivalent mantle sources. Trace element ratios indicate that residual Ti and K-bearing mantle phases (amphibole, phlogopite) probably played a major role in the source of alkaline group but a smaller role in the transitional group. Therefore, the varying compositions of the Maniototo volcanic rocks relates to melting of a heterogeneous lithospheric mantle, or/and varying degrees of interaction with mantle hydrous metasomes as the magmas ascended.
Introduction
The origin of dispersed intraplate volcanism is a contentious topic. Asthenospheric and/or lithospheric mantle involvement is commonly invoked to explain the range of magma compositions found in intraplate volcanic provinces (e.g. Finn et al. Citation2005; Hoernle et al. Citation2006; Pilet et al. Citation2008; Dasgupta et al. Citation2010; Rooney et al. Citation2017; Mather et al. Citation2020; Smith et al. Citation2021). Classically, such provinces are subdivided into sub-alkaline (silica-saturated) and alkaline (silica-undersaturated) evolution trends (Coombs and Wilkinson Citation1969; Irvine and Baragar Citation1971). However, with the collection of more data, it is becoming increasingly recognised that silica-saturated and silica-undersaturated magmas have occurred contemporaneously within single New Zealand magmatic provinces (Cook et al. Citation2005; Brenna et al. Citation2012; Pontesilli et al. Citation2021). These cases require the magmas to be derived from melting of multiple lithologically discrete mantle sources (e.g. McGee et al. Citation2013) and/or to have been modified during ascent through the lithosphere (e.g. Brenna et al. Citation2021).
Here, we investigate rocks in the Maniototo Basin in the NW area of the Dunedin Volcanic Group (). The intraplate volcanic field represented by the Dunedin Volcanic Group was distributed over ∼7,800 km2 with eruptions over a ∼15 Myr period that show no spatial systematic variation (Coombs et al. Citation1986, Citation2008; Scott et al. Citation2020a). Limited data collected as part of a regional review revealed that some volcanic rocks in the Maniototo Basin have sub-alkaline or alkaline compositions and are distinct from all other monogenetic components in this province (Scott et al. Citation2020a). Here, we provide a comprehensive analysis of the distribution, composition and mantle sources for these significant volcanic rocks through sampling, petrography, whole rock major and trace element data, Sr-Nd-Pb isotopic data and 40Ar/39Ar dating.
Geological setting
Intraplate volcanism has been occurring in Zealandia for ∼100 million years (Timm et al. Citation2010; Hoernle et al. Citation2006; van der Meer et al. Citation2017; Mortimer and Scott Citation2020). This volcanism in NZ is commonly characterised by small monogenetic eruptions and a lack of systemic relationship between volcanic field location and age or composition (Coombs et al. Citation1986; Cooper Citation1986; Reay et al. Citation1991; Baker et al. Citation1994; Hoernle et al. Citation2006; Panter et al. Citation2006; Sprung et al. Citation2007; Coombs et al. Citation2008; Timm et al. Citation2009, Citation2010; McCoy-West et al. Citation2010; Timm et al. Citation2010; van der Meer et al. Citation2013; Scott et al. Citation2013; McLeod and White Citation2018; van der Meer et al. Citation2016, Citation2017; Gamble et al. Citation2018; Scott and Turnbull Citation2018; Scott et al. Citation2020a, Citation2020b; Cooper Citation2020; Hopkins et al. Citation2021; Smith and Cronin Citation2021; Scott et al. Citation2023).
The Dunedin Volcanic Group in the South Island of New Zealand comprises more than 150 dispersed but eroded eruptive centres across an area of ∼ 7,800 km2 (Coombs et al. Citation1986, Citation2008; Scott et al. Citation2020a) (). 40Ar/39Ar and K-Ar dates indicate that eruptions occurred from 24 to 9 Ma (McDougall and Coombs Citation1973; Hoernle et al. Citation2006; Coombs et al. Citation2008; Timm et al. Citation2010; Fox et al. Citation2015; Kaulfuss et al. Citation2018; Scott et al. Citation2020a), although we have some reservations about the ages < 11 Ma due to their low radiogenic Ar yields (Scott et al. Citation2020b). The Dunedin Volcanic Group therefore represents one of the largest and longest-lived episodes of intraplate volcanism in Zealandia. Chemical and isotopic data for the province show the magmas to have been dominantly alkaline in composition (Coombs et al. Citation1986, Citation2008; Reay et al. Citation1991; Scott et al. Citation2020a), although some mafic magmas appear to have been sub-alkaline (Scott et al. Citation2020a; Pontesilli et al. Citation2021, Citation2022). The Dunedin Volcanic Group comprises rocks of:
The well-studied Dunedin Volcano (), which was a zone of focused melting and magma fractionation centred on Dunedin from ∼16 to 11 Ma (Benson Citation1941, Citation1942; Coombs et al. Citation1960; Coombs and Wilkinson Citation1969; Price and Compston Citation1973; Price and Taylor Citation1973; Price and Chappell Citation1975; Coombs et al. Citation2008; Price et al. Citation2003; Hoernle et al. Citation2006; Sprung et al. Citation2007; Scott et al. Citation2020a; Pontesilli et al. Citation2021, Citation2022; Baxter et al. Citation2022); and
the ∼24 to 9 Ma less well-studied remnants of outlying, widespread and typically monogenetic maars and lava flows dispersed in the surrounding Otago region (McDougall and Coombs Citation1973; Coombs et al. Citation1986; Reay et al. Citation1991; Nemeth and White Citation2003; Coombs et al. Citation2008; Scott et al. Citation2020a) (). These outlying volcanic rocks were formerly attributed to the Waipiata Volcanic Field, but this term is unnecessary because these rocks are spatially and temporally part of the Dunedin Volcanic Group (see Scott et al. (Citation2020a) for a discussion).
Rocks of the Dunedin Volcanic Group are primarily silica-undersaturated and represent a range of magma types. Basanite is most common but fractionation has resulted in phonolites and trachytes (Price et al. Citation2003; Coombs et al. Citation2008; Scott et al. Citation2020a; Pontesilli et al. Citation2021; Baxter et al. Citation2022; Pontesilli et al. Citation2022). Most of the Dunedin Volcano’s primitive components have potassic or sodic compositions whereas the outlying lavas are dominantly sodic (Reay et al. Citation1991; Coombs et al. Citation2008; McLeod and White Citation2018; Scott et al. Citation2020a; Pontesilli et al. Citation2021). Strontium, Nd, Pb and Hf isotopic data indicate that the mantle source to the bulk of the volcanic rocks trends towards the HIMU or FOZO mantle reservoirs (Price and Compston Citation1973; Hoernle et al. Citation2006; Sprung et al. Citation2007; Scanlan et al. Citation2020; Scott et al. Citation2020a; Pontesilli et al. Citation2021). Important recent discoveries are that the isotopic compositions of the alkaline volcanic rocks largely overlap with the isotopic properties of metasomatised mantle lithosphere beneath Otago (Scott et al. Citation2014a,b; McCoy-West et al. Citation2016; Scott et al. Citation2016; Dalton et al. Citation2017; Scott et al. Citation2023).
Central and eastern Otago – the area of our study – is characterised by a series of basins and ranges, of which one of the largest is the Maniototo Basin (Youngson et al. Citation1998; Craw et al. Citation2016) (). The basin basement comprises greenschist facies Otago Schist (Mortimer Citation2000) overlain by Cenozoic sediments, which are in turn overlain and intruded by Dunedin Volcanic Group rocks (e.g. Bishop Citation1974; Coombs et al. Citation1986; Youngson et al. Citation1998). While volcanic rocks in the Maniototo Basin have long been examined (e.g. Benson Citation1941, Citation1942), key observations are only provided by Bishop (Citation1974), Coombs et al. (Citation1986) and Nemeth and White (Citation2003). Of the > 90 dates for the entire volcanic field, only three are from the abundant volcanic rocks this basin (McDougall and Coombs Citation1973; Youngson et al. Citation1998) and the only published isotopic (Sr-Nd-Pb-Mg) compositions have been reported by Scott et al. (Citation2020a) as part of a regional synthesis.
Methods
Major and trace element analysis
Whole rock major and trace element analysis was performed on rocks that were first trimmed using a diamond tipped rock saw, with all cut surfaces then ground using 100 grade grit to remove saw marks. Samples were then double bagged and smashed with a hammer, and then crushed to a powder in a tungsten carbide rock mill. Whole rock analysis was undertaken by ALS Geochemistry in Brisbane, Australia. Loss on ignition (LOI) values were measured using ALS standard method ME-GRAO05, with 1 g of sample heated to 1000°C before being cooled, reweighed and loss on ignition calculated. Trace elements were measured by inductively coupled plasma mass spectrometry (ICP-MS), following standard method ME-MS81 on solutions made by dissolution of glass beads from lithium-borate fusion.
