Abstract
Although protein interaction studies are instrumental in understanding protein networks, most protein interaction techniques depend on use of sophisticated and expensive equipment. This makes it difficult for under-resourced laboratories to conduct protein–protein interaction studies. As such, we sought to explore the prospects of using ELISA and slot blot as alternate methods for analyzing protein–protein interactions in resource-limited settings. We used these two methods to explore the well established interaction of heat shock proteins (Hsps) of Plasmodium falciparum as a model. P. falciparum Hsp70-1 (PfHsp70-1) is a cytosol-nuclear localised molecular chaperone that interacts with several functional partners including P. falciparum Hsp70-Hsp90 organising protein (PfHop), P. falciparum Hsp40 (PfHsp40) and P. falciparum Hsp70-z (PfHsp70-z). To validate the application of ELISA and slot blot techniques in protein–protein studies, we employed these two techniques to explore the interaction of recombinant PfHsp70-1 with its partners. We further used the two techniques to explore the effects of mutating residues located in the GGMP repeat and linker motifs of PfHsp70-1 on the chaperone’s interaction with its functional partners. We established that despite requiring much larger amounts of protein compared to the more sensitive assays, the ELISA and slot blot assays were capable of detecting both nucleotide- and mutation-driven changes regulating the affinity of PfHsp70-1 for its interactors. Our findings highlight the utility of these two techniques under resource constraints in conducting routine protein–protein interaction studies and their possible application in the preliminary screening of inhibitors targeting protein networks.
1. INTRODUCTION
Various proteins participate in cellular processes in complex with other proteins. As such, the study of protein networks is an integral part towards understanding their function. In addition, protein–protein interactions (PPIs) are increasingly being targeted in intervention efforts against several communicable and non-communicable diseases. In light of this, several techniques routinely used to study PPIs receive sustained interest (reviewed in Berggård et al., Citation2007; Miura, Citation2018). However, most of these methods are tedious and inappropriate for routine analysis. In addition, not all of the methods provide detailed information on the kinetics of the PPIs. One of the most robust methods used to study PPIs in vitro is surface plasmon resonance (SPR) analysis (Nguyen et al., Citation2015) as it is quite sensitive and provides data on the kinetics of PPIs. Despite its versatility, the SPR instrument is highly expensive.
Enzyme-linked immunosorbent assay (ELISA) and slot blot approach are comparably cheaper assays that have previously been employed to conduct protein–protein interaction studies (Mabate et al., Citation2018; Makumire et al., Citation2021). Because of their possible utility in low-cost settings, it is important to ascertain their reliability in protein–protein analysis. To address this, we conducted ELISA and slot blot assays to demonstrate the interaction of the molecular chaperone, Plasmodium falciparum Hsp70-1 (PfHsp70-1) (Shonhai et al., Citation2007; Citation2008) with its functional partners, P. falciparum Hsp40 (PfHsp40; Botha et al., Citation2007); P. falciparum Hsp70-Hsp90 organising protein (PfHop; Gitau et al., Citation2012) and P. falciparum Hsp70-z (PfHsp70-z; Zininga et al., Citation2016). We further explored the utility of both ELISA and slot blot technique by comparing the binding capabilities of PfHsp70-1 (wild type) relative to its linker (Chakafana et al., Citation2021) and GGMP mutants (Makumire et al., Citation2021). Our findings suggest that the two assays are reproducible and may be ideal for routine use in low budget settings. In addition, our findings suggest that ELISA could be amenable to high throughput screening of small molecules targeting PPIs.
2. MATERIALS AND METHODS
2.1. Recombinant proteins and antibodies
The following recombinant proteins were produced as previously described: PfHsp70-1 (wild type); GGMP mutants of PfHsp70-1 (PfHsp70-1G632 and PfHsp70-1G648) (Makumire et al., Citation2021); the nucleotide-binding domain of PfHsp70-1 (PfHsp70-1NBD) (Zininga et al., Citation2016), the linker mutant of PfHsp70-1 (PfHsp70-1LS) (Chakafana et al., Citation2021); PfHop (Gitau et al., Citation2012) and PfHsp70-z (Zininga et al., Citation2016). BSA (Sigma Aldrich, USA) was used as a non-chaperone control. The following antibodies were used for slot blot and ELISA: α-PfHsp70-1 (Shonhai et al., Citation2008); α-PfHop (Gitau et al., Citation2012); α-PfHsp70-z (Zininga et al., Citation2015a); goat raised α-rabbit HRP conjugates (Abcam, USA) and α-PfHsp40 (Lebepe et al., Citation2020).
