Abstract
Soil respiration (SR) and microbial respiration (MR), which were primarily regulated by soil temperature, can act as a feedback to climate change. Although many studies suggest that global warming will accelerate carbon dioxide (CO2) emissions from soil, the magnitude of this feedback is unknown, mostly due to uncertainty in the temperature sensitivity (Q10, increaing ratio of SR and MR after a 10°C increase of temperature) of SR and MR. To investigate the seasonal variation of short-term Q10 and estimate how grazing impacts temperature sensitivity of SR and MR, we measured SR and MR in a fenced and a grazed grassland on the loess plateau, northwestern China, during 2008–2010. In this semiarid grassland ecosystem, soil temperature was the dominant factor controlling SR and MR during the experimental period. Short-term apparent Q10 of SR and MR had a clearly seasonal variation, and was significantly and negatively related to soil temperature at 2-cm depth. However, no relationship was found between soil moisture (0–10 cm soil layer) and apparent Q10. By decreasing soil organic carbon and root biomass, grazing reduced the long-term Q10 of SR and MR. In both the short term and the long term, Q10 of MR was lower than that of SR, suggesting that autotrophic respiration is more sensitive than heterotrophic respiration to temperature. We emphasize the importance of using Q10 when modeling trajectories of soil carbon stocks under climate change scenarios, and the seasonal variation of Q10 should also be regarded as a parameter in the global carbon models.
1. INTRODUCTION
Because soil respiration (SR) is an essential process of the global carbon cycle, SR has attracted considerable research interest in recent years. Even subtle changes in climate (e.g., atmospheric temperature, nitrogen deposition) could trigger significant changes in SR, which would markedly alter ecosystem carbon budgets. Aside from its large quantity, SR is exponentially related to soil temperature which has dramatically increased during the last three decades, and will continue to increase in this century (IPCC Citation2007) in most ecosystems (e.g., Xu and Qi Citation2001b; Sampson et al. Citation2007). Other factors can also profoundly influence SR, such as soil quality (soil organic carbon), nitrogen deposition, climate conditions and human disturbance (Qi et al. Citation2002; Davidson and Janssens Citation2006; Davidson et al. Citation2006; Mo et al. Citation2007). Among all factors, Jones et al. (Citation2003) suggested that climate change, particularly rising temperature, was the major cause of increasing SR. Q10 of SR under temperature T defined as SRT+10/SRT, where SRT was soil respiration under soil temperature of T°C (Pang et al. Citation2013), was used to describe the sensitivity of SR to temperature, and was usually estimated based on empirical functions (e.g., exponential equation) between soil temperature and SR (Lloyd and Taylor Citation1994). An increase in this apparent Q10 in response to rising temperature is expected to have a positive feedback to global warming (Jenkinson et al. Citation1991; Raich and Schlesinger Citation1992). Nonetheless, the magnitude of this feedback is uncertain.
SR can be partitioned into two dominant fluxes (controlled by distinct processes): plant root respiration (autotrophic respiration; RR) and soil organic carbon (SOC) decomposition by soil fauna and soil microbes (heterotrophic respiration or microbial respiration; MR) (Hanson et al. Citation2000). Accordingly, the response of SR to temperature is determined by the effects of temperature on RR, MR and their interactions, which are also impacted by soil moisture, nutrient cycling, substrate availability and plant species traits (Cornwell et al. Citation2008). The apparent Q10 value of MR is one of the most important uncertainties in predicting future climate and terrestrial ecosystem function (Holland et al. Citation2000; Jones et al. Citation2003; Lenton and Huntingford Citation2003). If global warming from anthropogenic carbon dioxide (CO2) stimulates the decomposition of SOC, the extra CO2 efflux may accelerate the warming trend, forming a positive feedback (Jenkinson et al. Citation1991). The strength of this feedback largely depends on the realized temperature sensitivity of MR (Q10). Therefore, the SOC decomposition response to temperature has been extensively studied in recent years. Despite numerous studies, the temperature sensitivity of MR remains controversial (Davidson and Janssens Citation2006; Kirschbaum Citation2006; Hakkenberg et al. Citation2008). For example, Suseela et al. (Citation2012) found the temperature sensitivity of heterotrophic respiration was unresponsive to warming treatments; however, a reduction of the temperature sensitivity of soil organic matter decomposition with sustained temperature increasing was reported by Craine et al. (Citation2013). The causes of this controversy may stem from confounding controlling factors such as soil moisture and substrate availability (Davidson and Janssens Citation2006; Kirschbaum Citation2006).
Confounding factors such as plant phenological processes (Curiel Yuste et al. Citation2004) and soil water status (Gaumont-Guay et al. Citation2006) can cause a discrepancy between long-term (e.g., annual) and short-term (e.g., diel) temperature response parameters (Q10). The apparent annual Q10 value of SR and MR may not reflect the true biotic temperature sensitivity if obscured by seasonally varying factors other than soil temperature (Curiel Yuste et al. Citation2004). This is consistent with the ongoing debate on the use of a fixed (universal) vs. variable (environmentally controlled) Q10 in carbon cycle prediction (Mahecha et al. Citation2010). Single-site studies have reported large seasonal variation and temperature dependence of short-term unconfounded Q10 estimates for SR in forest ecosystems cycle modeling (Janssens and Pilegaard Citation2003; Curiel Yuste et al. Citation2004; Gaumont-Guay et al. Citation2006). Because a small deviation of the Q10 of SR may cause a significant bias in the estimate of SR (Xu and Qi Citation2001a), the use of a constant, seasonal Q10 in models may result in significant errors in predictions of future soil carbon losses. Therefore, estimating both long-term and short-term apparent Q10 of SR and MR may provide insights into the mechanisms driving soil and microbial respiration in a grassland, and aid in model parameterization.
