ABSTRACT
Flooded rice fields are a significant anthropogenic source of methane (CH4) and nitrous oxide (N2O) from agriculture in Asia, Latin America, and the Caribbean regions. In this work, we comparatively assessed the potential of intermittent irrigation and continuous rice flooding for reducing soil CH4 and N2O emissions, partial global warming potential (pGWP), and its yield-scaled version (YpGWP) in northwestern Japan and southern Brazil. Seasonal CH4 emissions under continuous flooded soils were slight higher in Japan (738 ± 87 kg ha−1) than in Brazil (623 ± 197 kg ha−1), and they were probably related to the higher level of soil organic C and the longer period under flooding in the seedling transplanting system in the Japanese site. Intermittent irrigation had similar efficiency in decreasing soil CH4 emissions in both study areas, with the maximum mitigation potential of 71% in northwestern Japan and of 62% in southern Brazil. No significant difference in seasonal soil N2O emissions (−0.17 ± 0.05 to −0.24 ± 0.06 kg N2O ha−1 in Japan and 0.32 ± 0.08–1.16 ± 0.40 kg ha−1 in Brazil) or rice yield (7328–8064 kg ha−1 in Japan and 9391–10,231 kg ha−1 in Brazil) between irrigation systems was observed in either area. The potential of intermittent irrigation for reducing pGWP was around three times higher than that of continuous flooding in both sites. Thus, a reduction by 47–63% and 62–80% in yield-scaled pGWP was observed in southern Brazil and northwestern Japan, respectively. Like the well-established labor-intensive rice transplanting systems used in Asia, the introduction of feasible irrigation suppression systems in mechanized direct seeding rice fields in southern Brazil and other countries of Latin America and the Caribbean region is an effective choice for reducing greenhouse gas emissions with no adverse impact on rice yield.
1. Introduction
Flood irrigated rice spans an area of approximately 80 million ha and accounts for about 75% of the world’s rice production (IRRI Citation2013). Because of the anoxic soil environment, irrigated rice lowland is a significant anthropogenic source of methane (CH4) and nitrous oxide (N2O), two major greenhouse gases (GHGs) with a global warming potential (GWP) 25 and 298 times, respectively, greater than that of CO2 on a one hundred-year time horizon (IPCC Citation2007).
Although 90% of the world’s irrigated rice is grown in Asia, rice production in Latin America and the Caribbean (LAC) has grown substantially in recent times (at an annual rate of 2.8% over the period 2000–2010 according to GRiSP, 2013). Brazil, which is currently the greatest producer among LAC countries, accounts for nearly one-half of the regional rice production (GRiSP Citation2013). Brazilian rice comes mainly from the subtropic region (southern Brazil). Thus, the Rio Grande do Sul state is a rice granary with a cultivated area of more than 1 million ha (CONAB Citation2015) that accounts not only for nearly 70% of the national production (IBGE Citation2014) but also for 18% of the overall CH4 emissions from the national agricultural sector (SEEG Citation2014). The need of both Asia and LAC to increase rice production in order to fulfill the demands of an increasing population can be met more sustainably by intensifying rice production based on environmental friendly agricultural practices than by expanding the cultivated area.
Studies on soil CH4 mitigation in rice fields are abundant in Asia but scant in the LAC region. In Japan, the most effective way of alleviating soil CH4 emissions involves not only nitrogen fertilization and organic matter application but also the use of effective water management practices such as midseason drainage (MD) and intermittent irrigation to prevent the development of soil reductive conditions (Nishimura et al. Citation2004; Zou et al. Citation2005; Minamikawa et al. Citation2006). In Brazil, research in this field is fairly recent and only a few studies have dealt with the potential of no-till and anticipated tillage (Bayer et al. Citation2014; Bayer et al. Citation2015), and water management (Moterle et al. Citation2013; Zschornack et al. Citation2016) for mitigating soil CH4 emissions in subtropical lowland rice fields. In both world regions, intermittent water management has been found to produce soil N2O emissions similar to or even higher than those of continuous flooded rice fields (Cai et al. Citation1997; Yan et al. Citation2000; Zschornack et al. Citation2016); however, a positive GHG balance was invariably observed (Zschornack et al. Citation2016; Hou et al. Citation2012; Hadi et al. Citation2010).
Partly because of advanced research, the most widely used water management practices in labor-intensive rice transplanting programs in Japan are MD and intermittent irrigation (Minamikawa et al. Citation2006). In contrast, mechanized direct seeding rice in Brazil and LAC region is produced mainly under continuous flooding (CF) because of the uncertainty in the timing and span of water-saving regimes as regards to rice yields (Chirinda et al. Citation2017). Therefore, Asian farmers are currently exploiting the whole potential of effective water management practices for decreasing CH4 emissions, whereas Brazilian farmers are still to explore the ability of these practices to increase rice production and food security without increasing GHG emissions. Although adopting feasible water management systems to mitigate CH4 emissions from rice fields might benefit the whole LAC region, the actual effectiveness of this practice should be better assessed on a more local level.
The scarcity of studies in this direction in Brazil and the LAC region led us to comparatively assess the potential of feasible water management systems for decreasing GHG emissions in southern Brazil and northwestern Japan. Our starting hypothesis was that the potential of intermittent irrigation for decreasing soil CH4 emissions would be similar in both world regions and that the magnitude of the effect would vary with soil and climate. In addition, we hypothesized that the GHG mitigation potential of intermittent water management would be partially offset by increased soil N2O emissions relative to CF and also that properly performed water irrigation suppression would have no negative impact on rice yield.
