ABSTRACT
One mechanism of arsenic detoxification in plants is synthesis of phytochelatins from glutathione in a sulfur-dependent manner. This study examined the contribution of a sulfate transporter, SULTR1;2, in arsenic tolerance in terms of sulfur metabolism in Arabidopsis thaliana (L.) Heynh. Comparative analysis of SULTR mutants showed that defective mutations of SULTR1;2 resulted in an increased arsenic sensitivity, in both shoots and roots, establishing that SULTR1;2 is required for arsenic tolerance. We subsequently quantified total sulfur content and levels of sulfur compounds (phytochelatins, glutathione, cysteine) in a SULTR1;2 mutant, sel1-8. Arsenic treatments increased sulfur uptake of sel1-8, but the mutant was unable to maintain the proper basal level of sulfur. Despite drastic reduction of total sulfur content, the mutant accumulated substantial or rather excess phytochelatins in shoots under arsenic stress conditions. The levels of glutathione and cysteine in shoots were lower in sel1-8 than in the wild type, possibly representing partial disorder of sulfur nutrition under limited sulfur supply. Taken together, we propose that SULTR1;2 contributes to arsenic tolerance by maintaining proper sulfur nutrient status at high demand for sulfur by phytochelatins-synthetic pathway, and/or optimal level of phytochelatin synthesis in shoots.
KEY WORDS:
1. Introduction
Arsenic (As) is a well-known toxic metalloid and causes various diseases including cancers in humans. Globally, millions of people have been at risk for As exposure, and its significant pollution is of great global concern (Nordstrom Citation2002). Consumption of high-As-containing foods/crops produced in As-contaminated fields is one of the major sources of human As intake, following drinking of As-contaminated water (Meharg Citation2004). As is also toxic to plants; the strong toxicity of As reduces crop yields (Panaullash et al. Citation2009; Khan et al. Citation2010). To address these issues, a number of studies have been conducted to understand the mechanisms underlying As accumulation and toxicity in plants.
The major oxidation state of As is AsV in the form of arsenate (AsO43−) in upland fields. Arsenate is an analog of phosphate; thus, phosphate transporters mediate arsenate uptake in Arabidopsis thaliana (L.) Heynh (Shin et al. Citation2004) and probably in rice (Oryza sativa L.) (Wu et al. Citation2011; Kamiya et al. Citation2013). In rice, overexpression of phosphate transporters OsPT8 and OsPT1 enhanced As uptake and translocation into shoots (Wu et al. Citation2011; Kamiya et al. Citation2013). Absorbed arsenate is rapidly reduced to arsenite (AsO33−) by reductase activities; therefore, As is predominantly present as arsenite in plants (Chao et al. Citation2014; Sánchez-Bermejo et al. Citation2014). In flooded paddy conditions, reduced As (arsenite) is a dominant chemical form in soil (Abedin et al. Citation2002) and is absorbed by plant aquaporin-like proteins (Ma et al. Citation2008; Kamiya et al. Citation2009).
Arsenite has a strong affinity to thiol groups and inactivates various protein functions, resulting in severe physiological disorders and impairment in plants (Meharg and Hartley-Whitaker Citation2002). However, the strong affinity of arsenite also allows thiol-containing chelators to form complexes with arsenite. Phytochelatins (PCs) are well-studied metabolites capable of chelating toxic metals/metalloids including arsenite in plants (Grill et al. Citation1985). PCs have a general structure of (γ-Glu-Cys)nGly (n = 2–11). Phytochelatin synthase (PCS) synthesizes PCs generally using γ-Glu-Cys-Gly (GSH) as a substrate under the presence of metal/metalloid in cytosol (Vatamaniuk et al. Citation2000, Citation2004). PCS homologs are ubiquitously distributed throughout the plant kingdom and are also found in some other eukaryotic species (Clemens Citation2006; Kühnlenz et al. Citation2014). Non-functional PCS genes causes hypersensitivity to metal/metalloid in A. thaliana (Howden et al. Citation1995; Tennstedt et al. Citation2009; Fischer et al. Citation2014), Caenorhabditis elegans (Maupas, 1900) (Vatamaniuk et al. Citation2001) and Schizosaccharomyces pombe (Clemens et al. Citation1999), suggesting that the PC-mediated metal/metalloid detoxification system is widely conserved in eukaryote. The PC-metal/metalloid complex formed in cytosol is sequestrated into vacuoles, mediated by ATP-binding cassette subfamily C transporters (Song et al. Citation2010; Park et al. Citation2012). This step is also crucial for achieving metal/metalloid tolerance of A. thaliana.
