Abstract
Aquaculture, like terrestrial farming, cannot achieve economic and sustainable production without high performing genetic stocks tailored to the conditions under which they are grown. It is essential, therefore, that aquaculture investment includes genetics and biotechnology to adapt marine livestock to the novel conditions of intensive aquaculture and to the demanding markets into which they are sold. The return on investment in well-structured breeding programmes can be very high, and significant performance and economic gains have been demonstrated in multiple species. Many factors must be considered in designing a genetic improvement programme, including the reproductive biology of the species and the identification of realistic and commercially relevant breeding goals based on the resources and facilities available. This paper reviews the options available to aquaculturists and provides examples of how these are being applied to six aquaculture species in New Zealand: king salmon, hāpuku, kingfish, GreenshellTM mussels, Pacific oysters and pāua (abalone).
Introduction and background
Aquaculture is the fastest growing sector of agricultural production in New Zealand, and farmed pāua (Haliotis iris), GreenshellTM mussels (Perna calaniculus), Pacific oysters (Crassostrea gigas) and king salmon (Oncorhynchus tshawytscha) currently produce over $400 million in revenue (aquaculture.org.nz). Furthermore, the aquaculture industry has set an ambitious target to increase these revenues to $1 billion by the year 2025 through a combination of expanding production of these species and adding emerging high-value species such as kingfish, hāpuku and geoduck clams to the list of farmed species.
Genetic improvement programmes in aquaculture exist to enhance farmer profits in the short, medium and long term by maximising genetic gain per year while preserving the potential for future genetic gain. Gjedrem (Citation2005) estimated that less than 5% of world aquaculture production comes from scientifically managed breeding programmes and much of this is from salmonids in northern Europe and North and South America. Given the heterogeneity of wild stocks, and the potential homogeneity of captive stocks, there is significant opportunity to identify and select for traits in newly domesticated species with high economic impact. The economic value of traits to be selected varies over time, by species and market need. Rapid growth is of pre-eminent commercial importance in labour and capital intensive production systems such as land-based pāua farms. Feed use efficiency is very important in salmon farming where feed constitutes 44%–50% of the costs of production in conventional marine farms (Norwegian and Chilean averages, based on an economic feed conversion ratio of 1:1.17, Salmon Farming Industry Handbook 2012, Marine Harvest Citation2012). In bivalve farming, where food availability is impossible to control or supplement, food use efficiency may be a major determinant of local carrying capacity and thus have high economic value.
To be sustainable and profitable, marine farmers require not only a cost-effective production system and a reliable source of juveniles or ‘seed’ to stock it, but also strains or breeds that perform well under commercial culture conditions. It is essential, therefore, that initial investment includes genetics and biotechnology to adapt marine livestock to the novel conditions of intensive aquaculture and to the demanding markets into which they are sold. The return on investment (ROI) in well-structured breeding programmes that achieve these objectives can be very high (Gjedrem Citation2005), but many factors must be considered in designing a genetic improvement programme, and a poorly designed and implemented programme can fall far short of expectations.
Domestication and genetic improvement are impossible without sufficient control over the species' mating system, and pointless if genetically improved animals cannot be produced in sufficient quantities to support commercial-scale production. Accordingly, the typical first step towards domestication is the development of systems to hold and condition broodstock, spawn them, and rear their progeny to the point where they can be transferred to farms. Identification of the breeding goals is particularly important and ideally should include all traits of economic importance with sufficient genetic variation to support improvement and be formulated with a long-term perspective. The choice of which traits to include in the programme's breeding objectives and their prioritisation are ideally rooted in an economic analysis that ranks their economic value as objectively and quantitatively as possible. Without this level of discipline, a breeding programme can succeed in changing the characteristics of a stock dramatically but fail to produce economic gains. Successful breeding programmes ensure that they provide economic benefits to aquaculture industries through close cooperation and communication among all stakeholders (e.g. government, industry, research organisations), by focusing on clear and economically viable short, medium and long-term goals, and on appropriate resourcing and realistic planning. At the end of the day, a genetic improvement programme must be sustained directly by the economic benefits it delivers to industry.
There are several biological factors that can constrain the success of a genetic improvement programme. One of these is trade-offs in production traits between environments or genotype-by-environment (G×E) interactions (Falconer Citation1989, Citation1990; Lynch & Walsh Citation1998) that make it difficult or impossible to breed a single, all-purpose genetic strain or variety that performs well under a wide range of environmental conditions. If G×E interactions are absent or weak, performance may vary dramatically among regions, sites or even individual farms, but the same genotypes that rank highly in one place rank highly everywhere. If G×E interactions are strong, the ‘winners’ on some farms can be ‘losers’ on others, and in this case it may be necessary to breed environment-specific strains or stocks, increasing the size and complexity of the breeding programme. While all species are subject to a range of environmental stresses, G×E interactions are particularly important for aquaculture species that rely on naturally-occurring food that cannot be supplemented or controlled by the farmer, such as filter-feeding bivalves. A second potential complication is unfavourable genetic correlations between desirable traits such that improvement in one trait produces an undesirable change in another (Falconer Citation1989; Lynch & Walsh Citation1998). Depending on the specifics of the genetic mechanisms behind such trade-offs, they can be either insurmountable ‘hard’ constraints that require compromises to be made or softer constraints that can be circumvented by a well-designed genetic improvement strategy.
Genetic improvement strategies generally fall into four broad categories that are not mutually exclusive and can be combined in various ways if adequate resources are available. The first and most common is selection of individuals or families: identifying and breeding only the best animals or families to achieve predictable, cumulative improvement over multiple generations based on so-called ‘additive’ genetic effects, defined as the influence of particular variants of genes independent of the genetic background in which they occur. The second is interspecific hybridisation in which crosses between species are produced to either combine their characteristics or exploit interspecific hybrid vigour (Bartley et al. Citation2001). The third is cross-breeding, an approach designed to capture much less predictable favourable non-additive genetic effects (the influence of interacting gene combinations rather than single-gene effects) or intraspecific ‘hybrid’ vigour by first creating many highly inbred lines then testing most or all crosses between them with the hope of finding superior combinations. What the three strategies above share is that they use direct measurements of target traits in combination with some sort of statistical analysis to determine which animals to breed or cross. The fourth category, in contrast, incorporates the tools of molecular genetics and statistical approaches to identify the genes that control the traits of interest and quantify their effects, then uses this information to select parents based on their genotypes.
Selection-based breeding strategies aim to identify individuals with a relatively higher proportion of desirable alleles and lower proportion of less desirable alleles as parents. In aquaculture, the strategies available vary in the type and amount of information collected and used to identify these parents. The method of choice depends on several factors, including the heritability of the targeted trait(s), whether the traits are normally distributed or binary, whether records can be obtained on live individuals, the reproductive capacity of the species, and the facilities and financial resources available.
Individual (mass) selection is the simplest strategy and the cheapest to implement, and in many circumstances it yields a rapid response. The only information tracked and used are measurements taken directly on the candidate parents. This has several limitations and drawbacks for aquaculture species. First, it can only be applied to traits that can be measured non-lethally, which excludes most product quality characteristics such as meat quality (Nguyen et al. Citation2010), disease resistance (Odegard et al. Citation2011) and age at sexual maturity (Kolstad et al. Citation2006a). Second, individual selection provides no way to separate environmental and genetic influences on the traits of interest, making it necessary to take precautions to ensure that the environmental contributions are as consistent as possible. Third, it is impossible to evaluate G×E interactions or genetic correlations and incorporate them into the breeding strategy without knowledge of the relatedness between individuals. Finally, because aquaculture species generally have very high reproductive output, individual selection can promote inbreeding. However, if breeding individuals are genotyped parentage information can be used to avoid crossing relatives and better manage inbreeding (Sonesson et al. Citation2005, Citation2011; Nguyen et al. Citation2014).
Among-family selection uses information collected on large groups of brothers and sisters to make decisions about whether or not to use entire families as broodstock and requires the parentage of individuals to be determined. This approach makes it possible to sacrifice some members of a family for lethal measurements or challenge experiments and apply that information to the entire family. Families can also be evaluated in a variety of environments to estimate G×E interactions and estimate the genetic correlations between traits of interest. In order to keep the rate of inbreeding low and the selection intensity high, the number of family groups bred and measured each generation should not be less than 50–100 (Fjalestad Citation2005). DNA-based genetic markers offer the possibility of mixing families immediately post fertilisation to homogenise environmental effects and reconstruct parentage, but at significant cost (e.g. Sonesson et al. Citation2005, Citation2011). If tanks for rearing families are limited, more families can also be tested and consequently higher selection intensities can be applied by mixing families, generating greater selection responses.
Among-family selection can target only the fraction of the available genetic variation that is expressed between families, generally thought to be about 50% (Fjalestad Citation2005). The remaining genetic variation can be exploited by within-family selection on traits that can be measured non-lethally, but care must be taken to ensure that these traits are not negatively correlated with the traits used for among-family selection as these opposing selective pressures would tend to negate each other. Generally, pure within-family selection is less efficient than other mating schemes although it has the benefit of being easy to manage and inbreeding can be kept to a minimum if a structured mating scheme, such as a rotational mating plan, is used. This approach has been used in rainbow trout (Oncorhynchus mykiss) (Gall & Huang Citation1988) and tilapia (Oreochromis niloticus) (Camacho et al.Citation2001).
Individual and family-based selection are both forms of forward-selection that aim to identify the best parents in the current generation. In contrast, progeny testing, as its name implies, uses measurements on offspring to identify the best parents from preceding generations, sometimes referred to as backward selection. Progeny testing can only be implemented in species that reproduce repeatedly and have a high probability of surviving until their progeny can be tested. This approach also doubles the generation time, which slows the response to selection considerably.
