ABSTRACT
Central Anatolia, which suffers from salinity, alkalinity, and drought stresses, is one of the most important cultivation regions of barley (Hordeum vulgare) in Turkey. Arbuscular mycorrhizal fungi (AMF) could promote barley production under several stresses; however, only a little information is available for AMF community composition in Turkish arable soils. In this study, barley root samples were collected from eight sites in the Central Anatolian region during the growing season (GS: April) and the harvest season (HS: July) in 2012, and the composition of AMF communities were elucidated based on the partial sequence of the AMF 18S rRNA gene using high-throughput sequencing technology. As a result, barley-AMF symbioses in this region were highly dominated by Glomeraceae (71.8% in GS and 59.2% in HS), followed by Claroideoglomeraceae (10.3% in GS and 15.9% in HS), Gigasporaceae (9.1% in GS and 13.1% in HS), and Acaulosporaceae (5.8% in GS and 7.7% in HS). Compared to Glomeraceae and Claroideoglomeraceae families, communities of Acaulosporaceae, Diversisporaceae, Paraglomeraceae, and Gigasporaceae consisted of fewer AMF species. The AMF evenness significantly increased from GS to HS. The most dominant AMF sequence, VTX00248 in the MaarjAM database, was closely related to Rhizophagus, which occupied 25.8% and 14.7% of the total AMF sequences in GS and HS, respectively. The relative abundance of AMF related to Rhizophagus tended to be reduced in HS, suggesting that the species could form mycorrhiza in the early stages of barley growth in this region. On the other hand, the relative abundance of Claroideoglomeraceae and Scutellosporaceae tended to increase in HS. Soil CaCO3 content significantly influenced AMF community compositions in GS, while soil pH and EC showed no significant impact on AMF community compositions. Based on discriminant analysis, 11 VTXs (related Acaulospora, Claroideoglomus, Funneliformis, Gigaspora, and Glomus) showed higher abundance in the barley roots grown in the soil with relatively high CaCO3 content, suggesting that these sequences might be adapted to such an environment.
1. Introduction
Occupying approximately 19% of the total area of Turkey, Central Anatolia is the largest region of Turkey, where a semi-arid climate with less than 300 mm of annual rainfall causes a long drought period from June to mid-October. The typical grayish and brownish marly soils formed under such climates are often characterized by poor soil development with low organic matter content and water holding capacity (Kürschner and Parolly Citation2012). Although these harsh conditions and human activities such as overgrazing, intensive plowing, and the continuing expansion of arable lands have dramatically increased soil degradation over the years, Central Anatolia still accounts for one-third of the total crop production of Turkey, including hard wheat, barley, lentil, and chickpea (Kürschner and Parolly Citation2012).
Annual barley production in Turkey is approximately 7 million Mg per year (FAOSTAT Citation2019) over 36,000 km2 in central and southeast Anatolia Regions (Dizkirici et al. Citation2008), but recently the land surface reserved for barley agriculture was reported to have been reduced to 24,183 km2 (FAOSTAT Citation2019). This is likely due to lower commodity prices, insufficient subsidies, weaker supply chains, and inadequate seed production programs compared to those available for wheat production. Although barley has a lower production cost and a high adaptation potential to low input environments compared to other cereals, its production is also limited by low water supply and nutritional deficiencies in the highly saline-alkaline soil environment of Central Anatolia.
Increasing scientific and public attention to soil salinization has encouraged the sustainable use of saline-alkaline soil resources through approaches such as breeding salt-tolerant plants, engineering plants using different genes, and leaching excessive salts or desalinizing seawater for irrigation purposes (Evelin, Kapoor, and Giri Citation2009). However, most of these approaches are laborious and costly for developing countries (Cantrell and Linderman Citation2001) and, unfortunately, only a few genetic traits of salt tolerance have been explored so far (Schubert et al. Citation2009). On the other hand, for a few decades, the plants have been known to colonize naturally both by beneficial bacteria and fungi, which can improve plant performance under stress environments and, consequently, increase plant yield (Daei et al. Citation2009; Ruíz-Sánchez et al. Citation2011; Nadeem et al. Citation2014). In light of this fact, a large number of investigations have been carried out recently to elucidate the role of arbuscular mycorrhizal fungi (AMF) in alleviating salinity stress and the outcomes of these works were well summarized by several authors (Evelin, Kapoor, and Giri Citation2009; Porcel, Aroca, and Ruiz-Lozano Citation2012; Hameed et al. Citation2014).