Many studies have shown that warming accelerates MR, but most of these studies have examined soil in controlled laboratory conditions without considering many environmental constraints that could affect temperature sensitivity of organic matter decomposition. By using a root-exclusion method to partition SR, most of the ecosystem-scale experiments to date have focused on the contribution of RR and MR to total SR (Gaumont-Guay et al. Citation2008; Li et al. Citation2010; Ruehr and Buchmann Citation2010; Subke et al. Citation2011), rather than the effects of climate change on the components of SR and the Q10 values of each component. In this study, we compared the apparent Q10 of SR and MR by using a trenching method in a fenced grassland and a grazed grassland on the loess plateau, China. Specifically, our objectives were: (1) to investigate the variation of short-term Q10 of SR and MR in both fenced and grazed grassland; (2) to determine how soil temperature and moisture influence the short-term Q10 of SR and MR. In addition, as reported, grazing could influence the soil CO2 efflux (Wayne et al. Citation2008) and thereby SR sensitivity to temperature in grasslands (Paz-Ferreiro et al. Citation2012); one of our goals was to examine grazing effects on the long-term Q10 of SR and MR.
2. MATERIALS AND METHODS
2.1 Study sites
The experiment was conducted at the Semi-Arid Climate and Environment Observatory of Lanzhou University (SACOL), located at 35°57′N, 104°09′E (Gansu province, China), with a continental semiarid climate. A grazed grassland (G+) with a grazing rate of ~2 sheep per hectare and a fenced grassland (G–; about 500 m away, and fenced in 2005 to protect from grazing since then) were selected as the experimental site. The elevation of the site is 1966 m above sea level, the mean annual air temperature is 6.7°C and the mean annual precipitation is 382 mm. The soil is classified as a Sierozem, a calcareous soil which is characteristic of the loess plateau. The experimental site was a cultivated cropland before 1986, and had been restored from long-term farming since then. We divided the fenced and grazed grasslands into three subplots (50 m × 50 m) as replicates. The dominant plant species at the site were Stipa bungeana, Tripolium vulgare Ness. and Artemisia frigida.
2.2 Partitioning SR
We partitioned SR into MR and RR using the trench method described by Li et al. (Citation2010). In each subplot of both fenced and grazed grassland, 12 plots (0.5 m × 0.5 m in scale; plots were arranged in two lines, six plots in one line; and plots were separated by walkways of about 5 m) were selected as root-free plots, and close to (about 0.5 m from) each root-free plot, one control plot was selected (same size with root-free plots, and 12 control plots in total). In early May 2007, trenches (0.1 m wide and 0.5 m deep, based on the root status in the local grassland) were excavated around each root-free plot. After lining the trench with nylon mesh (0.038-mm mesh size), soil was refilled into the trench. This mesh prevents root growth into the plots but allows the movement of water, bacteria, organic matter and minerals (Moyano et al. Citation2007). The root-free plots were then kept free of vegetation by manual cutting (per fortnight) throughout the study, while extra care was taken to minimize disturbance to the soil. According to Li et al. (Citation2010), the CO2 efflux measured in the root-free plots represents MR, while CO2 efflux measured in the control plots represents SR. To minimize the error caused by the trench method, another 12 root-free plots in each subplot were rebuilt in May of each year during the experimental period (2008–2010), and each root-free plot was only used 1 year for the measurement of microbial respiration. All root-free plots were put to use for at least 8 months after the trenches had been built, in order to make sure the initial roots in the root-free plots were decomposed completely.
2.3 Measuring protocols
2.3.1 Soil respiration (SR) and microbial respiration (MR)
Soil CO2 efflux was measured on a clear day of each month in the growing season during 2008–2010 (for fenced grassland, 2008–2010; and for grazed grassland, 2009–2010). Before each sampling day, one polyvinyl chloride (PVC) collar (11 cm in diameter and 5 cm in height) was inserted into the soil to a 3-cm depth in each root-free plot and control plot in order to reduce CO2 efflux caused by disturbance. In the control plots, the plants in the collar were removed before the measurement in each sampling day. SR and MR were measured using a LI-6400 portable photosynthesis system attached to a soil CO2 flux chamber (991 cm3 in total volume; LI-COR 6400-09TC, LI-COR Inc., Lincoln, NE, USA). To avoid any pulse effect of precipitation on soil CO2 efflux, measurements were taken on the third or fourth day after a rain event. SR in each control plot and MR in each root-free plot were measured once a measuring day at 8:00 am and 11:00 am (local time). Each measurement usually took 1–2 minutes.
2.3.2 Soil temperature and moisture
Soil temperature and volumetric soil moisture were determined simultaneously with each measurement of soil CO2 flux. Soil temperature was measured using a thermocouple probe connected to the LI-6400 at a depth of 2 cm adjacent to each PVC collar, and volumetric soil moisture was determined at a depth of 0–10 cm using a TRIME TDR probe (IMKO, Ettlingen, Germany) adjacent to the sites where PVC collars were located.
2.3.3 Root biomass
Six quadrats were built in each subplot for measurements of root biomass in September of each year in both fenced and grazed grassland. Underground soil samples were recovered with an auger (9.3 cm inner diameter). In each quadrat, three soil cores to a depth of 50 cm were taken and divided into five 10-cm soil columns. Roots were collected by washing over a 0.5-mm sieve. No attempt was made to separate live and dead roots. To determine dry weight, all the samples were oven-dried at 85°C until a constant weight was reached.
2.3.4 Soil organic carbon (SOC)
In order to identify grazing effect on soil organic carbon, soil samples were collected in May of every year using an auger (5.5 cm inner diameter). In each control plot, one soil core to a depth of 10 cm was taken, then sieved through a 2-mm sieve. Soil organic carbon was determined with the oil bath–potassium dichromate (K2CrO7) titration method (oxidization with dichromate in presence of sulfuric acid (H2SO4), heated at 180°C for 5 minutes).
2.4 Data analyses and statistics
An exponential equation (Eq. 1) was selected to determine the relationships between CO2 flux and soil temperature (Suseela et al. Citation2012):
where CO2 flux stands for SR and MR, T is measured soil temperature at a depth of 2 cm, and α and β are regression coefficients.
