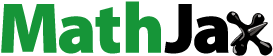
ABSTRACT
This review summarizes the recent studies on the synthesis of secondary amines by one-pot amination of aldehydes and ketones over heterogeneous catalysts. Amines are widely applied as the key intermediates in chemical industry for the synthesis of various commodities such as agrochemicals, drugs, detergents, lubricants, food-additives and polymers. Direct catalytic reductive amination of carbonyl compounds was considered which generally includes two steps: (i) formation of imines by interactions of aldehydes or ketones with amines, and (ii) subsequent hydrogenation of imines. Synthesis of secondary amines from carbonyl compounds and amines generated in situ under reaction conditions from their progenitors, e.g., respectively, alcohols or nitro-compounds, is also discussed in detail. Recent progress in application of hydrogen sources alternative to gaseous H2, such as formic acid, NaBH4, CO and water, favored development of metal-free catalysts including solid acid catalysts. The review addresses the scope of the amination reaction with aldehydes/ketones and nitro/amine compounds of different structure, the effect of the solvent, reaction conditions and catalyst properties. In addition, catalyst regeneration and reuse, kinetic regularities and kinetic modeling with an emphasis on the continuous mode of one-pot amination have been systematically summarized and discussed. It is suggested that the future work should focus on revealing the role of the catalytically active sites addressing their acid–base properties and the correlation between catalyst properties and the reaction performance, elucidating kinetic parameters and designing feasible reactor system for further industrial implementation.
1. Introduction
Efficient preparation of functionalized amines is of significant interest for synthesis of fine chemicals. Direct reductive amination of carbonyl compounds is in this context a promising method for preparation of amine derivatives, comprising first formation of imines by a reaction of aldehydes or ketones with amines and their subsequent hydrogenation. As with other one-pot processes such methodology brings clear ecological and economic benefits compared with the stepwise processes.[1,Citation2] One-pot reductive amination of carbonyl compounds to produce secondary amines is an important research field, because these products are used as intermediates for synthesis of drugs,[Citation3]such as, for example, 4-benzyl morpholine intermediate utilized in synthesis of drugs against lung cancer[Citation4] or N-benzylaniline applied for synthesis of dibenzazepines[Citation5] and neurogenic agents,[Citation6] as well as preparation of 2,5-dideoxy-2,5-imino-D-altritol (DIA), an inhibitor of α-galactosidase A and a valuable compound in the Fabry disease treatment.[Citation7] Pharmaceutical activity of N-substituted 5-(hydroxymethyl)-2-furfuryl amines as for example muscarinic agonists or cholinergic agents, has been also reported.[Citation8,Citation9] One-pot synthesis is highly desirable making the process more economically feasible avoiding separation of the intermediate, purification steps and consuming less energy.[Citation1,Citation2]
Synthesis of secondary amines from aldehydes and ketones has been intensively studied using amines as a nitrogen source and as an example reaction scheme for reductive amination of cyclohexanone with benzylamine is depicted ().[Citation2,Citation10–27]
Figure 1. Reductive amination of cyclohexanone with benzylamine. Adapted fromRef. [Citation10] Open access.
![Figure 1. Reductive amination of cyclohexanone with benzylamine. Adapted fromRef. [Citation10] Open access.](/cms/asset/c28ef87e-2779-4100-9e28-c6e3224c2c80/lctr_a_1942689_f0001_b.gif)
Synthesis of secondary am ines can be realized via the one-pot synthesis where carbonyl compounds as well as amines are generated in situ under reaction conditions from their progenitors, e.g. alcohols or nitro-compounds, respectively. In that case, aldehydes or ketones with nitro-compounds are introduced into a reactor where the latter reagents are rapidly converted under a reductive atmosphere forming in situ amines, which in turn react with a carbonyl group forming the corresponding imines. Similarly, in situ dehydrogenation of alcohols gives carbonyl compounds, which in the presence of amines result in targeted imines. In the second step, the formed imines are hydrogenated to the desired secondary amines, for example amination of nitrobenzene with benzaldehyde ().[Citation28–30] The latter reaction corresponds to a so-called hydrogen borrowing reaction described in detail in the literature[Citation31–35] and therefore not considered in the current review. Note that the first application of typical heterogeneous catalysts in direct terpene alcohol amination was demonstrated in a series of recent studies.[Citation36–43]
Figure 2. Reductive one-pot amination of nitrobenzene with benzaldehyde adapted from.[Citation28–30] Copyright permission from John Wiley & Sons and from Royal Society of Chemistry.
