ABSTRACT
Drug checking services provide individuals who use drugs with the ability to test samples of their drugs for the presence of highly potent substances. However, there has been recent concern about whether the existing repertoire of point-of-care drug checking technologies, such as immunoassay strips and Fourier-transform infrared spectroscopy (FTIR), are adequate in identifying substances in the unregulated drug supply. Carfentanil and nitazene opioids, substances that are even more potent than fentanyl in vitro, have been found in the unregulated supply in North America and pose a challenge to our existing drug checking strategy. For example, etizolam has recently permeated the unregulated drug supply in North America, and has demonstrated the ability to evade point-of-care drug checking technologies. In response to the incessantly changing nature of the unregulated supply, we argue that drug checking technologies and service delivery models must continuously adapt alongside constantly changing drug markets. We provide two examples of emerging technologies, paper spray-mass spectrometry and surface-enhanced Raman spectroscopy, which address many of the shortcomings of existing technologies. For both technologies, we discuss their feasibility, where they can be offered, their advantages, and how they address gaps in our existing technologies. We contend that these technologies, and other emerging technologies, can be integrated into a future approach to drug checking that flexibly uses different technologies and service delivery methods to adapt to changes in the drug supply.
Introduction
Addressing overdose mortality is an increasingly urgent challenge in regions across North America. The alarming prevalence of unregulated drug toxicity has been largely attributed to the presence of variable and highly potent substances, such as fentanyl and fentanyl analogues, in the unregulated drug supply (Citation1). Between 1999 and 2021, according to the Stanford-Lancet Commission on the North American Opioid Crisis, there were an estimated 600,000 overdose-related deaths in North America (Citation2). Although harm reduction interventions, such as naloxone programs, supervised injection programs, and opioid agonist treatment have been used to mitigate overdose mortality, these measures are only effective if they are accessed. In order to address the upward trajectory of overdose mortality and associated harms, and to reach individuals who cannot access other interventions, there has been a recent surge in interest in the utility of drug checking services as a tool for combatting this epidemic (Citation1).
Although recent research has evaluated the effectiveness of existing drug checking technologies and services, there have been growing concerns as to the extent to which these technologies can be used flexibly, to account for changes in the composition of the unregulated drug supply. Here, we sought to characterize the rising prevalence of novel psychoactive substances, discuss the inadequacies of a static repertoire of drug checking technologies, and propose a future direction in which drug checking technologies and delivery models adapt to trends in unregulated drug markets.
The incessantly changing drug supply and the inadequacy of a static technology repertoire
Recent changes in the unregulated drug supply have seen the increasing presence of fentanyl analogues (e.g., carfentanil) and nitazene opioids, several of which, including etonitazene, isotonitazene, and metonitazene, have demonstrated higher in vitro efficacy and potency than fentanyl (Citation3). Carfentanil is a fentanyl analog that was detected across North America (Citation4–7), and represents a growing threat to the safety of people who use drugs. Originally synthesized to be used as a general anesthetic for animals, carfentanil first infiltrated the unregulated supply in 2016 in the Midwest and Appalachia (Citation8). Over a two-month period between August and September of 2016, carfentanil-related overdoses claimed an estimated 300 lives in these two regions (Citation8). Carfentanil has since been found in the unregulated supply in other parts of North America, including in British Columbia (BC), where, according to the BC Coroners Service, the substance was detected in 152 overdose deaths between January and October of 2021, up 86 deaths from 2020 (Citation9). Because carfentanil is typically present in very low concentrations, it can go undetected by existing drug checking technologies (Citation10). However, given the high potency of carfentanil, even these undetectably low concentrations can cause toxicity and overdose mortality (Citation10).