Generally, the AMF-induced plant nutrient balance can enhance salinity and drought tolerance of crops in semiarid regions. This mechanism can be explained by better plant growth with a more intense development of mycorrhizal hyphae leading to more efficient water transport to the plant under drought conditions (Nelsen and Safir Citation1982). Khalvati et al. (Citation2005) also showed that mycorrhizal inoculation to barley increased water uptake by 4% compared to non-mycorrhizal treatment under drought conditions. In their study on the effects of mycorrhiza and phosphorus fertilization on growth and nutrient uptake of barley under changing salt levels, Mohammad, Malkawi, and Shibli (Citation2003) indicated that AMF inoculation increased plant growth, iron and zinc uptake, and the K/Na ratio as a key feature of plant salt tolerance.
While some studies have shown negative impacts of AMF infection on barley growth (Smith and Read Citation2008; Grace et al. Citation2009), positive effects were also found in the specific environments (Khalvati et al. Citation2010; Li et al. Citation2014). Very recently, Sendek et al. (Citation2019) reported antagonistic interactions between barley and AMF under drought conditions at higher AMF richness pointing to unexpected alterations of plant-soil biotic interactions and complex interactions between the genotypic richness of barley and the performance of the associated AMF community.
In the present study, we investigated AMF diversity across barley in Central Anatolian soils suffering from stress factors, e.g., salinity, alkalinity, and drought. To the best of our knowledge, in Turkey, only Suzuki et al. (Citation2014) has studied AMF phylotype compositions based on the nuclear ribosomal large subunit RNA genes in plant samples from the East Black Sea, Mediterranean, and Central Anatolian regions and showed that AMF communities in the Mediterranean region tended to be more diverse than those in the Central Anatolian region. No further information regarding the molecular ecology of AMF in Turkish arable soils has been made available.
Therefore, in the present work, using high-throughput sequencing technology, we aimed to investigate the diversity of AMF associated winter barley (Hordeum vulgare L.) and their seasonal changes and relationships with soil properties in the saline-alkaline soils of Central Anatolia.
2. Materials and methods
2.1. Study site, sampling, and soil analysis
The root samples of barley and corresponding soil samples were collected from eight locations in Central Anatolia. To investigate the seasonal impacts, root and soil sampling was conducted in the growing season (GS: 15 April 2012) and during the harvest season (HS: 6 July 2012) for each sample. The location of study sites and soil textures are shown in . Soil pH and EC of soil were measured in a 1:2.5 soil/water mixture (Richards Citation1954); CaCO3 contents by using a Scheibler calcimeter (Soil Survey Staff Citation1993); particle size distribution according to Bouyoucos (Citation1951) and organic matter contents of the soils were determined by a modified Walkley–Black Method (Jackson Citation1962). Root samples were cleaned and washed in an ultrasonic bath. Fine roots were ground into small fragments using liquid nitrogen. Root samples were kept in a freezer at −20°C before DNA extraction.
Table 1. Geographical information of study sites.
2.2. Molecular analysis
Total DNA was extracted from 0.1 g of root fragments using a GenElute plant genomic DNA miniprep kit (Sigma-Aldrich, MO, USA) according to the manufacturer’s instructions. The AMF partial 18S rRNA gene was amplified using AMF specific primer pairs AMV4.5NF and AMDGR (Sato et al. Citation2005). The primer sequences included overhang adaptor sequences for the Nextera XT index primer (Illumina Inc., CA, USA). PCR reactions were conducted in 25 µl mixtures consisting of 12.5 µl KOD-FX NEO (TOYOBO, Osaka, Japan), 0.2 µl of each primer (10 µM), 1 µl of the template, which is 10 times diluted extracted DNA. The reaction conditions were as follows: initial denaturation at 95°C for 5 min; 35 cycles of 95°C for 45 s, 58°C for 45 s, and 72°C for 1 min; and a final elongation step at 72°C for 7 min. PCR amplicons were purified using Agencourt AMPure XP (Beckman Coulter, CA, USA) according to manufacturer’s instructions and quantified through QuantiFluor (Promega, WI, USA). Index PCR was performed to attach dual index and the Illumina sequencing adaptors. The reaction conditions were as follows: initial denaturation at 95°C for 3 min; 8 cycles of 95°C for 30 s, 55°C for 30 s, and 72°C for 30 s; and a final elongation step at 72°C for 5 min. The amplicons were purified using Agencourt AMPure XP. Purified amplicons were pooled in equimolar and paired-end sequenced on an Illumina MiSeq platform at a read length of 2 × 300 bp using the MiSeq reagent kit v3.