![Figure 2. Reductive one-pot amination of nitrobenzene with benzaldehyde adapted from.[Citation28–30] Copyright permission from John Wiley & Sons and from Royal Society of Chemistry.](/cms/asset/a6e3466c-e6aa-4d10-9805-152c3649590c/lctr_a_1942689_f0002_b.gif)
Potentially attractive aldehydes and ketones can be obtained from the biorefinery processes,[Citation26,Citation44,Citation45] while nitrobenzene is obtained via nitration of benzene. In the transformations of carbonyl compounds with nitroaromatic compounds to secondary amines several monometallic supported catalysts have been applied, such as Pd,[Citation46–48] Pt,[Citation30] Au modified by NH2 groups,[Citation49] Ni supported on H- ZSM-5 zeolite (Ni/H-mZSM-5)[Citation50] and bimetallic noble metal catalysts.[Citation11,Citation51,Citation52] To overcome the high price of noble metal catalysts, cheaper transition metal catalysts have also been applied.[Citation23,Citation28,Citation50,Citation53]
Typically reactions are carried out in the temperature range of 25–190°C[Citation52,Citation53] under 1–50 bar hydrogen pressure[Citation28,Citation52] or with other hydrogen sources including in-situ generated hydrogen from the water gas shift reaction,[Citation23,Citation54] decomposition of formic acid[Citation53,Citation55,Citation56] and sodium borohydride.[Citation12,Citation19,Citation50] When other hydrogen sources are applied instead of hydrogen, no metal is required to dissociate molecular hydrogen and several other types of catalysts can be applied including solid acid catalysts (CSBA).[Citation12]
The reaction scope of amination has also been very intensively studied, i.e. by changing the structure of aldehyde/ketone and nitro/amine compounds as recently summarized in Ref. [Citation57,Citation58]. Typically the literature on amination provides only the yields of the desired product at the end of the reaction without reporting kinetics.[Citation1,Citation2,Citation23,Citation28,Citation29,Citation46,Citation47,Citation51,Citation52,Citation56,Citation59–61] In some studies, the reaction conditions for transformations of aldehyde and ketones with amines or secondary amines were different.[Citation11,Citation15,Citation17,Citation23,Citation49,Citation54,Citation61,Citation62]
The aim of this review is to elucidate amination of carbonyl compounds from the engineering point of view reporting the effect of the solvent,[Citation14,Citation23–26,Citation28,Citation49–52,Citation61] reaction conditions,[Citation2,Citation11,Citation14,Citation15,Citation19,Citation23,Citation48,Citation54,Citation61,Citation63,Citation64] catalyst properties,[Citation2,Citation10,Citation11,Citation14,Citation15,Citation17,Citation21,Citation23,Citation24,Citation26,Citation28,Citation30,Citation46–48,Citation50–52,Citation59–61,Citation65–69] regeneration and reuse,[Citation14,Citation23,Citation25,Citation46,Citation52,Citation53,Citation56,Citation63,Citation70,Citation71] kinetic regularities[Citation10,Citation23,Citation53,Citation61] and kinetic modeling.[Citation61] In addition, results on amination of carbonyl compounds in a continuous mode will be discussed in detail.[Citation20,Citation24,Citation63,Citation71–74] Another aim is also to understand the catalyst property–performance relationship and elucidate the reaction mechanisms,[Citation2,Citation48,Citation49,Citation54,Citation56,Citation59,Citation63] related to production of secondary amines from carbonyl compounds. Reductive amination of aldehyde/ketone with a primary amine forming in the first step an imine, which is then hydrogenated to an amine, is discussed in Section 2 (), while one-pot reductive amination of nitrocompounds with primary amines, which includes as an additional step hydrogenation of the nitro compound to amine, is summarized in Section 3 (). Even a ketone can be prepared via dehydrogenation from an alcohol. In addition, both in Section 2 and Section 3 also the results using other reductants than molecular hydrogen are reviewed. In Section 4, conclusions and future outlook are given.