PC biosynthesis is dependent on cellular sulfur (S) assimilation. Sulfate transported into cells is reduced and incorporated into Cys and subsequent GSH synthesis (Mendoza-Cózatl et al. Citation2005). It is known that sulfate uptake, distribution and transport within cells are mediated by the SULTR family of sulfate transporters (Takahashi et al. Citation2011). In A. thaliana, SULTR1;2 is the primary S uptake transporter in roots (Shibagaki et al. Citation2002; Yoshimoto et al. Citation2002, Citation2007; Barberon et al. Citation2008), and its expression is strongly induced by As stress along with the expression of other S-assimilation genes (Sung et al. Citation2009; Jobe et al. Citation2012). The up-regulation of SULTR homologs in response to As stress has also been observed in other species such as barley (Hordeum vulgare L.) and rice (Reid et al. Citation2013; Srivastava et al. Citation2014). In barley, total S concentration in tissues is increased by As exposure and its accumulation, which is accompanied by the induction of the SULTR homolog (HvST1) expression and the increase of thiol levels (Reid et al. Citation2013). These suggest that As stresses enhance S uptake by up-regulating SULTR transporters, and we hypothesize that proper S uptake and supply for S-metabolic pathways, including the PC-synthetic pathway, are important for achieving As tolerance of plants.
This study investigated the contribution of SULTR transporters to As tolerance of A. thaliana. First, responses of A. thaliana SULTR mutants were examined under As-stressed conditions, and we found that SULTR1;2 defective mutants showed enhanced As sensitivities. Next, in order to investigate the influence of SULTR1;2 disruption on the PC-synthetic pathway, accumulation patterns of total S, PCs and PC-precursor metabolites were analyzed in a SULTR1;2-knockout mutant, sel1-8. The analysis revealed that the shoot PC levels were abnormally higher in the mutant than in the wild type, whereas total S in the mutant was remarkably lower than the wild type. Taken together, we suggest that SULTR1;2 is essential for As tolerance of A. thaliana, probably by maintaining proper S nutrient status. Possible interactions between plant S nutrition status and PC synthesis under As exposure are also discussed.
2. Materials and methods
2.1. Plant materials and growth conditions
The sultr1;2 defective mutants of A. thaliana (sel1-8 and sel1-10) and their wild types (Col-0 and Ws, respectively) were used in this study (Shibagaki et al. Citation2002; Maruyama-Nakashita et al. Citation2003). The information of the other SULTR mutants is shown in the Supplementary material. Prior to stress tolerance or response assay, plants were established for 1 week on MGRL-based agar plates (Fujiwara et al. Citation1992) containing 1% sucrose, 1.2% type-A agar (Sigma-Aldrich Co.) and 5 mM 2-Morpholinoethanesulfonic acid (pH 5.7, potassium hydroxide) in a growth chamber at 22°C with 16-h light periods.
2.2. Stress tolerance assay under 10 µM Sodium arsenite (NaAsO2) or 100 µM Sodium arsenate (Na2HAsO4)
Established plants (1-week-old plants grown on agar plates) were exposed to a higher range of As concentration for a week on assay media supplemented with 10 µM NaAsO2, 100 µM Na2HAsO4 or no-treatment control medium. After the exposure period, the fresh weights (FW) of roots and shoots were determined.