2.2. Enzyme-linked immunosorbent assays
ELISA was employed to investigate the interaction of wild type PfHsp70-1 or its mutants (PfHsp70-1G632, PfHsp70-1G648, PfHsp70-1LS) with PfHop, PfHsp40 and PfHsp70-z. The ligands (PfHsp70-1/its mutants) were immobilised onto 96 well plates at a concentration of 5 µg/ml [0.068 µM]. A non-chaperone ligand, BSA, was also immobilised at 5 µg/ml [0.073 µM] as a negative control.
To allow for noncovalent immobilisation of respective ligands onto the well surfaces, each protein was prepared in 5 mM sodium bicarbonate (NaHCO3) at pH 9.5 and incubated overnight at 4 °C. The plates were subsequently washed once using Tris-buffered saline (TBS; 20 mM, pH 7.5, 500 NaCl) with 0.1% Tween-20 (TBST) to remove unbound protein. The wells were then blocked with 150 µL of 5% fat-free milk in TBST added to each well and incubated at 25°C for 1 hr. After blocking, the wells were washed with TBST thrice for a total of 10 min. Serial dilutions of PfHop, PfHsp40, and PfHsp70-z (0-1000 nM), which were used as analytes in independent assays were suspended in binding buffer (2.5% fat-free milk in TBST). To allow for the ligand-analyte association, the plates were then incubated for 2 hr at 25 °C before being washed by TBST to remove unbound analytes. The respective primary antibodies (α-PfHop/ α-PfHsp40/ α-PfHsp70-z) were introduced at a final ratio of 1:4000. The assay mix was left to stand at 25 °C for 1 hr. The wells were washed to remove residual unbound primary antibodies. Secondary HRP conjugated goat-raised α-rabbit antibody (1: 4000) was then added and the plate was incubated for 45 min at 25 °C. The plate was washed three times prior to detection using 3,3′5,5′ –tetramethylbenzidine (TMB) (Bio Scientific, USA) at 100 µL/ well. The 96 well plate was incubated at room temperature (25 °C) for 2 min. To monitor colour development, a Spectra max M3 Microplate reader (Molecular Devices, USA) was used. Absorbance readings were measured at 370 nm at various time intervals (0, 5, 10, 15, 20, 25, and 30 min). The assay was repeated in the presence of 5 mM ATP/ADP.
The analysis of data was conducted factoring double background subtraction of absorbance values from uncoated and BSA negative control wells for each serial dilution of the analyte. The resultant absorbance values obtained at the highest concentration of each analyte were averaged as maximum (100%) binding. Titration curves were then generated against a log scale of dilution concentrations using GraphPad prism 6.05 (GraphPad Software, USA).
2.3. Slot blot analysis
The slot blot assay was conducted to explore the interaction of either PfHsp70-1 (wild type) or its linker/GGMP mutant forms with PfHop following a previously described protocol (Zininga et al., Citation2016) with minor modifications. Briefly, various amounts (0, 1, 2, 4 μg) of PfHsp70-1 or its mutants of either PfHsp70-1 or its mutant (GGMP and linker) were prepared in buffer TSK (25 mM Tris-HCl pH 7.4, 140 mM NaCl, and 3.0 mM KCl). BSA (4 μg) was used as a negative control. The bait proteins were immobilised onto the nitrocellulose membrane gently by vacuuming using the BioDot microfiltration apparatus (BioRad, USA). The membrane was blocked using 5% fat-free milk in buffer TSKT (TSK plus 0.1% Tween-20) and incubated for 1 hr at room temperature with gentle shaking. After blocking the membrane was washed with TSKT three times for 10 min. The membrane was overlaid with a constant concentration of 4 μg/ml of PfHop suspended in binding buffer (2.5% fat-free milk in TSKT) and assay mix was left to stand overnight at 4 °C. The membrane was washed to remove the unbound analyte. The bound analyte was detected by a primary antibody, α-PfHop (1:2000, (Gitau et al., Citation2012), and incubated for 1 hr. The unbound primary antibody was washed before the addition of the secondary antibody HRP conjugated goat-raised α-rabbit IgG (1:4000). Detection of protein bands was performed using ECL and visualization was done using the Chemidoc (Bio-Rad, USA). Densitometric analyses were performed using the Image Lab software (Bio-Rad, USA). The assay was repeated in the presence of 5 mM ATP or ADP.