2. Material and methods
2.1. Sites and field management practices
2.1.1. Southern Brazil
The experiment was conducted in the crop season 2012/13 at the Research Station of the Riograndense Rice Institute (IRGA), Rio Grande do Sul State, southern Brazil. The soil is an Entisol as per the US Soil Taxonomy and the climate is humid subtropical, with warm summers and cool winters. Rainfall is evenly distributed through the year and no definite dry season exists (Peel et al. Citation2007). summarizes the properties of soil and climate in the studied area.
Table 1. Soil and climate characteristics of the studied sites and main characteristics of the experiments.
Soil tillage was performed in the autumn of 2012 and involved one plowing disk pass and two leveling disk passes to a depth of 20 cm. Approximately 6 t ha−1 of rice biomass was incorporated into the soil by tillage. Self-reseeding ryegrass was grown as a cover crop without irrigation during the winter months. A glyphosate-based herbicide at a rate of 3 L ha−1 was applied to the ryegrass in the spring, followed by machine chopping of the standing biomass. Rice was drill seeded and grown under different irrigation treatments during the summer.
Shortly after desiccant herbicide and the first nitrogen fertilization were applied, the field was flooded at rice development stage V3 (three leaves; Counce et al. Citation2000) in all treatments. Three different irrigation treatments were applied during the rice growing season, namely, (a) CF, with which the soil was kept under a 5–10-cm thick water layer throughout the growth season; (b) moderate intermittent irrigation (MI), which involved suppressing water at stages V6–V8 (6–8 leaves); and (c) severe intermittent irrigation (SI), where water was suppressed at stages V6–V8 and V8–V10 (8–10 leaves). Irrigation was suspended 15 days before rice harvest in all treatments.
All cultivation practices followed the technical recommendations for the rice in southern Brazil (Sosbai Citation2014). shows the daily air temperature and rainfall over the rice growing period as obtained from the National Meteorological Institute (INMET) of Brazil. provides additional information about the experimental design, rice variety, and fertilization procedure used.
Figure 1. Seasonal variations in daily rainfall (A,B), daily mean air temperature (C,D), surface water depth (E,F), CH4 flux (G,H), and N2O flux (I,J) under different water management systems at Cachoeirinha in southern Brazil and at Nagaoka in northwestern Japan. Grey areas in A–D indicate lack of observation data. Tp: Transplanting; N: nitrogen fertilization; Hv: harvest. Vertical bars in G–J indicate standard deviation (n = 3). Note: Baseline N application in Japan was done 14 days before transplanting.
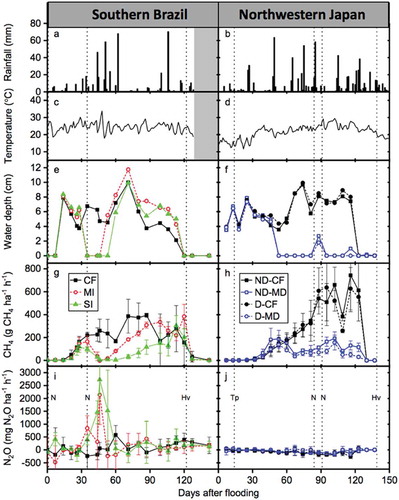
2.1.2. Northwestern Japan
The Asian experiment was conducted at the Niigata Agricultural Research Institute, Niigata Province, northwestern Japan. Niigata Province is on the coastal plain between the mountains and sea in Japan. It has a humid subtropical climate (Cfa as per Peel et al. Citation2007). Off-season is characterized by a cold winter with about 2 m of snowfall. The snow melts during late winter and spring, and rice is grown in summer.
Water management treatments were applied off-season and in the rice season. Off-season, the soil was subjected to two different treatments after the snow had melted, namely, drainage (D) and no drainage (ND). Also, two different irrigation treatments were applied in the rice season, namely, CF, with which the soil was kept under a 5–10-cm thick layer of water throughout the growth season; and mid-summer drainage (MD), which involved flooding before rice was transplanted and was followed by drainage around 15–20 days before maximum tillering, and by intermittent irrigation at 4-day intervals for 20 days before harvest (Shiratori et al. Citation2007), when irrigation was suspended altogether.
In autumn 2013, about 6 t ha−1 rice straw from the previous rice was incorporated into the surface soil layer (10 cm) by using a rotary hoe. In spring (May) 2014, three to four rice seedlings 2.5–2.7 cm high per pit were transplanted to the experimental area after flooding. The area was kept flooded until the seedlings began to grow and then the two irrigation treatments (CF and MD) were applied. Since MD is the irrigation system traditionally used by Japanese farmers, CF was only introduced for comparison of the impact of intermittent irrigation with that in southern Brazil.
The air temperature and rainfall during the rice growing season were measured on a daily basis at the Climate Station of the Niigata Agricultural Research Institute (see ).
2.2. Rice grain yield
Rice grain yield was manually assessed in an area of 10 m in southern Brazil and of 4 m2 in northwestern Japan. All yield data are expressed on a 13% humidity basis.
2.3. GHG measurement and calculations
GHGs were measured weekly by using the static chamber method (Minamikawa et al. Citation2012; Bayer et al. Citation2014) during the rice growing season (November–March in Brazil and May–September in Japan). Acrylic chambers 60 cm long × 30 cm wide × 60 cm high were placed on the soil surface on 60 × 30 × 5 cm PVC bases in northwestern Japan, whereas 60 × 60 × 30 cm aluminum chambers and 60 × 60 × 20 cm bases were used in southern Brazil.