2.3. Bioinformatics and statistical analysis
The primary analysis of the raw FASTQ data was processed with the QIIME2 pipeline (version 2018.8; http://qiime2.org/) (Caporaso et al. Citation2010). Error correction, quality filtering, chimera removal, and sequence variant calling of the Illumina amplicon sequences were performed using the DADA2 algorithm (Callahan et al. Citation2016). The forward and reverse reads were truncated at 220 and 200 bp, respectively, corresponding to a quality score of >20. After joining paired-end reads, singletons and doubletons were removed. For taxa comparisons, we used the QIIME2 q2-feature-classifier plugin and the Naïve Bayes classifier that was trained on the MaarjAM reference sequences (Öpik et al. Citation2010). The sequences assigned to the AMF family were defined as AMF sequences and the others were removed from further analysis.
AMF sequences obtained from three replications were combined into one sample by taking the ceiling of the mean of those frequencies. Combined sequences were rarefied at 15,000 sequences per sample for downstream analysis. Alpha diversities of AMF communities were calculated for the Shannon’s diversity index, Faith’s phylogenetic diversity, and Pielou’s evenness. Phylogenetic relationships of the obtained AMF virtual taxa (VTX) in the MaarjAM database were illustrated in phylogenetic trees using MEGA X (Kumar et al. Citation2018). Principal coordinate analysis (PCoA) was used to visualize dissimilarities within the AMF beta diversities based on the weighted unifrac distance among the samples according to the phylogenetic tree. Relationships of environmental factors (soil pH, EC, organic matter content, and CaCO3 content) to the AMF beta diversities were fitted onto the ordination using envfit function of R vegan packages (Oksanen et al. Citation2019).
3. Results
3.1. AMF alpha diversities and community compositions
After quality filtering, the remaining 423,037 sequences were clustered into 696 unique sequences and assigned to 47 MaarjAM VTXs. AMF alpha diversities between GS and HS are shown in . The Richness, Shannon’s diversity index, and Faith’s phylogenetic diversity showed no significant difference between two seasons. On the other hand, Pielou’s evenness was significantly increased in HS.
Table 2. AMF alpha diversity. The asterisk labeled implies the significant difference between growing season (GS) and harvest season (HS) (* p < 0.05 by Student t-test). Average ± standard deviation (n = 8).
AMF community compositions between growing and harvest seasons at the family level are shown in . AMF communities were dominated by Glomeraceae (71.8% in GS and 59.2% in HS), followed by Claroideoglomeraceae (10.3% in GS and 15.9% in HS), Gigasporaceae (9.1% in GS and 13.1% in HS), and Acaulosporaceae (5.8% in GS and 7.7% in HS). The relative abundances of Paraglomeraceae and Diversisporaceae were relatively small. In HS, the relative abundance of Glomeraceae tended to become lower, and Claroideoglomeraceae and Scutellosporaceae tended to increase their relative abundance.
Figure 1. Comparison of root AMF community compositions of barley between the growing season (GS) and the harvest season (HS). Average of AMF community compositions among eight study sites are shown at the family level.