The apparent Q10 values used in this study were calculated according to the following equation (Eq. 2) which was derived from Eq. 1 (Lloyd and Taylor Citation1994):
where β is the coefficient from Eq. 1. The short-term apparent Q10 values (exempt from seasonally confounding effects) were attained by the regression based on one day measurement (one measuring day timescale), while the long-term apparent Q10 was based on the entire experimental data (entire experimental timescale). For increasing the accuracy, all data in both fenced and grazed grassland (from three subplots) were parameterized into one regression equation. Then, in each sampling day, we only got one Q10 value for both fenced and grazed grassland, and the standard error (SE) of apparent Q10 values were computed by the method of Tu et al. (Citation2011).
We averaged SR and MR from the 12 collars in each subplot, and the means were used to represent each subplot for analysis. Data analyses were performed with SPSS 17.0 for Windows (USA). The independent T-test was employed to estimate the effect of grazing on SR and MR. One-way analysis of variance (ANOVA) was used to test the seasonal variations of SR and MR in both lands. An exponental equation was fitted for the relationships between short-term apparent Q10 values and soil temperature. There was no significant correlation between short-term apparent Q10 and soil moisture during the entire experiment. Statistical significance was defined at the 95% confidence level. The values in the text are means ± SE.
3. RESULTS
3.1 Soil microclimate, SR, MR and their relationships
The seasonal variation of soil temperature, soil moisture, and SR and MR from 2008 to 2010 are presented in . The soil temperature and moisture were not affected by trenching and grazing, and both variables exhibited clear seasonal patterns in all plots (P < 0.001, –). SR and MR also showed similar seasonal variations (P < 0.001), and SR in both fenced and grazed grassland was significantly higher than MR (). Additionally, SR and MR in fenced grassland were higher than that in grazed grassland (). SR and MR peaked in the growing season and bottomed out in winter during the whole experimental period. It appeared that SR and MR co-varied with soil temperature (). During the entire study period, SR and MR were strongly correlated with soil temperature (r from 0.91 to 0.97, ; ), but not with soil moisture ().
Table 1 Regression equation of soil and microbial respiration and soil temperature (2008–2010).
Figure 1 Seasonal variations in soil temperature (a: 2 cm), soil volumetric moisture (b: 0–10 cm) and soil and microbial respiration (c) measured in fenced (G–, 2008–2010) and grazed grassland (G+, 2009–2010). The bars in (b) represent precipitation. CO2: carbon dioxide.
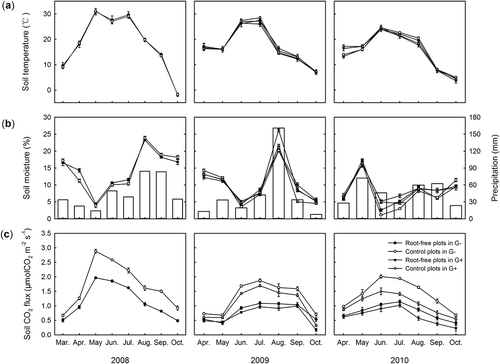
Figure 2 The relationship between soil respiration (SR) and microbial respiration (MR), and (a) soil temperature, and (b) soil moisture during the entire experiment. The open and black triangles indicate the data of SR and MR in the grazed grassland (G+); and open and black circles are data of SR and MR in the fenced grassland (G–). The solid line indicates the relationship between SR and soil temperature in G–; the medium dashed line shows the relationship between MR and soil temperature in G–; the long dashed line shows the relationship between SR and soil temperature in G+; and the short dashed line shows the relationship between MR and soil temperature in G+. CO2: carbon dioxide.
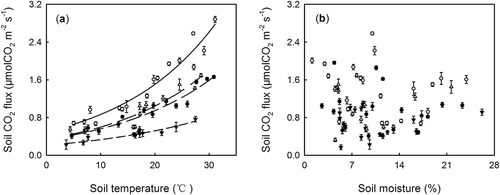
3.2 Seasonal variation of short-term apparent Q10 values of SR and MR
Regardless of treatments, the short-term apparent Q10 values of SR and MR showed clear seasonal variation (). The Q10 values of MR ranged from 1.19 to 10.36 and 1.42 to 8.06 in the fenced grassland and grazed grassland respectively, while Q10 values of SR ranged from 1.27 to 5.76 and 1.53 to 7.34 in the fenced grassland and grazed grassland respectively (). Q10 values of SR and MR in the growing season were lower than in the dormant season in the whole study period, and SR and MR exhibited no significant difference on short-term Q10 values in the growing season. Additionally, grazing did not affect the short-term apparent Q10 values of SR and MR significantly. In April and May of 2009 and October of 2010, Q10 values of MR were significantly higher than those of SR in fenced grassland (–).
3.3 Controlling factors of apparent Q10 values
An exponential regression was used to fit the relationship between short-term apparent Q10 of SR and MR and soil temperature, and we found a negative correlation (P < 0.05) between short-term apparent Q10 of SR and MR and soil temperature in both fenced and grazed grassland (). However, short-term apparent Q10 values of SR and MR in both grasslands were not correlated to soil moisture (P > 0.05, ).
Figure 4 Relationships between short-term Q10 values and soil temperature (at 2 cm depth). (a) Q10 of microbial respiration (MR) in the fenced grassland (G–); (b) Q10 of soil respiration (SR) in G–; (c) Q10 of MR in the grazed grassland (G+); (d) Q10 of SR in G+.
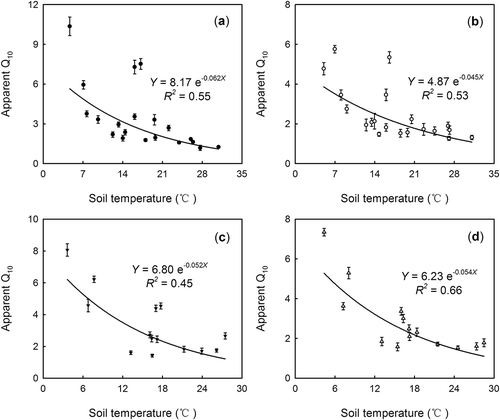
Figure 5 Relationships between short-term Q10 values and soil moisture (0–10 cm). Black circles: Q10 of microbial respiration (MR) in the fenced grassland (G–); open circles: Q10 of soil respiration (SR) in the fenced grassland (G–); black triangles: Q10 of microbial respiration in the grazed grassland (G+); open triangles: Q10 of soil respiration in the grazed grassland (G+).