The bases were driven 5 cm deep into the soil before flooding and remained in it throughout the growth season. Each base had an open bottom and sealable channels on the sides for irrigation water to flow freely, all being sealed during air sampling events. Each base covered three rice plant rows in southern Brazil and three rice seedlings in northwestern Japan. Additional 20 or 30 cm extensors were staked on the bases as the rice plants grew in order to accommodate plant growth, and the chamber volume considered in GHG emission calculations.
Each chamber top had a rubber septum sampling port, a stainless steel thermometer, and two or three battery operated fans to circulate and homogenize air inside the chamber (Banker et al. Citation1995). Chamber closing and air sampling were done from 09:00 to 11:00 am each day and involved four samplings (0, 5, 10, and 20 min) in Brazil and three (0, 15, and 30 min) in Japan. Air was drawn with polypropylene syringes (20 mL) and transferred to glass bottles with double wadded silicone caps (Labco Corp.) to determine CH4 and N2O on a gas chromatograph equipped with a flame ionization and electron capture detector.
GHG fluxes were calculated as follows:
where F is the gas emission rate (g CH4 m−2 h−1 or mg N2O m−2 h−1), dC/dt is the variation of the concentration of CH4 or N2O (mol h−1), is the gas molar mass (g mol−1), P is the atmospheric pressure in the chamber (assumed to be 1 atm), V is the chamber volume (L), R is the ideal gas constant (0.08205 atm L mol−1 K−1), T is the chamber temperature (K), and A the chamber basal area (m2). The flux rate of GHG from air sampling at 9:00–11:00 am was assumed to be equivalent to the mean daily flux rate (Costa et al. Citation2008). The gas production rate was subsequently converted into kg CH4 ha−1 day−1 and g N2O ha−1 day−1. Seasonal emissions were calculated by trapezoidal integration of the daily GHG fluxes throughout the growth season (Bayer et al. Citation2014).
The partial GWP (pGWP) was calculated by multiplying the seasonal CH4 and N2O emissions by their respective radiative forcing potential and combining the two:
where pGWP is the partial global warming potential (kg CO2 eq ha−1); CH4 and N2O are the seasonal emissions of methane and nitrous oxide, respectively (kg ha−1); and 25 and 298 are the radiative forcing potential of CH4 and N2O relative to carbon dioxide (CO2) on a 100-year horizon, respectively (IPCC Citation2007).
Yield-scaled pGWP (kg CO2 eq kg−1 grain yield) was calculated as the ratio of pGWP to rice grain yield:
where pGWP is the partial global warming potential (kg CO2 eq ha−1) and Y grain yield (kg ha−1).
2.4. Statistical analyses
Data were visually inspected for normality and constant variance of errors and subjected to appropriated transformations when the assumptions were violated. Analysis of variance was done by using the Mixed Procedure in SAS (SAS Institute Inc., Cary, NC, USA). Fluxes and seasonal soil CH4 and N2O emissions, pGWP, yield-scaled GWP (YpGWP), and grain yield were considered dependent variables, while irrigation systems were used as fixed effects and replication as random effect. The dependent variables seasonal soil CH4 and N2O emissions, pGWP, YpGWP, and grain yield in each site were analyzed by using GLM Procedure in SAS. All differences were deemed significant at the 95% level (P < 0.05) as per Tukey test.
3. Results
3.1. Soil CH4 and N2O fluxes
As can be seen from , mean soil CH4 fluxes differed significantly between irrigation systems in northwestern Japan and southern Brazil, intermittent irrigation during rice growth leading to low CH4 fluxes relative CF in both study areas (see ).
Table 2. Results of the ANOVA of fluxes and seasonal CH4 and N2O emissions, pGWP, and YpGWP in rice fields under different irrigation systems in southern Brazil (continuous flooding, intermediate intermittent, and severe intermittent irrigation) and northwestern Japan (continuous flooding and midsummer drainage in the rice season, and drainage or no drainage off-season).
Soil CH4 fluxes started to increase at 22 after flooding (days after flooding [DAF] in southern Brazil and 32 DAF in northwestern Japan; also, they peaked from 71 to 87 DAF in the former area and from 88 to 123 DAF in the latter (). In general, soil CH4 emissions were maximal at the vegetative and reproductive stages of rice growth with all treatments, but much lower with intermittent irrigation. Thus, the highest CH4 flux with CF in northwestern Japan was roughly twice that in southern Brazil (743 ± 220 vs. 395 ± 17 g ha−1 h−1; ).
Mean soil CH4 fluxes under CF in southern Brazil (173 ± 54 g ha−1 h−1) were significantly reduced by moderate and SI (to 125 ± 2 and 68 ± 22 g CH4 ha−1 h−1, respectively) (). A similar trend in soil CH4 fluxes with CF and intermittent irrigation (MD) was observed in northwestern Japan (). Thus, irrigation with MD was suppressed and a decrease in soil CH4 fluxes observed around 52 DAF. In contrast, a constant increase in CH4 fluxes was observed with CF (). Mean soil CH4 fluxes were higher with ND-CF (235 ± 28 g ha−1 h−1) and D-CF (228 ± 61 g ha−1 h−1) than with ND-MD (81 ± 11 g ha−1 h−1) and D-MD (48 ± 9 g ha−1 h−1) systems.