Combined and pedigree-based selection exploit multiple sources of information for a potential parent such as direct measurements, measurements on relatives in the same generation (e.g. full-sibs, half-sibs, cousins, etc.), and measurements on ancestors and/or progeny. Advanced statistical methods are used to arrive at a solution for obtaining the maximum rate of genetic gain. In principle this is always the best approach. The other simpler methods can be thought of as special cases of this method that use subsets of the potentially available information. Pedigree-based selection requires extensive record-keeping of parent–offspring relationships and the collection of individual-level data. The relationships between individuals are used to tease apart the genetic and environmental contributions to the traits of interest and generate an estimated breeding value (EBV) which represents the most accurate prediction of the mean value of an individual's progeny. A critical advantage of pedigree-based selection is that, by using information from all available relatives, it can produce EBVs for all animals in the pedigree for all traits, even for animals on which some, or even all, traits are not measured.
If the breeding objective includes multiple traits, they are typically combined into a selection index using weighting factors, ideally economic values (e.g. Atlantic salmon; Gjedrem Citation2010). Estimates of breeding values from multiple sources of information using best linear unbiased prediction (BLUP) analysis (Henderson & Quaas Citation1976) uses information from all known relatives, and produces EBVs for unmeasured animals with measured relatives. Thus, BLUP allows traits that can only be measured lethally to be included in the breeding goal. BLUP also simultaneously corrects for differences in defined environments or different periods of time and also among animals for which different amounts of information are available or that undergo different intensities of selection. In addition, because BLUP partitions genetic and non-genetic effects on performance it is increasingly applied in family-based breeding programmes (Gjøen & Gjerde Citation1998; Kolstad et al. Citation2006a,Citationb; Gjedrem Citation2010).
For all selection-based strategies, assembling a genetically diverse base population, and understanding the potential for genetic change and any genetic trade-offs between traits, is critical as these are population-specific rather than characteristic for the species. Selection response, or genetic gain, measured as incremental improvement per generation, is another critical aspect of the efficiency of a breeding programme (Gjedrem Citation2000; Fjalestad et al. Citation2003; Langdon et al. Citation2003; Dégremont et al. Citation2010). On average, response estimates for efficient programmes are around 12%–15% per generation for growth rate. This equates to a doubling of growth rate in less than seven generations, as demonstrated by the Atlantic salmon family-based breeding programme (Gjedrem Citation2010). Higher survival rates and improved yield will also reduce production costs.
Interspecific hybridisation involves crossing individuals from different species with the objective of exploiting ‘hybrid vigour’ for commercially important traits or to obtain specific combinations of valuable traits (Bartley et al. Citation2001). There are some applications of hybrids in brackish and freshwater aquaculture (Bartley et al. Citation2001; Garber & Sullivan Citation2006) and experiments have been conducted between marine fish species (Hulata Citation1995).
Inbreeding and cross-breeding strategies are a form of progeny testing designed to either stabilise a desirable variety or capture cross-specific non-additive genetic effects such as dominance (unequal influence of the two copies of a single gene) and epistasis (interactions between different genes). Cross-breeding requires multiple generations of close inbreeding or chromosome manipulations to create homozygous lines that are subsequently crossed in as many combinations as possible. For commercial-scale production, the best crosses must be recreated using the same inbred parent lines because the non-additive heterotic effects that make them superior are not heritable. Because inbreeding depression is particularly severe in highly fecund species that are largely undomesticated, such as those used in aquaculture and in particular bivalves (Launey & Hedgecock Citation1999, Citation2001; Evans et al. Citation2004; Camara et al. Citation2008), the production and maintenance of inbred lines can be difficult and costly, and their pure line performance is usually severely compromised. Most fish and shellfish breeding programmes focus on selection on additive genetic effects to exploit the abundant additive genetic variation in relatively undomesticated species, but cross-breeding between partially inbred lines is being applied to Pacific oysters in the USA (Hedgecock et al. Citation1995).
Finally, the recent explosion in molecular techniques and marker types has made it possible to exert direct selection on genes rather than on traits. These methods vary with regard to the type and number of genetic markers used and their degree of association with traits of interest. At one extreme, if markers associated with genes that exert a high degree of control over a trait of interest can be identified, it is possible to select parents with the desirable gene(s) directly using marker-assisted selection (MAS) (see Collard et al. Citation2005 for an overview). At the other extreme are situations in which a trait of interest is controlled by a very large number of genes with very small effects, making it difficult to understand the functional roles of these genes; in which case, advanced statistical approaches are used to identify the marker-trait relationships in a probabilistic rather than functional sense, including genetic linkage, and all of these relationships are incorporated into selection decisions using whole genome selection (Meuwissen et al. Citation2001; Goddard Citation2009). The theory is that specific DNA polymorphisms that contribute to variation in economically important traits can be used to generate genomic breeding values which, when used in conjunction with traditional selection, can lead to increased rates of genetic improvement. Advances in new single nucleotide polymorphism (SNP) genotyping technologies (e.g. Elshire et al. Citation2011) will reduce the cost of genotyping so that eventually the technology will become affordable. Use of molecular techniques and genomic selection has the potential to profoundly improve genetic gain by minimising inbreeding and improving the accuracy of selection especially for traits that cannot be measured on live candidates.
Current breeding programmes in New Zealand
Over the last two decades, the ability to improve aquaculture stock performance using genetic technologies has become established in New Zealand. In the following sections, we describe the current state of genetic improvement programmes for fish and shellfish in New Zealand.
King salmon
History
Chinook salmon (also known as Quinnat or king salmon) is successfully farmed in New Zealand. New Zealand king salmon is regarded as being of excellent quality and is free of major salmon pathogens. New Zealand king salmon is exported to 30 countries and is readily available at local supermarkets and restaurants around New Zealand. In 2012 the salmon industry generated $128 million in revenue (www.salmon.org.nz).
New Zealand has no native salmon species. All the salmonids were originally imported as ova by the Marine Department between 1901 and 1907, and were introduced into the Waitaki River (McDowall Citation1994) from the Baird Fish Station on the McLeod River, a tributary of the Sacramento River in California. Interest in salmon farming grew steadily during the 1970s as part of a worldwide trend towards commercial aquaculture. New Zealand's first commercial salmon farm was established in 1976 as an ocean ranching venture at Waikoropupū Springs in Golden Bay. The initial sales from this venture in 1978 were freshwater-reared salmon. Other early ocean ranching farms included an ICI/Wattie joint venture on the lower Clutha River, and larger-scale hatcheries on the Rakaia River and the nearby Tentburn coastal site. Overall, ocean ranching was not economically viable and farming was seen as a better, more reliable, alternative. The first sea-cage salmon farm was established in 1983 in Stewart Island's Big Glory Bay by BP New Zealand Ltd. This was soon followed by the development of farms in the Marlborough Sounds. Currently, most of New Zealand's salmon production occurs in sea-pens in the Marlborough Sounds and Stewart Island, with smaller-scale production in Akaroa; all the freshwater farms are in the South Island in the hydro-canals in the New Zealand MacKenzie Basin (www.salmon.org.nz).
The industry is made up of several companies all based in the South Island. summarises the companies involved, their location and production tonnages.
Table 1 New Zealand salmon farming companies, location and production tonnage.
King salmon spawn in April/May and each female produces approximately 5000–7000 eggs. The eggs can be collected by manual stripping and are then fertilised externally with sperm stripped from broodstock males, providing full control over mating. The eggs, fry and juveniles are reared in freshwater and are later transferred to sea farms as smolt in late spring or early winter, or are farmed whole of life in freshwater (hydro-canals) up to harvest. Harvest size is usually in the 3–5 kg range.
Breeding objectives
The industry in New Zealand is based on the sale of premium quality salmon to the local and global market. Therefore, it is essential that the salmon are not only healthy and grow well but also produce flesh of consistent good quality, colour, desirable fat content and lipid composition. For this paper the salmon farmers listed in were asked to identify the key attributes they look for when selecting broodstock. In general an individual that grows well and has good body conformation is preferred. Delayed age of sexual maturation (at 3 years or later) is also important in salmon as flesh quality deteriorates with the onset of maturation and the salmon die within a few months of becoming sexually mature. However, because of the association between faster growth and maturation, stocks that mature as 2-year-olds are now sometimes used if harvest size can be achieved before maturation becomes an issue. Farming a stock with good feed conversion efficiency is also important given the high cost of feed.
The larger farming companies operate breeding programmes based on the selection of pedigreed fish with multiple desirable traits. For some of the other companies there is also an objective, driven by customer preference and access to some specific markets, to produce stocks that are as close as possible to wild salmon populations. This objective requires the use of wild broodstock that return to freshwater to spawn and acceptance that the performance of their progeny may be less predictable.
Breeding strategy
There are two main breeding strategies employed by the industry: (1) family-based selective breeding for multiple traits; and (2) individual broodstock selection based on the phenotype and age of the breeding individual. The two largest companies both have family-based breeding programmes, whereas the smaller companies rely on individual selection.
The family programmes operate by establishing individual family crosses each year for rearing and evaluation. Evaluation includes rearing in a sea-pen to assess their performance for the commercial traits identified in the breeding objective. This includes terminal harvesting of enough individuals per family to provide genetic parameter estimates and EBVs for carcass quality traits. The breeding objective includes several different traits and these are combined based on their relative value and prioritisation into a selection index that is used to rank broodstock. A combination of among- and within-family selection is applied.
Individual selection for salmon is based on selecting broodstock of good size and appearance and appropriate age of maturation, either from broodstock held on site in freshwater, from sea-pens or wild returns (age unknown). This method excludes the potential for carcass quality traits to be assessed as only live salmon are part of the selection decision.
Special issues, challenges and opportunities
King salmon farmers have faced several challenges. One is the incidence of early maturation which, like for many salmonids, tends to be unfavourably correlated with growth (Gjerde et al. Citation1994). Family selection can help reduce early maturation (Gjedrem Citation2010) and has been effective in king salmon. Another strategy the industry has used to avoid precocious maturation in males is the farming of all-female stocks (Hunter et al. Citation1983). This was made easier in king salmon by the identification of four sex-linked DNA markers and the development of DNA-based tests for sexing (Devlin et al. Citation1991; Du et al. Citation1993). Most of the New Zealand farmed salmon is based on all-female salmon, but some mixed sex populations are also farmed. Recently, the use of lights to extend day length has been used to control maturation (Unwin et al. Citation2005) and in some farming situations maturation is now less of an issue.