In addition to carfentanil, nitazene opioids have also begun permeating the unregulated supply in recent months. Specifically, etonitazene and isotonitazene have recently been identified in the unregulated drug supply in Toronto (Citation11) and Montreal (Citation12). More recently, metonitazene has been detected in the unregulated supply in Knox County, Tennessee, where overdose mortality increased significantly from 2020 to 2021 (Citation13). Etonitazene and isotonitazene cannot be detected by fentanyl immunoassay strips, which are inexpensive and provide a simple positive or negative detection, but screen only for fentanyl and fentanyl analogues. In addition to immunoassay strips, Fourier-transform infrared spectroscopy (FTIR) is a widely used point-of-care drug checking technology that uses the differential absorption of infrared radiation to create unique spectra, which are then used to distinguish substances (Citation14). However, these emerging psychoactive substances may also evade detection by FTIR when present in low concentrations, a growing concern for drug checking services (Citation15). While less is known about the pharmacodynamics of nitazene opioids, the emergence of such adulterants that can evade detection by conventional drug checking technologies underscores the necessity of a diverse and adaptive repertoire of these tools.
Of recent concern, etizolam, a thienodiazepine with similar properties as benzodiazepines, has also been increasingly detected as an adulterant in the unregulated North American opioid supply (Citation16). Researchers have been particularly concerned by a recent rise in overdose deaths related to the co-ingestion of opioids, etizolam and other benzodiazepines (Citation16), a combination that can exacerbate respiratory depression and sedation, increasing the risk of overdose (Citation16). A recent study compared the accuracy of point-of-care technologies (benzodiazepine immunoassay strips and FTIR) in detecting benzodiazepines with laboratory-based technologies and found that these technologies had a combined false negativity rate of 29.2% and a false positivity rate of 17.8% (Citation16). Attempts to mitigate overdose mortality are further complicated by the fact that etizolam is not technically a benzodiazepine but a member of a related class of drugs, making it more difficult to identify using benzodiazepine strips and requiring a higher concentration of the substance to detect (Citation17).
The need for a broader array of drug checking technologies and implementation approaches
There is a growing interest in new point-of-care drug checking technologies under development. Recently, several promising new technologies that have the potential to greatly improve the existing repertoire of drug checking methods have been proposed (Citation18–31). For example, Vandergrift et al. described the potential use of paper spray-mass spectrometry (PS-MS) as an easy-to-use and inexpensive drug checking technology (Citation19). PS-MS utilizes paper spray ionization, an ionization method that circumvents the need for extensive sample preparation by making it possible to rapidly analyze a sample in open air (Citation20), making PS-MS a promising tool in point-of-care settings where rapid results are favored (Citation21). In PS-MS, less than 10 μL of a sample is loaded onto a triangular strip of paper to which solvent is added prior to exposing the paper strip to voltage (Citation19), which results in the creation of an electric field (Citation21) and ultimately results in dry ions that can be sorted by the mass spectrometer (Citation22). The mass spectrometer sorts ions by their mass-to-charge ratio and uses an ion detector to create mass spectra, which are then used to determine the composition of a sample (Citation22).
In their assessment of the technique, Vandergrift et al. found that PS-MS could detect low levels of fentanyl and norfentanyl in methanol, urine, and a mixture of other analgesic substances (Citation19). McCrae et al. (Citation23) investigated the limits of existing point-of-care technologies and found that immunoassay strips and FTIR were unable to detect fentanyl at concentrations at or below 5% and 10% (w/w%), respectively, levels that exceed the concentrations at which fentanyl analogues and other psychoactive substances can cause overdoses (Citation24). By contrast, in an assessment of the limits of detection of PS-MS in analyzing fentanyl analog, the technology was able to detect fentanyl analogs at concentrations ranging between 3.6 and 7.4 ng/g, a range lower than the levels that can precipitate overdoses (Citation25).
The feasibility of PS-MS was explored by Borden et al. (Citation26) in their two-day pilot study conducted in Vancouver, Canada, in which they found that PS-MS offered the ability to test 133 samples in a highly sensitive and rapid manner. PS-MS fills a gap in the existing landscape of drug checking technologies because, unlike fentanyl immunoassay strips, which can only detect fentanyl and its analogues, and FTIR, which is limited to a library of spectra that is limited in its ability to detect complex and novel compounds (Citation27), PS-MS is able to detect a wide variety of substances, including novel adulterants (Citation27).