Sr-Nd-Pb isotope analysis
Whole rock isotopic analysis was undertaken at the Department of Geological Sciences, University of Cape Town MC-ICP-MS facility. All analyses were conducted using a NuPlasma HR mass-spectrometer with a DSN-100 desolvating nebuliser, using laboratory and analytical procedures detailed by Harris et al. (Citation2015). For each sample, 50 mg of sample powder was dissolved in a 4:1 HF/HNO3 acid mixture before being converted to nitrates by applying a concentrated HNO3 solution. Strontium, Nd, and Pb fractions were isolated through sequential column chemistry (Pin et al. Citation2014). The neodymium elemental fractions were analysed as 50 ppb 2% HNO3 solutions and data normalised to 0.512115 for bracketing analyses, using Jndi-1 reference material (Tanaka et al. Citation2000). All Nd isotope data were corrected for Ce and Sm interference using the measured signals for 140Ce and 147Sm and the natural isotopic abundances of Sm and Ce. Instrumental mass fractionation was accounted for using the exponential law and a 146Nd/144Nd value of 0.7219. Strontium elemental fractions were analysed as 200 ppb 0.2% HNO3 solutions with data normalised against 0.710255 for the bracketing analyses, with NIST SRM987 used as a reference material. 87Sr/86Sr data were corrected for Rb interference using the measured signal for 85Rb and the natural 85Rb/87Rbratio, while instrumental mass fractionation was accounted for using the exponential law and an 86Sr/88Sr value of 0.1194. Lead elemental fractions were analysed as 50 ppb 2% HNO3 solutions. Aliquots of NIST SRM997 Tl standard were added to all standards and samples analysed to give ± 10:1 Pb:Tl ratios. NIST SRM981 was the reference standard, with the following reference values used: 208Pb/204Pb: 36.7219, 207Pb/204Pb: 15.4963, and 206Pb/204Pb 16.9405 (Galer and Abouchami Citation1998). Instrumental mass fractionation of the measured Pb isotope ratios corrected using the reference 205Tl/203Tl value of 2.3889 and the exponential law. BHVO-2 standards processed and analysed concurrently yielded: 143Nd/144Nd = 0.512988 ± 0.000013 (n = 3) (published value of 0.512984 ± 0.000011), 87Sr/86Sr = 0.703488 ± 0.000011 (n = 3) (published value of 0.703479 ± 0.000020), and 206Pb/204Pb = 18.6238 ± 0.0011 (n = 2) (published value of 18.6290 ± 0.0092), 207Pb/204Pb = 15.5281 ± 0.0011 (n = 2) (published value of 15.5334 ± 0.0094), and 208Pb/204Pb = 38.2193 ± 0.0036 (n = 2) (published value of 38.2367 ± 0.0182). Published results are from Weis et al. (Citation2006).
To assess the effect of post-magmatic alteration on the bulk-rock Sr analyses, in situ 87Sr/86Sr data for plagioclase and carbonate were obtained using a RESOlution 193 m ArF laser ablation system with an M-50 laser ablation cell coupled to a Nu Instruments Nu Plasma-HR MC-ICP-MS at the University of Otago, following the method and analytical configuration was reported in Scanlan et al. (Citation2018). Each analysis was performed in raster mode in a pure He atmosphere and ablated material transported into the MC-ICP-MS using an Ar carrier gas with a flow rate of ∼1 L/min. The beam was 90 μm beam with a 10 Hz repetition rate and an on-sample fluence of 2.5 J/cm2. Each analysis had a pre-ablation stage to remove possible surface contamination, followed by a 35 s cell gas purge before 20 to 40 s of sample ablation. Ion beam intensities at masses 88, 87, 86, 85.5, 85, 84, 83.5, 83, and 82 were monitored across an array of Faraday collectors operating with 1011ohm resistors. Instrumental mass fractionation was corrected for using the measured 86Sr/88Sr ratio normalized to 0.1194 and the exponential mass fractionation law. The 87Sr/86Sr results were checked after correction for Rb, molecular dimer, and REE interferences, as well as instrumental mass bias for accuracy and precision and normalised to Tridacna clam (0.709176; Neymark et al. Citation2014) with an in-house anorthoclase standard KAN providing a reference check. KAN analyses yielded values of 87Sr/86Sr = 0.70290 ± 0.00006, which overlap the published value (0.70290; Burgin et al., Citation2023).
40Ar/39Ar Isotope analysis
To determine their age, two samples were irradiated along with Fish Canyon sanidine standard for 17.5 h in the off axis position at the United States Geological Survey TRIGA Reactor. The irradiated samples were analysed using the automated LDEO-AGES GV 5400 noble gas mass spectrometer with MassSpec software from the Berkeley Geochronology Center. Measurements were made in peak hopping mode on an analogue multiplier, and corrections were made for backgrounds based on blank runs approximately every 4th sample run, mass discrimination based on air measurements approximately every 12th sample run, and nuclear interferences based on production ratios reported in Dalrymple et al. (Citation1981). The J-value applied, 3.648e−3 ± 2.5e−6 (0.07%) is based on the average values for Fish Canyon sanidine monitors from 4 pits. The individual dates are reported at ± 1σ analytical uncertainties, relative to the Fish Canyon age of 28.201 (Kuiper et al. Citation2008) and using the decay constants of Min et al. (Citation2000).
Results
The volcanic rocks mapped and sampled across the Maniototo Basin ( and , ) are described below. We first give a field and petrographic description of each unit, and then subsequently use this framework to present the whole rock major, trace and isotope analyses.
Figure 1. Geological map of the Maniototo Basin. Volcanic rocks are shown in red. Buried lavas were interpreted by Scott et al. (Citation2020a) using regional geophysical data.
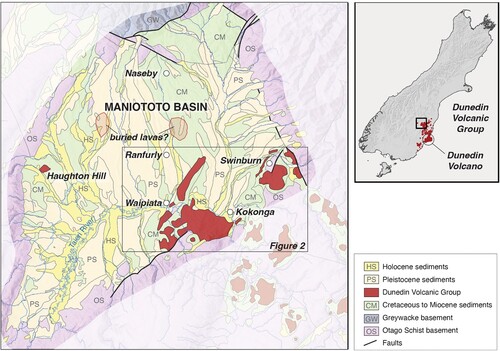
Figure 2. A, Geological maps of the eastern Maniototo Basin, showing the distribution of the different geological units discussed in the paper, with a close up B, of the area comprising a variety of different magmatic rocks on the northern edge of the Waipiata flow. Geology from QMAP and our own mapping.
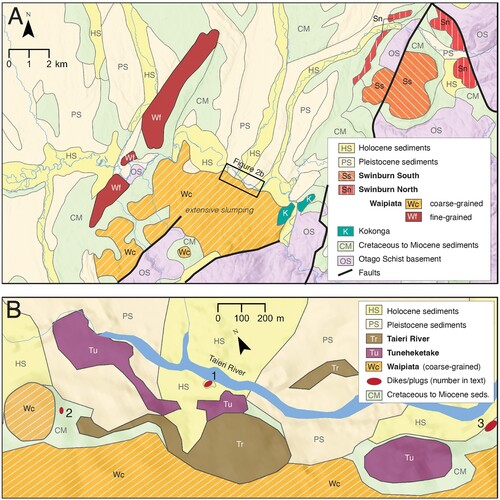
Table 1. Summary of the volcanic rocks units. Mineral abbreviations: Ol, olivine; Pl, plagioclase; Cpx, clinopyroxene; Il, ilmenite; Ti-mag, titanomagnetite, Carb, carbonate; Zeol, zeolite; Serp, serpentinite; Ne, nepheline; Gl, glass; Pal, palagonite; Idd, iddingsite.
Haughton Hill
Haughton Hill is an isolated flow in rolling farmland on the western side of the Maniototo Basin (). It is best exposed as a slightly greater than 10 m thick lava in an old quarry on the SW side of the hill (A). It overlies a thin tuff layer, which in turn sits on Miocene terrestrial sediments. The lava is medium to fine grained, holocrystalline and has a diktytaxitic porphyritic texture. It contains phenocrysts of olivine and plagioclase and rare glomerocrysts of olivine. The olivine crystals form subhedral, corroded megacrysts ∼1200 μm long that contain inclusions of < 20 μm spinel and have calcite-pseudomorphed rims and fractures. The plagioclase phenocrysts are subhedral to anhedral, up 1000 μm long, and have been partially grown over by groundmass, which consists of plagioclase, olivine, clinopyroxene, ilmenite and titanomagnetite. Sub-horizontal coarsely crystalline segregation veins running thorough the basalt comprise plagioclase that often contains needle-like apatite inclusions, pink and green zoned clinopyroxene, opaque minerals, zeolites and interstitial carbonate (B and A). Calcite-filled amygdales are common and carbonate is present in the ground mass. Haughton Hill has a 40Ar/39Ar date of 10.9 ± 0.3 Ma (unpublished data of D. Lee reported in Scott et al. (Citation2020a)).
Figure 3. A, Exposure in the Haughton Hill quarry, with inset B, showing an example of the sub-horizontal segregation veins. Fine-grained Waipiata flow overlying coal-bearing silty-mudstone. C, Swinburn North volcanic rocks dip gently northwest. D, The Swinburn South volcanic rocks form a large plateau. E, Swinburn South contains coarse segregation patches.
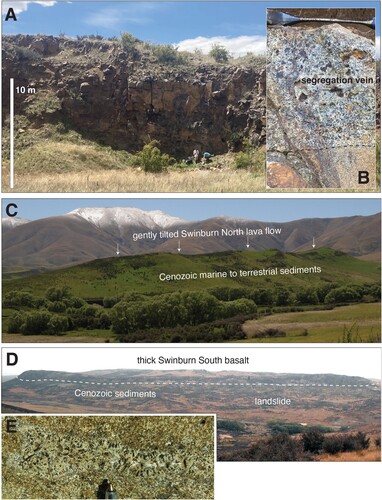
Kokonga
Kokonga is located on the east of the major Waipiata lava (A). It is a uniformly fine-grained, medium grey rock best exposed as an at least 5 m thick layer dipping gently northwards in the main roadside outcrop. Contacts with the adjacent Waipiata lava are unclear but it clearly overlies sedimentary rocks. Kokonga is holocrystalline, and has a porphyritic, intergranular texture with phenocrysts of olivine and plagioclase (B). Olivine forms clusters of subhedral phenocrysts ∼1500-2000 μm long or as subhedral and rounded grains that often contain inclusions of spinel and have sieve textures close to the rim. The plagioclase phenocrysts are subhedral and are mainly ∼500 μm long and ∼200 μm wide. The crystalline groundmass consists of clinopyroxene, plagioclase, ilmenite and titanomagnetite, with secondary calcite present within small vesicles. It has a K-Ar age of 11.8 ± 0.7 Ma (Coombs et al. Citation2008).