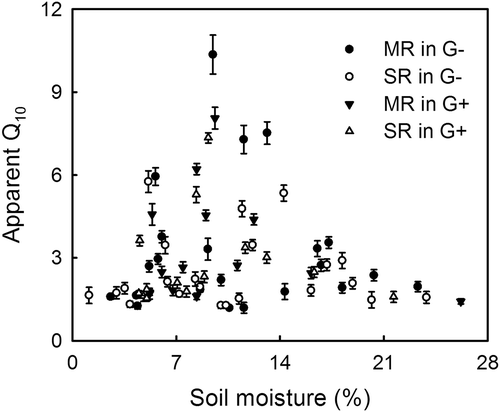
By analyzing the long-term apparent Q10 values, we found that fenced grassland had larger long-term apparent Q10 values of SR and MR when compared to grazed grassland (). In addition, long-term apparent Q10 values of MR in both grasslands were lower than those of SR (). Mean SR in fenced grassland was significantly higher than in grazed grassland, and so was mean MR (P < 0.001, ). In addition, mean MR values were lower than mean SR values. Means of root biomass (0–50 cm) and soil organic carbon (0–10 cm) in fenced grassland were significantly higher than in grazed grassland (P < 0.001, ).
Table 2 Long-term apparent Q10 (temperature sensitivity) values and means of soil and microbial respiration, root biomass and soil organic carbon in the fenced and grazed grassland during the entire experimental period.
4. DISCUSSION
4.1 The seasonal variation of short-term apparent Q10 of SR and MR
Our study indicated that short-term apparent Q10 of SR and MR had a clear seasonality, which is consistent with the recent results of Jia et al. (Citation2013) and Suseela et al. (Citation2012). Jia et al. (Citation2013) found that short-term Q10 was higher in the beginning of the growing season in a Chinese pine (Pinus tabulaeformis) plantation. Similarly, Suseela et al. (Citation2012) indicated that Q10 values in summer were lower than in fall and spring.
Short-term Q10 of SR and MR decreased with increasing soil temperature (), which was consistent with many previous studies (Kirschbaum Citation1995; Janssens and Pilegaard Citation2003; Curiel Yuste et al. Citation2004; Chen and Tian Citation2005; Gaumont-Guay et al. Citation2006; Peng et al. Citation2009; Wang et al. Citation2010). The reduction in Q10 with increasing soil temperature may be explained by the changes of limitation for SR and MR, from acclimation of enzymatic activity at low temperatures to limitation by substrate supply at high temperatures (Reichstein et al. Citation2005a; Gaumont-Guay et al. Citation2006). In addition, because Q10 is increasing in rate, it must be largely affected by the initial value. The same increment would be evaluated higher when the original value is smaller, and vice versa. For example, when the original respiration is very low (e.g., MR in October 2010, ), it would be easy to increase. However, if the initial respiration is high enough (e.g., SR in May 2008, ), it would hardly increase. This is one of the major reasons why Q10 takes a lower value under high soil temperature where respiration is already high enough. Soil moisture has been reported to regulate the seasonal temperature response of SR: e.g., Q10 decreases during drought (Xu and Qi Citation2001a; Craine and Gelderman Citation2011; Suseela et al. Citation2012). However, as reported by Luan et al. (Citation2013) and Jia et al. (Citation2013), the short-term apparent Q10 values of SR and MR had no significant relationship with soil moisture () in this study. Therefore, we suggest that SR and MR were mainly controlled by soil temperature, and less affected by soil moisture () in this semiarid ecosystem. The lack of regulation of SR by soil moisture has also been reported for temperate and boreal coniferous forests (Gaumont-Guay et al. Citation2008; Vargas et al. Citation2010; Jia et al. Citation2013) and an alpine meadow on the Tibetan Plateau (Suh et al. Citation2009). The relatively low soil moisture values () may help explain the absence of a soil moisture effect on SR and MR (Jia et al. Citation2013). This implies that there is a complex relationship between Q10 and soil moisture, which may result in contradictory results. Further research is needed to examine the effect of soil moisture on temperature sensitivity of SR and MR.
The short-term temperature responses of SR and MR deviated significantly from the long-term apparent Q10 values in both fenced and grazed grassland ( and ), because seasonally varying biophysical drives (e.g., root dynamics, plant photosynthesis and phenology) confound the relationship between SR and temperature (Curiel Yuste et al. Citation2004; Gaumont-Guay et al. Citation2006; Sampson et al. Citation2007). The variability of long-term apparent Q10 of SR was larger than that of MR, which may be due to the fact that Q10 values of SR are more closely related to seasonal changes in autotrophic respiration (Högberg Citation2010). And some studies have demonstrated that autotrophic respiration is more sensitive to temperature than heterotrophic respiration (Boone et al. Citation1998; Singh et al. Citation2003; Gaumont-Guay et al. Citation2008). The reason for this phenomenon may be that autotrophic respiration is not only controlled by soil temperature but also affected by plant photosynthetic activity or root growth dynamics, or both (which have larger variation on diurnal and seasonal scales). In the present study, the average short-term Q10 of MR and SR in both grasslands were higher than those obtained from the seasonal relationship ( and ). Apparent Q10 values calculated from the short-term (or high-frequency) temperature response are exempt from seasonally confounding effects, and thus are better able to reflect the biological sensitivity of respiration to temperature (Janssens and Pilegaard Citation2003; Curiel Yuste et al. Citation2004; Mahecha et al. Citation2010). However, terrestrial carbon models such as Roth-C, CENTURY and TEM generally assume a constant temperature sensitivity of SR regardless of the characteristics of the ecosystems (Jenkinson et al. Citation1991; Tian et al. Citation1999), although a study by Raich and Schlesinger (Citation1992) suggested that Q10 is likely to vary across different ecosystems and climatic conditions. Our study also demonstrated that the temperature sensitivity of soil organic matter decomposition is governed not only by substrate traits but also by the environmental constraints that are undergoing constant changes (); this is consistent with many other reports (Davidson and Janssens Citation2006; Conant et al. Citation2011; Suseela et al. Citation2012). We suggest that the short-term and long-term Q10 should both be used in predicting the future carbon cycle.