Because mass spectroscopy provides information about the isotopic proportions of different elements and molecular mass, it can be used to identify the structure of a sample and detect substances outside of preexisting reference libraries (Citation14). In the Vancouver pilot study, for example, PS-MS was able to detect an unexpected adulterant, like etizolam, in all but five of the substances expected to contain fentanyl, demonstrating the importance of using technologies that are able to detect novel substances (Citation26). Vandergrift et al. (Citation27) contended that, in order to be accessible to people who use drugs, drug checking technologies must be able to use small samples and provide rapid results. PS-MS meets both criteria, as it only requires ~1 mg, and it can provide highly accurate results within 5 min (Citation26). Because PS-MS is portable and can yield results within minutes, it demonstrates promise as a technology that can be introduced to community settings, such as supervised consumption sites. PS-MS is able to detect psychoactive substances outside of a pre-set library of spectra within 5 min and requires less than 1 mg of sample (Citation26). It is also able to detect fentanyl and its analogues at lower concentrations than immunoassay strips and FTIR (Citation25).
In response to the limited ability of point-of-care drug-checking technologies to reliably detect the presence of the increasingly prevalent adulterant etizolam (Citation16), Gozdzialski et al. (Citation28) demonstrated that portable surface-enhanced Raman spectroscopy (SERS) has a high specificity and sensitivity in detecting the substance in a community setting. Raman spectroscopy is a widely used technique in the forensic analysis of drugs that generates spectra based on differences in the frequency of scattered light produced when a sample is exposed to a monochromatic laser and the incident light itself (Citation29). Raman scattering refers to the portion of scattered light that has a frequency that differs from that of the incident light (Citation29). In SERS, when a sample is placed in close proximity to a roughened surface with metallic nanoparticles (e.g., gold, silver, and copper), illumination using a monochromatic laser can lead to the excitation of collective electron oscillations (plasmons) (Citation30), which, in turn, intensifies the local electromagnetic field and Raman scattering (Citation30,Citation31). The consequent increase in sensitivity is conducive to detecting substances at trace levels () (Citation28).
Figure 1. Schematic illustrations of surface enhanced Raman spectroscopy (a) and paper-spray mass spectrometer. (b) Created with BioRender.com. Adapted from Zheng and He (Citation32).
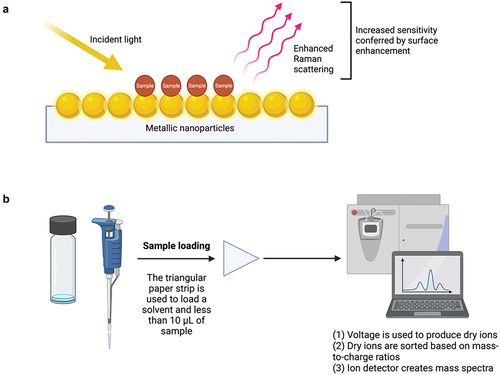
Due to its ability to detect etizolam, unlike existing point-of-care technologies, SERS demonstrates promise as a tool in adapting to the changing drug supply. To begin determining the feasibility of SERS in detecting etizolam in the unregulated drug supply, Gozdzialski et al. (Citation28) analyzed 100 opioid samples using the technology, establishing that SERS can be used to reliably detect novel adulterants like etizolam in street samples of opioid, each of which contained fentanyl in addition to complex mixtures of other substances. By positioning the sample in close contact with a metal surface or nanoparticles, studies have shown that SERS can exceed the sensitivity of conventional Raman spectroscopy and immunoassay strips (Citation28). Gozdzialski et al. (Citation28) found that the sensitivity (96%) of SERS in detecting etizolam was far higher than that of benzodiazepine immunoassay strips (8%). Like FTIR, drug checking using Raman spectroscopy involves comparing the spectra with a reference library to identify a compound (Citation14). Using a roughened surface with nanoparticles to enhance the sensitivity of Raman spectroscopy (Citation30), SERS is able to detect etizolam in the unregulated supply and is highly portable, requires small amounts of sample and provides results within minutes, therefore exhibiting promise as a future point-of-care drug checking technology () (Citation28).
Table 1. Comparison of recently deployed point of care drug-checking technologies: Fourier-transform infrared spectroscopy, paper spray mass spectrometry, and surface-enhanced Raman spectroscopy.
