ABSTRACT
Solarization makes a great impact on the abundance of ammonia oxidizers and nitrifying activity in soil. To elucidate fluctuations in the abundance of ammonia oxidizers and nitrification in solarized soil, copy numbers of amoA gene of ammonia-oxidizing bacteria (AOB) and archaea (AOA), viable number of ammonia oxidizers and inorganic nitrogen contents were investigated in greenhouse experiments. The copy number of amoA gene and the viable number of ammonia oxidizers were determined by the quantitative polymerase chain reaction and most probable number methods, respectively. Abundance of AOB based on the estimation of amoA gene copy numbers and viable counts of ammonia oxidizers was decreased by the solarization treatment and increased during the tomato (Solanum lycopersicum L.) cultivation period following the solarization. Effect of solarization on the copy number of amoA gene of AOA was less evident than that on AOB. The proportion of nitrate in inorganic nitrogen contents was declined by the solarization and increased during the tomato cultivation period following the solarization. Positive correlations were found between the proportion of nitrate in inorganic nitrogen content and the copy number of bacterial or archaeal amoA gene or the viable number of ammonia oxidizers; the copy number of bacterial amoA gene showed a strong correlation with the viable number of ammonia oxidizers. The present study revealed influences of solarization on the fluctuation in the abundance of ammonia oxidizers and dynamics of inorganic nitrogen contents in soil and the results indicate that the determination of amoA gene of AOB is possibly a quick and useful diagnostic technique for evaluating suppression and restoration of nitrification following solarization.
1. Introduction
Soil-disinfection techniques consist of chemical methods using agrochemicals and physical methods mainly by heating. The physical methods contain steam sterilization, hot water sterilization, and solarization (Kita Citation2006). Solarization is an environment-friendly and cost-effective soil disinfection technique, which is an alternative to soil fumigation with methyl bromide.
High soil temperature achieved by the solarization for the disinfestation of plant pathogens and pests (Katan Citation1981; Stapleton Citation2000) makes a great impact on soil microorganisms and their functions besides pathogenic microorganisms. It is well known that soil disinfection treatments inhibit the nitrification process, which supplies nitrate to plants. Rapid recovery of nitrification following soil disinfection is important to prevent growth retardation of crops by ammonium accumulation in the disinfested soil (Morikuni et al. Citation1999; Nishihara Citation2008). As for the solarization, inhibition and subsequent recovery of nitrification in soil have been already reported (Nishihara Citation2008; Wada et al. Citation2008; Ihara et al. Citation2014). Many studies reported a profound inhibition of nitrification concomitant with great decrease in the viable counts of ammonia oxidizers estimated by the most probable number (MPN) method by soil disinfection. Subsequent restoration of the nitrification was associated with recovery of the viable counts of ammonia oxidizers (Suzuki and Watanabe Citation1966; Suzuki et al. Citation1968; Tagawa and Tomaru Citation1969; Takei et al. Citation1974; Kato et al. Citation1984; Morikuni et al. Citation1999). Close relationships between the viable number of ammonia oxidizers estimated by the MPN method and nitrifying activity of soil after soil sterilization have been well documented (Watanabe Citation1974). The inhibition and recovery of nitrifiers were evaluated based on the viable counts by the MPN method (Nishihara Citation2008; Wada et al. Citation2008) and the nitrifying activity (Ihara et al. Citation2014), respectively.
Ammonia-oxidizing bacteria (AOB) and ammonia-oxidizing archaea (AOA) often play a key role in the nitrification process. Yokoe et al. (Citation2015) demonstrated a decrease in the copy number of amoA gene of AOB in soil by the solarization and a subsequent recovery of the number by the quantitative PCR (qPCR) method for the first time. This finding indicates that the qPCR estimation of the amoA gene of AOB is possibly a quick and useful diagnosis of the decline and recovery of ammonia oxidizers over the MPN method that takes about 1 month to obtain the viable counts. Recently, side effects of pesticides on ammonia oxidizers have been evaluated by qPCR determination of amoA transcripts and gene for AOB and AOA (Feld et al. Citation2015). However, the change in AOB abundance estimated by the qPCR method together with fluctuation in nitrifying activity by soil disinfections, not only solarization but also fumigation or steam sterilization, has not yet been investigated so far.
The objectives of the present study were to elucidate fluctuations in the abundance of ammonia oxidizers estimated by the qPCR and MPN methods and dynamics of inorganic nitrogen (N) contents in the solarized soil and to show a possibility of the molecular technique, the qPCR determination of amoA gene of ammonia oxidizers, for diagnosing suppression and restoration of nitrification following soil solarization.
2. Materials and methods
2.1. Study site
The greenhouse experiments with tomato (Solanum lycopersicum L.) cultivation were conducted in a greenhouse at Miyazaki Agricultural Research Institute, Miyazaki, Japan (32.004°N, 131.463°E) and in a greenhouse with isolated bed culture in the Nagoya University Farm (NUF), Togo, Aichi, Japan (35.110°N, 137.082°E).