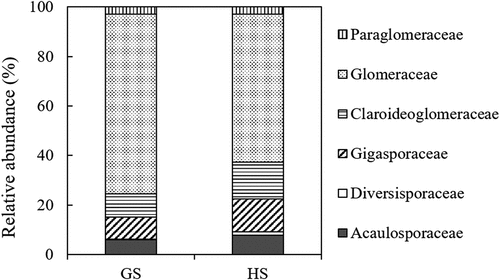
The phylogenetic relationship of detected AMF VTXs is shown in . Compared to Glomeraceae and Claroideoglomeraceae families, communities of Acaulosporaceae, Diversisporaceae, Paraglomeraceae, and Gigasporaceae consisted of fewer AMF VTXs. The most dominant AMF VTX was VTX00248 related to Rhizophagus (25.8% in GS and 14.7% in HS), followed by VTX00024 related to Acaulospora (8.7% in GS and 10.9% in HS) and VTX00056 related to Claroideoglomus (8.8% in GS and 3.7% in HS). The relative abundance of AMF VTXs related to Rhizophagus (VTX00092, VTX00108, VTX00100, VTX00113, VTX00114, VTX00248, and VTX00363) tended to be reduced in HS.
Figure 2. Phylogenetic relationships of obtained AMF virtual taxa (VTX) in the MaarjAM database. The evolutionary history was inferred using the Neighbor-Joining method. The bootstrap values of >70% are shown next to the branches. The evolutionary distances were computed using the Kimura two-parameter method. Average relative abundances of VTX in barley growing and harvest seasons are shown in parentheses (unit: %).
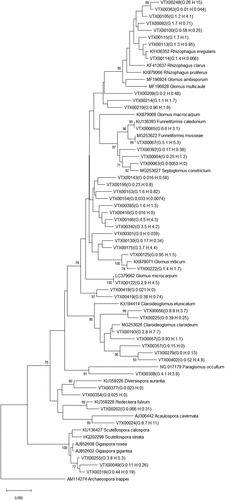
3.2. AMF beta diversity
Beta diversity of AMF communities among study sites and their relationship to the soil properties in GS and HS () are analyzed by PCoA based on weighted unifrac phylogenetic distance metrics. In GS, soil CaCO3 concentration significantly correlated to the ordination of AMF community differences ()), while the correlation was not detected in HS ()).
Table 3. Soil properties of the study sites.
Figure 3. Beta diversity of AMF communities among eight study sites and their relationships to soil chemical properties in barley growing (a) and harvest (b) season. Beta diversity was analyzed by Principal coordinate analysis (PCoA) based on weighted unifrac phylogenetic distance metrics and displayed in the scatter diagram of the first two axes. Solid arrow implies the significant relationship (p < 0.05).
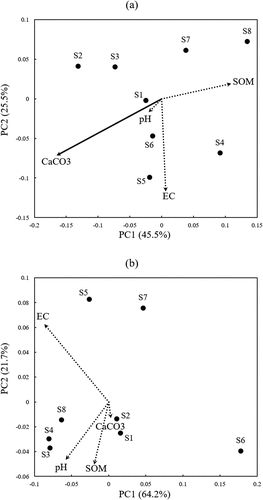
The correlations between the pairwise differences of soil CaCO3 content and weighted unifrac distances are shown in . Differences in soil CaCO3 content were significantly correlated to differences in AMF phylogenetic community composition in GS ()). On the other hand, this correlation was not shown in HS ()), suggesting that soil CaCO3 could influence AMF community compositions at the early stages of barley growth.
Figure 4. Relationships between AMF beta diversity and differences in soil CaCO3 content (%) of each two study sites in barley growing (a) and harvest (b) season. **Dotted line implies the significant correlation (Pearson’s correlation coefficient) at p < 0.01.
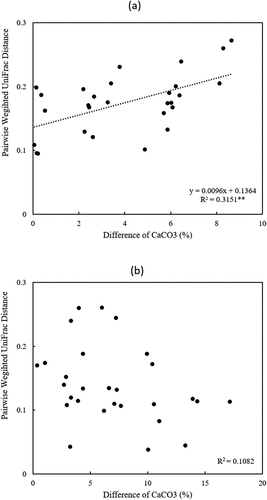
To explore the soil CaCO3 sensitive AMF VTXs, AMF community compositions of GS were separated into two groups according to soil CaCO3 content as follows: high-CaCO3 soils which had a >15% CaCO3 content and low-CaCO3 soils which had a <15% CaCO3 content. Based on the discriminant analysis (LDA score >2.0), VTX00024 (related Acaulospora), VTX00225 (related Claroideoglomus), VTX00064 and VTX00065 (related Funneliformis), VTX00049 (related Gigaspora), VTX00122, VTX00130, VTX00156, VTX00175, VTX00209, and VTX00342 (related Glomus) showed higher abundance in the high-CaCO3 soils. VTX00248 (related to Rhizophagus) showed higher abundance in the low-CaCO3 soils.