4.2 Effect of grazing on long-term Q10
Grazing decreased SR and MR and their long-term apparent Q10 values, which might be caused by the decrease in plant productivity and SOC (). Grazing animals affect soil organic matter quantity and quality in several ways, such as through trampling and defecation. Grazing especially plays a key role in governing the amount of plant residue and root exudates in soil by causing the plant productivity to differ and changing plant species composition, thereby possibly having profound effects on the activity and composition of soil microbial communities (Bardgett and Wardle Citation2003). These changes, in turn, affect rates of nutrient cycling, creating feedbacks to plant productivity that affect the amount of organic matter entering soil (Bardgett and Wardle Citation2003).
Luan et al. (Citation2013) found a positive relationship between apparent Q10 and fine root biomass, and an association between the fine root biomass and the fast-turnover carbon pool. The importance of plant activity in controlling the apparent temperature response of SR has been well documented (Reichstein et al. Citation2003; Curiel Yuste et al. Citation2004; Hogberg and Read Citation2006; Sampson et al. Citation2007). Several studies have also found a strong positive correlation between the basal rate of SR (SR at 10°C) and plant productivity across different forest sites (Sampson et al. Citation2007; Irvine et al. Citation2008). In general, the seasonal Q10 of SR can be stimulated by plant activity through several (at least two) mechanisms. First, plant activity has a direct effect on SR via root and rhizosphere respiration (Wang et al. Citation2010). Second, plant activity indirectly affects microbial decomposition of SOC via the so-called rhizosphere priming effect, in which interactions between roots and soil may accelerate microbial decomposition of native SOC (Dijkstra and Cheng Citation2007). The increased plant productivity and SOC in the fenced grassland is probably most responsible for the higher SR and MR and their apparent Q10 values ().
Many studies have examined the responses of the temperature sensitivities of labile and recalcitrant pools of SOC to warming (Giardina and Ryan Citation2000; Fang et al. Citation2006; Conant et al. Citation2008) as well as the mechanisms underlying those responses, such as the degree of acclimation of microbes (Bradford et al. Citation2008; Allison et al. Citation2010) or depletion of substrates (Kirschbaum Citation2004; Eliasson et al. Citation2005; Hartley and Ineson Citation2008). It has been suggested that different SOC pools should have different intrinsic temperature sensitivities (Knorr et al. Citation2005; Davidson and Janssens Citation2006; Feng and Simpson Citation2008; Hartley et al. Citation2008) so that changes in the relative abundance of different soil carbon substrates across sites may lead to differences in the temperature sensitivity of overall SOC decomposition (Knorr et al. Citation2005; Fierer et al. Citation2006). This may help explain the higher Q10 of MR in fenced grassland than in grazed grassland (fenced grassland had a higher SOC, ). According to the kinetic theory of Arrhenius, the temperature sensitivity increases with increasing activation energy. If the differences in decomposition rate are entirely due to the activation energy (the energy required for decomposers to access the material), the temperature sensitivity should increase with the recalcitrance (which is consistent with SOC) of the organic material (Davidson and Janssens Citation2006; Hartley and Ineson Citation2008). Additionally, the carbon quality temperature (CQT) hypothesis (Bosatta and Ågren Citation1999; Fierer et al. Citation2005; Davidson and Janssens Citation2006) predicts that the temperature sensitivity of organic matter decomposition increases with biochemical recalcitrance, which has been detected on a variety of substrates and spatial scales (Fierer et al. Citation2005, Citation2006; Conant et al. Citation2008; Craine et al. Citation2010). However, the evidence for the temperature sensitivity of organic matter decomposition correlated with biochemical recalcitrance is organic matter specific. For example, some studies were able to detect different Q10s for different substrates (Knorr et al. Citation2005; Leifeld and Fuhrer Citation2005; Fierer et al. Citation2006), whereas others were not able to do so or obtained inconsistent results (Fang et al. Citation2005; Reichstein et al. Citation2005b; Conen et al. Citation2006). Conant et al. (Citation2011) recently suggested that the physico-chemical protection from decomposition of organic matter (OM) would affect the temperature response of SOM. Further research is needed to quantify the effects of the different relative abundance of various soil carbon substrates on the different SR sensitivities to temperature observed at different sites.
5. CONCLUSIONS
While multiple factors can influence short-term apparent Q10 of both SR and MR, our findings show that, for this semiarid ecosystem, soil temperature is the primary reason why apparent Q10 has a clearly seasonal variation. The seasonal patterns are similar in both fenced and grazed grassland, with lower Q10s in growing season and larger Q10s in the beginning and end of growing season. No relationship was found between soil moisture and short-term apparent Q10 of SR and MR. Given the plant phenological dynamic, long-term apparent Q10 is different from short-term Q10. Thus, we conclude that climate change (e.g., rising temperature and nitrogen deposition) in this semiarid grassland ecosystem, as predicted by the Intergovernmental Panel on Climate Change (IPCC Citation2007), will result in a complex impact on temperature sensitivity of SR and especially MR. The information on long- and short-term apparent Q10 changes should be carefully incorporated into carbon cycle modelling processes, especially under global change scenarios.
Additionally, grazing has a negative effect on the long-term apparent Q10 of SR and MR. This result can be explained by the decrease of root biomass and soil organic carbon caused by livestock grazing. Furthermore, in general, the Q10 of MR is found to be lower than that of SR, which implies that autotrophic respiration is more sensitive to temperature than heterotrophic respiration is. We speculate that temperature sensitivity of respiration is influenced not only by abiotic factors but also by substrate availability.