2.1.1. Miyazaki Agricultural Research Institute (Miyazaki)
The site was the same as that in the previous study (Murase et al. Citation2015), but in a different greenhouse from the previous one. The soil was Typic Fluvaquent and the pH (H2O) was 6.0. Three plots with a randomized arrangement in triplicate (3.53 m2 each) were used: plots applied with liquid fertilizer prepared from distillery waste treatment of barley shochu (1 × 104 kg ha−1 [fresh weight], N–P2O5–K2O = 2.0–0.6–0.4%; Japanese liquor; Unkai Shuzo Co. Ltd., Miyazaki, Japan) before solarization (on 18 July, Sb) and after solarization (on 29 August, Sa) and the control plot (NS) applied with the liquid fertilizer (1 × 104 kg ha−1 [fresh weight]) on 29 August 2014. The solarization treatment was conducted for 33 days from 18 July to 20 August as explained in detail by Murase et al. (Citation2015). The treatment with a laminated aluminum sheet to prevent elevation of temperature was performed for the plot NS in the similar procedure and the plot NS was designated as a non-solarized plot. Tomato seedlings were planted on 22 September. Soil temperatures at 15 cm depth in the plots Sb and NS were measured every 10 min from 6 to 20 August with a temperature data logger (Ondotori Jr. RTR-502, T&D Corporation, Matsumoto, Japan).
Soil samples (about 500 g) were collected from the depth of 0–10 cm (at the middle of tomato plant hills in the tomato cultivation period) using a trowel on 18 July (before solarization [and before fertilization for the plot Sb]), 4 August (midpoint of the solarization period), 20 August (termination of solarization), 19 September (about 1 month after termination of solarization), and 28 November (about 10 weeks after termination of solarization). The soil samples were sieved through a 2-mm mesh screen, kept at 4°C, and subjected to the microbial and inorganic N analyses within a week. The soil samples for the DNA analysis were stored at −20°C until use.
2.1.2. Nagoya university farm
The greenhouse in the NUF was the same as that in the previous study and details of the bed culture, solarization, and soil managements were already described (Yokoe et al. Citation2015). The soil was Hapludult and the pH (H2O) was 5.9–7.4. Briefly, the greenhouse consisted of three beds (0.85 m width × 26 m length × 0.3 m depth; Kumiai Drain Bed, Katakura Chikkarin, Tokyo, Japan) with application (2 × 104 kg ha−1 [fresh weight]) of cattle manure compost (prepared in-house; bed A), compost of sewage sludge from food industry (N–P2O5–K2O = 3.5–4.6–1.8%, UT Compo, Kinuura Utility, Hekinan, Japan; bed B), and ‘bokashi’ compost of animal and plant wastes (N–P2O5–K2O = 4.0–5.5–3.0%, Aging, Chlorella Industry, Tokyo, Japan; bed C). Tomato plants were cultivated twice a year from February to June and from August to December. The soil solarization has been performed in the greenhouse annually since 2004 and was conducted for 48 days from 18 June to 5 August in 2014. The soil was fertilized and plowed on 5 August and tomato seedlings were planted on 11 August. Soil temperatures at 7.5 and 15 cm (at the bottom of the bed) depths were measured hourly throughout the solarization period at the center of the beds with a temperature data logger (Ondotori Jr. TR-52i, T&D Corporation).
Soil samples were collected at three points in each bed (located about 6 m apart) from the depths of 0–5, 5–10, and 10–15 cm (at the middle of tomato plant hills in the tomato cultivation period) using three sampling cores (DIK-1801 100 mL stainless steel sampling tube; Daiki Rika Kogyo, Tokyo, Japan) on 27 May (under cultivation of tomato; only from bed B), 18 June (before solarization), 9 July (midpoint of the solarization period), 5 August (termination of solarization [before fertilization]), 2 September (4 weeks after planting of tomato seedlings), and 21 October (11 weeks after planting of tomato seedlings). The three soil samples from each depth were mixed for each bed, sieved through a 2-mm mesh screen, kept at 4°C, and subjected to the microbial and inorganic N analyses within a week. The soil samples for the DNA analysis were stored at −20°C until use.
2.2. DNA extraction from soil
DNA for qPCR was extracted from 0.5 g of soil samples using the ISOIL for Beads Beating Kit for soil (NIPPON GENE, Tokyo, Japan) by the bead-beating method according to the manufacture’s instruction with two replicates. The extracted DNA was dissolved in 100 µL of TE buffer (10 mM Tris–HCl, 1 mM EDTA; pH 8.0).
2.3. Quantification of amoA of AOB and AOA
amoA genes of AOB and AOA were quantified using the primer pairs amoA1F/amoA2IR according to the method by Morimoto et al. (Citation2011) as described by Yokoe et al. (Citation2015) and AOAamoA19IF/CrenamoA616r according to the method by Tourna et al. (Citation2008) and Morimoto et al. (Citation2011) as described by Li et al. (Citation2014), respectively, with a Thermal Cycler Dice Real Time System (TaKaRa, Otsu, Japan).