Both PS-MS and SERS are examples of emerging tools that address the shortcomings of existing point-of-care technologies. Immunoassay strips and FTIR are limited in their ability to detect unexpected and novel substances, and, in the context of a ceaselessly dynamic unregulated supply, the technologies we employ must also be able to adjust to these changes. These emerging technologies, and others that are currently in development, display the capability to augment existing technologies both in terms of the substances they can detect and their ability to identify substances at extremely low concentrations. These new technologies can not only improve upon the sensitivity and specificity, but they also have the potential to fundamentally change our approach to drug checking from one that is static to a dynamic approach that is reactive to changes in the unregulated supply. However, the utility of these emerging technologies may be limited by the need for expertise to operate and manage the apparatus, electrical demands, and higher costs compared to simpler technologies like immunoassay strips (Citation26). Because studies on the application of PS-MS and SERS at a larger scale have yet to be conducted, and due to their ability to conduct highly sensitive, rapid, and portable drug checking, we encourage further research on the feasibility of using these emerging technologies outside of the context of pilot studies.
We argue that there is likely no “one size fits all” approach to drug checking and that, in addition to the implementation of technologies, approaches to service delivery also need to be nimble to respond to the emergence of novel psychoactive substances in different settings. Given the dynamic nature of the unregulated supply, the technologies and strategies that can be used to best respond to overdose mortality will likely change with time, reducing the effectiveness of best practice guidelines or strategies that are set prematurely. As such, the use of drug checking technology should evolve with the unregulated drug supply. There continue to be advantages to laboratory-based service delivery models to accurately identify novel substances that appear in the unregulated drug market; however, the resources required and time to return results are a significant drawback. Different service delivery models, such as mobile services and offsite services, should also be used strategically to optimize the technologies at our disposal and should cater to the accessibility needs of people who use drugs in their communities. A truly effective approach to drug checking, then, must account for the fact that each technology and service model has unique benefits and limitations, and should be selected based on logistical considerations and changes in the drug supply.
Conclusion
We contend that a stagnant approach to drug checking does not adequately respond to changes in the composition of the supply and risks underestimating the emergence of novel adulterants that are not detected by current technologies. We argue that there is an urgent need for a broader array of drug checking technologies and service delivery approaches that can adapt to the constantly changing North American market. Because each drug checking technology and service model is associated with its own limitations, we argue that, instead of a “one size fits all approach,” we flexibly employ a diverse repertoire of drug checking models and technologies to address the overdose crisis. Future research on drug checking should validate new technologies coming down the pipeline and explore how this information can be used to inform and enhance public health interventions aimed at mitigating overdose mortality.
Author contributions
Conception and design: LT, IB
Literature search, initial draft: IB
Writing of article: IB, ST, LT
Revisions of article, final approval: IB, ST, LT
Disclosure statement
No potential conflict of interest was reported by the author(s).
Additional information
Funding
References
- Maghsoudi N, McDonald K, Stefan C, Beriault DR, Mason K, Barnaby L, Altenberg J, MacDonald RD, Caldwell J, Nisenbaum R, et al. Drug checking services in Toronto working group. Evaluating networked drug checking services in Toronto, Ontario: study protocol and rationale. Harm Reduct J. 2020;17:9. doi:10.1186/s12954-019-0336-0. Cited in: PMID: 32204713.
- Humphreys K, Shover CL, Andrews CM, Bohnert ASB, Brandeau ML, Caulkins JP, Chen JH, Cuéllar MF, Hurd YL, Juurlink DN, et al. Responding to the opioid crisis in North America and beyond: recommendations of the Stanford-Lancet commission. Lancet. 2022;399:555–604. doi:10.1016/S0140-6736(21)02252-2. Cited in: PMID: 35122753.
- Vandeputte MM, Van Uytfanghe K, Layle NK, St Germaine DM, Iula DM, Stove CP. Synthesis, chemical characterization, and μ-Opioid receptor activity assessment of the emerging group of “Nitazene” 2-Benzylbenzimidazole synthetic opioids. ACS Chem Neurosci. 2021;12:1241–51. doi:10.1021/acschemneuro.1c00064. Cited in: PMID: 33759494.