Selecting stock in different environments (sea-pens versus freshwater) or in changing environments (e.g. diet or climate changes) also creates a challenge and it is possible that G×E interactions might influence family performance and ranking. To avoid this issue, broodstock are generally selected directly from the commercial rearing environment, or selection decisions are based on family performance in both environments. Fortunately, throughout multiple generations of evaluation, the New Zealand King Salmon Co. Ltd. (NZKS) has found a strong positive correlation between families reared in freshwater and seawater for production traits (Bailey Citation2011).
Commercial salmon diets are also changing, with fish meal and fish oils being replaced by plant-sourced proteins and oils (Tacon & Metian Citation2008). How this affects family performance and flesh composition is an area for further study (e.g. Bell et al. Citation2010; Morais et al. Citation2012). Similarly, the sea-pen environment and husbandry practices can change over time, and the question of how different stocks or families perform or tolerate such changing conditions remains to be evaluated.
An effective breeding programme, no matter how simple, should also manage inbreeding and avoid crossing relatives. This is easier to manage in family programmes with known pedigree, but is more difficult when individuals of unknown pedigree are selected. One strategy is to cross males and females from different populations. The risk of inbreeding is reduced but still remains a possibility. The risk of inbreeding can be reduced by determining the relatedness among the selected individual broodstock using microsatellite DNA genotyping. The cost of genotyping remains high but new SNP technologies should hopefully see the cost reduced over the next few years. A Chinook salmon SNP chip was recently developed by NIWA and AgResearch (Clarke et al. Citation2011) and there is the potential for this to be further developed as a cost-effective tool for parentage analysis in the future.
Progress to date
Family based selection: the New Zealand king salmon programme
The family breeding programme was established in 1994 at the Kaituna hatchery near Blenheim by Southern Ocean Seafood Ltd. The programme was then continued by NZKS when this company was formed in 1996 after the privatisation and merger of New Zealand's two largest salmon companies, Southern Ocean Seafood Ltd and Regal Salmon Ltd.
The programme, now in its seventh generation, establishes 90 to 100 families per year. Individual family fish are tracked using electronic tags inserted into their body cavity when they reach approximately 10–20 g. A subset from each family are reared in a sea-pen and harvested to provide detailed information on post slaughter quality traits (e.g. fillet colour and fat content) as well as harvest weight and incidence of 2 year maturation. Future broodstock from each family are reared in freshwater and several traits (e.g. weight, age of sexual maturation, body conformation) are recorded for the broodstock themselves. Within the breeding programme there are three cohorts of families running simultaneously, as the most common age for reproductive maturation is 3 years of age.
The breeding merit of each individual fish is assessed by BLUP analysis based on its own records and the records of its close relatives (Amer et al. Citation2001). Total genetic merit selection indexes are computed using EBVs for each trait, and sets of economic values specific to several different production systems, and for the breeding nucleus. NZKS operate three commercial production strategies in which both the absolute and the relative economic importance of the primary selection traits differs substantially. Thus, each candidate parent has to be sorted for use in the breeding nucleus among the three production strategies or, alternatively, rejected and not used at all. A sorting algorithm is used to optimise the allocation of parents to each production strategy (Amer et al. Citation2001). The algorithm is constrained such that the appropriate numbers of each sex of fish are assigned to production strategies based on planned future production levels. Mating pairs are established such that the co-ancestry between parents is below a pre-specified threshold.
The first step for the nucleus selection involves calculation of the number of individuals, if any, each full sib family should contribute. This is done in the context of maximising the expected genetic progress while maintaining co-ancestry among the parents, and hence maintaining acceptable levels of genetic variation. Meuwissen (Citation1997) developed a method that achieves this when selecting individual parents using an optimisation algorithm. An adaptation of this algorithm to consider optimal selection of full sib family contributions (Amer & Symonds Citation2000) is used in NZKS programme. Other strategies employed include the use of 2-year-old maturing males from families with an overall low incidence of female early maturation and the use of cryopreserved sperm from 3-year-old males, a year or more after they spawned. This effectively creates genetic links among the cohorts and generates one connected group of families.
Early analysis of the moderate to high heritability of production traits, such as harvest weight and fillet colour (Symonds et al. Citation2000), demonstrated the potential for selection. As a consequence, the NZKS programme has successfully improved growth so that consistent year round production of harvest size salmon is no longer a limitation (Bailey Citation2011). The pedigreed programme has also recently led to the development of a premium quality high-value brand, Ōra King salmon (http://orakingsalmon.co.nz).
In the NZKS programme, feed conversion efficiency (FCE) has not traditionally been included as a breeding objective (Walker et al. Citation2012) due to the difficulty and cost of assessing feed intake in individual fish. Recently, using families from the NZKS breeding programme, Walker et al. (Citation2012) demonstrated that labelled feed and portable digital X-ray technology provides a feasible method for assessing FCE, and reported high heritabilities for mean daily feed intake and moderate heritablities for natural log-transformed feed conversion ratio. The FCE data have since been applied within the NZKS breeding programme and 3-year-old broodstock were sorted into production strategies using the FCE information. This resulted in the most feed efficient fish, which spend the most time in the sea-pens, being directed into the production strategy most likely to return benefits from FCE improvements. Measuring FCE opens up new options for the industry as it is possible to use the X-radiography method in sea-pens or land-based tanks/ponds to estimate FCE in individual fish, and to better understand feeding behaviour and the relationship with growth, as well as select for improved FCE.
Family based selection: the Sanford's programme
The Sanford's family breeding programme was established in 2007 and is now in its second generation. About 150 families are generated each year with eggs from each family raised separately until just before hatching. At this stage, eggs from each family are pooled so that each family is equally represented in the pool. All breeding programme smolt are transferred to salt water at about 20 g. The male and female brood are raised together and culled based on weight before 2 years of age. About 50 % of the top ranked fish are individually tagged and DNA genotyped to identify parentage. Family evaluation takes place in a sea-pen farmed under commercial conditions and families are harvested before 2 years of age at an average weight of about 3 kg. All broodstock and evaluation fish (> 10,000) are measured for weight, maturity status at 2 years and conformation. A random selection of about 1800 evaluation fish are filleted and evaluated for quality traits (oil %, colour, tail fade and gaping) and genotyped to provide family information. In the remaining evaluation group, early maturing salmon and individuals with faults are also traced back to family.
Breeding values are calculated for all candidate broodstock using a BLUP procedure modified to manage the selective genotyping. Broodstock EBVs are based on individual and family data for weight gain and family data only for quality traits. The breeding fish are selected on an index which aims to maximise growth rate while maintaining quality at high levels and minimising losses from early maturity. The final brood selection uses an optimisation procedure to balance genetic gain and diversity in the candidate broodstock. Final mate selection uses an optimisation programme adapted from Kinghorn (Citation2011). This programme manages daily and overall family matings using an objective function to balance genetic gain and inbreeding. The genetic gains for growth achieved by this programme to date have exceeded the initial expectation of 10% per generation (M. Tate, Tate and Matthews Ltd, pers. comm. to JES, 8 November 2013).
Future prospects
The future prospects for the salmon industry are positive. Future expansion plans will see the industry grow with continued emphasis on the supply of high-value premium product. The breeding programmes will need to evolve to meet the challenges of changing environments and diet. In addition, more cost effective DNA genotyping should make inbreeding control more accessible to all farmers.
Kingfish and hāpuku
History
The expansion of the New Zealand aquaculture sector will benefit from diversification into new high-value finfish such as yellowtail kingfish (Seriola lalandi) and hāpuku (groper, Polyprion oxygeneios). To ensure the success of a new species, domesticated selected broodstock need to be established, along with systems that can reliably produce high quality gametes and juveniles. Over the last 10–12 years NIWA has established broodstock development programmes for yellowtail kingfish and hāpuku. Wild broodstock collections were completed from 2003 onwards and over 100 wild broodstock of both species have been reared in captivity since. Wild females of both species are very fecund and millions of eggs can be produced each season. While this provides significant production opportunities from only a small number of broodstock it also means additional broodstock management strategies are required to ensure enough individuals are kept to sustain a healthy breeding programme.
Breeding objectives
The breeding objectives for any new aquaculture species remain a work in progress and develop as the industry matures and better understands the biology, production challenges and market requirements for each species. Therefore, the current objectives are mainly focused on establishing selected populations of genetically variable offspring (F1/F2) produced by sufficient parents to sustain the initial development of the industry. The creation of offspring of known parentage also provides the opportunity to evaluate the genetics of growth, a high priority trait for farmers.
Breeding strategy
There are two main breeding strategies currently employed: (1) family-based selective breeding for growth; and (2) individual broodstock selection based on phenotype and inbreeding control using genotyping, plus selection based on the performance of an individual's offspring (progeny testing).
Due to the current reliance on communal group spawning for the production of hāpuku and kingfish fertilised eggs, genotyping panels comprising 9–10 variable microsatellite DNA markers have been developed by NIWA and AgResearch to determine the parentage of the resulting progeny (Symonds et al. Citation2012a).
Special issues, challenges and opportunities
Collecting large marine fish from the wild is challenging, especially for a deeper dwelling species such as hāpuku. Hāpuku are caught using hook and line and slowly raised to the surface. NIWA's experience has shown that individuals in the 10–12 kg range have a higher chance of survival and breeding in captivity. The large population sizes, high fecundities, and mobility of kingfish and hāpuku generally restrict genetic divergence (Nugroho et al. Citation2001; Smith Citation2008; Lane Citation2013). Therefore, collections have focused on locations with an increased likelihood of capture and survival.
Although kingfish and hāpuku are highly fecund, the survival of eggs and larvae is variable (Kohn & Symonds Citation2012). Even though F1 from multiple parents have been identified, a small number of broodstock produce a disproportionate number of the surviving progeny. This has highlighted the need to manage parental contribution to the F1, which would otherwise lead to the exclusion of many individuals during spawning. To increase the chances of different males and females contributing, multiple batches of eggs are collected at different times and are reared separately for as long as possible.
Kingfish are more easily manipulated during spawning than hāpuku and can be handled during the breeding season to establish different spawning groups, either as individual male and female pairs to generate families or to change the mix of parents in a tank (Symonds et al. Citation2012a). However, handling wild hāpuku is avoided during spawning and this restricts what can be produced.