Figure 4. Range of lithologies in the Maniototo basaltic rocks. A, Haughton Hill basalt with coarse segregations containing late stage minerals and green clinopyroxene overgrowths. B, Kokonga lava with diktytaxitic texture and vesicles infilled by carbonate. C, Swinburn North olivine-phyric lava with microcrystalline groundmass. D, Swinburn South coarsely-crystalline basalt (dolerite) with ophitic intergrowth of clinopyroxene and plagioclase. E, Tuneheketake finely crystalline lava containing kink-banded olivine xenocrysts. F, Waipiata coarsely crystalline lava with iddingsitised olivine phenocrysts. G, olivine phenocrysts in coarsely crystalline lava with unaltered rims over iddingsitised mantles. H, Waipiata finely crystalline dyke with zoned olivine phenocrysts. Mineral abbreviations: carb = carbonate, cpx = clinopyroxene, idd = iddingsite, plag = plagioclase, ol = olivine, zeol = zeolites.
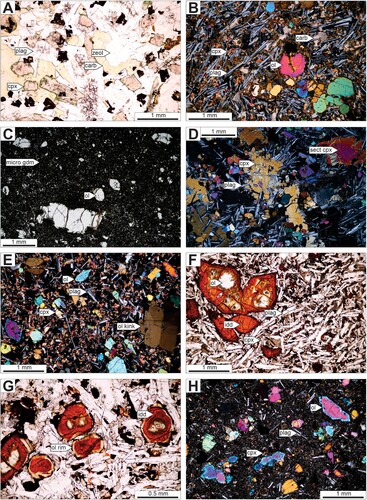
Swinburn North
Swinburn North is located north and east of State Highway 85 (A), and consists of thin lava (or lavas) that rest unconformably on Miocene terrestrial sediments that in turn overlie Cenozoic marine sediments (Youngson et al. Citation1998) (C). These rocks have a microlitic groundmass of clinopyroxene, plagioclase and Fe-Ti oxides with euhedral to subhedral microphenocrysts of olivine (C). Samples commonly contain many small cm or smaller sized peridotite (lherzolite) xenoliths. A Swinburn North sample has an unpublished K-Ar date of 13.4 ± 0.3 Ma (Youngson et al. Citation1998).
Swinburn South
Swinburn South is a major unit located along the eastern side of the Maniototo Basin (A and D). It is dominated by a flow that is relatively thick compared with others in the Maniototo Basin, but also comprises multiple scoria cones, tuff rings, small diatremes and feeder dykes. It sits above thin pyroclastic layers, which in turn rest on Miocene lacustrine sediments of varied thickness. The lava is locally >30 m thick in the southern area where it fills a well-defined paleo-valley, and is consistently coarsely crystalline basalt with impressive very coarsely crystalline segregation structures. There is a broad variety of lava textures and pyroclastic rock types, often in restricted, m-scale areas, with different units having complex intrusion and mingling relationships. Lithologies vary from those with distinctive ophitic to poikilitic texture, defined by clinopyroxene and olivine oikocrysts (0.5-2 mm wide) surrounding plagioclase chadacrysts (<2 mm long) (D), to finely crystalline olivine-free units. Segregation bodies have larger crystal sizes (5–20 mm long) and host late-stage evolved minerals such as rims of green clinopyroxene on pink cores, amphibole and zeolites. A slightly altered Swinburn South rock from a large disused quarry has two K-Ar ages of 16.5 ± 1.3 Ma and 15.6 ± 0.6 Ma (McDougall and Coombs Citation1973).
Taieri River
Taieri River is a dark grey, fine-grained body forming a small hill on the northeast side of the coarse-grained Waipiata flow (B and A). It is holocrystalline and has an intergranular texture and contains phenocrysts of olivine, plagioclase and titanomagnetite. The olivine phenocrysts are subhedral with spinel inclusions, but are have fractures containing green-yellow (serpentinite?) alteration. The groundmass consists of plagioclase, clinopyroxene, olivine and titanomagnetite. The clinopyroxene is euhedral and pale grey with hints of pink, existing as both individual crystals and clumps, with rare inclusions. It is undated and the stratigraphic position is unclear other than being in close proximity to Eocene and younger sediments.
Tuneheketake
Tuneheketake, named after a historical lake in the region, is a dark grey body exposed along the northern side of the Waipiata flow close to the Taieri River flow (A). The contacts between flows are not exposed and the flow has probably been extensively eroded. It has columnar jointing and has a vertical exposure of nearly 50 m in the southeastern portion (B), which may be a plug. The lithology contains abundant ∼200 μm olivine phenocrysts as well as coarser, generally <500 μm larger anhedral kink banded olivine set in a fine groundmass consisting of glass, olivine, titanomagnetite and rare ilmenite, plagioclase, nepheline, and clinopyroxene (E). The clinopyroxene is euhedral to subhedral and pale brown to pink and often forms rosette-like clumps. Green to brown alteration and rare zeolites are also present. This unit petrographically matches the sample reported as ‘Waipiata-Kokonga’ and K-Ar dated as 11.1 ± 0.7 Ma by Coombs et al. (Citation2008).
Figure 5. A, Major outcrop of Taieri River basalt. B, The Tuneheketake unit is exposed over 50 m vertical meters in the southern area of the unit and consist of vaguely columnar-jointed rocks. Person in the centre for scale. C, The Waipiata flow is one of the largest in Otago. It dips gently northwards towards the camera, and most of the background is composed of the coarse-grained facies. The photo is taken from the roadside on the fine-grained facies, looking south. D, The fine-grained Waipiata flow overlies coaliferous silt-mudstone sediments that may be laucustrine. This image is from close to the bridge over the Taieri River, south of Waipiata. E, Dike 3 intrudes Cenozoic sediments and is readily visible at low flow in the Taieri River.
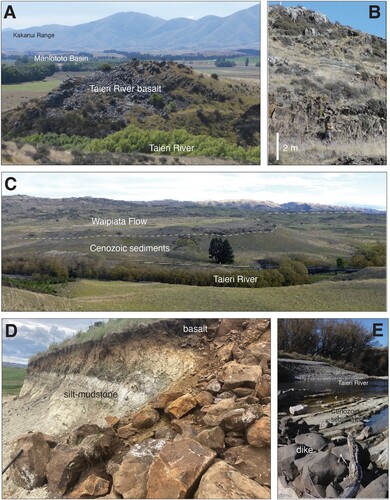
Waipiata
The Waipiata flow occurs over ∼ 30 km2 and is one of the largest in the entire Dunedin Volcanic Group (). It has medium to coarse-grained facies forming the largest portion, which has undergone extensive slumping, as well as a fine-grained facies that occurs along the western to north-western side (A and B). The medium to coarse-grained facies is up to ∼20 m thick but the fine-grained facies is only 2.5 m thick. Both sit stratigraphically above sedimentary rocks, although an exposed basal contact with underlying coalified (laucustrine?) sediments has only been observed beneath the fine-grained portion (D).
The medium to coarse-grained facies (herein: ‘coarse-grained’) is medium to pale grey, crystal rich and contains abundant micro-vesicles (B). It is holocrystalline with a porphyritic diktytaxitic texture, containing phenocrysts up to 2 mm of olivine and 0.5 mm zoned plagioclase (F). The groundmass consists of clinopyroxene, fine-grained plagioclase, ilmenite and titanomagnetite, and olivine rimed by clinopyroxene. Olivine phenocrysts are variably iddingsitised and also show unaltered overgrowths on iddingsite mantles (G).
The fine-grained facies has a porphyritic diktytaxitic texture. The phenocrysts consist of olivine, mostly between 0.5 and 1 mm, and rare euhedral to subhedral plagioclase (∼0.5 mm) with oscillatory zoning and tiny inclusions of clinopyroxene (< 20 μm). The groundmass consists of twinned plagioclase, clinopyroxene, olivine, ilmenite, titanomagnetite and spinel. Vesicles vary in size and shape, and are mainly filled with calcite and other secondary minerals. Some areas of the unit contain a small quantity of glass that has mostly altered to palagonite and other associated minerals.
40Ar/39Ar dates were obtained for the coarse- and fine-grained facies, and full raw data are provided in Supplementary Table 1. For each sample, the data were examined on a step heating diagram with ages calculated assuming atmospheric initial (Lee et al. Citation2006), each was plotted on an isochron, and each was plotted on a second step-heating diagram using the initial from the isochron. In the ideal case all three of these approaches would yield the same result and all data would fall on the plateau. As this is rarely the case, an unemotional strategy for eliminating steps must be used. We only omitted steps at the beginning and end of the experiment, leaving the maximum number of steps in the middle, while dropping the MSWD to acceptable levels (ideally ∼1). In the case of LW 23GW, all but the final 3 steps (92.7% of the 39Ar) lie on an isochron with age of 10.95 ± 0.15 Ma, initial of 295 ± 4, and MSWD of 1.1. In addition to significantly increasing the age scatter, the Ca/K of the last three steps is notably higher than the others and the %radiogenic is negative (values lower than atmosphere). The plateau age using the isochron initial is 10.95 ± 0.11 Ma, with MSWD. of 0.98 and p = 0.44 (A). The data have an isochron age of 10.95 ± 0.15 Ma with an 40Ar/36Ar intercept of 295 ± 4, which overlaps the atmospheric value and indicates that this sample contains very little (or no) excess Ar (B). The plateau age using the isochron initial is therefore the preferred age.
Figure 6. 40Ar/39Ar dates from two Waipiata samples. A, B, coarse-grained Waipiata; C, D, fine-grained Waipiata. For the plateaus, the dark shaded areas represent the preferred plateau steps used to calculate an age. For the isochrons, data excluded are shown in red and the letters correspond to step-heating stages. Errors are shown by the size of the boxes and ellipses.