3. RESULTS
3.1. ELISA and slot blot effectively detected nucleotide regulated interaction of PfHsp70-1 and its co-chaperone, PfHop
We previously demonstrated that PfHop interacts with PfHsp70-1 in a nucleotide-dependent fashion (Zininga et al., Citation2015b). Generally, PfHop associates with PfHsp70-1 with the least affinity in the presence of ATP compared to either the apo- or ADP states (Zininga et al., Citation2015b). In the current study, we observed a similar trend based on the ELISA () and slot blot assay (). In addition, both the ELISA and slot blot data demonstrated that higher signals for the interaction were generated with increasing levels of PfHop (Supplementary Figure S1). However, it should be noted that due to technical limitations, different slot blot strips were used to investigate the interaction of the two proteins under different nucleotide conditions (presence of ATP/ADP or absence of nucleotide). This may have skewed varied signals obtained under the different nucleotide conditions. However, the fact that the slot blot data obtained here are consistent with previously reported findings based on the use of the SPR technique to study PfHsp70-1-PfHop interaction (Zininga et al., Citation2015b) confirms the reliability of the slot blot approach.
Figure 1. ELISA is capable of detecting nucleotide dependent interaction of PfHop with PfHsp70-1. The ELISA representative binding curves for the interaction of PfHop with PfHsp70-1 GGMP and linker variants in the absence of nucleotide (NN) (A) and in the presence of 5 mM ATP (B) or 5 mM ADP (C). The resultant absorbance values obtained at the highest concentration of PfHop were averaged as the maximum (100%) binding for the protein. Bar graphs represent the relative intensity of binding between PfHsp70-1; PfHsp70-1G632, PfHsp70-1G648 and PfHsp70-1L with PfHop under the distinct nucleotide states normalised to PfHsp70-1 in the absence of nucleotides (D). Interaction of PfHop with the PfHsp70-1NBD was used as a baseline. The error bars represent standard errors of the mean obtained from three assays conducted using different batches of purified recombinant protein. Statistical analyses were conducted using a One-way ANOVA (p<0.01).
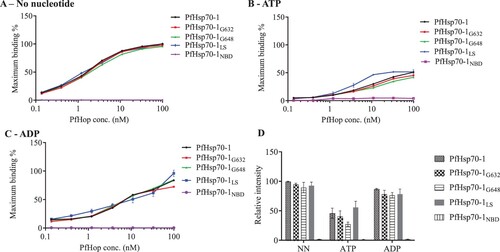
Figure 2. Slot blot data confirmed the compromised capability of the GGMP mutants of PfHsp70-1 to bind PfHop. Various quantities (1, 2, and 4 μg) of PfHsp70-1 or its GGMP mutants were spotted on the nitrocellulose membrane as prey protein. BSA (4 μg) was used as a negative control. Each respective protein concentration was spotted using a vacuum and overlaid with 4 μg of purified PfHop protein (A) and accompanying densitometry analysis. The assay was repeated in the presence of ADP or ATP (B and C) and accompanying densitometry analyses are shown on the right-hand side. α- PfHop antibody was used to detect the presence of bound PfHop. The data were generated from three independent assays. The broken rectangles represent the slot blot space onto which PfHop and BSA were loaded as controls while the panel labelled ‘’null’’ represents absence of loaded bait protein. Standard errors about the mean are indicated, and statistical analysis was conducted using two-way ANOVA (statistical significance at p<0.001* showing the variation of signals of GGMP derivatives of PfHsp70-1 versus wild type PfHsp70-1 with respect to their interaction with PfHop are shown).