Off-season field management (D or ND) in northwestern Japan had a significant influence on soil CH4 fluxes during the rice growing season ( and ), the fields where the soil was drained before the rice season exhibiting slightly lower mean soil CH4 fluxes (138 ± 35 g ha−1 h−1 on average for the irrigation treatments applied in the rice growing season) than those which were not drained (158 ± 19 g ha−1 h−1 under CF and MD).
Mean soil N2O fluxes were higher with intermittent irrigation than with CF in both study areas. In northwestern Japan, all irrigation treatments resulted in mostly negative soil N2O fluxes (influxes) that increased from a mean value of −62 ± 7 mg ha−1 h−1 with CF to −45 ± 8 mg ha−1 h−1 with intermittent irrigation; in southern Brazil, mean soil N2O fluxes increased from 65 ± 20 mg ha−1 h−1 with CF to 186 ± 15 and 386 ± 123 mg ha−1 h−1 under MI and SI, respectively.
3.2. Seasonal soil CH4 and N2O emissions
Seasonal soil CH4 emissions also differed significantly between irrigation systems in both northwestern Japan and southern Brazil ( and ), intermittent irrigation resulting in lower emissions than CF.
Table 3. Seasonal CH4 and N2O emissions (±SD values), partial global warming potential (pGWP), grain yield, and yield-scaled pGWP in irrigated rice fields under different irrigation systems in southern Brazil and northwestern Japan.
In northwestern Japan, seasonal soil CH4 emissions under CF amounted to 738 ± 87 kg ha−1 but were lower under ND-MD (159 ± 30 kg ha−1) and D-MD (262 ± 35 kg ha−1). No significant differences in soil CH4 emissions between off-season management systems were observed, however. In southern Brazil, seasonal emissions under CF were 623 ± 196 kg ha−1 (18% lower than in Japan) but lower with MI and SI (466 ± 5 and 237 ± 75 kg ha−1, respectively) (). Therefore, the maximum potential of intermittent irrigation for decreasing soil CH4 emissions was 71% in Japan (mean of drained and not drained soil off-season) and 62% in Brazil (SI).
The amount of seasonal N2O emissions during the rice season was not significantly affected by irrigation system in either study area (). Thus, emissions in southern Brazil ranged from 0.32 ± 0.08 to 1.16 ± 0.40 kg ha−1, whereas those in northwestern Japan consisted solely of N2O influxes to soil and ranged from −0.24 ± 0.06 to −0.09 ± 0.06 kg ha−1 ().
3.3. pGWP and YpGWP
pGWP (kg CO2 eq ha−1), which represents the combination of soil CH4 and N2O emissions accounting for the radiative forcing potential of each gas, was significantly affected by irrigation treatment in both northwestern Japan and southern Brazil ( and ). The mean pGWP values for CF (18,163 ± 2184 kg CO2 eq ha−1 in Japan and 15,670 ± 4869 kg CO2 eq ha−1 in Brazil) were roughly three times higher than those for intermittent irritation (5214 ± 822 kg CO2 eq ha−1 for the former country and 9043 ± 1098 kg CO2 eq ha−1 for the latter). The reduction in pGWP obtained by adopting intermittent irrigation was thus 71% on average in Japan and 42% in Brazil. There were no significant differences in pGWP between off-season management practices, however ().
No significant difference in rice grain yield between irrigation treatments was observed in either study area. Yields ranged from 7328 to 8390 kg ha−1 in northwestern Japan and from 9391 to 10,231 kg ha−1 in southern Brazil. Because intermittent irrigation reduced pGWP but water suppression had no effect on rice grain yield, YpGWP was significantly lower with intermittent irrigation than under CF in both Japan and Brazil ( and ). On average for the two off-season management systems in Japan, producing 1 kg of rice under CF caused the emission of 2.34 ± 0.46 kg CO2 eq to the atmosphere. The corresponding figure for Brazil was 27% lower (1.66 ± 0.52 kg CO2 eq). With intermittent irrigation, GHG emissions per kilogram of rice were low as 0.48 ± 0.09 kg CO2 eq in northwestern Japan and 0.61 ± 0.17 kg CO2 eq in southern Brazil ().
4. Discussion
4.1. Soil CH4 fluxes and seasonal emissions
Soil CH4 fluxes increased with the number of DAF in both northwestern Japan and southern Brazil; fluxes increased as the rice crops developed, with peaks at the vegetative (tillering) and reproductive (panicle initiation) stages. The increase can be ascribed to progress in soil reduction under CF and to increase root exudation (Denier vand der Gon, Neue HAC, Neue HU Citation1995; Lu et al. Citation2000). An apparent slight increase in soil CH4 fluxes was observed in northwestern Japan () that led to their peaking later than in southern Brazil ().
The less marked increase in soil CH4 fluxes in Japanese soil was probably the result of its high content in iron oxides (Fe2O3; ) relative to Brazilian soil since CH4 production by anaerobic decomposition of organic matter in soil only occurs after most substances with a greater affinity as electron acceptors have been reduced. Such substances (Fe3+, Mn4+, and ) are reduced to Fe2+, Mn2+, and S2−, respectively, prior to CH4 production (Yu et al. Citation2007). On the other hand, the fact that soil CH4 peaks were higher in Japan than in Brazil probably resulted from the higher content in organic C of soil in the former () since organic matter is known to be an effective substrate for methanogenic microorganisms (Minamikawa et al. Citation2006). The differences may also have arisen partly from differences in root exudation among rice varieties (Chirinda et al. Citation2017), but this is merely a speculative assumption that requires confirmation.