Progress to date
Family rearing and evaluation, even if only at a small scale, is essential for genetic evaluation and to determine genetic parameters in order to design optimised breeding programmes for kingfish and hāpuku. Selection of the best performing families under culture conditions can also help the initial stages of domestication of these species and provide the fledgling industry with more predictable, and economic, stock performance.
Kingfish
In the 2013 spawning season the focus was the production of families for evaluation. Thirty-six wild broodstock were distributed among five 20 m3 spawning tanks, each containing four to five females and two to four males. Fertilised eggs were collected from November through to March and batches were reared separately in six different groups in the nursery. To determine spawning contribution of individual broodstock, genotyping was completed on samples of 27 to 30 fertilised eggs (n = 2002) from 67 batches. All males, and up to 77.3% of the females, contributed to the genotyped eggs. Most individual spawning events (62.7%) involved only one female in each tank. In contrast, more than one male was always involved and frequently all males in an individual tank participated. In total, 51 different crosses, or families, were produced. Tagged individuals from the families are now undergoing growth performance analysis as the initial phase of their evaluation and eventual selection as broodstock. The prospects for genetic selection are good and a moderate to high heritability for growth is expected based on the results for Australian kingfish (Whatmore et al. Citation2013).
Hāpuku
As with kingfish, egg genotyping has proven to be a useful tool to assess parental contribution and spawning behaviour (Symonds et al. Citation2012a). Since 2008, 7408 individual eggs have been genotyped and parentage determined. In 2008 and 2010, an attempt to produce larger numbers of hāpuku juveniles resulted in a combined total of over 60,000 F1 juveniles; 4702 of these F1 were subsequently individually tagged and genotyped to determine their parentage. This resulted in the production of 86 families, with 56 families comprising seven individuals or more. The growth evaluation of tagged individuals from these families is ongoing and significant differences have been observed. For example, mean family weight ranged from 3.3 to 5.7 kg at 2.5 years post-hatch.
Unlike king salmon, individual hāpuku spawn annually. Therefore, the data collected on individual broodstock contribution during spawning, plus the performance of their progeny, can be used to identify good performing wild broodstock. These progeny-tested broodstock can then be targeted for spawning until the next generation reaches sexual maturation.
Future prospects
NIWA has developed a generic cost-benefit model for marine broodstock (J. Symonds (NIWA) and P. Amer (AbacusBio Ltd), unpubl. data, 2010) to establish the economic benefit and ROI of selective breeding based on different production tonnages and associated costs of broodstock rearing. The model compares two different breeding programme designs (family versus individual selection with genotyping), allowing genetic parameters and correlations for three traits to be entered as variables. Based on this model, an individual selection programme for a single trait–growth–with no unfavourable relationships with the other commercially valuable traits, provides a good ROI even when production tonnages are small. As production increases, or when there are unfavourable relationships with other traits, the benefits of family selection outweigh the additional costs. A similar model applied to genetic improvement of kingfish in Australia estimated a 60% internal rate of return from a breeding programme selecting for growth rate with 60 families (N. Robinson, Nofima, unpubl. data, 2009). As the industry develops, the priority traits will also likely include product quality traits and age of sexual maturation and these traits will be closely monitored from the outset.
Overall, good progress has been made towards the domestication of these species. In addition, by ensuring that multiple parents contribute to the programme, the future potential for selection has not been compromised. The next step is the production of offspring from selected F1 broodstock.
GreenshellTM mussel
History
The New Zealand GreenshellTM industry still depends almost entirely on wild-caught spat from two main sources: attached to seaweed washed onto Ninety Mile Beach (North Island) or collected using specially designed ropes in Golden Bay and Marlborough Sounds (South Island). These spat supplies are both limited and unpredictable, and the retention of spat on seaweed after translocation to mussel farms is typically 0%–5% (K. Heasman (Cawthron Institute) and D. McCall (SPATnz), pers. comm. to MDC). Farming wild spat precludes genetic improvement of any kind, but a new public/private partnership to build a commercial-scale hatchery in the Cawthron Aquaculture Park in Nelson and commercialise the pilot-scale mussel breeding programme has recently been established, and the industry is poised for dramatic change.
The initial emphasis of Cawthron's mussel programme was to develop the technology and background knowledge required to produce and deliver genetic gain once commercial-scale hatchery capacity becomes available. An early achievement was the development of a novel high-density flow-through larval rearing system (King et al. Citation2005) and optimisation of its performance to enable the simultaneous production of large numbers of families (Ragg et al. Citation2010). Cawthron produced its first founder cohort of 75 full sib families from wild parents in 2002 and another 69 founder families in 2003. Since then, six additional cohorts of about 50 families (mainly crosses between top-performing families from earlier cohorts, but including some new wild families) have been produced. All of the cohorts produced before 2008 were evaluated at a minimum of six sites and subsequent cohorts were evaluated at two to six sites. The selection protocol for these cohorts was relatively simple: site-specific family means were calculated for each trait and family-level estimates of genetic merit weighted these means according to site-specific heritabilities of the traits.
Breeding objectives
For cohorts produced between 2002 and 2011, parents and progeny have been measured for as many traits as possible to facilitate estimates of heritabilities and genetic correlations. These cohorts emphasise full sib crosses to simplify data analysis and whole families have been selected on the basis of the most economically valuable traits according to industry representatives, mainly shell length and meat weight. Top preference has been for families with shell length and meat weight well above average. Second preference has been for families where one of these traits is well above average and the other is average or above. Within-family selection has been imposed on the basis of shell length, since meat weight is not easily determined without sacrificing the individual. Initially, the programme imposed moderate among-family selection intensity, anticipating that the industry's breeding objectives will likely evolve, and there is long-term advantage in maintaining a broad breeding population with sufficient genetic variation to change direction or refocus as necessary.
Beginning in 2013, when the programme was commercialised as part of a private growth partnership (PGP) between the cultured mussel industry and government, it has developed a more intensive and rigorous selection index approach that combines four production-related traits: shell length; meat weight; shell weight; and a semi-quantitative, visually-assessed meat condition score. Shell length is included as the simplest measure of growth rate. Meat weight has been identified by industry as a top priority. Genetic analyses (see below) have found that shell weight has an extremely high genetic correlation with shell breaking strength, which has also been identified as an economically important trait by industry. Meat condition is based on how much of the shell is covered by the meat in a half-shell presentation and its plumpness, an important consumer attribute.
Research is in progress to evaluate the potential for incorporating the seasonal time-course of meat condition (i.e. reproductive cycle) to mitigate the current ‘winter window’ when most mussels are spawned out and unsuitable for harvesting, and for changing consumer attributes such as shell colour and content of high-value lipids.
Consistency of product within a growing unit (line) at the time of harvest has been suggested as an additional breeding objective. Reducing within-batch (or within-family) variation is potentially complex as it represents the combined effects of within-family genetic variation, within-family small-scale G×E related interactions (for example, depth within a mussel-growing line) and genotype-by-genotype interactions between individuals. Plant breeders typically use close inbreeding and/or cross-breeding to stabilise cultivar varieties, but inbreeding depression in bivalves is typically too severe to make this approach viable. A simpler strategy is to restrict commercial ‘seed lots’ or batches to crosses between unrelated pairs of families with similar characteristics, such as growth rate and timing of reproduction, to minimise within-family genetic variance without inbreeding. This approach has additional advantages in that using different, well-characterised parental families can also tailor batches to specific conditions or needs.
Breeding strategy
The crossing designs used before 2013 used ‘best × best’ assortative crosses and avoided matings between close relatives based on single-generation parental information. A disconnected factorial design was employed to allow for estimates of both additive and non-additive genetic variance and to explore the potential for backward selection of sires using cryopreserved sperm. This approach was adequate for early generations in which relatedness was very low, but does not rigorously account for deeper relationships in more advanced generations.
All families are grown in unreplicated, single-family tanks in the hatchery and nursery until they are large enough to engrave with their family identification number. Field trials are conducted by individually tagging representatives of all families when they reach about 50 mm shell length and mixing these marked animals for re-seeding onto rope ‘droppers’ to minimise common environmental effects in the field. These mixed-family droppers are harvested and destructively sampled to provide family-specific measurements of external shell dimensions, meat weight, shell weight and shell strength. Separate, single-family droppers are also held as broodstock.
Among-family selection is currently based on the family mean of estimated breeding values from a pedigree-based BLUP analysis of the mixed-family droppers in which four component traits (shell length, meat weight, shell weight and meat condition) are combined into a selection index with relative weightings of 2:2:1:1 and within-family selection is based entirely on size variation on single-family droppers. These weights will, however, be refined in the near future using a recently-developed process-based bioeconomic model of the GreenshellTM mussel industry (M. Camara, Cawthron Institute, unpubl. data).
Special issues, challenges and opportunities
The GreenshellTM mussel industry is fortunate that there are currently no major issues with disease or mortality. Perhaps the biggest challenge, however, is the fact that extremely high allocation of energy reserves to gametogenesis, and an annual cycle of spawning, produces a ‘winter window’ when post-spawning mussels have such poor meat condition that they are unsuitable for harvest and processing, while at other times large volumes of crop must be harvested quickly in anticipation of spawning and loss of meat condition. Currently, the industry uses wild-caught spat from different areas to provide some control of harvest condition seasonality. Wild spat caught in Golden Bay tend to be harvestable during spring and summer, while wild spat from Kaitaia tend to be harvestable from summer through to early winter. The popular belief is that genetic differences between the various stock types are responsible for these seasonality differences. However, when families from different stock types are grown in parallel under controlled conditions in the same hatchery and nursery, differences in condition between the stock types are not apparent (N. King and M. Camara, Cawthron Institute, unpubl. data). This leads to the hypothesis that the time of spawning and other environmental factors may play a more important role in determining condition timing than genetics, but this has not been rigorously tested. If genetic differences in conditioning and spawning behaviour could be identified, this would enable limited control of the seasonality of meat condition. Triploids are also likely to provide some control of condition, but are unlikely to completely replace the use of diploid stock because triploids do not produce the orange-coloured female mussels desired by export markets.