2.4. Enumeration of viable number of ammonia oxidizer
The 10-g moist soil sample was dispersed in 90 mL of 10 mM Tris–HCl (pH 7.2) using a blender (HOMO BLENDOR, Sakuma, Tokyo, Japan) at the maximum speed (16,000–18,000 rpm) for 3 min (Kanazawa et al. Citation1986) and a 10-fold dilution series was prepared with the same buffer. A quantity of 0.5 mL of a 10−2–10−8-fold diluted suspension with five replicates per dilution was inoculated to 1.5 mL of the medium for enumeration of ammonia oxidizers (Kimura Citation1992) in a 24-well microtitration plate (Wada et al. Citation2008) and the plate was incubated at 30°C for 4 weeks under dark conditions. Growth of ammonia oxidizers was judged on the production of nitrite and nitrate using Griess Ilosuvay reagent and zinc powder, respectively, and viable numbers of ammonia oxidizers were calculated from a table of MPNs for 5 replicates per dilution at a dilution ratio of 10 according to Kimura (Citation1992).
2.5. Inorganic nitrogen content in soil
The 10-g moist soil was suspended in 90 mL of 2 M KCl solution for 1 h by shaking and the suspension was filtered with a filter paper (Advantec no. 6, Toyo Roshi, Tokyo, Japan) for extraction of ammonium-N. The concentration of ammonium-N in the filtrate was determined by the indophenol blue method using a UV spectrophotometer (UV-2450, Shimadzu, Kyoto, Japan) (Hidaka Citation1997). For extraction of nitrite and nitrate, the 10-g moist soil was suspended in 50 mL of distilled water for 1 h by shaking (Kamewada Citation1997) and the suspension was filtered with a filter paper (Advantec no. 6) and then a membrane filter (pore size 0.45 µm; Advantec Dismic-25CS). The concentrations of nitrite- and nitrate-N were measured with an ion chromatograph (PIA-1000, Shimadzu) as described by Baba et al. (Citation2016). Extraction was conducted each for triplicate soil samples from Miyazaki and it was duplicated for each soil sample from NUF. Proportion of nitrate to inorganic N content was calculated.
2.6. Statistical analysis
ANOVA, Tukey test, paired t-test, Friedman test, and correlation analysis were performed using the PAST program (Hammer et al. Citation2001). Logarithmically transformed values of the copy numbers of amoA gene and viable numbers of ammonia oxidizers were used for the tests.
3. Results
3.1. Elevation of temperature by solarization
Maximum soil temperatures above 40°C were recorded in 14–41 days in the plot Sb of Miyazaki at 15 cm depth and the beds of NUF at 7.5 and 15 cm depths. The temperature was significantly higher at 7.5 cm depth than at 15 cm depth in the beds of NUF (P < 0.001, paired t-test). In the plot NS of Miyazaki, the temperature ranged between 35 and 40°C in most days and the maximum temperatures above 40°C were recorded only in 5 days (Fig. S1).
3.2. Fluctuation in abundance of ammonia oxidizer
3.2.1. Copy number of bacterial amoA gene
Copy number of ammonia-oxidizing bacterial amoA gene was conspicuously decreased to one-tenth or less of that before the solarization, even at the depth of 10–15 cm in the beds of NUF, by the solarization treatment ( and ). A significant difference (P < 0.01 or P < 0.001) was found among the treatments or the sampling periods for the data in Miyazaki (Table S1). Among the treatments, the plots Sa and Sb showed significantly lower copy numbers than the plot NS on 4 and 20 August and 19 September (P < 0.01, Tukey test). The copy number was significantly lower at the termination of solarization on 20 August than the number before the solarization on 18 July in the plots Sb and Sa (P < 0.01, paired t-test) and the number in the tomato cultivation period after the solarization on 28 November in the plot Sa (P < 0.05, paired t-test). A significant difference (P < 0.01 or P < 0.001) was found for the data in each bed of NUF among the sampling periods (Table S1). The copy number was significantly lower at the termination of solarization on 5 August than the number before solarization on 18 June (P < 0.01, paired t-test) and the number in the tomato cultivation period after solarization on 21 October (P < 0.001 [bed A] or P < 0.05 [beds B and C], paired t-test). In contrast, the decrease during the solarization in the plot NS of Miyazaki was not significant (P = 0.06, paired t-test) and within one order of magnitude from 3.1 × 108 (18 July) to 3.5 × 107 (20 August) copies g−1 soil, though the number on 20 August was significantly lower than the number on 28 November (P < 0.01, paired t-test).