ACKNOWLEDGMENTS
This study was supported by the National Basic Research Program of China (2014CB138703), the “Strategic Priority Research Program – Climate Change: Carbon Budget and Related Issues” of the Chinese Academy of Sciences (XDA05050406-8), the Natural Science Foundation of China (31070412 and 31201837), the Fundamental Research Funds for Central Universities (lzujbky-2014-78), and the Program for Changjiang Scholars and Innovative Research Team in University (IRT13019). The authors are grateful to the Semi-Arid Climate and Environment Observatory of Lanzhou University (SACOL) for supporting the field work.
REFERENCES
- Allison SD, Wallenstein MD, Bradford MA 2010: Soil-carbon response to warming dependent on microbial physiology. Nat. Geosci., 3, 336–340. doi:10.1038/ngeo846
- Bardgett RD, Wardle DA 2003: Herbivore-mediated linkages between aboveground and belowground communities. Ecology, 84, 2258–2268. doi:10.1890/02-0274
- Boone RD, Nadelhoffer KJ, Canary JD, Kaye JP 1998: Roots exert a strong influence on the temperature sensitivityof soil respiration. Nature, 396, 570–572. doi:10.1038/25119
- Bosatta E, Ågren GI 1999: Soil organic matter quality interpreted thermodynamically. Soil Biol. Biochem., 31, 1889–1891. doi:10.1016/S0038-0717(99)00105-4
- Bradford MA, Davies CA, Frey SD, Maddox TR, Melillo JM, Mohan JE, Reynolds JF, Treseder KK, Wallenstein MD 2008: Thermal adaptation of soil microbial respiration to elevated temperature. Ecol. Lett., 11, 1316–1327. doi:10.1111/ele.2008.11.issue-12
- Chen H, Tian H-Q 2005: Does a general temperature-dependent Q10 model of soil respiration exist at biome and global scale? J. Integr. Plant Biol., 47, 1288–1302. doi:10.1111/jipb.2005.47.issue-11
- Conant RT, Drijber RA, Haddix ML, Parton WJ, Paul EA, Plante AF, Six J, Steinweg JM 2008: Sensitivity of organic matter decomposition to warming varies with its quality. Glob Change Biol., 14, 868–877. doi:10.1111/gcb.2008.14.issue-4
- Conant RT, Ryan MG, Ågren GI et al. 2011: Temperature and soil organic matter decomposition rates - synthesis of current knowledge and a way forward. Glob Change Biol., 17, 3392–3404. doi:10.1111/j.1365-2486.2011.02496.x
- Conen F, Leifeld J, Seth B, Alewell C 2006: Warming mineralises young and old soil carbon equally. Biogeosciences, 3, 515–519. doi:10.5194/bg-3-515-2006
- Cornwell WK, Cornelissen JH, Amatangelo K et al. 2008: Plant species traits are the predominant control on litter decomposition rates within biomes worldwide. Ecol. Lett., 11, 1065–1071. doi:10.1111/j.1461-0248.2008.01219.x
- Craine J, Spurr R, McLauchlan K, Fierer N 2010: Landscape-level variation in temperature sensitivity of soil organic carbon decomposition. Soil Biol. Biochem., 42, 373–375. doi:10.1016/j.soilbio.2009.10.024
- Craine JM, Fierer N, McLauchlan KK, Elmore AJ 2013: Reduction of the temperature sensitivity of soil organic matter decomposition with sustained temperature increase. Biogeochemistry, 113, 359–368. doi:10.1007/s10533-012-9762-8
- Craine JM, Gelderman TM 2011: Soil moisture controls on temperature sensitivity of soil organic carbon decomposition for a mesic grassland. Soil Biol. Biochem., 43, 455–457. doi:10.1016/j.soilbio.2010.10.011
- Curiel Yuste J, Janssens IA, Carrara A, Ceulemans R 2004: Annual Q10 of soil respiration reflects plant phenological patterns as well as temperature sensitivity. Glob. Change Biol., 10, 161–169. doi:10.1111/gcb.2004.10.issue-2
- Davidson EA, Janssens IA 2006: Temperature sensitivity of soil carbon decomposition and feedbacks to climate change. Nature, 440, 165–173. doi:10.1038/nature04514
- Davidson EA, Janssens IA, Luo Y 2006: On the variability of respiration in terrestrial ecosystems: moving beyond Q10. Glob. Change Biol., 12, 154–164. doi:10.1111/gcb.2006.12.issue-2
- Dijkstra FA, Cheng W 2007: Interactions between soil and tree roots accelerate long-term soil carbon decomposition. Ecol. Lett., 10, 1046–1053. doi:10.1111/j.1461-0248.2007.01095.x
- Eliasson PE, McMurtrie RE, Pepper DA, Stromgren M, Linder S, Agren GI 2005: The response of heterotrophic CO2 flux to soil warming. Glob. Change Biol., 11, 167–181. doi:10.1111/gcb.2005.11.issue-1
- Fang C, Smith P, Smith J 2006: Is resistant soil organic matter more sensitive to temperature than the labile organic matter? Biogeosciences, 3, 65–68. doi:10.5194/bg-3-65-2006
- Fang CM, Smith P, Moncrieff JB, Smith JU 2005: Similar response of labile and resistant soil organic matter pools to changes in temperature. Nature, 433, 57–59. doi:10.1038/nature03138
- Feng XJ, Simpson MJ 2008: Temperature responses of individual soil organic matter components. J. Geophys. Res., 113(G3).