4. Discussion
We conducted our study to reveal the characteristics of AMF diversities associated with winter barley and their relationship with soil properties in saline-alkaline soils of Central Anatolia. The six AMF families (Glomeraceae, Claroideoglomeraceae, Acaulosporaceae, Diversisporaceae, Paraglomeraceae, and Gigasporaceae) were detected and the most dominant AMF family was Glomeraceae (). The dominance of Glomeraceae is a common feature in various environments (Öpik et al. Citation2010). With more detailed classification, the most dominant AMF VTX was VTX00248 related to Rhizophagus, followed by VTX00024 related to Acaulospora and VTX00056 related to Claroideoglomus. The genus Rhizophagus including Rhizophagus intraradices is often found in various environments such as moist conditions, sulfuric acid soils, forests, and grasslands (Öpik et al. Citation2006) as well as in semi-arid areas (Zarei et al. Citation2010). VTX00248 was also detected in the semi-arid areas of Madagascar and Uganda (Yamato, Ikeda, and Iwase Citation2009). VTX00024 related to Acaulospora was also detected from roots of woody vegetation in a neotropical mountain forest in South America (Haug et al. Citation2004), and VTX00056 related to Claroideoglomus was detected in a subtropical dry broadleaf forest in North America (Douhan et al. Citation2005). These VTXs might have tolerance to various environments including semi-arid saline-alkaline conditions. With regard to seasonality, the AMF evenness increased from GS to HS (). Similar to this trend, Bainard et al. (Citation2014), who elucidated the AMF communities using a high-throughput sequencer in a semi-arid prairie agroecosystem in North America, reported that the richness and diversity of root AMF communities of three crops increased from June to July, although the AMF communities were quite different from those in our study (Paraglomeraceae was the most dominant in their study). These suggest that AMF community compositions in early stages of the host plants were immature and biased compared to the potential diversities. The relative abundance of AMF VTXs related to Rhizophagus (VTX00092, VTX00105, VTX00108, VTX00100, VTX00113, VTX00114, VTX00248, and VTX00363) and VTX00056 related to Claroideoglomus tended to be reduced in HS. These VTXs could adapt to the environment and could form mycorrhiza in the early stages of barley growth in this region.
In the region of this study, the rainfall decreased from spring to summer which may induce changes in the soil chemical properties in some sites. The results of PCoA showed the relationship between AMF community compositions and soil environmental factors. AMF community compositions obtained from S3, S4, S5, and S8 were relatively similar in direction of PC1 in HS compared to those in GS ()). Although S3, S4, S5, and S8 had the common features that the soil EC increased from GS to HS, and the values were relatively high in HS, there was no significant correlation between AMF community compositions and soil EC (). On the other hand, it was reported that soil EC affects AMF community composition (Bainard et al. Citation2014). The reason why soil EC did not affect the AMF community composition in this study may be that the selection for AMF community by soil EC has already been completed due to long-term continuous cropping.
The soil CaCO3 content was correlated to the variation in AMF community compositions ()) and AMF beta diversities ()) in GS. The reason why the significant difference in the effects of CaCO3 was found only in GS and not in HS is still unclear. As a result of discriminant analysis with a soil CaCO3 concentration of 15% as a threshold, 11 VTX with various classifications showed significantly higher abundance in the high-CaCO3 soils, and the abundance of VTX00248 was higher in low-CaCO3 soil. VTX00209 and VTX00065 were included 11 VTXs in the high-CaCO3 soils, also detected in Scottish grassland (Vandenkoornhuyse et al. Citation2002) and the Panama rainforest region (Husband et al. Citation2002), respectively. These may be VTXs that can not only exist in various environments as generalists but also act effectively alongside the growth of barley in this region, especially in high CaCO3 conditions.