Figure 1. Abundance of copy numbers of amoA gene of ammonia-oxidizing bacteria and archaea, viable number of ammonia oxidizers, and contents of ammonium- and nitrate-nitrogen in solarized soils in the plots of Miyazaki. Open circles, open diamonds, closed circles, open triangles, and open squares indicate copy numbers of amoA of ammonia-oxidizing bacteria, ammonia-oxidizing archaea, viable number of ammonia oxidizers, contents of ammonium- and nitrate-nitrogen, respectively. Bars show standard errors of triplicate plots. Numbers marked with # are below the detection limit of most probable number method (about 36 cells g−1 of moist soil).
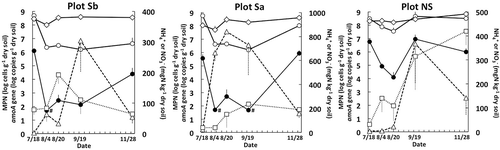
Figure 2. Abundance of copy numbers of amoA gene of ammonia-oxidizing bacteria and archaea, viable number of ammonia oxidizers, and contents of ammonium- and nitrate-nitrogen in solarized soils in the beds of Nagoya University Farm. Open circles, open diamonds, closed circles, open triangles, and open squares indicate copy numbers of amoA of ammonia-oxidizing bacteria, ammonia-oxidizing archaea, viable number of ammonia oxidizers, contents of ammonium- and nitrate-nitrogen, respectively. Numbers marked with # are below the detection limit of most probable number method (about 36 cells g−1 of moist soil).
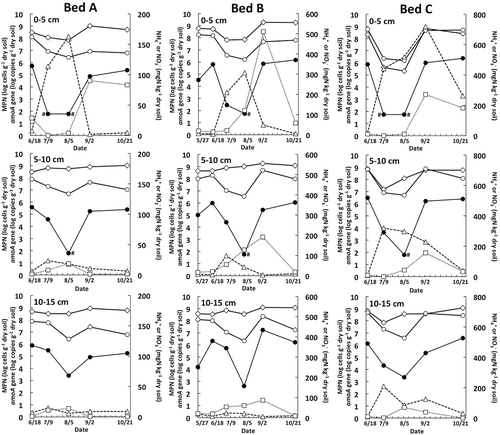
3.2.2. Copy number of archaeal amoA gene
The decrease in the copy number of amoA gene of AOA was less evident than that of AOB. The decrease was within one order of magnitude at most at the depth of 0–5 cm in the beds A and B of NUF and the plots Sb and Sa of Miyazaki ( and ). The copy number of amoA gene of AOA in the beds A and B of NUF was not significantly different among sampling dates (P > 0.05; Table S1). A significant difference was found among sampling dates in the plots of Miyazaki (P < 0.001) and the bed C of NUF (P < 0.05) (Table S1). The copy number in the bed C of NUF showed a different trend (). The decrease in the copy number of amoA gene of AOA was almost same as the decrease in the copy number of amoA gene of AOB from 18 June (before the solarization) to 9 July (midpoint of the solarization period). The decrease in the number from 18 June to 9 July in the bed C was not significant (P = 0.07, paired t-test). The copy number of amoA gene of AOA at the termination of solarization on 20 August (Miyazaki) or 5 August (NUF) was not significantly different from the numbers before the solarization on 18 July (Miyazaki) or 18 June (NUF) and in the tomato cultivation period after the solarization on 28 November (Miyazaki) or 21 October (NUF), respectively (P > 0.05, paired t-test). An exception was that the number in the plot NS of Miyazaki on 28 November was slightly higher than the number on 20 August (P < 0.05, paired t-test).
3.2.3. Viable number of ammonia oxidizer (MPN)
Viable number of ammonia oxidizers (MPN) was greatly decreased by the solarization. The number was decreased to below the detection limit (about 36 cells g−1 of moist soil) of the MPN method on 4 August (midpoint of the solarization period) in the plots Sb and Sa of Miyazaki and on 5 August (termination of the solarization) at 0–5 cm and 5–10 cm depths in the beds of NUF ( and ). The number was significantly different among the treatments in Miyazaki (P < 0.001; Table S1) and was lower in the plots Sa and Sb than in the plot NS on 4 and 20 August and 19 September (P < 0.01 or P < 0.001, Tukey test). The numbers were significantly different among the sampling dates (P < 0.01 or P < 0.001; Table S1). The number at the termination of solarization on 20 August (Miyazaki) or 5 August (NUF) was significantly lower than the number before the solarization on 18 July (Miyazaki; P < 0.01 [plot Sa] or P < 0.05 [plots Sb and NS], paired t-test) or 18 June (NUF; P < 0.01 [bed B] or P < 0.05 [beds A and C], paired t-test). The number in the tomato cultivation period after the solarization on 28 November (Miyazaki) or 21 October (NUF) was higher than the number on 20 August (Miyazaki; P < 0.01 [plot NS] or P < 0.05 [plots Sb and Sa], paired t-test) or 5 August (NUF; P < 0.01 [bed B] or P < 0.05 [beds A and C], paired t-test), where the number in the plot NS at the midpoint of the solarization period on 4 August was on the level of 104 cells g−1 dry soil ().