2.3. Stress response assay with 0–5 µM Sodium arsenite (NaAsO2) and quantification of As, S and thiol derivatives
Established plants (1-week-old plants grown on agar plates) were exposed to a lower range of As concentration for 9 d on assay plates supplemented with 0, 2.5 or 5 µM NaAsO2. After the exposure period, the plants were separated into shoots and roots, rinsed with deionized water and blotted dry, prior to determination of the FWs. Fresh shoot and root samples were individually quantified for concentrations of As, S and thiol derivatives. To determine the concentrations of As and S, the samples were digested in concentrated nitric acid (HNO3) and hydrogen peroxide (H2O2) in a heat block and diluted with 0.08 M HNO3. The concentrations of As and S were determined using an inductively coupled plasma mass spectrometer (ICP-MS, SPQ9700, Hitachi High-Tech Science Co., Tokyo, Japan). To determine thiol concentration, thiols were extracted from frozen tissues and derivatized with monobromobimane (mBBr) following the method of Minocha et al. (Citation2008). Thiol derivatives were separated with Shim-pac FC-ODS (150 × 4.6 mm, Shimadzu, Kyoto, Japan) on High performance liquid chromatography (HPLC) (Shimadzu) and detected by SPD-M20A (Shimadzu). Standards of PCs were synthesized by Bonac cooperation (Fukuoka, Japan), and GSH and Cys were purchased from Wako Pure Chemicals (Osaka, Japan).
3. Results
3.1. SULTR1;2 is required for As tolerance
As a preliminary experiment, growth levels between A. thaliana SULTR mutants and wild types were compared under 10 µM arsenite. A sultr1;2 defective mutant, sel1-8 (Shibagaki et al. Citation2002), was negatively affected in terms of root length and shoot growth compared to other mutants (Supplementary Fig. 1). sel1-8 consistently showed reduced root weight in replicated experiments using 10 µM arsenite and another As condition, 100 µM arsenate (). To further investigate the negative effect of SUTLR1;2-disruption on plant As tolerance, the relative shoot/root FW was measured as an indicator of overall growth under the same As conditions in two allelic mutants, namely sel1-8 (Col-0 background) and sel1-10 (Ws-background; Maruyama-Nakashita et al. Citation2003). Both sel1-8 and sel1-10 exhibited about 50% less growth in roots and shoots compared to their wild types under 10 µM arsenite. This established that SULTR1;2 is required for As tolerance (, ).
Figure 1. Arsenic (As) tolerance in the Arabidopsis thaliana wild type and sultr1;2 defective mutants. (A) One-week-old seedlings were exposed to 10 µM arsenite or 100 µM arsenate conditions for a week. (B, C) Relative fresh weights (FW) of the shoots and roots, per those of Col-0 grown under control condition. Data are geometric means ± standard deviation (SD), n = 4.
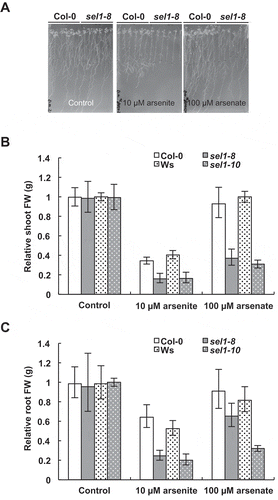
3.2. Increased S uptake in response to As treatments in Col-0 and sel1-8
Next, to investigate the influence of SULTR1;2 knockout in S uptake in response to As, total S accumulation was examined across the given range of As levels and the resulting As accumulation in sel1-8 and Col-0, in shoot and root, individually. In order to confirm the ability of the plants to uptake sulfate and synthesize S-compounds under As conditions, sel1-8 mutants were exposed to arsenite with lower concentrations than those in the tolerance assay. Shoot growth of sel1-8 was 20% less than Col-0 under 5 µM arsenite in comparison, but no significant difference was found under 2.5 µM arsenite between the lines (). The root growth of sel1-8 was 24% and 55% less than that of Col-0 under 2.5 µM and 5 µM arsenite, respectively (). In both sel1-8 and Col-0, concentrations of As in shoots and roots were increased in response to the dose of arsenite in media (, ). There was no significant difference between the lines, except for the mutant-root As accumulation under 5 µM arsenite (). This result indicates that increased sensitivity to arsenite by SULTR1;2 disruption is not due to increased As accumulation. Although not statistically significant, S concentration tended to increase by 20–40% in the roots and shoots of Col-0 by As treatments (, ). This trend of increased S accumulation was also significantly observed in sel1-8 under As stress conditions (, ). These results imply that even without functional SULTR1;2, A. thaliana plants have the ability to accumulate more S in response to As. Meanwhile, shoot S concentration in sel1-8 was always one third of that in Col-0 (), and root S concentration was 30–50% lower in sel1-8 than in Col-0 (). Although the S level is decreased in the mutant, it should be noted that the growth was comparable to that of the wild type under normal conditions ().