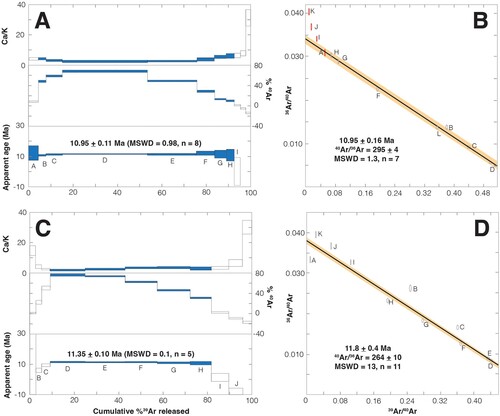
Sample LW 2GW contains several steps at the beginning and end of analysis with low apparent ages calculated using the assumption of atmospheric initial (C). The five steps that define the plateau account for 72.6% of the 39Ar and these yield a plateau age of 11.12 ± 0.10 Ma, with MSWD of 0.7 and p of 0.59. The isochron plot is messy, with MSWD of 13 and p of 0 (D). The 5 steps that define the plateau yield an anomalously low MSWD of 0.082 with p of 0.92. Accordingly the plateau age using the Lee et al. (Citation2006) initial is considered the best estimate of the age of this sample. The two sample ages LW 23GW = 10.95 ± 0.11 Ma and LW 2GW = 11.12 ± 0.10 Ma are indistinguishable, and we note with interest that both end with steps that have much higher Ca/K and anomalously low 40Ar/36Ar, potentially related to the eruption or early alteration processes.
Dikes
Several dikes and/or plugs occur in sediments (B and E) but are not readily attributed to any other component. Dike 1 is dark grey with olivine phenocrysts in a very fine groundmass and olivine, plagioclase, opaque minerals and rare clinopyroxene (H). Dike 2, consisting of multiple offshoots emplaced into fine sediments and very well exposed in the riverbed, is black, weathering to dark brown, and fine-grained. Small (≤ 0.5 mm) crystals of olivine, clinopyroxene and plagioclase are visible, as well as small xenoliths of incorporated sediment (< 3%; < 5 mm across). Dike 3 forms a singular isolated outcrop between the Waipiata and Kokonga flows and emplaced into the same sediments. The body is light to medium grey in colour, with varying concentrations of vesicles, small phenocrysts of olivine and plagioclase and rare (< 1%), small (< 1 cm) schist and tiny peridotite xenoliths.
Whole rock geochemistry
As the volcanic rocks of the Maniototo Basin show signs of alteration, we first assess the affect of this on major and trace element compositions before classifying the suites. Using our new data coupled with that from Donnelly (Citation1996), Coombs et al. (Citation2008) and Scott et al. (Citation2020a) (), there is major element variation that correlates with LOI. CaO, for example, shows a positive trend against LOI in Taieri River, Waipiata, Haughton Hill and, possibly, Swinburn South samples (A). There is slight K2O loss in the Swinburn South samples (B). Fe2O3 and MgO have negative trends in Taieri River, Swinburn South and Haughton Hill rocks (C, D). The MgO content of the Waipiata samples either shows a slight positive trend with LOI, or scatter towards low MgO contents at low LOI (D). On the basis of trends against LOI, we therefore exclude from the characterisation: Haughton Hill 1aHH and OU66560; Swinburn North SP; Swinburn South G15 and STOP3; Waipiata OU66561, 5aW, 20aUL and 25aFL; and Taieri River 11aUL.
Table 2. Whole rock major and trace element analyses of samples analysed in this study, together with those from Donelly (Citation1996), Coombs et al. (Citation2008) and Scott et al. (Citation2020a).
Table 2. Continued
Table 2. Continued
The filtered Maniototo Basin volcanic rocks comprise two major groups. On a total alkali versus silica diagram, the Haughton Hill, Kokonga, Swinburn South and Waipiata samples are mostly classified as basalt and plot close to or across the alkaline-subalkaline divide (A). They vary between silica-saturated and undersaturated (B) and these rocks are herein referred to as the transitional group. On the other hand, the Swinburn North, Tuneheketake and the three dike samples are mostly basanites and fall within the alkaline field (A). They are moderately to strongly silica-undersaturated (3.8 to 21.8 wt% normative nepheline) and are herein referred to as the alkaline group. Taieri River plots between the alkaline and transitional groups; it is basalt (A) and only weakly alkaline (B). Mg numbers (Mg#, where Mg# = 100*Fe2+/(Fe2++Mg)) of the groups range between 44 and 65 with no correlation with alkali content, other than the alkaline group having a very weak negative correlation (B).
Figure 8. A, Total alkalis versus silica diagram (Le Bas et al. Citation1986) showing the classification of the different magmatic rocks in the Maniototo Basin as basalt or basanite. The grey field is other Dunedin Volcanic Group rocks, taken from Scott et al. (Citation2020a). B, Mg# versus normative nepheline illustrating the degree of alkalinity of the Maniototo Basin components.
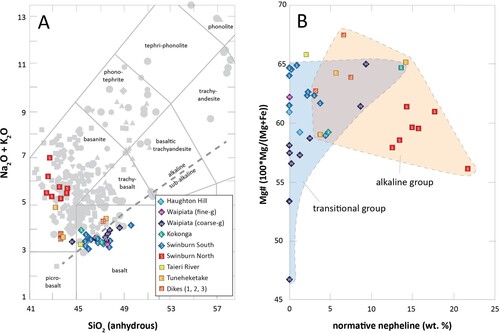
In terms of major elements, the filtered alkaline group tends to have lower SiO2 and Al2O3 (A) but higher Na2O (Swinburn North only) and K2O (B), P2O5 (not shown), TiO2 (C) than the filtered transitional group (). The two groups have very similar MgO except in samples above ∼47 wt% SiO2 (D). Taieri River is anomalous in that it has the high SiO2 content mostly seen in the transitional group (A), but a K2O content equivalent to the alkaline group, as well as lower Na2O than most other samples (B). One dike and one Tuneheketake sample also have major element compositions more similar to the transitional group than the alkaline group; however, their trace element abundance and isotopic ratios (discussed below) indicate that they belong to the alkaline group. Within the transitional group, the Swinburn South rocks are slightly anomalous because they have lower TiO2 (C) and slightly higher MgO (D) than the other rocks. The Light Rare Earth Element (REE) to Heavy REE concentrations and ratios (La/Yb) show the alkaline group samples to have steeper REE concentrations than the transitional group (E). The Sr concentrations of the alkaline group are also higher than those of the transitional group (F), except that several of the Swinburn South rocks have distinctly high and anomalous Sr abundances ().
Figure 9. Diagrams showing relationships between the different magmatic groups and trends in the elements and element ratios.
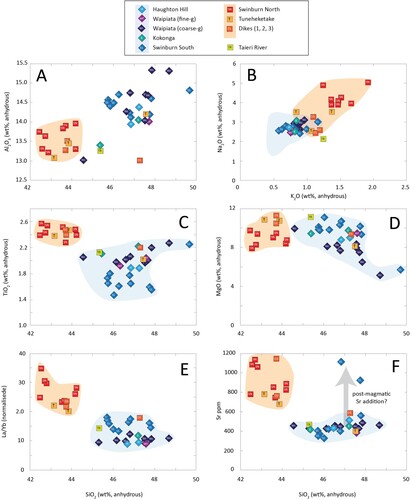
A trace element normalized diagram of all analyses shows that the transitional group is relatively depleted compared to the alkaline group, with Taieri River plotting at intermediate values (). Transitional group rocks exhibit small Ti* ( = TiN/(EuN x GdN)^0.5, where N = primitive mantle-normalised) of 0.9 to 1.1 compared to the alkaline group (0.6 to 0.9). The trend is similar in K*( = KN/(NbN x LaN)^0.5): the transitional group (K* = 0.7 to 1.1) has a smaller negative anomaly compared to the alkaline group (0.3 to 0.6), with the exception of Swinburn South (K* of 0.4 to 0.8, median 0.6). This widespread negative K* anomaly cannot be a function of alteration, even though K may be slightly affected by this process (B), because the anomaly is pervasive. Similarly, the positive P anomaly () is also pervasive. ZirconiumN/HfN is also slightly lower in the transitional rocks (1.03 to 1.14) compared to the alkaline group (1.15 to 1.31), once again excluding Swinburn South (1.03 to 1.17). Taieri River is has intermediate ratios (ZrN/HfN = 1.10 to 1.19).
Figure 10. Primitive mantle-normalised data illustrating the trace element differences and similarities between the different Maniototo Basin volcanic rocks. Data are from this study and also Scott et al. (Citation2020a).
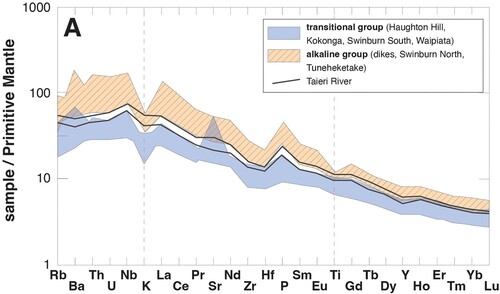
Sr-Nd-Pb isotopes
New Sr-Nd-Pb isotopic analyses are presented for 21 Maniototo Basin volcanic rocks to aid distinction of the magmas and characterisation of their sources. In addition, we incorporate published data from two Haughton Hill samples, four Swinburn South, three Swinburn North, one Waipiata and one Kokonga sample that were reported by Scott et al. (Citation2020a) (). The 87Sr/86Sr and 143Nd/144Nd data are all corrected to 11 Ma based on the interpretation of existing K-Ar and new 40Ar/39Ar data, although in detail this makes little difference to the results because the age correction from measured values is commonly less than 2 standard errors on the measurements. All Pb data are uncorrected due to a lack of sufficient quality Pb, U and Th concentration data.