A somewhat different, but potentially very important, opportunity is to help the mussel industry to expand by developing strains or breeds that make it economically viable to farm at sites where this is currently not the case, such as open ocean sites, low-productivity sites or sites where environmental conditions such as water temperature or salinity are outside of the current tolerances of GreenshellTM mussels. The key question is whether there are strong G×E interactions or unfavourable genetic correlations that would necessitate specialist strains for these currently marginal habitats, or if genetic gains produced in a generalised breeding nucleus would suffice to expand the economically viable range of GreenshellTM mussels. To address this, the programme is currently conducting a multi-year, multi-site study of families produced by re-spawning the same parents. In addition, studies are underway to determine the degree to which variation in basal metabolic rate and FCE are under genetic control, with the idea that highly efficient mussels may be better suited to sites with low primary productivity (Lurman et al. Citation2013).
The mussel industry is also gradually shifting from a production to a value chain focus, and consumer attributes such as shell colour have started to receive attention. Currently marketed as the Greenshell™ on the basis of shell colour, it would seem logical to make use of the very apparent differences in shell colour between families. One company is already using golden shelled broodstock to produce gold-coloured mussels. As well, GreenshellTM mussels have high levels of compounds with putative anti-inflammatory properties, and research is just beginning to determine how these levels are affected by environmental and genetic factors.
Progress to date
Analyses of all cohorts for which complete data are available (i.e. produced prior to 2012) using mixed-model BLUP indicate that the heritabilities for the economically important traits measured so far are moderate to high, there are no strong adverse genetic correlations between traits () and that, while genotype-by-environment interactions are statistically significant, they are not so strong as to preclude breeding a single strain for use under a wide range of environmental conditions (M. Camara, Cawthron Institute, unpubl. data). After two rounds of selection, shell length and meat weight show strong responses, even with the conscious decision to impose only moderate selection intensity in the early generations of the programme (, ), as do shell weight, gonad condition and the weighted four-trait selection index (–), even though these traits were not selected for directly.
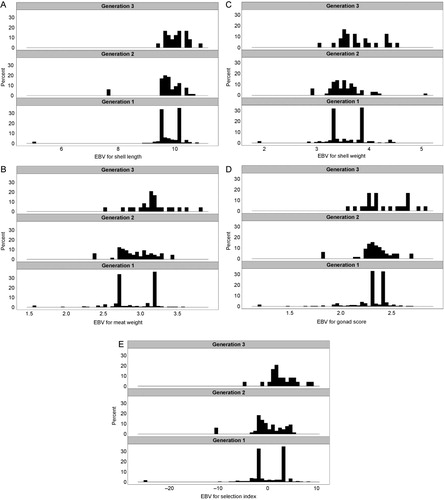
Table 2 Genetic parameter estimates (standard error) from mixed-model analysis of records from the 2008 and 2009 GreenshellTM mussel cohorts.
As part of the commercialisation of the breeding programme, a process-based bioeconomic model of the industry has been developed and is being used to generate an appropriately weighted multi-trait selection index. A data analysis pipeline has been created to both estimate BLUP breeding values using pedigree-based mixed models and balance genetic gain versus the loss of genetic diversity using an optimal contribution approach (Meuwissen & Luo Citation1992) implemented in the EVA programme (Berg et al. Citation2006). As well, the programme is devoting considerable effort to physiological-level research aimed at developing ways to measure metabolic efficiency in order to ensure that fast growth does not lead to a reduction in farm-level carrying capacity, biochemical research to better evaluate stress responses and product quality traits such as lipid and carbohydrate content. Molecular tools to enable quantitative trait locus mapping, marker-assisted selection and whole genome selection, are also being developed.
Future prospects
The future prospects for hatchery-based GreenshellTM mussel production are bright. A commercial-scale hatchery is currently under construction as part of a 7-year PGP, and the selective breeding programme has transitioned from a pilot-scale effort, focused on developing the required technologies and background information, to imposing high selection intensity on multiple traits in proportion to their economic impacts using pedigree-based estimated breeding values. Physiological, biochemical and genomic-level research in progress will facilitate the maturation of the breeding programme and help to create new opportunities for the industry.
Pacific oyster
History
The New Zealand Pacific oyster industry has been traditionally based on wild spat caught on sticks, mainly in Kaipara Harbour in the North Island, which are then transferred to other North Island harbours for on-growth. The Cawthron Institute initiated a Pacific oyster breeding programme in 1999 with the initial objectives of breeding high-surviving, fast-growing oysters with good shell shape and a high meat-to-shell ratio using a combination of between- and within-family selection. Preliminary evaluations found high survival of all of the nine families tested, but large differences in family-specific growth rates and no evidence of strong G×E interactions. Accordingly, from 2003 to 2010, the programme's breeding objectives were mainly faster growth and aesthetic qualities, such as higher meat volume, desirable shell characteristics (e.g. shape, colour and degree of ridging) and uniformity (King & Janke Citation2006), with selections based on family-level phenotypes for semi-quantitative family rankings by panels of producers and processors–an approach akin to what Harris (Citation1998) has called the traditional ‘art’ of breeding. The first two cohorts consisted of 60 families, but limited funding necessitated reducing subsequent cohorts to about 30 families.
In 2010, sudden and unanticipated mass mortalities of both cultured and wild oyster populations on the North Island and in Cawthron's oyster hatchery on the South Island, caused by a highly pathogenic variant of the oyster herpes virus (OsHV-1 µ-var), precipitated an equally sudden shift in the programme's objectives and strategies. After a brief closure of the hatchery and consultations with the Ministry of Primary Industries (MPI), Cawthron developed upgraded biosecurity systems and procedures, and increased surveillance of its broodstock conditioning and hatchery facilities to allow the continued production of selected families and the use of survivors from field trials at OsHV-1 infected sites as broodstock, once it was determined that the virus is not transmitted from parents to offspring during strip spawning. Under these new guidelines, all broodstock and larvae are strictly quarantined, with effluent from both the conditioning system and hatchery sterilised using UV light and chlorination. All incoming water is UV sterilised as an extra precaution against introducing the virus from the environment, and all animals are tested before being transferred from the hatchery to non-quarantined nursery systems or from the nursery to field sites. There have been no positive tests for OsHV-1 since the initial outbreak in 2010.
Breeding objectives
The devastating economic impact of the OsHV-1 virus on the Pacific oyster industry has transformed survival from a non-issue to the single most important breeding objective. The current challenge is to enhance resilience to the OsHV-1 virus as rapidly as possible while retaining as much of the genetic gain achieved during the previous decade of selection for quality-related traits as possible.
Breeding strategy
In 2013, the programme transitioned to using pedigree-based mixed-model BLUP to estimate genetic parameters and additive breeding values using quantitative measurements on individuals. At present, the only available metric for OsHV-1 tolerance is survival at infected sites when the virus is active, but a laboratory-based viral challenge model for spat and juveniles is currently under development through a collaboration with Australian researchers (P. Kirkland, NSW Department of Primary Industries, pers. comm. to MDC, 1 May 2014) and will be incorporated into the breeding strategy as soon as it is available. Measures such as individual meat/shell ratios, meat coverage of the bottom valve and appropriate ratios of shell dimensions will replace quality scores after consultation with industry to determine how to best capture their subjective impressions with objective, quantitative, data.
In response to the oyster industry's desire to exploit the putative impacts of natural selection for OsHV-1 on naturalised oyster populations in OsHV-1 infected harbours, some wild-set oysters grown on sticks were incorporated into the mating design of the 2011 and 2013 cohorts using two strategies implemented in parallel: single-pair crosses and multi-parent mixed ‘seed lots.’ All wild parents were chosen by industry based on external characteristics. Single-pair crosses between randomly chosen pairs of parents were raised in separate tanks and evaluated as first generation (founder) families in the nucleus breeding population. In contrast, the mixed-parent seed lots used a small number of larval tanks to screen a large number of wild × wild families using a procedure similar to the ‘walkback selection’ procedure of Doyle & Herbinger (Citation1994). This strategy pools large numbers of wild families in a single tank to simultaneously minimise the number of tanks, maximise selection intensity and eliminate the confounding of tank effects with family effects. Eggs from a large number of wild-caught females are pooled in roughly equal numbers, after which the pool is split into aliquots, each of which is fertilised using sperm from a different wild-collected male resulting in a factorial mating design. After fertilisation, the fertilised aliquots are re-pooled, raised as a group, and exposed to the OsHV-1 virus in the field as small spat. Mortality imposes between- and within-family selection on viral tolerance, and at harvest size the survivors are evaluated for growth/size and external shell shape. DNA markers are used to determine the parentage of the most desirable ‘elite’ survivors and to identify the most abundant families in this group. This information is then used to manage relatedness between the animals used as parents in single-pair crosses in the following generation.
Special issues, challenges and opportunities
Breeding for OsHV-1 resilience is challenging for a variety of reasons. As described above, the first challenge has been to develop and implement biosecurity systems that allow the use of potentially infected broodstock in a hatchery located on the South Island where OsHV-1 is not a problem. Without this capability, it would only be possible to impose pure among-family selection on naive broodstock held in a virus-free site, but this approach cannot exploit within-family genetic variance and would likely reduce the response to selection by about half (Fjalestad Citation2005). Another serious problem is the programme's reliance on field challenges, which can be unpredictable in time and space and highly variable in intensity. The single year of field challenges conducted to date in New Zealand resulted in large differences between families that were consistent between sites and at two times; but in Australia, where more field challenges have been attempted, the results have been mixed. In some challenges the OsHV-1 virus has been present but mortality was not observed, and in others mortality was observed with no virus detected (M. Dove and W. O'Connor (New South Wales Ministry of Primary Industries) and P. Kube (CSIRO), pers. comm. to MDC). Because molluscan cell lines are not available to culture the virus, developing a standardised live-virus assay is challenging. However, collaborative efforts between New Zealand and Australia have made substantial progress towards amplification of the virus in live animals and its purification and long-term storage for laboratory-based challenge experiments (P. Kirkland, NSW Department of Primary Industries, pers. comm. to MDC).