The less substantial increase in soil CH4 was offset by the highest peaks resulting in higher seasonal soil CH4 emissions under CF in northwestern Japan (738 ± 87 kg ha−1) than in southern Brazil (623±kg ha−1). Since the mean air temperature in the studied period was slightly lower at the Japanese site (22.1°C) than in southern Brazil (24.5°C), the increased CH4 emissions in northwestern Japan may again have resulted from its increased content in soil organic C, which acts as an energy source for methanogenic microorganisms (Itoh et al. Citation2011; Silva et al. Citation2011; Ali et al. Citation2013). However, differences in seeding methods may also have contributed to the higher seasonal soil CH4 emissions observed in northwestern Japan. Unlike transplanting rice in a previously flooded field in Japan, direct seeding in dry soil and flooding fields only after rice has emerged in southern Brazil results in 20–40 fewer days of soil saturation and leads to less favorable conditions for methanogenesis. Previous studies in Asia suggest that soil CH4 emissions associated with direct seeding may be 8-92% lower than those observed from transplanted rice (DeAngelo et al. Citation2006; Kumar and Ladha Citation2011).
In general, while soil CH4 production increased continuously with time under CF, water suppression in intermittent irrigation arrested the emission reduction process in soil and decreased soil CH4 fluxes. Suppressing irrigation facilitates diffusion of O2 from the atmosphere into the soil, thereby promoting re-oxidation of the soil and hindering CH4 production (Zhang et al. Citation2012; Kim et al. Citation2014). In this study, soil CH4 fluxes resumed during intermittent irrigation periods and increased when the soil was re-flooded. This deleterious effect of intermittent irrigation may also have arisen from population changes in methanogenic and methanotrophic microorganisms in the soil as previously observed by Ma and Lu (Citation2011). According to these authors, soil drainage suppresses growth of methanogenic microorganisms, and emission reduction during draining events can be ascribed in part to methanotrophic microorganisms remaining active thanks to O2 availability and CH4 trapping in the soil.
Repeated drainage in northwestern Japan (every 4 days from tillering to 20 days before harvest) decreased seasonal soil CH4 emissions by 71% (mean of the two off-season treatments), which exceeds the reduction observed with a single water suppression event (6–8 leaves) in the MI treatment, 40%, and a little higher than reduction observed with repeated water suppression (6–8 leaves and 8–10 leaves) in the SI treatment (SI) in southern Brazil (62%). The magnitude of the reduction of seasonal soil CH4 emissions under intermittent irrigation is related to the number and duration of the water suppression or drainage events (Zhang et al. Citation2012; Ma et al. Citation2013; Zschornack et al. Citation2016). Thus, water suppression should last long enough to promote soil re-oxidation; also, the more suppression events during the rice growing season, the more efficient intermittent irrigation in decreasing soil CH4 emissions (Towprayoon et al. Citation2005). Since soil reduction is quite a slow process, the decrease in seasonal soil CH4 emissions is usually more marked with repeated events of water suppression or drainage than with a single one (Zhang et al. Citation2012).
In addition to soil and climate, the efficiency with which soil CH4 emissions are reduced in different parts of the world is influenced by the design of the intermittent irrigation system (particularly by frequency and duration of water suppression or drainage events). Based on the scant available literature on the topic, the potential of intermittent irrigation systems for decreasing soil CH4 emissions exceeds that of CF by 40–85% in southern Brazil (Moterle et al. Citation2013; Zschornack et al. Citation2016). This range is similar to the values of 12–88% derived from massive literature from Japan and other countries on the Asian Continent (Malyan et al. Citation2016).
Although no significant difference in seasonal soil CH4 emissions between off-season treatments (D or ND) was observed, the low emission levels from drained soil (720 kg ha−1 under CF and 159 kg ha−1 under MD) relative to undrained soil (738 kg ha−1 under CF and 262 kg ha−1 under MD) apparently follow the same logic as the adoption of intermittent irrigation in the rice growing season. According to Shiratori et al. (Citation2007), draining fields off-season causes stronger decomposition of organic compounds in soil owing to the prevalence of aerobic conditions; as a result, it decreases the amount of C available as a substrate for methanogenic microorganisms under anaerobic conditions in the rice growing season. Also, off-season soil drainage promotes re-oxidation of iron oxides, which remain in their reduced forms by effect of soil saturation in the absence of drainage. In this way, after flooding in the rice season, undrained soil may contain smaller amounts of reducible iron Fe than drained soil and hence lead to earlier soil CH4 emissions.
4.2. Soil N2O fluxes and seasonal emissions
As can be seen from , top-dress N fertilization had no effect on soil N2O fluxes under continuously flooding (). This result is consistent with those of other studies (Bayer et al. Citation2014, Citation2015; Liu et al. Citation2010) and may be ascribed to sequential reduction of N2O to N2 under low Eh soil conditions (Reddy and DeLaune Citation2008) and also to N absorption by rice plants.
Since the formation of CH4 and N2O in soil requires contrasting redox potential conditions, reduced soil CH4 emissions in periods of water suppression are in contrast with increased N2O fluxes under intermittent irrigation ( and ). This is a well-documented trade-off (Zschornack et al. Citation2016; Hou et al. Citation2012; Johnson-Beebout et al. Citation2009; Liu et al. Citation2010; Zou et al. Citation2004). In this study, seasonal soil N2O emissions in intermittent systems in southern Brazil were three times higher than in flooded soils. However, the difference was not significant at Tukey’s 0.05 level owing to the high variability in the data among replicates. In northwestern Japan, seasonal soil N2O influxes were lower than in southern Brazil, partly as a result of the also lower N application rates used in the former area (45 vs. 150 kg ha−1). The low soil N availability (Chapuis-Lardy et al. Citation2007) and the number of days allocated between irrigation periods in Japan proved insufficient to increase Eh (Towprayoon et al. Citation2005) may be related to soil N2O influxes.