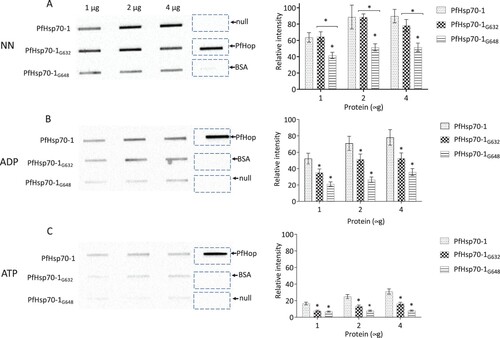
Using SPR analysis, we previously demonstrated that PfHsp70-1 linker and GGMP mutants bound PfHop with less affinity compared to the wild type PfHsp70-1 (Chakafana et al., Citation2021; Makumire et al., Citation2021). Based on the ELISA data (), PfHsp70-1 and its linker or GGMP mutants bound PfHop with distinguishable affinities only in the presence of ATP. On the other hand, the slot blot data () demonstrated that PfHsp70-1G632 and PfHsp70-1G648 had less affinity for PfHop than wild type PfHsp70-1 irrespective of nucleotide status. Typically, the affinity of PfHsp70-1 for PfHop is higher in the presence of ADP than in the presence of ATP (Gitau et al., Citation2012; Zininga et al., Citation2015b). In addition, in the absence of nucleotide, PfHsp70-1 bound to PfHop with fairly high affinity. This is consistent with the previously reported findings suggesting that apo-PfHsp70-1 and ADP-PfHsp70-1 exhibit higher affinity for PfHop than the ATP-PfHsp70-1 (Zininga et al., Citation2015b). Altogether, the slot blot assay was more effective in detecting the reduced propensity of GGMP mutants of PfHsp70-1 to bind PfHop than the ELISA (; 2). We validated the specificity of PfHsp70-1 interaction with PfHop based on both ELISA and slot blot assay using BSA which as expected, did not interact with PfHop ().
3.2. ELISA confirms the compromised propensity of the linker mutant of PfHsp70-1 to bind both PfHsp40 and PfHsp70-z
In a previous study (Chakafana et al., Citation2021), we demonstrated that a linker mutant of PfHsp70-1 (PfHsp70-1LS) harbouring the linker of another chaperone, PfHsp70-z was less effective at refolding luciferase in the presence of two functional partners (PfHsp40 and PfHsp70-z) (Chakafana et al., Citation2021). Using SPR analysis, we previously demonstrated that in the presence of ATP, PfHsp70-1LS lost its affinity for PfHsp40 by two orders of magnitude compared to wild type PfHsp70-1 (Chakafana et al., Citation2021). In the current study (A-D), we observed the same trend using ELISA (Supplementary Figure S2; S3). In addition, using ELISA, we demonstrated that PfHsp70-1LS had a lower affinity for PfHsp70-z than wild type PfHsp70-1 (E-H).
Figure 3. ELISA confirms the compromised affinity of PfHsp70-1LS for both PfHsp40 and PfHsp70-z. Representative ELISA curves for the association between PfHsp40 and PfHsp70-1/PfHsp70-1LS based on assay conducted in the absence of nucleotides (A) or presence of either 5 mM ATP (B) or ADP (C). Bar graphs represent the comparative association of Hsp40 with PfHsp70-1/PfHsp70-1LS as determined by the relative maximum binding in each case compared to the maximum binding of the wildtype PfHsp70-1 in the absence of nucleotides (D). Representative ELISA curves for the association between PfHsp70-z with PfHsp70-1/PfHsp70-1LS in the absence of nucleotide (E), or presence of ATP (F) or ADP (G). Bar graphs represent the relative association of each respective protein as determined by comparing the intensities to the intensity of wildtype PfHsp70-1 in the absence of nucleotide (H). The data is an average from three independent ELISA runs. Statistical analyses were conducted using a one-way ANOVA with a p<0.01.