Another challenge for selecting for OsHV-1 resilience is the potential confounding of family-specific common environmental effects with genetic effects, especially in the hatchery and nursery when families are raised separately in unreplicated larval tanks (Fjalestad Citation2005). The oyster larval rearing and nursery protocols employed before 2013 included a combination of intense size-based screening of larvae and reactive tank-specific husbandry practices such as adjusting food levels based on tank/family-specific larval density or biomass and treating problems such as bacterial outbreaks on a tank-specific basis. Size-based screening biases within-family genetic variance in family-specific ways, potentially complicating comparisons between families, especially if the relevant traits (e.g. OsHV-1 susceptibility) are measured on small animals. Family-specific larval rearing procedures can also complicate comparisons if this variation in environmental conditions induces physiological or biochemical variation that affects OsHV-1 resilience. The breeding programme is currently working on modifications to the larval rearing procedures to minimise these potential problems.
In a larger context, the fact that a majority of the oyster producers are not equipped to grow single-seed oysters in bags or baskets makes it challenging to deliver hatchery-reared oyster seed to their stick-based farms. So-called ‘remote setting’ of hatchery-reared larvae onto hard substrates by oyster farmers on or near their farm sites is common in some countries (e.g. USA, France), but not in New Zealand where the required infrastructure does not yet exist. As well, whereas epinephrine-induced setting of larvae in the hatchery can achieve very high setting rates (80%–90% is not unusual), remote setting is much less efficient. Typically, about 5%–20% of the eyed larvae shipped from a hatchery successfully set, making it necessary to produce approximately an order of magnitude more competent larvae to fully stock a farm through remote setting compared to single seed, but it is also worth noting that this approach eliminates the need for costly and complicated upwelling nursery systems.
Finally, the severity of the economic impacts of OsHV-1 on the New Zealand oyster industry are imposing challenging time constraints. Many oyster farms are small, family-owned businesses that cannot survive for long under the current circumstances, making it paramount that the breeding programme develops an oyster strain with adequate survival in OsHV-1 infected sites to be economically viable very quickly.
Progress to date
Before the onset of OsHV-1, the pragmatic approach of selecting for aesthetic qualities using semi-quantitative assessment criteria was quite successful. While the use of subjective criteria that change over time precludes quantitative analyses of genetic gains, it is clear that the minority of growers who produced single-seed oysters from selectively-bred seed were satisfied that this approach enhanced the quality and marketability of their product. Somewhat paradoxically, however, the companies that process live oysters into the frozen half-shell product which dominates the export market pay the same farm-gate prices for selectively-bred single-seed oysters and wild-caught spat grown on sticks, providing little incentive for the majority of growers to invest in single-seed growout systems and buy, rather than collect, seed oysters. However, because OsHV-1 has dramatically reduced wild spat collection, the situation is rapidly changing.
It is still too early to evaluate genetic gain for OsHV-1 resilience. Only one cohort of families, produced in 2011, has been evaluated using a field challenge. Encouragingly, these families showed a wide range of mortality that was consistent across two test sites and two test dates (). However, a preliminary genetic analysis produced a lower than expected heritability for survival in these challenges (0.18), a larger than expected non-additive genetic proportion of total variance (0.35), and a fairly strong negative genetic correlation between survival and growth (–0.55). These estimates, however, are likely to be compromised by common environmental effects in the hatchery and nursery (discussed above). A second cohort of families was produced in late 2013 and will be challenged in the field (and hopefully in the laboratory) in early 2014. These challenges will tell us if crosses between parents from high-surviving families in the 2011 cohort produce high-surviving progeny. If the negative genetic correlation is verified this would imply that improving survival will result in a reduction in growth rate.
Future prospects
The future of the New Zealand oyster industry is uncertain. If OsHV-1 continues to kill the vast majority of wild-caught oyster spat, the only way to re-stock currently empty farms will be to use hatchery-reared seed capable of surviving at sufficient rates to make them profitable. One company (Pacific Marine Farms) has adopted a strategy of two-staged production in which single-seed oysters are grown to intermediate size at a disease-free South Island site during the summer months when the virus is active at North Island sites and then transferred to North Island in winter when the virus is dormant. Some of these oysters reach harvest size before the next disease outbreak and those that do not generally have higher survival rates than smaller animals.
This strategy, however, is not viable for smaller companies that do not have access to South Island sites nor the financial resources to acquire such access and to gear up for the transition from stick farming to growing oysters in bags or baskets. These farmers will only survive if wild spatfall recovers or the breeding programme can produce an oyster strain that can be set remotely and survive exposure to OsHV-1 at an early age. The main objective of the breeding programme, therefore, must be to enhance survival of very young spat.
Pāua (abalone)
History
There is a high demand worldwide for the seafood delicacy known in New Zealand as pāua, and elsewhere as abalone. A decline in wild stocks due to overfishing and poaching means that the world market for this gastropod is undersupplied. In New Zealand, the black-footed pāua (Haliotis iris) is commercially fished, and farming, albeit mostly on small scale, has been conducted since the 1980s. Pāua farming has excellent potential for further development in New Zealand because of strong overseas demand and high prices. Currently, OceaNZ Blue (OB) is the largest commercial company, producing approximately 100 tonnes per annum (www.oceanzblue.co.nz). OB supply pāua over 75 mm in length to international markets and specialise in high quality canned and cryogenically frozen products.
In the 1980s, NIWA scientists developed techniques for spawning and hatching pāua, and it became possible to control mating and set up specific crosses for evaluation (Moss Citation1998). An industry led initiative in 2003 started a broodstock development programme, and in 2007 this developed into a family-based selective breeding programme (Symonds & Heath Citation2008) funded initially by the Foundation for Research Science and Technology (FRST) and now through NIWA's core funding for aquaculture supported by MBIE. OB also operate an in-house individual selection programme incorporating microsatellite DNA based genotyping to avoid inbreeding.
Breeding objectives
A key issue for pāua farmers is slow growth: it can take over 4 years for a cultured pāua to reach current market size of 70+ mm. Growth tends to decline in individuals over 50–60 mm so growth from this size to marketable size is very important (Roberts Citation2011). Pāua are sold as two main products: whole pāua sold live or frozen; or whole canned pāua. For the whole pāua market, the growth of the whole animal (shell and total body weight) is important, whereas for the canned product, bled meat weight and yield are important.
Other traits of economic importance include FCE (food is the second largest cost for OB; Roberts Citation2011), survival and general robustness, environmental tolerance (in particular to warmer temperatures), foot darkness and shell colour.
Breeding strategy
There are two main breeding strategies currently employed: (1) family-based selective breeding for multiple traits (NIWA/OB collaborative programme); and (2) individual broodstock selection based on the phenotype and age of the breeding individual (e.g. OB's in-house programme).
The family-based breeding programme has two main aims: (1) supply of selectively bred stock to the industry; and (2) the evaluation of quantitative genetic parameters, heritability and phenotypic and genetic correlations among priority traits. In addition, for future breeding programme design, it is important to estimate the influence of early rearing effects and environment on family performance. This included assessing the feasibility and impact of rearing families pooled during early rearing versus rearing families separately up to tagging. The pooled groups enabled the confounding effect of family and rearing tank to be separated in the statistical analysis. Families were also reared in two different environments: NIWA's cool water Mahanga Bay facility and in OB's warmer water commercial facility in Northland.
The family programme was established in 2007 and opted to use wild broodstock from three different populations around New Zealand (Chatham Islands, Tory Channel and Kaikoura) and a small number of selected F1 broodstock, to increase genetic variation in the founding population. This was based on evidence that New Zealand wild pāua populations are moderately genetically differentiated (Smith Citation2008; Will et al. Citation2011).
The OB in-house programme tracks multiple similar-aged cohorts through their commercial production facility and selects the fastest growing individuals for future breeding, providing a high selection intensity. The selected broodstock are genotyped and relatedness among the broodstock is estimated (Wang Citation2002). Only unrelated individuals are used to establish crosses. The growth improvement in the F3 generation has been very encouraging (L. Suvalko, OceaNZ Blue, pers. comm. to JES, 13 December 2013).
Special issues, challenges and opportunities
The slow growth of pāua means families have to be reared for 9–14 months before they reach a mean shell length of c. 15–20 mm and can be tagged using spring tags. This increases the risk of introducing early environmental effects due to the confounding effect of tank environment and family. Despite efforts to equalise family numbers and density, early differential survival and growth post-settlement make this difficult. The resulting highly variable number of surviving individuals per family impacts density and has a significant influence on the mean length of the families post tagging (Symonds et al. Citation2012b).
Pooling the families (at fertilisation, hatching or at larval settlement) reduces early environmental effects, but can result in very uneven family contributions due to differential survival and competition (Symonds et al. Citation2012b). Despite the issues, pooling remains a feasible alternative to separate family rearing, as many more families can be established at one time. Genotyping costs, although considerable, may also be less than the infrastructure and labour required to rear sufficient families separately for 9–14 months.
The slow growth of the pāua also impacts the time it takes to reach sexual maturation, which can be 4 to 5 years. Such a long generation time slows the rate of genetic progress. Spawning has been unpredictable in the F1 and F2 generations, especially for the males. However, improved broodstock conditioning and knowledge of broodstock rearing requirements have reduced this problem for OB (L. Suvalko (OceaNZ Blue), pers. comm. to JES, 13 December 2013). Selective breeding provides a significant opportunity, not only for reducing the time to reach market size, but also to increase genetic progress by reducing the generation time.
Progress to date
Family-based selection
From 2007 to 2009 a total of 101 individual family crosses were established. The total number of parents in the analysis comprised 43 males and 41 females that produced 95 surviving families and a total of 29,069 offspring. Overall, 92% of the parents produced at least two families. Five parents were reused across the three groups to create genetic linkage between the year classes.
Measurements on individual tagged pāua were conducted biannually from tagging onwards, usually in late autumn and late spring to capture the end of the summer and winter growth periods. Standard assessments included shell length and total weight. Later measurements also included gonad development, foot darkness and shell colour. Harvest evaluation was completed on 20 to 34 individuals per family of appropriate harvest size. Harvest traits included whole weight, shell weight, total meat weight, bled meat weight and meat yield.