- Belzak L, Halverson J. Evidence synthesis - the opioid crisis in Canada: a national perspective. Health Promot Chronic Dis Prev Can. 2018;38:224–33. doi:10.24095/hpcdp.38.6.02. Cited in: PMID: 29911818.
- Shanks KG, Behonick GS. Detection of carfentanil by LC-MS-MS and reports of associated fatalities in the USA. J Anal Toxicol. 2017;41:466–72. doi:10.1093/jat/bkx042. Cited in: PMID: 28830120.
- Papsun D, Isenschmid D, Logan BK. Observed carfentanil concentrations in 355 blood specimens from forensic investigations. J Anal Toxicol. 2017;41:777–78. doi:10.1093/jat/bkx068. Cited in: PMID: 28977381.
- Swanson DM, Hair LS, Strauch Rivers SR, Smyth BC, Brogan SC, Ventoso AD, Vaccaro SL, Pearson JM. Fatalities involving carfentanil and furanyl fentanyl: two case reports. J Anal Toxicol. 2017;41:498–502. doi:10.1093/jat/bkx037. Cited in: PMID: 28575422.
- Armenian P, Vo KT, Barr-Walker J, Lynch KL. Fentanyl, fentanyl analogs and novel synthetic opioids: a comprehensive review. Neuropharmacology. 2018;134:121–32. doi:10.1016/j.neuropharm.2017.10.016. Cited in: PMID: 29042317.
- British Columbia Coroners Service. Illicit drug toxicity type of drug data (to Oct. 31, 2021). Government of British Columbia; 2022 Jun 9 [accessed 2022 Jul 2]. https://www2.gov.bc.ca/assets/gov/birth-adoption-death-marriage-and-divorce/deaths/coroners-service/statistical/illicit-drug-type.pdf.
- Leen JLS, Juurlink DN. Carfentanil: a narrative review of its pharmacology and public health concerns. Can J Anaesth. 2019;66:414–21. English. doi:10.1007/s12630-019-01294-y. English.
- Ontario Agency for Health Protection and Promotion. Novel non-fentanyl synthetic opioids: risk assessment and implications for practice. Public Health Ontario; 2021 Oct 8 [accessed 2021 Dec 22]. https://www.publichealthontario.ca/-/media/documents/e/2021/evidence-brief-novel-opioids-risk-analysis-implications.
- Santé Montréal. Risk of death linked to use of isotonitazene pills. Government of Quebec; 2020 Nov 5 [accessed 2021 Dec 24]. https://santemontreal.qc.ca/en/public/fh/news/news/risk-of-death-linked-to-use-of-isotonitazene-pills/.
- Trecki J, Gerona RR, Ellison R, Thomas C, Mileusnic-Polchan D. Notes from the field: increased incidence of fentanyl-related deaths involving para -fluorofentanyl or Metonitazene — Knox County, Tennessee, November 2020–August 2021. MMWR Morb Mortal Wkly Rep. 2022;71:153–55 doi:10.15585/mmwr.mm7104a3external_icon.
- Harper L, Powell J, Pijl EM. An overview of forensic drug testing methods and their suitability for harm reduction point-of-care services. Harm Reduct J. 2017;14:52. doi:10.1186/s12954-017-0179-5.
- Tobias S, Shapiro A, Wu H, Ti L. Xylazine identified in the unregulated drug supply in British Columbia, Canada. Can J Addict. 2020;11:28–32. doi:10.1097/CXA.0000000000000089.
- Laing MK, Ti L, Marmel A, Tobias S, Shapiro AM, Laing R, Lysyshyn M, Socías ME. An outbreak of novel psychoactive substance benzodiazepines in the unregulated drug supply: preliminary results from a community drug checking program using point-of-care and confirmatory methods. Int J Drug Policy. 2021;93:103169. doi:10.1016/j.drugpo.2021.103169. Cited in: PMID: 33627302.
- Shapiro A, Sim D, Wu H, Mogg M, Tobias S, Patel P, Ti L.Detection of etizolam, flualprazolam, and flubromazolam by benzodiazepine-specific lateral flow immunoassay test strips. British Columbia Centre for Substance Use; 2020 Jul 1 [accessed 2021 Dec 27]. https://www.bccsu.ca/wp-content/uploads/2020/08/BenzoTestStrip_Report.pdf.