3.3. Inorganic N content in soil
Content of ammonium-N in soil tended to be increased by the solarization and decreased after the solarization concomitant with increase in the content of nitrate in soil ( and ), where the nitrite content was almost a trace or below the detection limit. However, the fluctuation in values of the ammonium- and nitrate-N contents in soil could be influenced by the chemical property and application time of organic fertilizers as well as the nitrifying activity of the soil. This may make it difficult to infer nitrifying activity of soil simply based on fluctuation in the values of ammonium-N or nitrate-N content. Therefore, proportion of nitrate to inorganic N content in soil (Kato et al. Citation1984) instead of the absolute quantities of ammonium-N or nitrate-N was used as an alternative indicator of the nitrifying activity. The proportion values in the plots Sb and Sa of Miyazaki and in the beds A, B, and C at 0–5 cm soil depth of NUF were significantly different among the sampling dates (P < 0.05, Friedman test) and the values are shown in . The proportion value tended to decrease by the solarization and increase during tomato cultivation periods after the solarization. The values that showed the lowest among the sampling dates, on 4 August (plot Sa; midpoint of the solarization period) and 19 September (plot Sb; 1 month after termination of solarization), in Miyazaki () were significantly lower than the values before the solarization on 18 July, respectively (P < 0.05 based on the Studentized range). The difference between 4 August and 28 November (10 weeks after termination of solarization) in the plot Sa was also significant (P < 0.05 based on the Studentized range). In the beds of NUF at 0–5 cm soil depth, the lowest value was observed at the midpoint of the solarization period on 9 July () and was significantly lower than the values in the tomato cultivation period after the solarization on 21 October (P < 0.05 based on the Studentized range).
Figure 3. Proportion of nitrate in inorganic nitrogen content in solarized soils in the plots of Miyazaki (a) and the beds at 0–5 cm soil depth of Nagoya University Farm (b). Circles, triangles, and squares denote plots Sb, Sa, and NS (a) and beds A, B, and C (b), respectively. Bars show standard errors of triplicate plots of Miyazaki (a).
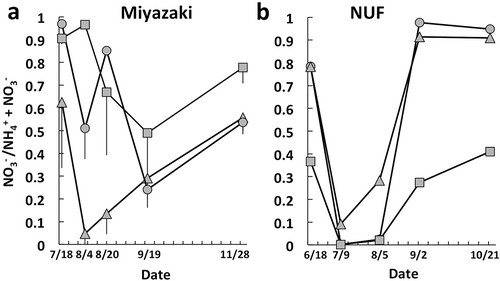
4. Discussion
Maximum temperatures above 40°C were achieved for 2 weeks in all the plots of Miyazaki and the beds of NUF except for the plot NS of Miyazaki (Fig. S1). Fukui et al. (Citation1981) reported that 14 days with the maximum temperature above 40°C were needed to disinfect Fusarium oxysporum f. sp. fragariae and the lethal condition was generally applicable to most of other soil-borne pathogens. Therefore, the solarization treatment in the present experiments seemed to be almost sufficient for controlling soil-borne diseases. The temperature condition ranging between 35 and 40°C except for 5 days in the plot NS was not lethal enough to disinfect soil-borne pathogens. Although the plot NS was the control plot with the treatment for preventing temperature elevation by solarization, the moderate elevation of temperature may have slightly affected nitrification in the soil.
Viable number of ammonia oxidizers (MPN) was greatly decreased by the solarization and the decrease to below the detection limit of the MPN method was observed in the plots of Miyazaki and the beds of NUF except for the plot NS and at the depth of 10–15 cm of NUF ( and ). Many investigations have shown the detrimental effect of soil disinfestation on ammonia oxidizers by the MPN method. Nishihara (Citation2008) and Wada et al. (Citation2008) also reported the influences on the viable counts of ammonia oxidizers with the decrease to less than 1/100–1/1000 by solarization treatments. The present study confirmed the findings in the previous studies that solarization decreased viable number of ammonia oxidizers. The number in the plot NS was also decreased about three orders of magnitude (). The elevation of temperature up to around 40°C (max. 41.7°C) in the NS plot, although it was a control plot, may have affected the viable number of ammonia oxidizers because Myers (Citation1975) reported that nitrification rate in soil declined rapidly at the incubation temperature over 35°C.
Copy number of amoA gene of AOB was decreased to less than 1/10–1/100 by the solarization in the plots of Miyazaki and the beds of NUF except for the plot NS ( and ). The decreasing trend of the copy number by the solarization in the beds of NUF was almost same as that in the same field reported by Yokoe et al. (Citation2015). These findings confirmed that the great impact on AOB abundance by the solarization was detected at the amoA gene level. On the other hand, the copy number also tended to decrease in the plot NS of Miyazaki though the decrease was not significant (P = 0.06) and within one order of magnitude, which was much smaller than the decreases in the plots Sb and Sa. The soil temperature in the plot NS reached to around 40°C. This elevation of temperature may have slightly affected the AOB abundance based on amoA gene analysis since Xu et al. (Citation2017) evaluated the growth of AOB in the incubation of an acidic soil based on quantification of the amoA gene and found that it was strongly inhibited at 40°C.