4.3. pGWP, rice yield, and YpGWP
The increased pGWP values obtained with CF in both study areas can be ascribed to the major contribution of soil CH4 emissions during the rice growing season (). By contrast, the contribution of N2O emissions to pGWP was very small in southern Brazil (0.6–6.0% pGWP) and negative in northwestern Japan, even under intermittent irrigation. These results suggest that efforts should focus on reducing CH4 emissions during rice growth. Itoh et al. (Citation2011) previously found the contribution of N2O to pGWP at nine sites with different irrigation systems in Japan to be very small (<10%).
The similarity of yields under CF and intermittent irrigation in both study areas reinforce previous results of Hou et al. (Citation2012) and Kim et al. (Citation2014) and suggest that water supply from both systems fulfills the requirements of rice crops. This is especially so in Asia, where some vagueness in timing and span remains but appropriate water management was previously found to result in similar or even higher rice yields (Minamikawa et al. Citation2006). In contrast, these results are very important for Brazil and other LAC countries where CF is the prevalent water management practice. In this region, farmers have been largely reluctant to adopt intermittent irrigation despite its emission mitigation benefits (Mushtaq et al. Citation2009). One of the main challenges of intermittent irrigation is avoiding the yield losses of inappropriate implementation (e.g., if drying is done at critical stages of plant growth such as flowering). Avoiding the yield penalties associated with intermittent irrigation requires better awareness of good water scheduling approaches and also exploring new irrigation techniques with more easily controlled timing of drying. More importantly, promoting the adoption of intermittent irrigation requires developing effective policies to create an enabling environment and infrastructure for water delivery and control by farmers (Chirinda et al. Citation2017).
Yield-scaled pGWP (YpGWP) with intermittent irrigation systems was more than twice lower than those obtained with flooding in both northwestern Japan and southern Brazil (). Therefore, appropriate water management in intermittent irrigation systems can produce more than twice the amount of grain rice with current GHG emissions, or vice versa. Similar results were obtained by Zschornack et al. (Citation2016) in southern Brazil. These authors observed a mean reduction of ca. 60% in YpGWP with intermittent irrigation relative to CF (0.33 vs. 0.81 kg CO2 eq kg−1 rice) over two rice growing seasons.
These results are very important for southern Brazil regions and other rice-growing LAC countries, where rice production can be increased without increasing GHG emissions by adopting intermittent irrigation – a technology Asian farms are enjoying already – on commercial farms.
5. Conclusions
Seasonal soil CH4 emissions under CF in northwestern Japan are slightly higher than they are in southern Brazil as a consequence of the increased contents in organic carbon of Japanese soil and also, probably, of seedling transplantation to a previously flooded field expanding the period under flooding relative to direct seeding in dry soil in southern Brazil. Intermittent irrigation systems in paddy fields are similarly efficient in reducing soil CH4 emissions as CF systems in southern Brazil and northwestern Japan. In both areas, irrigation systems failed to significantly affect seasonal soil N2O emissions or rice grain yield. Intermittent irrigation reduced yield-scaled pGWP by one-half in relation to CF in both study areas. Intermittent irrigation is a key agricultural practice with a view to meeting a future increase in rice production not associated to an increase in GHG emissions in southern Brazil and other countries of LAC, where this technology is still to be widely adopted the farmers.
Acknowledgments
The authors are grateful to the staff of the Niigata Agricultural Research Institute and the National Institute for Agro-Environmental Sciences in Japan, and also in the Riograndense Rice Institute in Brazil, for their help with field and laboratory activities. They also thank the National Council for Scientific and Technological Development (CNPq) and Foundation for Research Support of Rio Grande do Sul State (Fapergs) for funding this work and the Coordination for the Improvement of Personnel with Graduate Education (CAPES) for award of a sandwich doctorate scholarship.
Additional information
Funding
References
- Ali M, Hoque MA, Kim P 2013: Mitigating global warming potentials of methane and nitrous oxide gases from rice paddies under different irrigation regimes. Ambio, 42, 357–368. doi:10.1007/s13280-012-0349-3
- Banker BC, Kludze HK, Alford DP, LeLaune RD, Lindau CW 1995: Methane sources and sinks in paddry rice soils: relationship to emissions. Agric. Ecosyst. Environ., 53, 243–251. doi:10.1016/0167-8809(94)00578-3
- Bayer C, FdS C, Pedroso GM, Zschornack T, Camargo ES, MAd L, Frigheto RTS, Gomes J, Marcolin E, Macedo VRM 2014: Yield-scaled greenhouse gas emissions from flood irrigated rice under long-term conventional tillage and no-till systems in a Humid Subtropical climate. Field Crops Res, 162, 60–69. doi:10.1016/j.fcr.2014.03.015
- Bayer C, Zschornack T, Pedroso GM, Rosa CM, Camargo ES, Boeni M, Marcolin E, Reis CES, Santos DC 2015: A seven-year study on the effects of fall soil tillage on yield-scaled greenhouse gas emission from flood irrigated rice in a humid subtropical climate. Soil Till. Res., 145, 118–125. doi:10.1016/j.still.2014.09.001
- Cai ZC, Xing G, Yan X, Xu H, Tsuruta H, Yagi K, Minami K 1997: Methane and nitrous oxide emissions from paddy fields as affected by nitrogen fertilizers and water management. Plant Soil, 196, 7–14. doi:10.1023/A:1004263405020
- Chapuis-Lardy L, Wrage-Mönnig N, Metay A, Chotte JL, Bernoux M 2007: Soils, a sink for N2O? A review. Glob. Chane. Biol., 13, 1–17. doi:10.1111/j.1365-2486.2006.01280.x
- Chirinda N, Arenas LN, Katto MC, et al. 2017: Sustainable and low greenhouse gas emitting rice production in Latin America and the Caribbean: a dream or a fantasy? Sustainability.