Figure 2. Arsenic (As) and sulfur (S) concentrations in the wild type and sultr1;2 defective mutant. One-week-old plants were exposed to 0–5 µmol L−1 As (arsenite) for 9 d. (A, D) Fresh weight, (FW) (B, E) As concentrations and (C, F) S concentrations in shoots and roots. Data are means ± standard deviation (SD), n = 4. Asterisks at each As treatment indicate significant difference (P < 0.05) between Col-0 and sel1-8. In (C) and (F), the different letters in each series (upper case for Col-0 and lower case for sel1-8) indicate a statistically significant difference (P < 0.05, analysis of variance (ANOVA) Tukey’s Honestly Significant Difference (HSD)) between treatments.
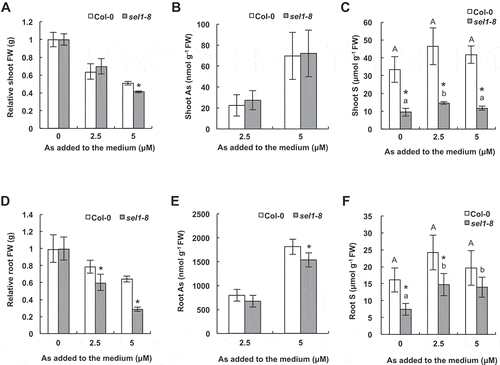
3.3. Excess accumulation of PCs in shoots without those of GSH and Cys in sel1-8
To investigate the thiol profile in response to arsenite, PC and its precursors (GSH and Cys) were quantified by HPLC. The specific peaks of PC2, PC3 and PC4 were detected in both sel1-8 and Col-0 in the presence of arsenite (Supplementary Fig. 2). PC, GSH and Cys levels in both Col-0 and sel1-8 were generally increased by As treatments in a dose-dependent manner except for shoot GSH in sel1-8 treated with 5 µM arsenite, which was comparable to that of 2.5 µM treatment (). Comparing Col-0 and sel1-8, concentrations of these thiols were more or less at similar levels except for PCs in shoots (). This result suggests that synthesis of these S-compounds was basically maintained in the mutant despite a drastic decrease in total S accumulation (, , , , , ). Interestingly, in sel1-8 shoots, accumulation of PCs in response to arsenite was significantly higher compared to Col-0 (). On the other hand, the levels of GSH and Cys in shoots were rather lower in sel1-8 compared to Col-0 (, ).
Figure 3. Thiol concentrations in the wild type and sultr1;2 defective mutant. One-week-old plants of Col-0 and sel1-8 were exposed to 0–5 µmol L−1 As (arsenite) for 9 days. (A, D) Phytochelatins (PCs) (B, E) cysteine (Cys) and (C, F) glutathione (GSH) in shoots and roots. Data are means ± standard deviation (SD), n = 4. Asterisks at each As treatment indicate significant difference (P < 0.05) between Col-0 and sel1-8. The different letters in each series (upper case for Col-0 and lower case for sel1-8) indicate a statistically significant difference (P < 0.05, analysis of variance (ANOVA) Tukey’s Honestly Significant Difference (HSD)) between treatments.
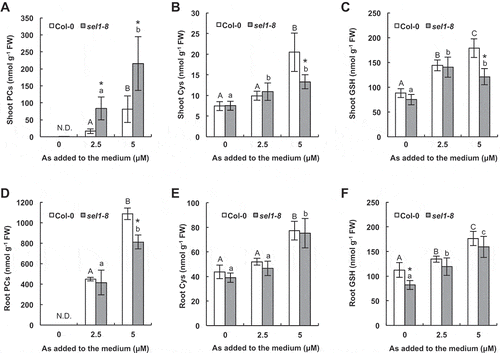
4. Discussion
It has been established that AtPCS1-mediated PC synthesis is crucial for As and other toxic element tolerance in A. thaliana (Howden et al. Citation1995; Ha et al. Citation1999; Tennstedt et al. Citation2009; Fischer et al. Citation2014). Vacuolar sequestration of the PC-metal/metalloid complex also contributes to achieving the metal/metalloid tolerance of Arabidopsis, which is mediated by ATP-binding cassette transporters AtABCC1 and AtABCC2 (Song et al. Citation2010; Park et al. Citation2012). These studies demonstrate the importance of PCs in plant metal/metalloid detoxification. Previous studies on S nutrition and As stresses indicate that up-regulation of SULTR-mediated S uptake is likely to be important for plant As tolerance (Sung et al. Citation2009; Jobe et al. Citation2012; Reid et al. Citation2013; Srivastava et al. Citation2014). These observations imply the significance of S nutrition and transport for supporting substantial synthesis of such Cys-containing metabolites.