The decrease in the copy number of amoA gene of AOA was less than that of AOB ( and ), indicating that AOA exhibited somewhat thermotolerant traits. Among seven AOA strains/enrichment cultures isolated from soil environments so far (Lehtovirta-Morley et al. Citation2016), the maximum growth temperature was 45°C (‘Candidatus Nitorosocosmicus franklandus’ C13) (Lehtovirta-Morley et al. Citation2016) to 47°C (Nitrososphaera viennensis EN76) (Tourna et al. Citation2011). AOA might have a little higher thermotolerance than AOB since typical AOB in soil have been generally recognized as mesophiles (Hatzenpichler Citation2012). The AOA members inhabiting the soils in the present study need to be examined for further investigation.
The decreasing and increasing trends in the proportion of nitrate to inorganic N content in soil were observed in the periods of the solarization and tomato cultivation after the treatment (). These findings indicate that nitrifying activity was suppressed by the solarization and gradually recovered during the tomato cultivation subsequent to the solarization. Accumulation of ammonium-N after solarization (Ihara et al. Citation2014) and after soil disinfection treatments such as steam sterilization (e.g., Morikuni et al. Citation1999; Tanaka et al. Citation2003) has been reported in several studies and the accumulation kept till 4 months (Tanaka et al. Citation2003). The proportion value in the plot Sb of Miyazaki after 3 months of the solarization treatment was not fully recovered to the same degree before the treatment (). Recovery of the abundance of AOB was a little retarded in the plot Sb compared with the plot Sa () and this may have been related to the increasing trend of the proportion value in the plot. In addition, the bed C of NUF showed lower proportion values than those in the beds A and B (). Some properties of organic fertilizers, i.e., the liquid fertilizer prepared from distillery waste treatment in the plots of Miyazaki and the ‘bokashi’ compost in the bed C of NUF, might have affected nitrification in the soils.
A positive correlation was found between the proportions of nitrate in inorganic N content and the viable number of ammonia oxidizers estimated by the MPN method in the plots of Miyazaki and the beds of NUF at the depth of 0–5 cm (Fig. S2; r = 0.663, P < 0.001). In addition, copy numbers of amoA gene of AOB and AOA exhibited positive correlations with the proportion (Fig. S2; r = 0.468 [P < 0.01] and 0.610 [P < 0.001], respectively). These findings indicate that not only the viable number of ammonia oxidizers (MPN) but also the copy numbers of amoA gene could be a potential indicator of nitrifying activity of the soils though the gene copy number would represent the abundance of existent community of ammonia oxidizers, not the abundance of the active community. Relationships between the copy numbers of amoA gene of AOB and AOA and the viable numbers of ammonia oxidizers by the MPN method in all the plots of Miyazaki and the beds of NUF are shown in . Both the copy numbers showed positive correlations with the viable number (P < 0.001) and the correlation coefficient for AOB (r = 0.838) was significantly higher than that for AOA (r = 0.534) (P < 0.001). Contribution of AOA to ammonia oxidation in soil is still under debate. The preference of AOA for low pH and low ammonium concentrations has been hypothesized (Hatzenpichler Citation2012). The soil conditions with relatively high contents of ammonium-N ( and ) and near neutral pH values (5.9–7.4, Murase et al. Citation2015; Yokoe et al. Citation2015) would have excluded major contribution of AOA to ammonia oxidation of the soils in the present study. Small fluctuation in the copy number of amoA gene except for the bed C of NUF () might have been attributed to the possible mixotrophic growth of AOA (Hatzenpichler Citation2012). In contrast, the copy number of amoA gene of AOB was fluctuated concomitantly with the viable number of ammonia oxidizers (MPN) though range of the fluctuation in the copy number was less than that in the viable number (, , and ). Thus, the abundance of AOB based on qPCR estimation of amoA gene is possibly a diagnostic indicator of suppression and restoration of nitrification followed by solarization, which is an alternative to the abundance of ammonia oxidizers by the MPN method.
Figure 4. Relationships between copy numbers of amoA gene of ammonia-oxidizing bacteria (AOB) and ammonia-oxidizing archaea (AOA), and viable number of ammonia oxidizers (MPN).