5. Conclusion
The results of this study suggest that the barley-AMF symbiosis in Central Anatolia is highly dominated by Glomeraceae (especially VTX00248, closely related to Rhizophagus) in GS. On the other hand, in the HS, other AMF also formed symbiotic relationships and increased AMF diversities. In addition, 11 VTXs (related Acaulospora, Claroideoglomus, Funneliformis, Gigaspora and Glomus) significantly increased their abundance in relatively high CaCO3 conditions. These findings contribute to our understanding of the ecology of AMF communities associated with barley under the semi-arid stressed condition.
Disclosure statement
No potential conflict of interest was reported by the authors.
Additional information
Funding
References
- Bainard, L. D., J. D. Bainard, C. Hamel, and Y. Gan. 2014. “Spatial and Temporal Structuring of Arbuscular Mycorrhizal Communities Is Differentially Influenced by Abiotic Factors and Host Crop in a Semi-arid Prairie Agroecosystem.” FEMS Microbiology Ecology 88 (2): 333–344. doi:10.1111/fem.2014.88.issue-2.
- Bouyoucos, G. J. 1951. “A Calibration of the Hydrometer for Making Mechanical Analysis of Soils.” Agronomy Journal 43: 434–438. doi:10.2134/agronj1951.00021962004300090005x.
- Callahan, B. J., P. J. McMurdie, M. J. Rosen, A. W. Han, A. J. Johnson, and S. P. Holmes. 2016. “DADA2: High Resolution Sample Inference from Illumina Amplicon Data.” Nature Methods 13 (7): 581–583. doi:10.1038/nmeth.3869.
- Cantrell, I. C., and R. G. Linderman. 2001. “Preinoculation of Lettuce and Onion with VA Mycorrhizal Fungi Reduces Deleterious Effects of Soil Salinity.” Plant and Soil 233 (2): 269–281. doi:10.1023/A:1010564013601.
- Caporaso, J. G., J. Kuczynski, J. Stombaugh, K. Bittinger, F. D. Bushman, E. K. Costello, N. Fierer, et al. 2010. “QIIME Allows Analysis of High Throughput Community Sequencing Data.” Nature Methods 7 (5): 335–336. doi:10.1038/nmeth.f.303.
- Daei, G., R. Ardekani, F. Rejali, S. Teimuri, and M. Miransari. 2009. “Alleviation of Salinity Stress on Wheat Yield, Yield Components, and Nutrient Uptake Using Arbuscular Mycorrhizal Fungi under Field Conditions.” Journal of Plant Physiology 166: 617–625. doi:10.1016/j.jplph.2008.09.013.
- Dizkirici, A., H. E. Guren, S. Onde, F. Temel, T. Akar, H. Budak, and Z. Kaya. 2008. “Microsatellite (SSR) Variation in Barley Germplasm and Its Potential Use for Marker Assisted Selection in Scald Resistance Breeding.” International Journal of Integrative Biology 4 (1): 9–15.
- Douhan, G. W., C. Petersen, C. S. Bledsoe, and D. M. Rizzo. 2005. “Contrasting Root Associated Fungi of Three Common Oak-woodland Plant Species Based on Molecular Identification: Host Specificity or Non-specific Amplification?” Mycorrhiza 15 (5): 365–372. doi:10.1007/s00572-004-0341-2.
- Evelin, H., R. Kapoor, and B. Giri. 2009. “Arbuscular Endophytic Fungi in Alleviation of Salt Stress: A Review.” Annals of Botany 104 (7): 1263–1280. doi:10.1093/aob/mcp251.
- FAOSTAT. 2019. “FAO Statistics.” Food and Agriculture Organization of the United Nations. Accessed 14 September 2019. http://www.fao.org/faostat/en/#home
- Grace, E. J., O. Cotsaftis, M. Tester, F. A. Smith, and S. E. Smith. 2009. “Arbuscular Mycorrhizal Inhibition of Growth in Barley Cannot Be Attributed to Extent of Colonization, Fungal Phosphorus Uptake or Effects on Expression of Plant Phosphate Transporter Genes.” New Phytologist 181 (4): 938–949. doi:10.1111/j.1469-8137.2008.02720.x.
- Hameed, A., E. Dilfuza, E. F. Abd-Allah, A. Hashem, A. Kumar, and P. Ahmad. 2014. “Salinity Stress and Arbuscular Mycorrhizal Symbiosis in Plants.” In Use of Microbes for the Alleviation of Soil Stresses, Volume 1, edited by M. Miransari, 149–172. New York, NY: Springer.