Table 3. Sr-Nd-Pb isotopic data for the studied samples. Several data are from Scott et al. (Citation2020a).
The transitional group (Haughton Hill, Waipiata, Swinburn South and Kokonga) range from 87Sr/86Sr11 Ma of 0.70309 to 0.70554 (). The Waipiata fine-grained and coarse-grained flows are isotopically indistinguishable and are very similar to Kokonga, whereas Haughton Hill and Swinburn South extend to significantly more radiogenic Sr values (A). However, as a test for whether the bulk rock Sr in those radiogenic samples could be due to post-magmatic alteration, the compositions of magmatic feldspars and post-magmatic carbonate in the two Haughton Hill rocks were analysed via in-situ laser ablation. These data show the feldspars to have 87Sr/86Sr values of 0.70326 ± 0.00016 and the post-magmatic carbonates to have 87Sr/86Sr of 0.70785 ± 0.00016 (). Similarly, the most radiogenic Swinburn South sample also has the highest Sr content (921 ppm compared to the average of 388 ppm for the other three isotopically analysed samples) (F) and contains post-magmatic carbonate. The measured 87Sr/86Sr data for the alkaline rocks (Taieri River, Tuneheketake, Swinburn North and the three dikes) range from 0.70293 to 0.70425, and are age-corrected to 0.70291 to 0.70424 (). Dike 3 has anomalously high 87Sr/86Sr and Sr (1140 ppm. F) compared to the other alkaline samples (87Sr/86Sr ≤ 0.70317, Sr ≤ 755 ppm).
Figure 11. A, Age-corrected 143Nd/144Nd data versus 87Sr/86Sr data, plotted against the larger Dunedin Volcanic Group, which has two clusters in Pb isotopes. In-situ 87Sr/86Sr data for Haughton Hill feldspar (n = 14) are shown with a single symbol that is larger than the errors, whereas the Haughton Hill carbonate data (0.7078-0.7079; ) plot off the x-axis to the right. B, Age corrected 207Pb/204Pb and 206Pb/204Pb illustrate the broad differences between the different volcanic rocks. Data in the figures are from this study and the compiled data in Scott et al. (Citation2020a) and from Pontesilli et al. (Citation2021).
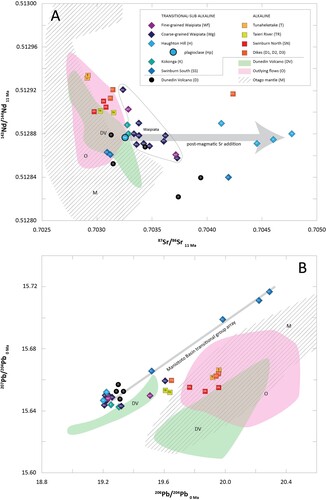
Table 4. In-situ 87Sr-86Sr analyses of feldspar and carbonate in the Haughton Hill flow.
The 143Nd/144Nd data generally distinguish the two major groups (A, ). The transitional group has a 143Nd/144Nd spread of 0.51286 to 0.51292 (ϵNd11 Ma = +3.9 to +5.5, median of +4.7), which tends to be slightly less radiogenic than most of the alkaline rocks (0.51290 to 0.51293; ϵNd11 Ma = +5.1 to +5.8, median of +5.4). The Taieri River rocks plot at the intersection of both groups.
Like Nd, the Pb isotopic data largely subdivide the two major groups (B, ). The alkaline group has 206Pb/204Pb of 19.64 to 19.96 and 208Pb/204Pb of 39.30 to 39.52, whereas the transitional group has 206Pb/204Pb of 19.21 to 19.61 and 208Pb/204Pb of 38.99 to 39.27. Taieri River once again has values at the intersection of the two groups. All 207Pb/204Pb data plot between 15.64 to 15.67 and do not distinguish the two suites (B), except for Swinburn South data, which plot on an array of elevated 207Pb/204Pb for a given 206Pb/204Pb or 208Pb/204Pb value. This array intercepts the other transitional-subalkaline magmas in the Maniototo Basin.
Discussion
Crystal fractionation and crustal contamination
Intraplate monogenetic basalts are often near-primary magmas and therefore can provide insight into mantle source regions (e.g. McGee and Smith Citation2016). However, prior to investigating any source associations, it is necessary to evaluate potential modification by crystal fractionation or crustal assimilation. Although the studied transitional rocks have MgO contents that range from 5 to 11 wt%, most of the rocks cluster between ∼8 and 11% (D). The only systematic variation in MgO with increasing SiO2 occurs within the Waipiata (D) and may be caused by a combination of alteration, crystal fractionation and/or samples with different crystal abundances. The alkaline group also ranges from 8-11 wt% MgO and there is no obvious trend.
Thorium and Nb ratios are often used as guide for crustal assimilation because the crust is high in Th (e.g. Hofmann et al. Citation1986). All Nb-Th-U data from the volcanic rocks in the Maniototo Basin fall between depleted mantle (Nb/Th = 14 vs Nb/U = 50) and primitive mantle (Nb/Th = 8 vs Nb/U = 30) (Sun and McDonough Citation1989; McDonough and Sun Citation1995) (A). The lowest values of Nb/Th and Nb/U occur in the transitional lavas. Assuming a depleted mantle source, the variation on display could result from up to ∼1% crustal contamination (Nb/Th has no relationship to LOI); however, given the heterogeneity in other chemical parameters, especially the isotopic data, it is more likely that the range observed is controlled by heterogeneity within the mantle source. The combination of high Nb/Th and Nb/U, as well as a lack of systematic relationship between Ba/Nb and MgO (B), suggests there has been little if any crustal contamination. Thus, we interpret that most of the rocks retain the trace element and isotopic character of their parental source-derived magmas. This interpretation has the important implication that the isotopic range displayed should also therefore represent the mantle sources, with the exception of the elevated Sr due to post-magmatic alteration. The isotopic distinction between the alkaline group and transitional group (B) also means that these magmas cannot be caused by simply different degrees of melting of the same source.
Mantle sources for maniototo basin volcanic rocks
The Zealandia sub-continental lithospheric mantle has experienced varying levels of re-enrichment due to metasomatism, most obviously manifested through wide-scale isotopic and chemical changes in mantle peridotite xenolith clinopyroxene compositions via reaction with carbonatitic melts (e.g. Scott et al. Citation2014a, Citation2014b; McCoy-West et al. Citation2015, Citation2016; Scott et al. Citation2016a, b; Dalton et al. Citation2017; van der Meer et al. Citation2019) or the formation of pyroxenites via reaction with silicate melts (Bonnington et al. Citation2023). Ti/Eu may be used to investigate the contribution of a carbonatite-metasomatized component to a magma because carbonatites carry abundant REE but little Ti (e.g. Klemme et al. Citation1995) and the negative correlation between Ti/Eu and La/Yb (C) indicates that the strongest ‘carbonatitic’ signature occurs in the alkaline group. However, this interpretation is complicated if phlogopite and amphibole, both Ti-rich phases, were residual in the mantle sources – as will be outlined below.
Metasomatic process may form veins of hydrous minerals within the lithospheric mantle. These veins usually contain amphibole and/or phlogopite (e.g. Foley Citation1992). Amphibole and phlogopite have been found in peridotite – albeit extremely sparsely – across Otago (e.g. Klemme Citation2004; Scott et al. Citation2014b). The occurrence of these minerals is significant because, assuming typical F-free compositions, they are stable at temperature and pressure conditions only within the lithosphere. Phlogopite exists up to ∼1300°C at < 5 GPa (Sato et al. Citation1997), while F-free pargasitic amphibole, which is more common than K-richterite in mantle xenoliths in alkaline basalts, is stable to ∼1100°C and 2.5 Gpa (e.g. Foley Citation1992; Green et al. Citation2014; Pilet et al. Citation2008). Amphibole and phlogopite can act as residual phases during partial melting, with these phases manifest as a primitive mantle-normalised negative K* and Ti* anomalies (e.g. LaTourrette et al. Citation1995; Späth et al. Citation2001; Panter et al. Citation2006). Alkaline basalt trace element compositions have been experimentally created through the melting of amphibole-rich metasomatic veins, which, when reacted with ambient lherzolite, create the range from high alkali basanite to mid alkaline basalt (e.g. Merrill and Wylie Citation1975; Pilet et al. Citation2008). Many of Maniototo volcanic rocks show a negative K*, of which the strongest ne-normative alkaline samples have the lowest K* and lowest Ti* (D). The transitional samples subtly show this trend, except for Swinburn South, which shows no variation in Ti* with decreasing K*. These relationship suggest that residual amphibole and/or phlogopite may be controlling these anomalies, with a greater residual volume in the alkaline rocks. Swinburn South evidently had a source with residual mineralogy that retained K but not Ti.