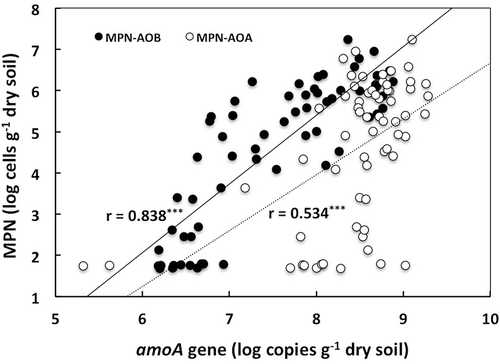
The present study revealed fluctuations in the abundance of ammonia oxidizers estimated by the qPCR and MPN methods and dynamics of inorganic N contents in the solarized soil. The results showed a possibility of the molecular technique, the qPCR determination of amoA gene of AOB, for diagnosing suppression and restoration of nitrification following soil solarization. Because enumeration of ammonia oxidizers by the MPN method takes about 1 month to obtain the viable counts, the qPCR estimation of the amoA gene of AOB is promisingly a quick and useful diagnostic technique for evaluating the effect of solarization on nitrification.
Suppl.zip
Download Zip (2.2 MB)Acknowledgments
We thank Professor Takashi Tsuge of the Graduate School of Bioagricultural Sciences, Nagoya University for helpful advices on the study. This study was supported in part by Science and Technology Research Promotion Program for Agriculture, Forestry, Fisheries and Food Industry (grant no. 25060C).
Supplemental Data
Supplemental data for this article can be accessed here.
Additional information
Funding
References
- Baba R, Asakawa S, Watanabe T 2016: H2-producing bacterial community during rice straw decomposition in paddy field soil: estimation by an analysis of [FeFe]-hydrogenase gene transcripts. Microbes Environ. 31, 226–233. 10.1264/jsme2.ME16036
- Feld L, Hjelmsø MH, Nielsen MS, Jacobsen AD, Rønn R, Ekelund F, Krogh PH, Strobel BW, Jacobsen CS 2015: Pesticide side effects in an agricultural soil ecosystem as measured by amoA expression quantification and bacterial diversity changes. PLoS ONE. 10, e0126080. 10.1371/journal.pone.0126080
- Fukui T, Kodama T, Nakanishi Y 1981: Solar heating sterilization in the closed vinyl house against soil-borne diseases. IV. Solar heating sterilization by polyethylene mulching in the open-field. Bull. Nara Pref. Agric. Exp. Stn. 12, 109–119. (in Japanese with English summary).
- Hammer Ø, Harper DAT, Ryan PD 2001: PAST: paleontological statistics software package for education and data analysis. Palaeontol. Electron. 4, 9.
- Hatzenpichler R 2012: Diversity, physiology, and niche differentiation of ammonia-oxidizing archaea. Appl. Environ. Microbiol. 78, 7501–7510. 10.1128/AEM.01960-12
- Hidaka S 1997: Nitrogen. In Analytical Methods for Soil and Environments, Ed. Konno T, pp. 231–255. Hakuyusha, Tokyo. (in Japanese).
- Ihara H, Kato N, Takahashi S, Nagaoka K 2014: Effect of soil solarization on subsequent nitrification activity at elevated temperatures. Soil Sci. Plant Nutr. 60, 824–831. 10.1080/00380768.2014.947233
- Kamewada K 1997: Water-soluble cation and anion. In Analytical Methods for Soil and Environments, Ed. Konno T, pp. 204–208. Hakuyusha, Tokyo. (in Japanese).
- Kanazawa S, Takeshima S, Ohta K 1986: Effect of Waring blender treatment on the counts of soil microorganims. Soil Sci. Plant Nutr. 32, 81–89. 10.1080/00380768.1986.10557483
- Katan J 1981: Solar heating (solarization) of soil for control of soil-borne pests. Annu. Rev. Phytopathol. 19, 211–236. 10.1146/annurev.py.19.090181.001235
- Kato T, Asano M, Arisawa M, Takei A 1984: Studies on behavior of nutrient in soil resulting from soil disinfection: (III) effect of compost application on recovery of nitrifying population and basal dressing rate of nitrate-nitrogen to ammonium-nitrogen under the soil disinfection. Res. Bull. Aichi Agric. Res. Cent. 16, 219–227. (in Japanese with English summary).
- Kimura R 1992: Enumeration of nitrifying bacteria. In Experimental Methods for Soil Microorganisms, Ed. Japanese Society of Soil Microbiology, pp. 210–212. Yokendo, Tokyo. (in Japanese).
- Kita N 2006: Physical soil sterilization for soil-borne disease control. Proc. Vege. Tea Sci. 3, 7–15. (in Japanese with English summary).
- Lehtovirta-Morley LE, Ross J, Hink L, Weber EB, Gubry-Rangin C, Thion C, Prosser JI, Nicol GW 2016: Isolation of ‘Candidatus Nitrosocosmicus franklandus’, a novel ureolytic soil archaeal ammonia oxidiser with tolerance to high ammonia concentration. FEMS Microbiol. Ecol. 92, fiw057. 10.1093/femsec/fiw057
- Li Y, Watanabe T, Murase J, Asakawa S, Kimura M 2014: Abundance and composition of ammonia oxidizers in response to degradation of root cap cells of rice in soil microcosms. J. Soils Sed. 14, 1587–1598. 10.1007/s11368-014-0910-8
- Morikuni H, Niizuma S, Shimada N 1999: Recovery of nitrifying bacteria after sterilization and the method of nitrogen application to decrease blossom end rot of tomatoes grown in isolated bed. Jpn. J. Soil Sci. Plant Nutr. 70, 542–549. 10.20710/dojo.70.4_542 (in Japanese with English summary).