The present study explored the possible involvement of Arabidopsis S transporters in As tolerance. Two independent loss-of-function alleles of SULTR1;2, sel1-8 and sel1-10, showed increased sensitivity to As. To exclude the possibility that reduced S uptake in these mutants (, ) simply impairs their basal growth, we compared the growth under normal conditions. The growth of the mutants was comparable to that of the wild type under normal conditions (). Furthermore, in sel1-8, the tissue S level was not decreased, but rather increased by As treatments. These suggest that the As-sensitive phenotype of SULTR1;2 mutants is mainly attributed to As stress, and thus our results demonstrate that SUTLR1;2 is essential for As tolerance in A. thaliana.
We expected poor PC production in these mutants due to insufficient S uptake and supply to cells, which could explain the enhanced As sensitivity of the sultr1;2 mutants. We further investigated thiol profiles of sel1-8 and, surprisingly, found that substantial or rather excess PCs were accumulated in the shoots of sel1-8 exposed to arsenite. Molar ratios of PC-derived thiol to As were then calculated for the wild type and sel1-8 (Supplementary Table S1). Three thiols of PCs are theoretically required for full chelation of one arsenite ion (Schmöger et al. Citation2000). In the wild type shoots, the thiol-to-As ratio was in the range of 1.17–2.24; however, the molar ratio in the mutant shoots was estimated to be more than 5. This calculation suggests that the excess amount of PCs is produced in the mutant shoots. Previous reports have shown that the over-accumulation of PCs can negatively affect cadmium tolerance in AtPCS1-overexpressing A. thaliana (Lee et al. Citation2003; Li et al. Citation2004). The adverse effect of over-accumulated PCs is likely to be because of depletion of GSH, a key metabolite for the cellular redox state. In sel1-8, the loss of As tolerance could be caused by supra-optimal PC accumulation, which would accompany the decline of cellular GSH. But, in fact, accumulations of GSH and Cys in sel1-8 were increased in response to arsenite. Compared to the wild type, GSH and Cys levels of sel1-8 shoots were significantly lower under 5 µM arsenite treatment. Relatively lower GSH and Cys and excess PCs in the mutant shoots indicate an imbalance of the upstream S metabolites to synthesis of PCs, possibly representing a partial disorder of S nutrition under limited S supply due to a defect of SULTR1;2. It is also possible that disruption of SULTR1;2 affects not only S uptake and de novo Cys and GSH synthesis, but also the long-distance transport of the Cys and GSH from roots.
In roots, increased levels of S compounds (PCs, GSH, Cys) under As stresses were less affected by disruption of SULTR1;2, even though As toxicity was more pronounced there than in shoots. Sensitivity of mutant roots toward As toxicity was observed, with an As concentration-dependent manner. Thus, unlike the case in shoots, As toxicity and growth inhibition in the mutant roots appear not to be attributable to an imbalance in the PC-synthetic pathway. As described in comparison, SULTR1;2 might have different roles in roots and shoots. Further analyses are needed to clarify the mechanisms at work in As-sensitive phenotypes of SULTR1;2 mutants.
Plant As tolerance has been improved by the enhancement of PCs synthesis (Li et al. Citation2004). However, the present study demonstrates that increased PC accumulation does not necessarily enhance As tolerance. We propose that an optimal balance between proper PC biosynthesis and plant S nutrient status is important to achieve better As tolerance. Our results for sultr1;2 mutants provide a clue to improve plant As tolerance by bioengineering, such as of PC synthesis. Transcriptomic and metabolomic approaches on the mutant may further dissect mechanisms underlying the regulation of PC and S metabolite production under As stress conditions.
Acknowledgments
We thank Hideki Takahashi (Michigan University) for providing sultr mutant seeds and Shinichi Nakamura (Akita Prefectural University) for providing PC standards. We also thank Stephan Clemens lab (University of Bayreuth) for technical advice on thiol analysis, and Ai Kitazumi and Myles M. Butler (The University of Maine) for the English language review. This work was supported in part by Grants-in-Aid for Scientific Research to TF (No. 25221202) and Grant-in-Aid for JSPS Fellows to SN (No. 13J08293).