- Haug, I., J. Lempe, J. Homeier, M. Weiß, S. Setaro, F. Oberwinkler, and I. Kottke. 2004. “Graffenrieda Emarginata (Melastomataceae) Forms Mycorrhizas with Glomeromycota and with a Member of the Hymenoscyphus Ericae Aggregate in the Organic Soil of a Neotropical Mountain Rain Forest.” Canadian Journal of Botany 82 (3): 340–356. doi:10.1139/b03-153.
- Husband, R., E. A. Herre, S. L. Turner, R. Gallery, and J. P. W. Young. 2002. “Molecular Diversity of Arbuscular Mycorrhizal Fungi and Patterns of Host Association over Time and Space in a Tropical Forest.” Molecular Ecology 11 (12): 2669–2678. doi:10.1046/j.1365-294X.2002.01647.x.
- Jackson, M. L. 1962. Soil Chemical Analysis. Englewood Cliffs: Prentice-Hall.
- Khalvati, M., B. Bartha, A. Dupingny, and P. Schröder. 2010. “Arbuscular Mycorrhizal Association Is Beneficial for Growth and Detoxification of Xenobiotics of Barley under Drought Stress.” Journal of Soils and Sediments 10 (4): 54–64. doi:10.1007/s11368-009-0119-4.
- Khalvati, M. A., Y. Hu, A. Mozafar, and U. Schmidhalter. 2005. “Quantification of Water Uptake by Arbuscular Mycorrhizal Hyphae and Its Significance for Leaf Growth, Water Relations, and Gas Exchange of Barley Subjected to Drought Stress.” Plant Biology 7 (6): 706–712. doi:10.1055/s-2005-872893.
- Kumar, S., G. Stecher, M. Li, C. Knyaz, and K. Tamura. 2018. “MEGA X: Molecular Evolutionary Genetics Analysis across Computing Platforms.” Molecular Biology and Evolution 35 (6): 1547–1549. doi:10.1093/molbev/msy096.
- Kürschner, H., and G. Parolly. 2012. “The Central Anatolian Steppe.” In Eurasian Steppes, Ecological Problems and Livelihoods in a Changing World, edited by M. J. A. Werger and M. A. van Staalduinen, 149–172. Dordrecht: Springer.
- Li, T., G. Lin, X. Zhang, Y. Chen, S. Zhang, and B. Chen. 2014. “Relative Importance of an Arbuscular Mycorrhizal Fungus (Rhizophagus Intraradices) and Root Hairs in Plant Drought Tolerance.” Mycorrhiza 24: 595–602. doi:10.1007/s00572-014-0578-3.
- Mohammad, M. J., H. I. Malkawi, and R. Shibli. 2003. “Effects of Arbuscular Mycorrhizal Fungi and Phosphorus Fertilization on Growth and Nutrient Uptake of Barley Grown on Soils with Different Levels of Salts.” Journal of Plant Nutrition 26 (1): 125–137. doi:10.1081/PLN-120016500.
- Nadeem, S. M., M. Ahmad, Z. A. Zahir, A. Javaid, and M. Ashraf. 2014. “The Role of Mycorrhizae and Plant Growth Promoting Rhizobacteria (PGPR) in Improving Crop Productivity under Stressful Environments.” Biotechnology Advances 32: 429–448. doi:10.1016/j.biotechadv.2013.12.005.
- Nelsen, C. E., and G. R. Safir. 1982. “Increased Drought Tolerance of Mycorrhizal Onion Plants Caused by Improved Phosphorus Nutrition.” Planta 154 (5): 407–413. doi:10.1007/BF01267807.
- Oksanen, J., F. G. Blanchet, M. Friendly, R. Kindt, P. Legendre, D. McGlinn, P. R. Minchin, et al. 2019. “Vegan: Community Ecology Package. R Package Version 2.5-4.” Accessed 5 July 2019. https://CRAN.R-project.org/package=vegan
- Öpik, M., M. Moora, J. Liira, and M. Zobel. 2006. “Composition of Root Colonizing Arbuscular Mycorrhizal Fungal Communities in Different Ecosystems around the Globe.” Journal of Ecology 94 (4): 778–790. doi:10.1111/jec.2006.94.issue-4.