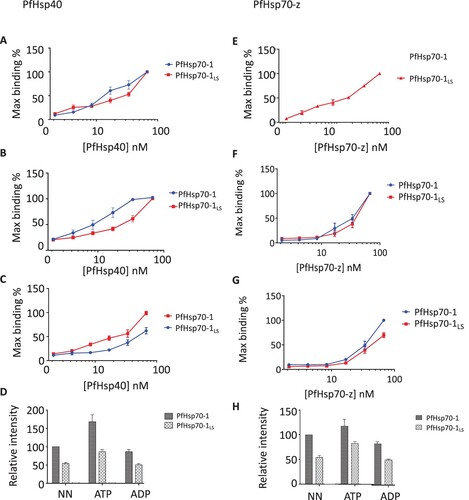
4. DISCUSSION
PPI studies are central to our understanding of the function of proteins, especially with respect to their participation in metabolic pathways. In addition, PPI based assays are crucial in drug development efforts. However, the limitations of conducting PPI assays include the high cost of reagents and instruments they require. In the current study, we investigated the application of slot blot and ELISA approaches as comparably cheaper and technically less demanding methods to study PPI. We explored the application of these two techniques on the well-established interaction of PfHsp70-1 and its co-chaperones (PfHop, PfHsp70-z and PfHsp40). In general, both assays confirmed the interaction dynamics of PfHsp70-1 and its co-chaperones as previously reported using reliable and sensitive assays such as SPR analysis (Zininga et al., Citation2015b; Chakafana et al., Citation2021; Makumire et al., Citation2021).
The sensitivity of both ELISA and slot blot assay was further demonstrated by the capability of both methods to detect the reduced affinity of linker and GGMP mutants of PfHsp70-1 for the co-chaperones. Thus, the current data confirmed similar findings as reported using SPR analysis (Zininga et al., Citation2015b; Chakafana et al., Citation2021; Makumire et al., Citation2021). It is encouraging to note that data obtained using both ELISA and slot blot assay reconciled with our understanding of the role of nucleotides in modulating the interaction of PfHsp70-1 with its functional partners. For example, ADP is known to promote the association of PfHsp70-1 with either PfHop or PfHsp70-z more effectively than ATP (Gitau et al., Citation2012; Zininga et al., Citation2016; Lebepe et al., Citation2020). The ELISA and slot data obtained in the current study demonstrated this phenomenon, further demonstrating that these two methods generate data that mirror assays such as SPR analysis, co-affinity and immunoprecipitation (Gitau et al., Citation2012; Zininga et al., Citation2015b, Citation2016; Lebepe et al., Citation2020).
To design new therapeutics, the drug discovery efforts rely heavily in part on identifying small molecule inhibitors targeting the interaction of key proteins with their network partners. As such, quantitative analysis of protein interactions is important in identifying proteins that are drug targets. In addition, these methods can be extended to study the effects of various events regulating protein function such as posttranslational modifications. Small molecule inhibitors such as Epigallocatechin-3-gallate (EGCG) (Zininga et al., Citation2017a) and polymyxin B (PMB) (Zininga et al., Citation2017b) have been shown to inhibit the interaction of PfHsp70-1 wit its co-chaperones. In the current study, we demonstrate that routine PPI studies as well as the screening of small molecule inhibitors targeting them could be conducted using ELISA and slot blot as cheaper alternative approaches. The main setback with the slot blot approach is that it is not amenable to high-throughput screening. We propose that both ELISA and slot blot assay are approaches that could be adopted for use in PPI and inhibitor screening studies in low-cost settings. Alternatively, the methods could be applied for preliminary PPI and inhibitor screening studies.
ACKNOWLEDGEMENTS
The authors would like to acknowledge the University of Cape Town, Faculty of Health Sciences for financial support to SM and Stellenbosch University Sub-Committee B for financial support to TZ.
DISCLOSURE
The authors declare no potential conflict of interest.
SUPPLEMENTAL DATA
Supplemental data for this article can be accessed here https://doi.org/10.1080/0035919X.2022.2144536
Additional information
Funding
References
- Berggård, T., Linse, S. & James, P. 2007. Methods for the detection and analysis of protein-protein interactions. Proteomics 7 (16): 2833–42. doi:10.1002/pmic.200700131.
- Botha, M., Pesce, E.R. & Blatch, G.L. 2007. The Hsp40 proteins of Plasmodium falciparum and other apicomplexa: regulating chaperone power in the parasite and the host. Int J Biochem Cell Biol 39 (10): 1781–1803. doi:10.1016/j.biocel.2007.02.011.
- Chakafana, G., Mudau, P.T., Zininga, T. & Shonhai, A. 2021. Characterisation of a unique linker segment of the Plasmodium falciparum cytosol localised Hsp110 chaperone. International Journal of Biological Macromolecules 180: 272–285. doi:10.1016/j.ijbiomac.2021.03.056.