Supplementary material
Supplemental data for this article can be accessed here.
References
- Abedin MJ, Cotter-Howells J, Meharg AA 2002: Arsenic uptake and accumulation in rice (Oryza sativa L.) irrigated with contaminated water. Plant Soil, 240, 311–319. doi:10.1023/A:1015792723288
- Barberon M, Berthomieu P, Clairotte M, Shibagaki N, Davidian JC, Gosti F 2008: Unequal functional redundancy between the two Arabidopsis thaliana high-affinity sulfate transporters SULTR1; 1 and SULTR1; 2. New Phytol., 180, 608–619. doi:10.1111/j.1469-8137.2008.02604.x
- Chao DY, Chen Y, Chen J, Shi S, Chen Z, Wang C, Danku JM, Zhao FJ, Salt DE 2014: Genome-wide association mapping identifies a new arsenate reductase enzyme critical for limiting arsenic accumulation in plants. PLoS Biol., 12, e1002009. doi:10.1371/journal.pbio.1002009
- Clemens S 2006: Evolution and function of phytochelatin synthases. J. Plant Physiol., 163, 319–332. doi:10.1016/j.jplph.2005.11.010
- Clemens S, Kim EJ, Neumann D, Schroeder JI 1999: Tolerance to toxic metals by a gene family of phytochelatin synthases from plants and yeast. EMBO J., 18, 3325–3333. doi:10.1093/emboj/18.12.3325
- Fischer S, Kühnlenz T, Thieme M, Schmidt H, Clemens S 2014: Analysis of plant Pb tolerance at realistic submicromolar concentrations demonstrates the role of phytochelatin synthesis for Pb detoxification. Environ. Sci. Technol., 48, 7552–7559. doi:10.1021/es405234p
- Fujiwara T, Hirai MY, Chino M, Komeda Y, Naito S 1992: Effects of sulfur nutrition on expression of the soybean seed storage protein genes in transgenic petunia. Plant Physiol., 99, 263–268. doi:10.1104/pp.99.1.263
- Grill E, Winnacker E-L, Zenk MH 1985: Phytochelatins: the principal heavy-metal complexing peptides of higher plants. Science, 230, 674–676. doi:10.1126/science.230.4726.674
- Ha SB, Smith AP, Howden R, Dietrich WM, Bugg S, O’Connell MJ, Goldsbrough PB, Cobbett CS 1999: Phytochelatin synthase genes from Arabidopsis and the yeast Schizosaccharomyces pombe. Plant Cell, 11(6), 1153–1163. doi:10.1105/tpc.11.6.1153
- Howden R, Goldsbrough PB, Andersen CR, Cobbett CS 1995: Cadmium-sensitive, cad1 mutants of Arabidopsis thaliana are phytochelatin deficient. Plant Physiol., 107, 1059–1066. doi:10.1104/pp.107.4.1059
- Jobe TO, Sung D-Y, Akmakjian G, Pham A, Komives EA, Mendoza-Cózatl DG, Schroeder JI 2012: Feedback inhibition by thiols outranks glutathione depletion: a luciferase-based screen reveals glutathione-deficient γ-ECS and glutathione synthetase mutants impaired in cadmium-induced sulfate assimilation. Plant J., 70, 783–795. doi:10.1111/j.1365-313X.2012.04924.x
- Kamiya T, Islam R, Duan G, Uraguchi S, Fujiwara T 2013: Phosphate deficiency signaling pathway is a target of arsenate and phosphate transporter OsPT1 is involved in As accumulation in shoots of rice. Soil Sci. Plant Nutr., 59, 580–590. doi:10.1080/00380768.2013.804390
- Kamiya T, Tanaka M, Mitani N, Ma JF, Maeshima M, Fujiwara T 2009: NIP1;1, an aquaporin homolog, determines the arsenite sensitivity of Arabidopsis thaliana. J. Biol. Chem., 284, 2114–2120. doi:10.1074/jbc.M806881200
- Khan MA, Stroud JL, Zhu Y-G, McGrath SP, Zhao F-J 2010: Arsenic bioavailability to rice is elevated in Bangladeshi paddy soils. Environ. Sci. Technol., 44, 8515–8521. doi:10.1021/es101952f