Growth rates (µm/day) between assessments were also included in the trait analysis, including estimated time (days) to reach 70 mm, an important economic trait given the slow growth of pāua (). The heritabilities were high for all traits measured, including length and weight traits, even at the earlier assessments when early rearing effects are likely to result in high environmental variance. Bled meat and harvest meat yield traits had low to moderate negative genetic correlations with the growth traits, which suggests that the shell is growing at a faster rate than the meat. Overall, the results indicate that high rates of response to selection for increased weight or length can be expected. The EBVs for the trait ‘days to 70 mm’ have been used to predict the reduction in time to harvest as a result of combined family selection and indicate that total production time could be reduced by over 6 months. This represents a substantial economic benefit to OB.
Table 3 Pāua summary statistics including heritability (h2). Genetic parameter analysis completed by AbacusBio Ltd.
Future prospects
Opportunities for improving pāua growth through selective breeding, and thus reducing the time to market or increasing market size, are good. Through discussion with OB the next stage will involve developing a multi-trait selection index to identify elite broodstock. These high-performance broodstock are valuable assets and will be used to create the next generation of offspring for farming and for future selective breeding. The combined genetic and biological information obtained from the NIWA/OB family programme will be used to design the most appropriate breeding programme for OB's commercial application.
Conclusions
Aquaculture is the fastest growing sector of agricultural production in New Zealand, and farmed king salmon, GreenshellTM mussels, Pacific oysters and pāua currently produce significant revenue. Selective breeding programmes are now in place for all four established species either through centralised programmes or in-house programmes operated by the larger companies. Broodstock management and selection programmes are also underway for newly emerging species hāpuku and kingfish. The main approach is combined family pedigree based selection with the focus on the production of families and evaluation of multiple commercially valuable traits. Significant progress has been made through the development of strategies to reduce early rearing effects and better understand the influence of G×E interactions. Defining the traits to be selected, and their relative economic value, continues to evolve, especially for oysters in response to the mortality caused by the oyster herpes virus. All programmes have verified the significant potential for genetic improvement and the moderate to high heritabilities for many of the production traits evaluated are encouraging. Significant economic gains through selection have been demonstrated in established salmon breeding programmes and have been estimated for traits such as reduced time to harvest (pāua) and improved FCE (salmon). Molecular tools are also being developed and the future will likely see a combination of traditional and genome wide selection approaches for some species. Selective breeding is now well established in New Zealand aquaculture and will continue to provide the industry with improved stocks into the foreseeable future.
Acknowledgements
Nick King and Achim Janke generously provided MDC with data from and information about the histories of the oyster and mussel breeding programmes at the Cawthron Institute on which this review is based. The technical staff of the Cawthron Aquaculture Park did all the hard work of producing the animals and collecting those data. MDC also wishes to thank New Zealand mussel and oyster growers and processors and the staff of SPATnz for their help and guidance to understand New Zealand's shellfish farming industries and their perspectives on genetic improvement efforts, as well as their generous co-funding for these programmes. Most of the work on mussels and oysters summarised in this paper was supported by a contract between the Cawthron Institute and the New Zealand Ministry of Business Innovation and Employment entitled ‘Adding Value to New Zealand's Cultured Shellfish Industry: Maximising Profit, Minimising Risk’ (contract # CAWX0802). JES is grateful to the New Zealand salmon farmers for sharing information about the current status of their broodstock management, selective breeding programmes and future goals. JES thanks staff at NIWA's Bream Bay Aquaculture Park and Mahanga Bay facility for their technical assistance and scientific input, GenomNZ (AgResearch) for the pāua, hāpuku and kingfish genotyping and AbacusBio Ltd for the pāua genetic parameter analysis and genetics input. In addition, collaboration with OceaNZ Blue made the pāua selective breeding programme possible in conjunction with funding received through the National Institute of Water and Atmospheric Research Aquaculture Programme 3 (2012/13 SCI; 2013/14 SCI). Funding for the hāpuku and kingfish broodstock development and salmon FCE research was received through the National Institute of Water and Atmospheric Research Aquaculture Programmes 1 and 3.
References
- Amer P, Jopson N, Dodds K, Symonds J, Hills J 2001. Optimal utilization of genetic resources in aquaculture. Proceedings of the New Zealand Society of Animal Production 61: 35–37.
- Amer PR, Symonds JE 2000. Practical application of an inbreeding control algorithm for salmon. In: Benzie JAH ed. Genetics in aquaculture VII: proceedings of the seventh International Symposium on Genetics in Aquaculture, held in Townsville, Qld, 15–22 July 2000. Amsterdam: Elsevier. Abstracts, p. 3.
- Bailey J 2011. New Zealand king salmon applied breeding. Proceedings of the Centre for Reproduction and Genomics Aquaculture Workshop, 10 June 2011. Invermay, New Zealand, AgResearch. Pp. 28–30.
- Bartley D, Rana K, Immink A 2001. The use of inter-specific hybrids in aquaculture and fisheries. Reviews in Fish Biology and Fisheries 10: 325–337. 10.1023/A:1016691725361
- Bell JG, Pratoomyot J, Strachan F, Henderson RJ, Fontanillas R, Hebard A et al. 2010. Growth, flesh adiposity and fatty acid composition of Atlantic salmon (Salmo salar) families with contrasting flesh adiposity: effects of replacement of dietary fish oil with vegetable oils. Aquaculture 306: 225–232. 10.1016/j.aquaculture.2010.05.021
- Berg P, Nielsen J, Sørensen MK 2006. EVA: Realized and predicted optimal genetic contributions. CD communication 27–09, 2 pp. WCGALP, 2006, s.246.
- Camacho A, Abella T, Tayamen M 2001. Fish genetics research and development in the Philippines. In: Gupta MV AB ed. Genetics in aquaculture: ICLARM Conference Proceedings 64. Penang, Malaysia, International Center for Living Aquatic Resources Management. Pp. 71–76.
- Camara MD, Evans S, Langdon CJ 2008. Parental relatedness and survival of Pacific oysters from a naturalized population. Journal of Shellfish Research 27: 323–336. 10.2983/0730-8000(2008)27[323:PRASOP]2.0.CO;2
- Clarke S, Brauning R, Fisher PHB, Dodds K, Bailey J, Walker S et al. 2011. Proceedings of the Centre for Reproduction and Genomics Aquaculture Workshop, 10 June 2011. Invermay, New Zealand, AgResearch. Pp. 31–39.
- Collard BCY, Jahufer MZZ, Brouwer JB, Pang ECK 2005. An introduction to markers, quantitative trait loci (QTL) mapping and marker-assisted selection for crop improvement: the basic concepts. Euphytica 142: 169–196. 10.1007/s10681-005-1681-5
- Dégremont L, Bédier E, Boudry P 2010. Summer mortality of hatchery-produced Pacific oyster spat (Crassostrea gigas). II. Response to selection for survival and its influence on growth and yield. Aquaculture 299: 21–29. 10.1016/j.aquaculture.2009.11.017
- Devlin R, McNeil B, Groves T, Donaldson E 1991. Isolation of a Y-chromosomal DNA probe capable of determining genetic sex in Chinook salmon (Oncorhynchus tshawytscha). Canadian Journal of Fisheries and Aquatic Sciences 48: 1606–1612. 10.1139/f91-190
- Doyle RT, Herbinger C 1994. The use of DNA fingerprinting for high-intensity, within-family selection in fish breeding. Proceedings of the Fifth World Congress of Genetics Applied to Livestock Production. Pp. 364–371.
- Du S, Devlin R, Hew C 1993. Genomic structure of growth hormone genes in Chinook salmon (Oncorhynchus tshawytscha): presence of two functional genes, GH-I and GH-II, and a male-specific pseudogene, GH-psi. DNA Cell Biology 12: 739–751. 10.1089/dna.1993.12.739
- Elshire RJ, Glaubitz JC, Sun Q, Poland JA, Kawamoto K, Buckler ES et al. 2011. A robust, simple genotyping-by-sequencing (GBS) approach for high diversity species. PLoS ONE 6: e19379. 10.1371/journal.pone.0019379
- Evans F, Matson S, Brake J, Langdon C 2004. The effects of inbreeding on performance traits of adult Pacific oysters (Crassostrea gigas). Aquaculture 230: 89–98. 10.1016/j.aquaculture.2003.09.023
- Falconer DS 1989. Introduction to quantitative genetics. New York, Longman.
- Falconer DS 1990. Selection in different environments: effects on environmental sensitivity (reaction norm) and on mean performance. Genetical Research 56: 57–70. 10.1017/S0016672300028883
- Fjalestad KT 2005. Selection methods. In: Gjedrem T ed. Selection and breeding programs in aquaculture. Dordrecht, Springer. Pp. 159–171.
- Fjalestad KT, Moen T, Gomez-Raya L. 2003. Prospects for genetic technology in salmon breeding programmes. Aquaculture Research 34: 397–406. 10.1046/j.1365-2109.2003.00823.x
- Gall G, Huang N 1988. Heritability and selection schemes for rainbow trout: body weight. Aquaculture International 73: 43–56. 10.1016/0044-8486(88)90040-3
- Garber A, Sullivan C 2006. Selective breeding for the hybrid striped bass (Morone chrysops, Rafinesque x M. saxatilis, Walbaum) industry: status and perspectives. Aquaculture Research 37: 319–338. 10.1111/j.1365-2109.2005.01439.x
- Gjedrem T 2000. Genetic improvement of cold-water fish species. Aquaculture Research 31: 25–33. 10.1046/j.1365-2109.2000.00389.x
- Gjedrem T 2005. Status and scope of aquaculture. In: Gjedrem T ed. Selection and breeding programs in aquaculture. Dordrecht, Springer. Pp. 1–8.