The High Field Strength Elements (HFSE; Nb, Ta, Zr, Hf) are often used to investigate processes of partial melting involving variably metasomatized peridotite. Nb and Ta are preferentially incorporated in Ti-bearing oxide minerals, while major silicate phases usually contain only small abundances and have partition coefficients < 1 for both elements (e.g. Green Citation1995; Kalfoun et al. Citation2002). Despite their overall incompatibility, mantle lithosphere minerals such as amphibole and phlogopite can also incorporate considerable Nb and Ta (Ionov and Hofmann Citation1995; Pfänder et al. Citation2007). Zr and Hf tend to have greater compatibility in silicate phases compared to Nb and Ta (Green et al. Citation2000, Pfänder et al. Citation2007, Tiepolo et al. Citation2001). When considering the major metasomatic mineral phases (amphibole, phlogopite, clinopyroxene), there are some distinctions in partitioning that can be employed to discriminate the influence of different minerals. Despite some uncertainty, cpx/meltDHf or Zr tend to be one order of magnitude larger than cpx/meltDNb or Ta, phlog/meltDHf or Ta tend to be one order of magnitude larger than phlog/meltDNb or Zr, whereas amph/meltD HFSE are all within similar magnitude (Green et al Citation2000, Pfänder et al. Citation2007, Tiepolo et al. Citation2001). Within the more primitive Maniototo rocks, the transitional group has mostly lower Zr/Hf (37-42.5) compared to the alkaline group (42-48) (E). Primitive mantle has relatively low Zr/Hf (26-37; Sun and McDonough Citation1989; McDonough and Sun Citation1995) and metasomatically modified mantle commonly has higher Zr/Hf as a result of the fluid chemistries (e.g. Bizimis et al. Citation2003). Considering the respective D values, phlogopite would be an effective residual metasomatic phase to fractionate Zr with respect to Hf, hence affecting their ratio. In this case the high-Zr/Hf alkaline rocks appear to have a greater residual phlogopite influence. In contrast, there is only small variation in Nb/Ta (13-19) in the Maniototo Basin basalts and overall the range is similar for both alkaline and transitional groups. The data are similar to the average of OIB (Pfänder et al. Citation2007), although it is clear that Swinburn South and Swinburn North extend to slightly lower values than Tuneheketake and the dikes. A greater distinction exists in Zr/Ta (or likewise Hf/Nb) (E) and either residual clinopyroxene or amphibole would be the most effective phase in fractionating Zr (and Hf) with respect to Ta (and Nb). Again, the alkaline group having lower Zr/Ta suggests a role for residual metasomatic mantle minerals during magma generation or modification processes. Swinburn South is distinct as it has both low Zr/Hf and Zr/Ta (E) in addition to the anomalous K* but not Ti* outlined above. Although the latter characteristics might indicate a phlogopite influence, the relatively low Zr/Ta and Zr/Hf points to a lack thereof. Alternatively, phlogopite may occur in conjunction with metasomatic amphibole and clinopyroxene that would complicate/reverse chemical signatures. Overall, however, on the basis of their trace element chemistry, the Maniototo Basin alkaline rocks were therefore likely derived from, or interacted with, a mantle that contained a greater metasomatic component than the Maniototo Basin transitional lavas.
Zones of amphibole and/or phlogopite-rich mantle are known as metasomes (Rooney et al. Citation2017) and these have been proposed as possible melt sources or components in the formation of alkaline lavas both within the Dunedin Volcanic Group (e.g. Price et al. Citation2003; Sprung et al. Citation2007; Scott et al. Citation2020a; Pontesilli et al. Citation2021). However, the combination of the difference in trace element anomalies between alkaline and transitional lavas (e.g. negative Zr-Hf anomaly) and the systematic isotopic placement of the Maniototo Basin transitional group largely outside the known Otago lithospheric mantle range (A and B) suggests that there is a source component that is only tapped or clearly represented in the latter melts. Pontesilli et al (Citation2021) proposed that the range of high to low alkaline basalts of Dunedin Volcano could have been generated by asthenospheric melts (low-alkaline) interacting with lithospheric metasomes, with the result being transformation towards high-alkaline magma (e.g. Foley Citation1992; Rooney et al. Citation2017). In this case, the higher 87Sr/86Sr and lower 143Nd/144Nd in the low-alkaline rocks of the Maniototo Basin would likely be from components within the convecting mantle, with this composition diluted and overprinted depending on the volume of reaction with the lithospheric mantle metasomes to give rise to increasingly alkaline rocks (e.g. Pontesilli et al. (Citation2021)). The origin of the intermediate composition Taieri River flow remains unclear; however, an alkaline melt reacting with peridotite would raise the SiO2 via orthopyroxene dissolution (Pilet et al. Citation2008) yet likely retain the alkaline isotopic ratios.
Conclusions
A range of alkaline and subalkaline Miocene-erupted volcanic rocks occur in the Maniototo Basin in the northwest of the Dunedin Volcanic Group. We present the first comprehensive description of their occurrences, mineralogy, geochemistry and Sr-Nd-Pb isotopes as well as two 40Ar/39Ar dates. None of the rocks show significant crustal contamination, although some show post-magmatic modification of Sr isotope compositions and there is evidence of some post-depositional alteration. The alkaline magmas are trace element enriched compared to the transitional (alkaline to subalkaline) group and also have larger Ti and K negative anomalies indicative of residual amphibole and/or phlogopite in one of the mantle sources. The transitional group of magmas show less effect of amphibole and phlogopite. Since these two hydrous phases are present only in the lithosphere (unless as high-T K-richterite), an explanation for the variation in the Maniototo Basin volcanic rocks is that the melts represent different degrees of mantle lithospheric modification as magmas ascended, or the melts were derived from different mantle sources. In either case, the variability appears to be due to mantle-related processes and heterogeneity.
Acknowledgments
Field and analytical costs of the MSc of Wilson and the PhD of Giacalone were covered by the University of Otago Geology department. Giacalone was supported by an Otago Doctoral Scholarship. We thank all the landowners for permission to cross their land. Two reviewers (anonymous) and the editor (H. Dalton) are thanked for their comments.
Disclosure statement
No potential conflict of interest was reported by the author(s).
Data availability statement
All geochemical and geochronological data used in this paper are openly available in the text or in the supplemental files deposited in figshare at https://doi.org/10.6084/m9.figshare.24427378.v2.
References
- Baker JA, Gamble JA, Graham IJ. 1994. The age, geology, and geochemistry of the Tapuaenuku igneous complex, Marlborough, New Zealand. New Zealand Journal of Geology and Geophysics. 37:249–268.
- Bas ML, Maitre RL, Streckeisen A, Zanettin B, IUGS Subcommission on the Systematics of Igneous Rocks. 1986. A chemical classification of volcanic rocks based on the total alkali-silica diagram. Journal of Petrology. 27(3):745–750.
- Baxter RJ, White JD, Brenna M, Ohneiser C. 2022. Pre-eruption magma staging at the long-lived intraplate Dunedin Volcano, New Zealand. Terra Nova. 34:253–263.
- Benson WN. 1941. The basic igneous rocks of eastern Otago and their tectonic environment, Part 1. Transactions of the Royal Society of New Zealand. 71:208–222.
- Benson WN. 1942. The basic igneous rocks of eastern Otago and their tectonic environment, Part II. Transactions of the Royal Society of New Zealand. 72:85–118.
- Bishop DG. 1974. Tertiary and early Quaternary geology of the Naseby and Kyeburn areas, Central Otago. New Zealand Journal of Geology and Geophysics. 17:19–39.
- Bizimis M, Salters VJ, Dawson JB. 2003. The brevity of carbonatite sources in the mantle: evidence from Hf isotopes. Contributions to Mineralogy and Petrology. 145:281–300.
- Bonnington SJ, Scott JM, Palmer MC, Cooper NP, Reid MR, Stirling CH. 2023. Composition and Miocene deformation of the lithospheric mantle adjacent to the Marlborough Fault System in North Canterbury. New Zealand Journal of Geology and Geophysics. 1–17.
- Brenna M, Cronin SJ, Smith IE, Sohn YK, Maas R. 2012. Spatio-temporal evolution of a dispersed magmatic system and its implications for volcano growth, Jeju Island Volcanic Field, Korea. Lithos. 148:337–352.
- Brenna M, Ubide T, Nichols AR, Mollo S, Pontesilli A. 2021. Anatomy of intraplate monogenetic alkaline basaltic magmatism: clues from magma, crystals, and glass. In: Crustal magmatic system evolution: anatomy, architecture, and physico-chemical processes; p. 79–103.
- Burgin DL, Scott JM, le Roux PJ, Howarth G, Palmer MC, Czertowicz TA, Negrini M, Reid MR, Stirling CH. 2023. Rapid characterisation of Mars’ mantle reservoirs by in situ laser ablation 87Sr/86Sr analysis of shocked feldspar (maskelynite). Geochimica et Cosmochimica Acta. 341:46–61.
- Cook C, Briggs RM, Smith IE, Maas R. 2005. Petrology and geochemistry of intraplate basalts in the South Auckland volcanic field, New Zealand: evidence for two coeval magma suites from distinct sources. Journal of Petrology. 46:473–503.
- Coombs DS, Adams CJ, Roser BP, Reay A. 2008. Geochronology and geochemistry of the Dunedin Volcanic Group, eastern Otago, New Zealand. New Zealand Journal of Geology and Geophysics. 51:195–218.
- Coombs DS, Cas RA, Kawachi Y, Landis CA, McDonough WF, Reay A. 1986. Cenozoic volcanism in north, east and central Otago. Royal Society of New Zealand Bulletin. 23:278–312.
- Coombs DS, White AJR, Hamilton D, Couper RA. 1960. Age relations of the Dunedin volcanic complex and some paleogeographic implications–Part II. New Zealand Journal of Geology and Geophysics. 3:572–579.
- Coombs DS, Wilkinson JFG. 1969. Lineages and fractionation trends in undersaturated volcanic rocks from the East Otago volcanic province (New Zealand) and related rocks. Journal of Petrology. 10:440–501.
- Cooper AF. 1986. A carbonatitic lamprophyre dike swarm from the Southern Alps, Otago and Westland. Late Cenozoic volcanism in New Zealand. Royal Society of New Zealand bulletin. 23:313–336.
- Cooper AF. 2020. Petrology and petrogenesis of an intraplate alkaline lamprophyre-phonolite-carbonatite association in the Alpine Dyke Swarm, New Zealand. New Zealand Journal of Geology and Geophysics. 63:469–488.