- Kühnlenz T, Schmidt H, Uraguchi S, Clemens S 2014: Arabidopsis thaliana phytochelatin synthase 2 is constitutively active in vivo and can rescue the growth defect of the PCS1-deficient cad1-3 mutant on Cd-contaminated soil. J. Exp. Bot., 65, 4241–4253. doi:10.1093/jxb/eru195
- Lee S, Moon JS, Ko TS, Petros D, Goldsbrough PB, Korban SS 2003: Overexpression of Arabidopsis phytochelatin synthase paradoxically leads to hypersensitivity to cadmium stress. Plant Physiol., 131, 656–663. doi:10.1104/pp.014118
- Li Y, Dhankher OP, Carreira L, Lee D, Chen A, Schroeder JI, Balish RS, Meagher RB 2004: Overexpression of phytochelatin synthase in Arabidopsis leads to enhanced arsenic tolerance and cadmium hypersensitivity. Plant Cell Physiol., 45, 1787–1797. doi:10.1093/pcp/pch202
- Ma JF, Yamaji N, Mitani N, Xu XY, Su YH, McGrath SP, Zhao FJ 2008: Transporters of arsenite in rice and their role in arsenic accumulation in rice grain. Proc. Natl. Acad. Sci. USA, 105, 9931–9935. doi:10.1073/pnas.0802361105
- Maruyama-Nakashita A, Inoue E, Watanabe-Takahashi A, Yamaya T, Takahashi H 2003: Transcriptome profiling of sulfur-responsive genes in Arabidopsis reveals global effects of sulfur nutrition on multiple metabolic pathways. Plant Physiol., 132, 597–605. doi:10.1104/pp.102.019802
- Meharg AA 2004: Arsenic in rice – understanding a new disaster for South-East Asia. Trends Plant Sci., 9, 415–417. doi:10.1016/j.tplants.2004.07.002
- Meharg AA, Hartley-Whitaker J 2002: Arsenic uptake and metabolism in arsenic resistant and nonresistant plant species. New Phytol., 154, 29–43. doi:10.1046/j.1469-8137.2002.00363.x
- Mendoza-Cózatl D, Loza-Tavera H, Hernández-Navarro A, Moreno-Sánchez R 2005: Sulfur assimilation and glutathione metabolism under cadmium stress in yeast, protists and plants. FEMS Microbial. Rev., 29, 653–671. doi:10.1016/j.femsre.2004.09.004
- Minocha R, Thangavel P, Dhankher OP, Long S 2008: Separation and quantification of monothiols and phytochelatins from a wide variety of cell cultures and tissues of trees and other plants using high performance liquid chromatography. J. Chromatogr., 1207, 72–83. doi:10.1016/j.chroma.2008.08.023
- Nordstrom DK 2002: Public health – worldwide occurrences of arsenic in ground water. Science, 296, 2143–2145. doi:10.1126/science.1072375
- Panaullah GM, Alam T, Hossain MB, Loeppert RH, Lauren JG, Meisner CA, Ahmed ZU, Duxbury JM 2009: Arsenic toxicity to rice (Oryza sativa L.) in Bangladesh. Plant Soil, 317, 31–39. doi:10.1007/s11104-008-9786-y
- Park J, Song WY, Ko D, Eom Y, Hansen TH, Schiller M, Lee TG, Martinoia E, Lee Y 2012: The phytochelatin transporters AtABCC1 and AtABCC2 mediate tolerance to cadmium and mercury. Plant J., 69, 278–288. doi:10.1111/j.1365-313X.2011.04789.x
- Reid R, Gridley K, Kawamata Y, Zhu Y 2013: Arsenite elicits anomalous sulfur starvation responses in barley. Plant Physiol., 162, 401–409. doi:10.1104/pp.113.216937
- Sánchez-Bermejo E, Castrillo G, Del Llano B et al. 2014: Natural variation in arsenate tolerance identifies an arsenate reductase in Arabidopsis thaliana. Nat. Commun., 5. doi:10.1038/ncomms5617
- Schmöger ME, Oven M, Grill E 2000: Detoxification of arsenic by phytochelatins in plants. Plant Physiol., 122, 793–802. doi:10.1104/pp.122.3.793