- Gitau, G.W., Mandal, P., Blatch, G.L., Przyborski, J. & Shonhai, A. 2012. Characterisation of the Plasmodium falciparum Hsp70-Hsp90 organising protein (PfHop). Cell Stress and Chaperones 17 (2): 191–202. doi:10.1007/s12192-011-0299-x.
- Lebepe, C.M., Matambanadzo, P.R., Makhoba, X.H., Achilonu, I., Zininga, T. & Shonhai, A. 2020. Comparative characterization of Plasmodium falciparum Hsp70-1 relative to E. coli DnaK reveals the functional specificity of the parasite chaperone. Biomolecules 10 (6): 856.
- Mabate, B., Zininga, T., Ramatsui, L., Makumire, S., Achilonu, I., Dirr, H.W. & Shonhai, A. 2018. Structural and biochemical characterization of Plasmodium falciparum Hsp70-x reveals functional versatility of its C-terminal EEVN motif. Proteins 86 (11): 1189–1201. doi:10.1002/prot.25600.
- Makumire, S., Dongola, T.H., Chakafana, G., Tshikonwane, L., Chauke, C.T., Maharaj, T., Zininga, T. & Shonhai, A. 2021. Mutation of GGMP repeat segments of Plasmodium falciparum Hsp70-1 compromises chaperone function and Hop co-chaperone binding. International Journal of Molecular Sciences 22 (4): 2226. doi:10.3390/ijms22042226.
- Miura, K. 2018. An overview of current methods to confirm protein-protein interactions. Protein Pept Lett 25 (8): 728–733. doi:10.2174/0929866525666180821122240.
- Nguyen, H.H., Park, J., Kang, S. & Kim, M. 2015. Surface plasmon resonance: a versatile technique for biosensor applications. Sensors (Basel) 15 (5): 10481–10510. doi:10.3390/s150510481.
- Shonhai, A., Boshoff, A. & Blatch, G.L. 2007. The structural and functional diversity of Hsp70 proteins from Plasmodium falciparum. Protein Science 16 (9): 1803–1818. doi:10.1110/ps.072918107.
- Shonhai, A., Botha, M., de Beer, T., Boshoff, A. & Blatch, G.L. 2008. Structure-function study of a Plasmodium falciparum Hsp70 using three dimensional modelling and in vitro analyses. Protein & Peptide Letters 15 (10): 1117–1125.
- Zininga, T., Achilonu, I., Hoppe, H., Prinsloo, E., Dirr, H.W. & Shonhai, A. 2015a. Overexpression, purification and characterisation of the Plasmodium falciparum Hsp70-z (PfHsp70-z) protein. PLoS One 10 (6): e0129445.
- Zininga, T., Achilonu, I., Hoppe, H., Prinsloo, E., Dirr, H.W. & Shonhai, A. 2016. Plasmodium falciparum Hsp70-z, an Hsp110 homologue, exhibits independent chaperone activity and interacts with Hsp70-1 in a nucleotide-dependent fashion. Cell Stress and Chaperones 21 (3): 499–513. doi:10.1007/s12192-016-0678-4.
- Zininga, T., Makumire, S., Gitau, G.W., Njunge, J.M., Pooe, O.J., Klimek, H., Scheurr, R., Raifer, H., Prinsloo, E., Przyborski, J.M., Hoppe, H. & Shonhai, A. 2015b. Plasmodium falciparum Hop (PfHop) interacts with the Hsp70 chaperone in a nucleotide-dependent fashion and exhibits ligand selectivity. PLoS One 10 (8): e0135326. doi:10.1371/journal.pone.0135326.
- Zininga, T., Pooe, O.J., Makhado, P.B., Ramatsui, L., Prinsloo, E., Achilonu, I., Dirr, H. & Shonhai, A. 2017b. Polymyxin B inhibits the chaperone activity of Plasmodium falciparum Hsp70. Cell Stress and Chaperones 22 (5): 707–715. doi:10.1007/s12192-017-0797-6.
- Zininga, T., Ramatsui, L., Makhado, P.B., Makumire, S., Achilinou, I., Hoppe, H., Dirr, H. & Shonhai, A. 2017a. (-)-Epigallocatechin-3-Gallate inhibits the chaperone activity of Plasmodium falciparum Hsp70 chaperones and abrogates their association with functional partners. Molecules 22: 12. doi:10.3390/molecules22122139.