- Morimoto S, Hayatsu M, Takada-Hoshino Y, Nagaoka K, Yamazaki M, Karasawa T, Takenaka M, Akiyama H 2011: Quantitative analyses of ammonia-oxidizing archaea (AOA) and ammonia-oxidizing bacteria (AOB) in fields with different soil types. Microbes Environ. 26, 248–253. 10.1264/jsme2.ME11127
- Murase J, Shinohara Y, Yokoe K, Matsuda R, Asakawa S, Hashimoto T 2015: Impact of soil solarization on the ciliate community structure of a greenhouse soil. Soil Sci. Plant Nutr. 61, 927–933. 10.1080/00380768.2015.1079799
- Myers RJK 1975: Temperature effects on ammonification and nitrification in a tropical soil. Soil Biol. Biochem. 7, 83–86. 10.1016/0038-0717(75)90003-6
- Nishihara M 2008: The effect of soil disinfection by improved Miyazaki model solar disinfection. Jpn. J. Soil Sci. Plant Nutr. 79, 393–396. 10.20710/dojo.79.4_393 (in Japanese).
- Stapleton JJ 2000: Soil solarization in various agricultural production systems. Crop. Prot. 19, 837–841. 10.1016/S0261-2194(00)00111-3
- Suzuki T, Tokunaga Y, Watanabe I 1968: Re-establishment of nitrifying microorganisms after soil sterilization (Part 2): effect of crop cover on the re-establishment and the source of re-establishing nitrifiers after chloropicrin fumigation. J. Sci. Soil Manure Japan. 39, 224–227. 10.20710/dojo.39.4_224 (in Japanese).
- Suzuki T, Watanabe I 1966: Re-establishment of nitrifying microorganisms in field soil after chloropicrin fumigation applied in different ways: re-establishment of nitrifying microorganisms after soil sterilization (Part 1). J. Sci. Soil Manure Japan. 37, 579–584. 10.20710/dojo.37.12_579 (in Japanese).
- Tagawa H, Tomaru K 1969: The effect of chloropicrin treatment on the soil microflora. Bull. Hatano Tobacco Exp. Stn. 65, 63–75. (in Japanese with English summary).
- Takei A, Hayakawa I, Shimada N 1974: Studies on analysis and improvement of the soil conditions in plastic house and greenhouse: V. Seasonal changes of nitrifying microorganisms. Res. Bull. Aichi Agric. Res. Cent. Ser. B. 6, 25–30. (in Japanese with English summary).
- Tanaka S, Kobayashi T, Iwasaki K, Yamane S, Maeda K, Sakurai K 2003: Properties and metabolic diversity of microbial communities in soils treated with steam sterilization compared with methyl bromide and chloropicrin fumigations. Soil Sci. Plant Nutr. 49, 603–610. 10.1080/00380768.2003.10410050
- Tourna M, Freitag TE, Nicol GW, Prosser JI 2008: Growth, activity and temperature responses of ammonia-oxidizing archaea and bacteria in soil microcosms. Environ. Microbiol. 10, 1357–1364. 10.1111/j.1462-2920.2007.01563.x
- Tourna M, Stieglmeier M, Spang A et al. 2011: Nitrososphaera viennensis, an ammonia oxidizing archaeon from soil. Proc. Natl. Acad. Sci. USA. 108, 8420–8425. 10.1073/pnas.1013488108
- Wada S, Fujino C, Toyota K, Okamoto T 2008: Effect of soil disinfection with 1,3-dichrolopropene, chloropicrin and solarization on cellulose-decomposing ability and nitrifying bacteria. Soil Microorganisms. 62, 21–31. 10.18946/jssm.62.1_21 (in Japanese with English summary).
- Watanabe I 1974: Re-establishment of nitrifying microorganisms after soil sterilization: a statistical study on the relationship between Nitrosomonas population and nitrifying activity of soil (Part 3). J. Sci. Soil Manure, Japan. 45, 279–284. 10.20710/dojo.45.6_279 (in Japanese).
- Xu X, Liu X, Li Y, Ran Y, Liu Y, Zhang Q, Li Z, He Y, Xu J, Di H 2017: High temperatures inhibited the growth of soil bacteria and archaea but not that of fungi and altered nitrous oxide production mechanisms from different nitrogen sources in an acidic soil. Soil Biol. Biochem. 107, 168–179. 10.1016/j.soilbio.2017.01.003
- Yokoe K, Maesaka M, Murase J, Asakawa S 2015: Solarization makes a great impact on the abundance and composition of microbial communities in soil. Soil Sci. Plant Nutr. 61, 641–652. 10.1080/00380768.2015.1048183