- Shibagaki N, Rose A, McDermott JP, Fujiwara T, Hayashi H, Yoneyama T, Davies JP 2002: Selenate-resistant mutants of Arabidopsis thaliana identify Sultr1;2, a sulfate transporter required for efficient transport of sulfate into roots. Plant J., 29, 475–486. doi:10.1046/j.0960-7412.2001.01232.x
- Shin H, Shin HS, Dewbre GR, Harrison MJ 2004: Phosphate transport in Arabidopsis: Pht1;1 and Pht1;4 play a major role in phosphate acquisition from both low- and high-phosphate environments. Plant J., 39, 629–642. doi:10.1111/j.1365-313X.2004.02161.x
- Song WY, Park J, Mendoza-Cózatl DG et al. 2010: Arsenic tolerance in Arabidopsis is mediated by two ABCC-type phytochelatin transporters. Proc. Natl. Acad. Sci. USA, 107, 21187–21192. doi:10.1073/pnas.1013964107
- Srivastava AK, Srivastava S, Mishra S, D’Souza SF, Suprasanna P 2014: Identification of redox-regulated components of arsenate (AsV) tolerance through thiourea supplementation in rice. Metallomics, 6, 1718–1730. doi:10.1039/C4MT00039K
- Sung DY, Kim TH, Komives EA, Mendoza-Cózatl DG, Schroeder JI 2009: ARS5 is a component of the 26S proteasome complex, and negatively regulates thiol biosynthesis and arsenic tolerance in Arabidopsis. Plant J., 59, 802–813. doi:10.1111/j.1365-313X.2009.03914.x
- Takahashi H, Kopriva S, Giordano M, Saito K, Hell R 2011: Sulfur assimilation in photosynthetic organisms: molecular functions and regulations of transporters and assimilatory enzymes. Annu. Rev. Plant Biol., 62, 157–184. doi:10.1146/annurev-arplant-042110-103921
- Tennstedt P, Peisker D, Böttcher C, Trampczynska A, Clemens S 2009: Phytochelatin synthesis is essential for the detoxification of excess zinc and contributes significantly to the accumulation of zinc. Plant Physiol., 149, 938–948. doi:10.1104/pp.108.127472
- Vatamaniuk OK, Bucher EA, Ward JT, Rea PA 2001: A new pathway for heavy metal detoxification in animals. Phytochelatin synthase is required for cadmium tolerance in Caenorhabditis elegans. J. Biol. Chem., 276, 20817–20820. doi:10.1074/jbc.C100152200
- Vatamaniuk OK, Mari S, Lang A, Chalasani S, Demkiv LO, Rea PA 2004: Phytochelatin synthase, a dipeptidyltransferase that undergoes multisite acylation with γ-Glutamylcysteine during catalysis. Stoichiometric and site-directed mutagenic analysis of Arabidopsis thaliana PCS1-catalyzed phytochelatin synthesis. J. Biol. Chem., 279, 22449–22460. doi:10.1074/jbc.M313142200
- Vatamaniuk OK, Mari S, Lu YP, Rea PA 2000: Mechanism of heavy metal ion activation of phytochelatin (PC) synthase Blocked thiols are sufficient for PC synthase-catalyzed transpeptidation of glutathione and related thiol peptides. J. Biol. Chem., 275, 31451–31459. doi:10.1074/jbc.M002997200
- Wu Z, Ren H, McGrath SP, Wu P, Zhao F-J 2011: Investigating the contribution of the phosphate transport pathway to arsenic accumulation in rice. Plant Physiol., 157, 498–508. doi:10.1104/pp.111.178921
- Yoshimoto N, Inoue E, Watanabe-Takahashi A, Saito K, Takahashi H 2007: Posttranscriptional regulation of high-affinity sulfate transporters in Arabidopsis by sulfur nutrition. Plant Physiol., 145, 378–388. doi:10.1104/pp.107.105742
- Yoshimoto N, Takahashi H, Smith FW, Yamaya T, Saito K 2002: Two distinct high-affinity sulfate transporters with different inducibilities mediate uptake of sulfate in Arabidopsis roots. Plant J., 29, 465–473. doi:10.1046/j.0960-7412.2001.01231.x