- Craw D, Craw L, Burridge CP, Wallis GP, Waters JM. 2016. Evolution of the Taieri River catchment, East Otago, New Zealand. New Zealand Journal of Geology and Geophysics. 59:257–273.
- Dalrymple GB, Clague DA, Garcia MO, Bright SW. 1981. Petrology and K-Ar ages of dredged samples from Laysan Island and Northampton Bank volcanoes, Hawaiian ridge, and evolution of the Hawaiian-Emperor chain. Geological Society of America Bulletin. 92(6_Part_II):884–933.
- Dalton HB, Scott JM, Liu J, Waight TE, Pearson DG, Brenna M, le Roux PJ, Palin JM. 2017. Diffusion-zoned pyroxenes in an isotopically heterogeneous mantle lithosphere beneath the Dunedin Volcanic Group, New Zealand, and their implications for intraplate alkaline magma sources. Lithosphere. 9:463–475.
- Dasgupta R, Jackson MG, Lee CTA. 2010. Major element chemistry of ocean island basalts—conditions of mantle melting and heterogeneity of mantle source. Earth and Planetary Science Letters. 289:377–392.
- Donnelly KE. 1996. The Waipiata volcanic formation [MSc thesis]. University of Otago.
- Finn CA, Müller RD, Panter KS. 2005. A Cenozoic diffuse alkaline magmatic province (DAMP) in the southwest Pacific without rift or plume origin. Geochemistry, Geophysics, Geosystems. 6.
- Foley S. 1992. Vein-plus-wall-rock melting mechanisms in the lithosphere and the origin of potassic alkaline magmas. Lithos. 28:435–453.
- Fox BR, Wartho J, Wilson GS, Lee DE, Nelson FE, Kaulfuss U. 2015. Long-term evolution of an Oligocene/Miocene maar lake from Otago, New Zealand. Geochemistry, Geophysics, Geosystems. 16:59–76.
- Galer SJG, Abouchami W. 1998. Practical application of lead triple spiking for correction of instrumental mass discrimination. Mineralogical Magazine. 62:491–492.
- Gamble JA, Adams CJ, Morris PA, Wysoczanski RJ, Handler M, Timm C. 2018. The geochemistry and petrogenesis of Carnley Volcano, Auckland Islands, SW Pacific. New Zealand Journal of Geology and Geophysics. 61:480–497.
- Green DH, Hibberson WO, Rosenthal A, Kovács I, Yaxley GM, Falloon TJ, Brink F. 2014. Experimental study of the influence of water on melting and phase assemblages in the upper mantle. Journal of Petrology. 55:2067–2096.
- Green TH. 1995. Significance of Nb/Ta as an indicator of geochemical processes in the crust-mantle system. Chemical Geology. 120:347–359.
- Green TH, Blundy JD, Adam J, Yaxley GM. 2000. SIMS determination of trace element partition coefficients between garnet, clinopyroxene and hydrous basaltic liquids at 2–7.5 GPa and 1080–1200 C. Lithos. 53:165–187.
- Harris C, le Roux P, Cochrane R, Martin L, Duncan AR, Marsh JS, le Roex P, Class C. 2015. The oxygen isotope composition of Karoo and Etendeka picrites: high δ18 O mantle or crustal contamination? Contributions to Mineralogy and Petrology. 170:1–24.
- Hoernle K, White JDL, van den Bogaard P, Hauff F, Coombs DS, Werner R, Timm C, Garbe-Schönberg D, Reay A, Cooper AF. 2006. Cenozoic intraplate volcanism on New Zealand: upwelling induced by lithospheric removal. Earth and Planetary Science Letters. 248:350–367.
- Hofmann AW, Jochum KP, Seufert M, White WM. 1986. Nb and Pb in oceanic basalts: new constraints on mantle evolution. Earth and Planetary Science Letters. 79:33–45.
- Hopkins JL, Smid ER, Eccles JD, Hayes JL, Hayward BW, McGee LE, van Wijk K, Wilson TM, Cronin SJ, Leonard GS, et al. 2021. Auckland Volcanic Field magmatism, volcanism, and hazard: a review. New Zealand Journal of Geology and Geophysics. 64:213–234.
- Ionov DA, Hofmann AW. 1995. Nb/ Ta-rich mantle amphiboles and micas: implications for subduction-related metasomatic trace element fractionations. Earth and Planetary Science Letters. 131:341–356.
- Irvine TN, Baragar WRA. 1971. A guide to the chemical classification of the common volcanic rocks. Canadian Journal of Earth Sciences. 8:523–548.
- Kalfoun F, Ionov D, Merlet C. 2002. HFSE residence and Nb/Ta ratios in metasomatised, rutile-bearing mantle peridotites. Earth and Planetary Science Letters. 199:49–65.
- Kaulfuss U, Lee DE, Wartho J, Bowie E, Lindqvist JK, Conran JG, Bannister JM, Mildenhall DC, Kennedy EM, Gorman AR. 2018. Geology and palaeontology of the Hindon Maar Complex: a Miocene terrestrial fossil Lagerstätte in southern New Zealand. Palaeogeography, Palaeoclimatology, Palaeoecology. 500:52–68.
- Klemme S. 2004. Evidence for fluoride melts in Earth's mantle formed by liquid immiscibility. Geology. 32:441–444.
- Klemme SV, Van der Laan SR, Foley SF, Günther D. 1995. Experimentally determined trace and minor element partitioning between clinopyroxene and carbonatite melt under upper mantle conditions. Earth and Planetary Science Letters. 133:439–448.
- Kuiper KF, Deino A, Hilgen FJ, Krijgsman W, Renne PR, Wijbrans AJ. 2008. Synchronizing rock clocks of Earth history. Science. 320(5875):500–504.
- LaTourrette T, Hervig RL, Holloway JR. 1995. Trace element partitioning between amphibole, phlogopite, and basanite melt. Earth and Planetary Science Letters. 135:13–30.
- Lee JY, Marti K, Severinghaus JP, Kawamura K, Yoo HS, Lee JB, Kim JS. 2006. A redetermination of the isotopic abundances of atmospheric Ar. Geochimica et Cosmochimica Acta. 70(17):4507–4512.
- Mather BR, Muller RD, Seton M, Ruttor S, Nebel O, Mortimer N. 2020. Intraplate volcanism triggered by bursts in slab flux. Science Advances. 6:eabd0953.
- McCoy-West AJ, Baker JA, Faure K, Wysoczanski R. 2010. Petrogenesis and origins of mid-Cretaceous continental intraplate volcanism in Marlborough, New Zealand: implications for the long-lived HIMU magmatic mega-province of the SW Pacific. Journal of Petrology. 51:2003–2045.
- McCoy-West AJ, Bennett VC, Amelin Y. 2016. Rapid Cenozoic ingrowth of isotopic signatures simulating “HIMU” in ancient lithospheric mantle: distinguishing source from process. Geochimica et Cosmochimica Acta. 187:79–101.
- McCoy-West AJ, Bennett VC, O’Neill HSC, Hermann J, Puchtel IS. 2015. The interplay between melting, refertilization and carbonatite metasomatism in off-cratonic lithospheric mantle under Zealandia: an integrated major, trace and platinum group element study. Journal of Petrology. 56:563–604.
- McDonough WF, Sun S-S. 1995. The composition of the Earth. Chemical Geology. 120:223–253.
- McDougall I, Coombs DS. 1973. Potassium-argon ages for the Dunedin volcano and outlying volcanics. New Zealand Journal of Geology and Geophysics. 16:179–188.
- McGee LE, Smith IE. 2016. Interpreting chemical compositions of small scale basaltic systems: a review. Journal of Volcanology and Geothermal Research. 325:45–60.
- McGee LE, Smith IE, Millet MA, Handley HK, Lindsay JM. 2013. Asthenospheric control of melting processes in a monogenetic basaltic system: a case study of the Auckland Volcanic Field, New Zealand. Journal of Petrology. 54:2125–2153.
- McLeod OE, White JD. 2018. Petrogenetic links between the Dunedin Volcano and peripheral volcanics of the Karitane Suite. New Zealand Journal of Geology and Geophysics. 61(4):543–561.
- Merrill RB, Wyllie PJ. 1975. Kaersutite and kaersutite eclogite from Kakanui, New Zealand—water-excess and water-deficient melting to 30 kilobars. Geological Society of America Bulletin. 86:555–570.
- Min K, Mundil R, Renne PR, Ludwig KR. 2000. A test for systematic errors in 40Ar/39Ar geochronology through comparison with U/Pb analysis of a 1.1-Ga rhyolite. Geochimica et Cosmochimica Acta. 64(1):73–98.
- Mortimer N. 2000. Metamorphic discontinuities in orogenic belts: example of the garnet–biotite–albite zone in the Otago Schist, New Zealand. International Journal of Earth Sciences. 89:295–306.
- Mortimer N, Scott JM. 2020. Volcanoes of Zealandia and the southwest Pacific. New Zealand Journal of Geology and Geophysics. 63:371–377.
- Németh K, White JD. 2003. Reconstructing eruption processes of a Miocene monogenetic volcanic field from vent remnants: Waipiata Volcanic Field, South Island, New Zealand. Journal of Volcanology and Geothermal Research. 124:1–21.
- Neymark LA, Premo WR, Melnikov NN, Emsbo P. 2014. Precise determination of δ 88 Sr in rocks, minerals, and waters by double-spike TIMS: a powerful tool in the study of geological, hydrological and biological processes. Journal of Analytical Atomic Spectrometry. 29:65–75.
- Panter KS, Blusztajn J, Hart SR, Kyle PR, Esser R, McIntosh WC. 2006. The origin of HIMU in the SW Pacific: evidence from intraplate volcanism in southern New Zealand and subantarctic islands. Journal of Petrology. 47:1673–1704.