- Climate-data 2014: Clima: Cachoeirinha. https://pt.climate-data.org/region/187/?page=5 e Clima: Nagaoka. https://pt.climate-data.org/location/5520/5
- CONAB 2015: Companhia Nacional De Abastecimento - Acompanhamento Da Safra Brasileira De Grãos. Safra 2014/15- Quinto Levantamento, pp. 1–116. Companhia Nacional de Abastecimento, Brasília (in Portuguese).
- Costa FdS, Bayer C, Lima MA, Frighetto RTS, Macedo VRM, Marcolin E 2008: Variação diária da emissão de metano em solo cultivado com arroz irrigado no Sul do Brasil. Ciência Rural, 38, 2049–2053. doi:10.1590/S0103-84782008000700041
- Counce PA, Keisling TC, Michell AJ 2000: A uniform, objective, and adaptative system for expressing rice development. Crop Sci., 40, 436–443. doi:10.2135/cropsci2000.402436x
- DeAngelo BJ, Chesnaye FC, Beach RH, Sommer A, Murray BC 2006: Methane and nitrous oxide mitigation in agriculture. Energy J, Multi-Greenhouse Gas Mitigation Climate Policy Special Issue, 27, 89–108.
- Denier vand der Gon, Neue HAC, Neue HU 1995: Influence of organic matter incorporation on the methane emission from a wetland rice field. Global Biochem. Cycles, 7, 11–12.
- GRiSP (Global Rice Science Partnership) 2013: Annual progress report 2013: CCIAR Research Program on Rice (GRiSP). http://hdl.handle.net/10947/3085 (March, 2017).
- Hadi A, Inubushi K, Yagi K 2010: Effect of water management on greenhouse gas emissions and microbial properties of paddy soils in Japan and Indonesia. Paddy. Water. Environ., 8, 319–324. doi:10.1007/s10333-010-0210-x
- Hou H, Peng S, Xu J, Yang S, Mao Z 2012: Seasonal variations of CH4 and N2O emissions in response to water management of paddy fields located in Southeast China. Chemosphere, 89, 884–892. doi:10.1016/j.chemosphere.2012.04.066
- IBGE 2014 Statistics of Agricultural Production. (in portuguese). ftp://ftp.ibge.-gov.br/Producao_Agricola/Fasciculo_Indicadores_IBGE/estProdAgr_201404.pdf (1 May, 2017)
- IPCC 2007: Intergovernmental Panel on Climate Change. Climate change 2007-The Physical Science Basis. Contribution of working group I to the fourth assessment report of the Intergovernmental Panel on Climate Change, http://www.ipcc.ch/pdf/assessment-rport/ar4/wg1/ar4_wg1_full_report.pdf (10 July, 2017).
- IRRI 2013: Rice facts. http://irri.org (24 August, 2016).
- Itoh M, Sudo S, Mori S et al. 2011: Mitigation of methane emissions from paddy fields by prolonging midseason drainage. Agric. Ecosyst. Environ, 141, 359–372. doi:10.1016/j.agee.2011.03.019
- Johnson-Beebout SE, Angeles OR, Alberto MCR, Buresh RJ 2009: Simultaneous minimization of nitrous oxide and methane emission from rice paddy soils is improbable due to redox potential changes with depth in a greenhouse experiment without plants. Geoderma, 149, 45–53. doi:10.1016/j.geoderma.2008.11.012
- Kim GY, Gutierrez J, Jeong HC, Lee JS, Haque MDM, Kim P 2014: Effect of intermittent drainage on methane and nitrous oxide emissions under different fertilization in a temperate paddy soil during rice cultivation. J. Korean Soc. Appl. Biol. Chem., 57, 229–236. doi:10.1007/s13765-013-4298-8
- Kumar V, Ladha JK 2011: Direct seeding of rice: recent developments and future research needs. Adv. Agron., 111, 297–413.
- Liu S, Qin Y, Zou J, Liu Q 2010: Effects of water regime during rice-growing season on annual direct N2O emission in a paddy rice–winter wheat rotation system in southeast China. Sci. Total Environ., 408, 906–913. doi:10.1016/j.scitotenv.2009.11.002
- Lu Y, Wassmann R, Neue HU, Changyong H 2000: Dynamics of dissolved organic carbon and methane emissionsin a flooded rice soil. Soil. Sci. Soc. Am. J, 64, 2011–2017. doi:10.2136/sssaj2000.6462011x
- Ma K, Conrad R, Lu Y 2013: Dry/Wet cycles change the activity and population dynamics of methanotrophs in rice field soil. App. Environm. Ecol., 79, 4932–4939.