- Gjedrem T 2010. The first family-based breeding program in aquaculture. Reviews in Aquaculture 2: 2–15. 10.1111/j.1753-5131.2010.01011.x
- Gjerde B, Simianer H, Refstie T 1994. Estimates of genetic and phenotypic parameters for body weight, growth rate, and sexual maturity in Atlantic salmon. Livestock Production Science 38: 133–143. 10.1016/0301-6226(94)90057-4
- Gjøen H, Gjerde B 1998. Comparing breeding schemes using individual phenotypic values and BLUP breeding values as selection criteria. In: Proceedings of the 6th World Congress on Genetics Applied to Livestock Production (WCGALP). Armidale, NSW, Australia, WCGALP. Pp. 111–114.
- Goddard ME 2009. Genomic selection: prediction of accuracy and maximisation of long term response. Genetica 136: 245–257. 10.1007/s10709-008-9308-0
- Harris DL 1998. Livestock improvement: art, science, or industry? Journal of Animal Science 76: 2294–302.
- Hedgecock D, McGoldrick DJ, Bayne BL 1995. Hybrid vigor in Pacific oysters: an experimental approach using crosses among inbred lines. Aquaculture 137: 285–298. 10.1016/0044-8486(95)01105-6
- Henderson CR, Quaas RL 1976. Multiple trait evaluation using relatives' records. Journal of Animal Science 43: 1188–1197.
- Hulata G 1995. A review of genetic improvement of the common carp (Cyprinus carpio L.) and other cyprinids by crossbreeding, hybridization and selection. Aquaculture International 129: 143–155. 10.1016/0044-8486(94)00244-I
- Hunter G, Donaldson E, Stoss J, Baker I 1983. Production of monosex female groups of chinook salmon (Oncorhynchus tshawytscha) by the fertilization of normal ova with sperm from sex-reversed females. Aquaculture International 33: 355–364. 10.1016/0044-8486(83)90414-3
- King N, Janke A 2006. Oyster breeders look for quality and quantity. New Zealand Aquaculture 11: 10–12.
- King N, Janke A, Kaspar H, Foster S 2005. An intensive low volume larval rearing system for the simultaneous production of many families of the Pacific oyster Crassostrea gigas. Larvi ‘05-Fish & Shellfish Larviculture Symposium. Oostende, Belgium, European Aquaculture Society. Pp. 236–237.
- Kinghorn B 2011. An algorithm for efficient constrained mate selection. Genetics Selection Evolution 43: 4. 10.1186/1297-9686-43-4
- Kohn Y, Symonds J 2012. Evaluation of egg quality parameters as predictors of hatching success and early larval survival in hapuku (Polyprion oxygeneios). Aquaculture International 342–343: 42–47. 10.1016/j.aquaculture.2012.02.014
- Kolstad K, Thorland I, Refstie T, Gjerde B 2006a. Body weight, sexual maturity, and spinal deformity in strains and families of Atlantic cod (Gadus morhua) at two years of age at different locations along the Norwegian coast. ICES Journal of Marine Science 63: 246–252. 10.1016/j.icesjms.2005.11.007
- Kolstad K, Thorland ITR, Gjerde B 2006b. Genetic variation and genotype by location interaction in body weight, spinal deformity and sexual maturity in Atlantic cod (Gadus morhua) reared at different locations off Norway. Aquaculture International 259: 66–73. 10.1016/j.aquaculture.2005.12.022
- Lane H 2013. Characterisation of the mitochondrial genome and the population genetics of Polyprion oxygeneios (hapuku) from around New Zealand. Unpublished thesis. Wellington, Victoria University.
- Langdon C, Evans F, Jacobson D, Blouin M 2003. Yields of cultured Pacific oysters Crassostrea gigas Thunberg improved after one generation of selection. Aquaculture 220: 227–244. 10.1016/S0044-8486(02)00621-X
- Launey S, Hedgecock D 1999. Genetic load causes segregation distortion in oysters: mapping at 6 hours. International Conference on the Status of Plant and Animal Genome Research VII, San Diego, CA. Abstracts, p. 33.
- Launey S, Hedgecock D 2001. High genetic load in the Pacific oyster Crassostrea gigas. Genetics 159: 255–265.
- Lurman GJ, Hilton Z, Ragg NLC 2013. Energetics of byssus attachment and feeding in the green-lipped mussel Perna canaliculus. The Biological Bulletin 224: 79–88.
- Lynch M, Walsh B 1998. Genetics and analysis of quantitative traits. Sunderland, Sinauer Associates Inc. Pp. xiii + 980 p.
- Marine Harvest 2012. The Marine Harvest salmon industry handbook. Bergen, Norway, Marine Harvest. 72 p.
- McDowall R 1994. The origins of New Zealand's Chinook salmon, Oncorhynchus tshawytscha. Marine Fisheries Review 56: 1–7.
- Meuwissen T, Luo Z 1992. Computing inbreeding coefficients in large populations. Genetics Selection Evolution 24: 305–313. 10.1186/1297-9686-24-4-305
- Meuwissen TH 1997. Maximizing the response of selection with a predefined rate of inbreeding. Journal of Animal Science 75: 934–940.
- Meuwissen THE, Hayes BJ, Goddard ME 2001. Prediction of total genetic value using genome-wide dense marker maps. Genetics 157: 1819–1829.
- Morais S, Silva T, Cordeiro O, Rodrigues P, Guy DR, Bron JE et al. 2012. Effects of genotype and dietary fish oil replacement with vegetable oil on the intestinal transcriptome and proteome of Atlantic salmon (Salmo salar). BMC Genomics 13: 448. 10.1186/1471-2164-13-448
- Moss G 1998. Effect of temperature on the breeding cycle and spawning success of the New Zealand abalone, Haliotis australis. New Zealand Journal of Marine and Freshwater Research 32: 139–146. 10.1080/00288330.1998.9516813
- Nguyen N, Ponzoni R, Hamzah A, Yee H, Abu-Bakar K, Khaw H 2010. Genetics of flesh quality in fish. In: Proceedings of the 9th World Congress on Genetics Applied to Livestock Production (WCGALP). Leipzig, Germany, WCGALP.
- Nguyen TTT, Hayes BJ, Ingram BA 2014. Genetic parameters and response to selection in blue mussel (Mytilus galloprovincialis) using a SNP-based pedigree. Aquaculture 420–421: 295–301. 10.1016/j.aquaculture.2013.11.021
- Nugroho E, Ferrell DJ, Smith P, Taniguchi N 2001. Genetic divergence of kingfish from Japan, Australia and New Zealand inferred by microsatellite DNA and mitochondrial DNA control region markers. Fisheries Science 67: 843–850. 10.1046/j.1444-2906.2001.00331.x
- Odegard J, Baranski M, Gjerde B, Gjedrem T 2011. Methodology for genetic evaluation of disease resistance in aquaculture species: challenges and future prospects. Aquaculture Research 42: 103–114. 10.1111/j.1365-2109.2010.02669.x
- Ragg NLC, King N, Watts E, Morrish J 2010. Optimising the delivery of the key dietary diatom Chaetoceros calcitrans to intensively cultured Greenshell™ mussel larvae, Perna canaliculus. Aquaculture 306: 270–280. 10.1016/j.aquaculture.2010.05.010
- Roberts R 2011. Abalone (paua) selective breeding: a commercial perspective. Proceedings of the Centre for Reproduction and Genomics Aquaculture Workshop, 10 June 2011. Invermay, New Zealand, AgResearch. Pp. 41–44.
- Smith P 2008. Population biology and genetics of paua, hapuku and kingfish: sourcing fish for broodstock development. Technical Report 132. 44 p.
- Sonesson AK, Gjerde B, Meuwissen THE 2005. Truncation selection for BLUP-EBV and phenotypic values in fish breeding schemes. Aquaculture 243: 61–68. 10.1016/j.aquaculture.2004.09.024
- Sonesson AK, Gjerde B, Robinson N 2011. A simple selection scheme to improve disease resistance and growth. Aquaculture 319: 337–341. 10.1016/j.aquaculture.2011.07.009
- Symonds J, Heath P 2008. Getting picky with paua: selective breeding to improve productivity. Water & Atmosphere 16: 20–21.
- Symonds J, Walker S, Amer P, Dodds K 2000. Selective breeding for improved performance of farmed New Zealand Chinook salmon. In: Benzie JAH ed. Genetics in aquaculture VII: proceedings of the seventh International Symposium on Genetics in Aquaculture, held in Townsville, Qld, 15–22 July 2000. Amsterdam: Elsevier. P. 115.
- Symonds J, Walker S, van de Ven I, Marchant A, Irvine G, Pether S et al. 2012a. Developing broodstock resources for farmed marine fish. Proceedings of the New Zealand Society of Animal Production 72: 222–226.
- Symonds J, Moss G, Jopson N, Roberts R, Birss J, Heath P et al. 2012b. Family-based selective breeding of New Zealand abalone, Haliotis iris: challenges and opportunities. Proceedings of the New Zealand Society of Animal Production 72: 216–221.
- Tacon A, Metian M 2008. Global overview on the use of fish meal and fish oil in industrially compounded aquafeeds: trends and future prospects. Aquaculture International 285: 146–158. 10.1016/j.aquaculture.2008.08.015
- Unwin M, Rowe D, Poortenaar C, Boustead N 2005. Suppression of maturation in 2-year-old Chinook salmon (Oncorhynchus tshawytscha) reared under continuous photoperiod. Aquaculture International 246: 239–250. 10.1016/j.aquaculture.2005.01.022
- Walker SP, Ingram M, Bailey J, Dodds KG, Fisher PJ, Amer PR et al. 2012. Chinook salmon (Oncorhynchus tshawytscha) feed conversion efficiency: evaluation and potential for selection. Proceedings of the New Zealand Society of Animal Production 72: 227–230.
- Wang J 2002. An estimator for pairwise relatedness using molecular markers. Genetics 160: 1203–1215.
- Whatmore P, Nguyen NH, Miller A, Lamont R, Powell D, D'Antignana T et al. 2013. Genetic parameters for economically important traits in yellowtail kingfish Seriola lalandi. Aquaculture 400–401: 77–84. 10.1016/j.aquaculture.2013.03.002
- Will M, Hale M, Schiel D, Gemmell N 2011. Low to moderate levels of genetic differentiation detected across the distribution of the New Zealand abalone, Haliotis iris. Marine Biology 158: 1417–1429. 10.1007/s00227-011-1659-x