- Fierer N, Colman BP, Schimel JP, Jackson RB 2006: Predicting the temperature dependence of microbial respiration in soil: a continental-scale analysis. Glob. Biogeochem. Cy., 20, GB3026. doi:10.1029/2005GB002644
- Fierer N, Craine JM, McLauchlan K, Schimel JP 2005: Litter quality and the temperature sensitivity of decomposition. Ecology, 86, 320–326. doi:10.1890/04-1254
- Gaumont-Guay D, Black TA, Barr AG, Jassal RS, Nesic Z 2008: Biophysical controls on rhizospheric and heterotrophic components of soil respiration in a boreal black spruce stand. Tree Physiol., 28, 161–171. doi:10.1093/treephys/28.2.161
- Gaumont-Guay D, Black TA, Griffis TJ, Barr AG, Jassal RS, Nesic Z 2006: Interpreting the dependence of soil respiration on soil temperature and water content in a boreal aspen stand. Agr. Forest Meteorol., 140, 220–235. doi:10.1016/j.agrformet.2006.08.003
- Giardina CP, Ryan MG 2000: Evidence that decomposition rates of organic carbon in mineral soil do not vary with temperature. Nature, 404, 858–861. doi:10.1038/35009076
- Hakkenberg R, Churkina G, Rodeghiero M, Börner A, Steinhof A, Cescatti A 2008: Temperature sensitivity of the turnover times of soil organic matter in forests. Ecol. Appl., 18, 119–131. doi:10.1890/06-1034.1
- Hanson P, Edwards N, Garten C, Andrews J 2000: Separating root and soil microbial contributions to soil respiration: a review of methods and observations. Biogeochemistry, 48, 115–146. doi:10.1023/A:1006244819642
- Hartley IP, Hopkins DW, Garnett MH, Sommerkorn M, Wookey PA 2008: Soil microbial respiration in arctic soil does not acclimate to temperature. Ecol. Lett., 11, 1092–1100. doi:10.1111/j.1461-0248.2008.01223.x
- Hartley IP, Ineson P 2008: Substrate quality and the temperature sensitivity of soil organic matter decomposition. Soil Biol. Biochem., 40, 1567–1574. doi:10.1016/j.soilbio.2008.01.007
- Högberg P 2010: Is tree root respiration more sensitive than heterotrophic respiration to changes in soil temperature? New Phytol., 188, 9–10. doi:10.1111/j.1469-8137.2010.03366.x
- Hogberg P, Read DJ 2006: Towards a more plant physiological perspective on soil ecology. Trends Ecol. Evol., 21, 548–554. doi:10.1016/j.tree.2006.06.004
- Holland EA, Neff JC, Townsend AR, McKeown B 2000: Uncertainties in the temperature sensitivity of decomposition in tropical and subtropical ecosystems: implications for models. Global Biogeochem. Cy., 14, 1137–1151. doi:10.1029/2000GB001264
- IPCC 2007: Climate change 2007-the physical science basis: working group I contribution to the fourth assessment report of the IPCC, Cambridge University Press.
- Irvine J, Law BE, Martin JG, Vickers D 2008: Interannual variation in soil CO2 efflux and the response of root respiration to climate and canopy gas exchange in mature ponderosa pine. Glob. Change Biol., 14, 2848–2859. doi:10.1111/j.1365-2486.2008.01682.x
- Janssens IA, Pilegaard K 2003: Large seasonal changes in Q10 of soil respiration in a beech forest. Glob. Change Biol., 9, 911–918. doi:10.1046/j.1365-2486.2003.00636.x
- Jenkinson D, Adams D, Wild A 1991: Model estimates of CO2 emissions from soil in response to global warming. Nature, 351, 304–306. doi:10.1038/351304a0
- Jia X, Zha TS, Wu B et al. 2013: Temperature response of soil respiration in a Chinese pine plantation: hysteresis and seasonal vs. diel Q10. PLoS One, 8, e57858.
- Jones CD, Cox P, Huntingford C 2003: Uncertainty in climate-carbon-cycle projections associated with the sensitivity of soil respiration to temperature. Tellus B, 55, 642–648. doi:10.1034/j.1600-0889.2003.01440.x
- Kirschbaum MUF 1995: The temperature dependence of soil organic matter decomposition, and the effect of global warming on soil organic C storage. Soil Biol. Biochem., 27, 753–760. doi:10.1016/0038-0717(94)00242-S
- Kirschbaum MUF 2004: Soil respiration under prolonged soil warming: are rate reductions caused by acclimation or substrate loss? Glob. Change Biol., 10, 1870–1877. doi:10.1111/j.1365-2486.2004.00852.x
- Kirschbaum MUF 2006: The temperature dependence of organic-matter decomposition-still a topic of debate. Soil Biol. Biochem., 38, 2510–2518. doi:10.1016/j.soilbio.2006.01.030
- Knorr W, Prentice I House J, Holland E 2005: Long-term sensitivity of soil carbon turnover to warming. Nature, 433, 298–301. doi:10.1038/nature03226
- Leifeld J, Fuhrer J 2005: The temperature response of CO2 production from bulk soils and soil fractions is related to soil organic matter quality. Biogeochemistry, 75, 433–453. doi:10.1007/s10533-005-2237-4
- Lenton TM, Huntingford C 2003: Global terrestrial carbon storage and uncertainties in its temperature sensitivity examined with a simple model. Glob. Change Biol., 9, 1333–1352. doi:10.1046/j.1365-2486.2003.00674.x
- Li XD, Fu H, Guo D, Li XD, Wan CG 2010: Partitioning soil respiration and assessing the carbon balance in a Setaria italica (L.) Beauv. Cropland on the Loess Plateau, Northern China. Soil Biol. Biochem., 42, 337–346. doi:10.1016/j.soilbio.2009.11.013
- Lloyd J, Taylor J 1994: On the temperature dependence of soil respiration. Funct. Ecol, 315–323.
- Luan JW, Liu SR, Wang JX, Zhu XL 2013: Factors affecting spatial variation of annual apparent Q10 of soil respiration in two warm temperate forests. PLoS One, 8, e64167.