- Pfänder JA, Münker C, Stracke A, Mezger K. 2007. Nb/Ta and Zr/Hf in ocean island basalts—implications for crust–mantle differentiation and the fate of Niobium. Earth and Planetary Science Letters. 254:158–172.
- Pilet S, Baker MB, Stolper EM. 2008. Metasomatized lithosphere and the origin of alkaline lavas. Science. 320:916–919.
- Pin C, Gannoun A, Dupont A. 2014. Rapid, simultaneous separation of Sr, Pb, and Nd by extraction chromatography prior to isotope ratios determination by TIMS and MC-ICP-MS. Journal of Analytical Atomic Spectrometry. 29:1858–1870.
- Pontesilli A, Brenna M, Mollo S, Masotta M, Nazzari M, le Roux P, Scarlato P. 2022. Trachyte-phonolite transition at Dunedin Volcano: fingerprints of magma plumbing system maturity and mush evolution. Lithos. 408:106545.
- Pontesilli A, Brenna M, Ubide T, Mollo S, Masotta M, Caulfield J, Le Roux P, Nazzari M, Scott JM, Scarlato P. 2021. Intraplate basalt alkalinity modulated by a lithospheric mantle filter at the Dunedin Volcano (New Zealand). Journal of Petrology. 62:egab062.
- Price RC, Chappell BW. 1975. Fractional crystallisation and the petrology of Dunedin Volcano. Contributions to Mineralogy and Petrology. 53:157–182.
- Price RC, Compston W. 1973. The geochemistry of the Dunedin Volcano: strontium isotope chemistry. Contributions to Mineralogy and Petrology. 42:55–61.
- Price RC, Cooper AF, Woodhead JD, Cartwright I. 2003. Phonolitic diatremes within the Dunedin Volcano, South Island, New Zealand. Journal of Petrology. 44:2053–2080.
- Price RC, Taylor SR. 1973. The geochemistry of the Dunedin volcano, east Otago, New Zealand: rare earth elements. Contributions to Mineralogy and Petrology. 40:195–205.
- Reay A, McIntosh PE, Gibson IL. 1991. Lherzolite xenolith bearing flows from the east Otago province: crystal fractionation of upper mantle magmas. New Zealand Journal of Geology and Geophysics. 34:317–327.
- Rooney TO, Nelson WR, Ayalew D, Hanan B, Yirgu G, Kappelman J. 2017. Melting the lithosphere: metasomes as a source for mantle-derived magmas. Earth and Planetary Science Letters. 461:105–118.
- Sato K, Katsura T, Ito E. 1997. Phase relations of natural phlogopite with and without enstatite up to 8 GPa: implication for mantle metasomatism. Earth and Planetary Science Letters. 146:511–526.
- Scanlan EJ, Scott JM, le Roux PJ. 2020. Pyrometamorphosed Otago Schist xenoliths cause minor contamination of Dunedin Volcanic Group basanite. New Zealand Journal of Geology and Geophysics. 63:530–546.
- Scanlan EJ, Scott JM, Wilson VJ, Stirling CH, Reid MR, Le Roux PJ. 2018. In situ 87Sr/86Sr of scheelite and calcite reveals proximal and distal fluid-rock interaction during orogenic W-Au mineralization, Otago Schist, New Zealand. Economic Geology. 113(7):1571–1586.
- Scott JM, Brenna M, Crase JA, Waight TE, van der Meer QHA, Cooper AF, Palin JM, le Roux PJ, Münker C. 2016a. Peridotitic lithosphere metasomatized by volatile-bearing melts, and its association with intraplate alkaline HIMU-like magmatism. Journal of Petrology. 57:2053–2078.
- Scott JM, Cooper AF, Craw D, le Roux PJ, Dalton HB, Palmer MC. 2023. Basanite cobbles in Pleistocene sediments in Central Otago and their implications for intraplate volcanism and Clutha River paleo-drainage. New Zealand Journal of Geology and Geophysics. 1–16.
- Scott JM, Hodgkinson A, Palin JM, Waight TE, van der Meer QHA, Cooper AF. 2014a. Ancient melt depletion overprinted by young carbonatitic metasomatism in the New Zealand lithospheric mantle. Contributions to Mineralogy and Petrology. 167:963.
- Scott JM, Liu J, Pearson DG, Waight TE. 2016. Mantle depletion and metasomatism recorded in orthopyroxene in highly depleted peridotites. Chemical Geology. 441:280–291. doi:10.1016/j.chemgeo.2016.08.024.
- Scott JM, Pontesilli A, Brenna M, White JDL, Giacalone E, Palin JM, le Roux PJ. 2020a. The Dunedin Volcanic Group and a revised model for Zealandia's alkaline intraplate volcanism. New Zealand Journal of Geology and Geophysics. 63:510–529.
- Scott JM, Turnbull IM. 2018. Geology of New Zealand’s Sub-Antarctic Islands. New Zealand Journal of Geology and Geophysics. 62:291–317.
- Scott JM, Turnbull IM, Auer A, Palin JM. 2013. The sub-Antarctic Antipodes Volcano: a < 0.5 Ma HIMU-like Surtseyan volcanic outpost on the edge of the Campbell Plateau, New Zealand. New Zealand Journal of Geology and Geophysics. 56:134–153.
- Scott JM, Waight TE, van der Meer QHA, Palin JM, Cooper AF, Münker C. 2014b. Metasomatized ancient lithospheric mantle beneath the young Zealandia microcontinent and its role in HIMU-like intraplate magmatism. Geochemistry, Geophysics, Geosystems. 15:3477–3501.
- Scott JM, White JD, le Roux PJ. 2020b. Intraplate volcanism on the Zealandia Eocene-Early Oligocene continental shelf: the Waiareka-Deborah Volcanic Field, North Otago. New Zealand Journal of Geology and Geophysics. 63:450–468.
- Smith IE, Brenna M, Cronin SJ. 2021. The magma source of small-scale intraplate monogenetic volcanic systems in northern New Zealand. Journal of Volcanology and Geothermal Research. 418:107326.
- Smith IE, Cronin SJ. 2021. Geochemical patterns of late Cenozoic intraplate basaltic volcanism in northern New Zealand and their relationship to the behaviour of the mantle. New Zealand Journal of Geology and Geophysics. 64:201–212.
- Späth A, Le Roex AP, Opiyo-Akech N. 2001. Plume–lithosphere interaction and the origin of continental rift-related alkaline volcanism—the Chyulu Hills volcanic province, southern Kenya. Journal of Petrology. 42:765–787.
- Sprung P, Schuth S, Münker C, Hoke L. 2007. Intraplate volcanism in New Zealand: the role of fossil plume material and variable lithospheric properties. Contributions to Mineralogy and Petrology. 153:669–687.
- Sun S-S, McDonough WF. 1989. Chemical and isotopic systematics of oceanic basalts: implications for mantle composition and processes. Geological Society, London, Special Publications. 42:313–345.
- Tanaka T, Togashi S, Kamioka H, Amakawa H, Kagami H, Hamamoto T, Yuhara M, Orihasi Y, Yoneda S, Shimizu, H, et al. 2000. JNdi-1: a neodymium isotopic reference in consistency with LaJolla neodymium. Chemical Geology. 168:279–281.
- Tiepolo M, Bottazzi P, Foley SF, Oberti R, Vannucci R, Zanetti A. 2001. Fractionation of Nb and Ta from Zr and Hf at mantle depths: the role of titanian pargasite and kaersutite. Journal of Petrology. 42(1):221–232.
- Timm C, Hoernle K, Van Den Bogaard P, Bindeman I, Weaver S. 2009. Geochemical evolution of intraplate volcanism at Banks Peninsula, New Zealand: interaction between asthenospheric and lithospheric melts. Journal of Petrology. 50:989–1023.
- Timm C, Hoernle K, Werner R, Hauff F, van den Bogaard P, White J, Mortimer N, Garbe-Schönberg D. 2010. Temporal and geochemical evolution of the Cenozoic intraplate volcanism of Zealandia. Earth-Science Reviews. 98:38–64.
- van der Meer QHA, Scott JM, Serre SH, Whitehouse MJ, Kristoffersen M, le Roux PJ, Pope EC. 2019. Low-δ18O zircon xenocrysts in alkaline basalts; a window into the complex carbonatite-metasomatic history of the Zealandia lithospheric mantle. Geochimica et Cosmochimica Acta. 254:21–39.
- van der Meer QHA, Scott JM, Waight TE, Sudo M, Scherstén A, Cooper AF, Spell TL. 2013. Magmatism during Gondwana break-up: new geochronological data from Westland, New Zealand. New Zealand Journal of Geology and Geophysics. 56:229–242.
- van der Meer QHA, Storey M, Scott JM, Waight TE. 2016. Abrupt spatial and geochemical changes in lamprophyre magmatism related to Gondwana fragmentation prior, during and after opening of the Tasman Sea. Gondwana Research. 36:142–156.
- van der Meer QHA, Waight TE, Scott JM, Münker C. 2017. Variable sources for Cretaceous to recent HIMU and HIMU-like intraplate magmatism in New Zealand. Earth and Planetary Science Letters. 469:27–41.
- Weis D, Kieffer B, Maerschalk C, Barling J, De Jong J, Williams GA, Hanano D, Pretorius W, Mattielli N, Scoates JS, et al. 2006. High-precision isotopic characterization of USGS reference materials by TIMS and MC-ICP-MS. Geochemistry, Geophysics, Geosystems. 7(8).
- Youngson JH, Craw D, Landis CA, Schmitt KR. 1998. Redefinition and interpretation of late Miocene-Pleistocene terrestrial stratigraphy, central Otago, New Zealand. New Zealand Journal of Geology and Geophysics. 41:51–68.