- Ma K, Lu Y 2011: Regulation of microbial methane production and oxidation by intermittent drainage in rice field soil. FEMS Microbiol. Ecol., 75, 446–456. doi:10.1111/j.1574-6941.2010.01018.x
- Malyan SK, Bhatia A, Kumar A, Gupta DK, Singh R, Kumar SS, Tomer R, Kumar O, Jain N 2016: Methane production, oxidation and mitigation: a mechanistic understanding and comprehensive evaluation of influencing factors. Sci. Total environm. 572, 874–896. doi:10.1016/j.scitotenv.2016.07.182
- Minamikawa K, Sakai N, Yagi K 2006: Methane emission from paddy fields and its migitation options on a field scale. Microbes. Environ., 21, 135–147. doi:10.1264/jsme2.21.135
- Minamikawa K, Yagi K, Tokida T, Sander BO, Wassmann R 2012: Appropriate frequency and time of day to measure methane emissions from an irrigated rice paddy in Japan using the manual closed chamber method. Greenhouse Gas Meas. Manag., 2, 118–128. doi:10.1080/20430779.2012.729988
- Moterle DF, Silva LS, Moro VJ, Bayer C, Zschornack T, Avila LA, Bundt AC 2013: Methane efflux in rice paddy field under different irrigation managements. R. Bras. Ci. Solo, 37, 431–437. doi:10.1590/S0100-06832013000200014
- Mushtaq S, Khan S, Haffez N, Hanjra MA 2009: Does reliability of water resources matter in the adoption of water-saving irrigation practices? A case study in the Zhanghe irrigation system. China. Water Policy, 11, 661–679. doi:10.2166/wp.2009.033
- Nishimura S, Sawamoto T, Akiyama H, Sudo S, Yagi K 2004: Methane and nitrous oxide emission and controls from a paddy field with Japanese conventional water management and fertilizer application. Global Biogeochem. Cycles, 18. doi:10.1029/2003GB002207
- Peel MC, Finlayson BL, McMahon TA 2007: Updated world map of the Köppen-Geiger climate classification. Hydrol. Earth Syst. Sci., 11, 1633–1644. doi:10.5194/hess-11-1633-2007
- Reddy KR, DeLaune RD 2008: Biogeochemistry of Wetlands: science and Applications, CRC Press, Florida.
- SEEG 2014: Análise Da Evolução Das Emissões De GEE No Brasil (1990-2012): setor agropecuário/Instituto De Manejo E Certificação Florestal E Agrícola, pp. 32. Observatório do Clima, São Paulo. (in Portuguese).
- Shiratori Y, Watanabe H, Furukawa Y, Tsuruta H, Inubushi K 2007: Effectiveness of a subsurface drainage system in poorly drained paddy fields on reduction of methane emissions. Soil Sci. Plant Nutr., 53, 387–400. doi:10.1111/j.1747-0765.2007.00171.x
- Silva LS, Griebeler G, Moterle DF, Bayer C, Zschornack T, Pocojeski E 2011: Dinamics of methane emissions in soils under rice cultivation in Southern Brazil. R. Bras. Ci. Solo, 35, 473–481. (in Portuguese with English abstract). doi:10.1590/S0100-06832011000200016
- Sosbai 2014: Arroz Irrigado: recomendações Técnicas Da Pesquisa Para O Sul Do Brasil, pp. 192. Sociedade Sul-Brasileira de Arroz Irrigado, Santa Maria. (in Portuguese).
- Towprayoon S, Smakgahn K, Poonkaew S 2005: Mitigation of methane and nitrous oxide emissions from drained irrigated rice fields. Chemosphere, 59, 1547–1556. doi:10.1016/j.chemosphere.2005.02.009
- Yan X, Du L, Shi S, Xing G 2000: Nitroux oxide emission from wetland rice soil as affected by the application of controlled-availabity fertilizers and mid-season aeration. Biol. Fertil. Soils, 32, 60–66. doi:10.1007/s003740000215
- Yu K, Bohme F, Rinklebe J, Neue HU, DeLaune RD 2007: Major biogeochemical processes in soils - a microcosm incutation from reducing to oxidizing conditions. Soil. Sci. Soc. Am. J, 71, 1406–1417. doi:10.2136/sssaj2006.0155
- Zhang G, Ji Y, Ma J, Xu H, Cai Z, Yagi K 2012: Intermittent irrigation changes production, oxidation, and emission of CH4 in paddy fields determined with stable carbon isotope technique. Soil Biol. Biochem., 52, 108–116. doi:10.1016/j.soilbio.2012.04.017
- Zou J, Huang Y, Jiang J, Zheng X, Sass RL 2005: A 3-year field measurement of methane and nitrous oxide emissions from rice paddies in China: effects of water regime, crop residue, and fertilizer application. Global Biogeochem Cycles, 19, GB, 2021. doi:10.1029/2004GB002401
- Zou J, Huang Y, Zong L, Zheng X, Wang Y 2004: Carbon dioxide, methane, and nitrous oxide emissions from a rice-wheat rotation as affected by crop residue incorporation and temperature. Adv. Atmos Sci., 21, 691–698. doi:10.1007/BF02916366
- Zschornack T, Rosa CM, Pedroso GM, Marcolin E, Silva PRF, Bayer C 2016: Mitigation of yield-scaled greenhouse gas emissions in subtropical paddy rice under alternative irrigation systems. Nutr. Cycl Agroecosyst, 105, 61–73. doi:10.1007/s10705-016-9775-0