- Mahecha MD, Reichstein M, Carvalhais Net al. 2010: Global convergence in the temperature sensitivity of respiration at ecosystem level. Science, 329, 838–840. doi:10.1126/science.1189587
- Mo JM, Zhang W, Zhu WX, Gundersen P, Fang Y, Li D, Wang H 2007: Nitrogen addition reduces soil respiration in a mature tropical forest in southern China. Glob. Change Biol., 14, 403–412. doi:10.1111/j.1365-2486.2007.01503.x
- Moyano FE, Kutsch WL, Schulze E 2007: Response of mycorrhizal, rhizosphere and soil basal respiration to temperature and photosynthesis in a barley field. Soil Biol. Biochem., 39, 843–853. doi:10.1016/j.soilbio.2006.10.001
- Pang X, Bao W, Zhu B, Cheng W 2013: Responses of soil respiration and its temperature sensitivity to thinning in a pine plantation. Agr. Forest Meteorol., 171-172, 57–64. doi:10.1016/j.agrformet.2012.12.001
- Paz-Ferreiro J, Medina-Roldán E, Ostle NJ, McNamara NP, Bardgett RD 2012: Grazing increases the temperature sensitivity of soil organic matter decomposition in a temperate grassland. Environ. Res. Lett., 7, 014027.
- Peng SS, Piao SL, Wang T, Sun JY, Shen ZH 2009: Temperature sensitivity of soil respiration in different ecosystems in China. Soil Biol. Biochem., 41, 1008–1014. doi:10.1016/j.soilbio.2008.10.023
- Qi Y, Xu M, Wu JG 2002: Temperature sensitivity of soil respiration and its effects on ecosystem carbon budget: nonlinearity begets surprises. Ecol. Model., 153, 131–142. doi:10.1016/S0304-3800(01)00506-3
- Raich JW, Schlesinger WH 1992: The global carbon dioxide flux in soil respiration and its relationship to vegetation and climate. Tellus B, 44, 81–99. doi:10.1034/j.1600-0889.1992.t01-1-00001.x
- Reichstein M, Falge E, Baldocchi D et al. 2005a: On the separation of net ecosystem exchange into assimilation and ecosystem respiration: review and improved algorithm. Glob. Change Biol., 11, 1424–1439. doi:10.1111/j.1365-2486.2005.001002.x
- Reichstein M, Rey A, Freibauer A et al. 2003: Modeling temporal and large-scale spatial variability of soil respiration from soil water availability, temperature and vegetation productivity indices. Glob. Biogeochem. Cy., 17, 1104. doi:10.1029/2003GB002035
- Reichstein M, Subke J-A, Angeli AC, Tenhunen JD 2005b: Does the temperature sensitivity of decomposition of soil organic matter depend upon water content, soil horizon, or incubation time? Glob. Change Biol., 11, 1754–1767. doi:10.1111/gcb.2005.11.issue-10
- Ruehr NK, Buchmann N 2010: Soil respiration fluxes in a temperate mixed forest: seasonality and temperature sensitivities differ among microbial and root-rhizosphere respiration. Tree Physiol., 30, 165–176. doi:10.1093/treephys/tpp106
- Sampson DA, Janssens IA, Curiel Yuste J, Ceulemans R 2007: Basal rates of soil respiration are correlated with photosynthesis in a mixed temperate forest. Glob. Change Biol., 13, 2008–2017. doi:10.1111/gcb.2007.13.issue-9
- Singh B, Nordgren A, Ottosson Löfvenius M, Högberg M, Mellander PE, Högberg P 2003: Tree root and soil heterotrophic respiration as revealed by girdling of boreal Scots pine forest: extending observations beyond the first year. Plant Cell Environ., 26, 1287–1296. doi:10.1046/j.1365-3040.2003.01053.x
- Subke J-A, Voke NR, Leronni V, Garnett MH, Ineson P 2011: Dynamics and pathways of autotrophic and heterotrophic soil CO2 efflux revealed by forest girdling. J. Ecol., 99, 186–193. doi:10.1111/jec.2010.99.issue-1
- Suh S, Lee E, Lee J 2009: Temperature and moisture sensitivities of CO2 efflux from lowland and alpine meadow soils. J. Plant Ecol., 2, 225–231. doi:10.1093/jpe/rtp021
- Suseela V, Conant RT, Wallenstein MD, Dukes JS 2012: Effects of soil moisture on the temperature sensitivity of heterotrophic respiration vary seasonally in an old-field climate change experiment. Glob. Change Biol., 18, 336–348. doi:10.1111/j.1365-2486.2011.02516.x
- Tian H, Melillo J, Kicklighter D, McGuire A, Helfrich J 1999: The sensitivity of terrestrial carbon storage to historical climate variability and atmospheric CO2 in the United States. Tellus B, 51, 414–452. doi:10.1111/teb.1999.51.issue-2
- Tu L-H, Hu T-X, Zhang J, Li R-H, Dai H-Z, Luo S-H 2011: Short-term simulated nitrogen deposition increases carbon sequestration in a Pleioblastus amarus plantation. Plant Soil, 340, 383–396. doi:10.1007/s11104-010-0610-0
- Vargas R, Baldocchi DD, Allen MF et al. 2010: Looking deeper into the soil: biophysical controls and seasonal lags of soil CO2 production and efflux. Ecol. Appl., 20, 1569–1582. doi:10.1890/09-0693.1
- Wang XH, Piao SL, Ciais P, Janssens IA, Reichstein M, Peng S, Wang T 2010: Are ecological gradients in seasonal Q10 of soil respiration explained by climate or by vegetation seasonality? Soil Biol. Biochem., 42, 1728–1734. doi:10.1016/j.soilbio.2010.06.008
- Wayne PH, Frank AB, Sanabria J, Phillips RL 2008: Interannual variability in carbon dioxide fluxes and flux-climate relationships on grazed and ungrazed northern mixed-grass prairie. Glob. Change Biol., 14, 1620–1632. doi:10.1111/j.1365-2486.2008.01599.x
- Xu M, Qi Y 2001a: Soil-surface CO2 efflux and its spatial and temporal variations in a young ponderosa pine plantation in northern California. Glob. Change Biol., 7, 667–677. doi:10.1046/j.1354-1013.2001.00435.x
- Xu M, Qi Y 2001b: Spatial and seasonal variations of Q10 determined by soil respiration measurements at a Sierra Nevadan forest. Glob. Biogeochem. Cy., 15, 687–696. doi:10.1029/2000GB001365