- Impact Canada. Drug checking technology challenge. Government of Canada; [accessed 2022 Jul 2]. https://impact.canada.ca/challenges/drug-checking-challenge/results.
- Vandergrift GW, Hessels AJ, Palaty J, Krogh ET, Gill CG. Paper spray mass spectrometry for the direct, semi-quantitative measurement of fentanyl and norfentanyl in complex matrices. Clin Biochem. 2018;54:106–11. doi:10.1016/j.clinbiochem.2018.02.005. Cited in: PMID: 29432758.
- Espy RD, Muliadi AR, Ouyang Z, Cooks RG. Spray mechanism in paper spray ionization. Int J Mass Spectrom. 2012;325-327:167–71. doi:10.1016/j.ijms.2012.06.017.
- Wang H, Liu J, Cooks RG, Ouyang Z. Paper spray for direct analysis of complex mixtures using mass spectrometry. Angew Chem Int Ed Engl. 2010;49:877–80. doi:10.1002/anie.200906314.
- Urban PL. Quantitative mass spectrometry: an overview. Philos Trans A Math Phys Eng Sci. 2016;374:20150382. doi:10.1098/rsta.2015.0382.
- McCrae K, Tobias S, Grant C, Lysyshyn M, Laing R, Wood E, Ti L. Assessing the limit of detection of Fourier-transform infrared spectroscopy and immunoassay strips for fentanyl in a real-world setting. Drug Alcohol Rev. 2020 Jan;39:98–102. doi:10.1111/dar.13004. Cited in: PMID: 31746056.
- Sutter ME, Gerona RR, Davis MT, Roche BM, Colby DK, Chenoweth JA, Adams AJ, Owen KP, Ford JB, Black HB, et al. Fatal fentanyl: one pill can kill. Acad Emerg Med. 2017;24:106–13. doi:10.1111/acem.13034. Cited in: PMID: 27322591.
- Borden SA, Saatchi A, Krogh ET, Gill CG. Rapid and quantitative determination of fentanyls and pharmaceuticals from powdered drug samples by paper spray mass spectrometry. Anal Sci Adv. 2020;1:97–108. doi:10.1002/ansa.202000083.
- Borden SA, Saatchi A, Vandergrift GW, Palaty J, Lysyshyn M, Gill CG. A new quantitative drug checking technology for harm reduction: pilot study in Vancouver, Canada using paper spray mass spectrometry. Drug Alcohol Rev. 2022;41:410–18. doi:10.1111/dar.13370. Cited in: PMID: 34347332.
- Vandergrift GW, Gill CG. Paper spray mass spectrometry: a new drug checking tool for harm reduction in the opioid overdose crisis. J Mass Spectrom. 2019;54:729–37. doi:10.1002/jms.4431. PMID: 31432563.
- Gozdzialski L, Rowley A, Borden SA, Saatchi A, Gill CG, Wallace B, Hore DK. Rapid and accurate etizolam detection using surface-enhanced Raman spectroscopy for community drug checking. Int J Drug Policy. 2022;102:103611. doi:10.1016/j.drugpo.2022.103611. Cited in: PMID: 35151084.
- Bumbrah GS, Sharma RM. Raman spectroscopy – Basic principle, instrumentation and selected applications for the characterization of drugs of abuse. Egypt J Forensic Sci. 2016;6:209–15. doi:10.1016/j.ejfs.2015.06.001.
- Uskoković-Marković S, Kuntić V, Bajuk-Bogdanović D, Holclajtner-Antunović I. Surface-ehnanced raman scattering (SERS) biochemical applications. In: Encyclopedia of spectroscopy and spectrometry. 3rd ed. Academic Press; 2017. p. 383–88. doi:10.1016/B978-0-12-409547-2.12163-8.
- Azimi S, Docoslis A. Recent advances in the use of surface-enhanced raman scattering for illicit drug detection. Sensors (Basel). 2022;22:3877. doi:10.3390/s22103877.
- Zheng J, He L. Surface-enhanced raman spectroscopy for the chemical analysis of food. Compr Rev Food Sci Food Saf. 2014;13:317–28. doi:10.1111/1541-4337.12062.