- Öpik, M., A. Vanatoa, E. Vanatoa, M. Moora, J. Davison, J. M. Kalwij, Ü. Reier, and M. Zobel. 2010. “The Online Database MaarjAM Reveals Global and Ecosystemic Distribution Patterns in Arbuscµlar Mycorrhizal Fungi (Glomeromycota).” New Phytologist 188 (1): 223–241. doi:10.1111/j.1469-8137.2010.03334.x.
- Porcel, R., R. Aroca, and J. M. Ruiz-Lozano. 2012. “Salinity Stress Alleviation Using Arbuscular Mycorrhizal Fungi. A Review.” Agronomy for Sustainable Development 32 (1): 181–200. doi:10.1007/s13593-011-0029-x.
- Richards, L. A. 1954. Diagnosis and Improvement of Saline and Alkali Soils. USDA Handbook 60. Washington, D.C.: US Government Printing Office.
- Ruíz-Sánchez, M., E. Armada, Y. Munoz, I. E. G. de Salamone, R. Aroca, J. M. Ruíz-Lozano, and R. Azcón. 2011. “Azospirillum and Arbuscular Mycorrhizal Colonization Enhance Rice Growth and Physiological Traits under Well-watered and Drought Conditions.” Journal of Plant Physiology 168: 1031–1037. doi:10.1016/j.jplph.2010.12.019.
- Sato, K., Y. Suyama, M. Saito, and K. Sugawara. 2005. “A New Primer for Discrimination of Arbuscµlar Mycorrhizal Fungi with Polymerase Chain Reaction-denature Gradient Gel Electrophoresis.” Grassland Science 51 (2): 179–181. doi:10.1111/j.1744-697X.2005.00023.x.
- Schubert, S., A. Neubert, A. Schierholt, A. Sümer, and C. Zörb. 2009. “Development of Salt-resistant Maize Hybrids: The Combination of Physiological Strategies Using Conventional Breeding Methods.” Plant Science 177 (3): 196–202. doi:10.1016/j.plantsci.2009.05.011.
- Sendek, A., C. Karakoç, C. Wagg, J. Domínguez-Begines, G. M. Do Couto, M. G. A. van der Heijdenand, A. A. Naz, et al. 2019. “Drought Modulates Interactions between Arbuscular Mycorrhizal Fungal Diversity and Barley Genotype Diversity.” Scientific Reports 9650 (9): 1–15.
- Smith, S. E., and D. J. Read. 2008. Mycorrhizal Symbiosis. 3rd ed. Cambridge: Academic Press.
- Soil Survey Staff. 1993. Soil Survey Manual. USDA Handbook. No. 18. Washington, D.C.: US Government Printing Office.
- Suzuki, K., O. C. Turgay, M. O. Akca, N. Harada, and M. Nonaka. 2014. “Molecular Diversity of Indigenous Arbuscular Mycorrhizal Fungi in Three Different Agricultural Regions of Turkey.” Soil Science and Plant Nutrition 60 (3): 367–376. doi:10.1080/00380768.2014.890916.
- Vandenkoornhuyse, P., R. Husband, T. J. Daniell, I. J. Watson, J. M. Duck, A. H. Fitter, and J. P. W. Young. 2002. “Arbuscular Mycorrhizal Community Composition Associated with Two Plant Species in a Grassland Ecosystem.” Molecular Ecology 11 (8): 1555–1564. doi:10.1046/j.1365-294X.2002.01538.x.
- Yamato, M., S. Ikeda, and K. Iwase. 2009. “Community of Arbuscular Mycorrhizal Fungi in Drought-resistant Plants, Moringa Spp., In Semiarid Regions in Madagascar and Uganda.” Mycoscience 50 (2): 100–105. doi:10.1007/S10267-008-0459-8.
- Zarei, M., S. Hempel, T. Wubet, T. Schäfer, G. Savaghebi, G. S. Jouzani, M. K. Nekouei, and F. Buscot. 2010. “Molecular Diversity of Arbuscular Mycorrhizal Fungi in Relation to Soil Chemical Properties and Heavy Metal Contamination.” Environmental Pollution 158 (8): 2757–2765. doi:10.1016/j.envpol.2010.04.017.