2. Amination of aldehydes and ketones with amines
2.1. Molecular hydrogen as a hydrogen source
2.1.1. Catalyst selection for the synthesis of secondary amines from carbonyl compounds with amine using molecular hydrogen
Amination of aldehydes and ketones has been typically studied with amines as reactants.[Citation11,,Citation15,,Citation17,,Citation71,,Citation72] Several researchers have studied amination of benzaldehyde with aniline or their substituted analogues.[Citation11,Citation15,Citation17,Citation24,Citation65,Citation71,Citation72] Monometallic commercial catalysts, such as Pd/C and Ru/C, displayed low selectivity in one-pot amination of benzaldehyde with aniline.[Citation11] Similarly, Ru/C and Rh/C catalysts in the amination of 4-fluorobenzaldehyde with 4-methoxyaniline[Citation75] as well as Cu/Al2O3 in the amination of 4-methoxyacetophenone[Citation69] were not selective clearly showing a need for bifunctional catalysts in amination of carbonyl compounds.[Citation61] Small Pd particles supported on NiO,[Citation15] bimetallic Fe@Pd/C,[Citation11] ligand attached Pd particles supported on a polymer,[Citation17] Pd-Cu-β-cyclodextrin,[Citation66] Pd/C covered by carbonaceous particles[Citation67] and UiO-67-para-phenylenediamine-Pd[Citation68] have been very efficient catalysts for amination of aldehydes with amines (, entries 1–3, 5–9, 11–13 and , entries 1–6). It should be pointed out here that aldehydes are typically easier to be transformed to secondary amines in comparison with ketones as will be discussed further. When comparing properties of these catalysts, it was observed that the hydrogenation activity of Pd was suppressed by embedding it into a polymeric support,[Citation17] cyclodextrin matrix,[66] carbonaceous support[Citation67] or by using for example Fe@Pd/C catalyst with a lower hydrogenation ability. The latter catalyst contains iron atoms partially substituted with Pd. In addition ionic Pd2+ species coordinated to a nitrogen containing polymer are also present.[Citation68] It is also interesting to compare amine structure in reductive amination of benzaldehyde. For example, Pd/NiO with rather small Pd particles (5 nm) (Table S2, entry 1) was an efficient catalyst in reductive amination of benzaldehyde with cyclohexylamine under mild conditions (, entry 1), however, its amination with aniline resulted in a slightly lower yield of the secondary amine (, entry 6) under comparative conditions.[Citation15] It was furthermore stated that even a reversible dehydrogenation reaction of the N-benzylaniline can occur, thus lowering its yield after prolonged reaction times.[Citation15] In Ref. [Citation15], the reactions were performed in a reaction tube, however, not clearly specifying if there was any stirring and what was the catalyst particle size. Thus, these results might have been obtained under diffusional limitations. As a comparison, a two-step process was proposed in Ref. [Citation71], in which benzaldehyde reacted with aniline in the absence of a catalyst in methanol forming an imine, which in the second step was hydrogenated at 120°C under 20 bar H2 in a flow reactor giving a high yield of benzyl aniline (, entry 4).[Citation71] A constant yield of N-benzylamine was obtained during 6 h time-on-stream. Overall, reductive amination of benzaldehyde and its para-substituted derivatives has resulted in high yields of secondary amines. The reported literature suffers, however, from incomplete data, as for example, in reductive amination of benzaldehyde with 4-chloroaniline only the yield of amine was given (, entry 7).[Citation17]
Table 1. Amination of aldehyde and ketones with amines using molecular hydrogen.a Notation: AM amine, IM imine, AL alcohol, KET ketone, ALD aldehyde.
Table 2. Reductive amination of α-methyltryptamine derivative to N-alkyl-α-methyltryptamine derivative after 18 h. Adapted from Ref. [Citation27] Copyright permission from Elsevier Ltd.
Polystyrene embedded N-heterocyclic carbine-linked Pd was also an efficient catalyst in furfural amination with aniline (, entry 12) analogously to benzaldehyde.[Citation17] Even Pd/C with the palladium particle size of 2.7 nm (Table S1, entry 5) was very efficient giving only amine as the product (, entry 13), while Pd/Al2O3 resulted in only 80% yield of the amine at 100°C under 3 bar hydrogen.[Citation67] The reason for this were strong interactions of furfural with Pd(111) on Al2O3 confirmed by DRIFTs measurements, while Pd/C contains less Pd(111) sites. In furfural amination, the furan ring was hydrogenated over Pd/Al2O3. In reductive amination of furfural gold, platinum, and iridium colloids supported on sulfonic acid-functionalized silica have been utilized (, entries 14–17 and Table S1, entries 13–17).[Citation26] Such colloids were uniformly dispersed across the support. Interactions of sulfonic acid groups and metal sites confirmed by XPS are favorable for the imine formation and subsequent hydrogenation. Activity of Pt and It in reductive amination of furfural was similar exceeding that of gold, which can be associated with a limited ability of gold to catalyze the hydrogenation step. It was reported that selectivity to the amine was independent on the metal nature as not affected by the metal type, further side reactions were prevented because of the interactions of sulfonic groups with the metal.[Citation26] Similar amine yields of ca. 8% were obtained on Pt on SiO2 prepared by the colloidal method or by impregnation, while introduction of sulfonic groups elevated the amine yield to 23% over Pt/SiO2–SO3H illustrating the role of acidic sites in reductive amination of aldehydes with amines.[Citation26]
Reductive amination of HMF and its derivatives has been intensively investigated (, entries 18–30).[Citation24,Citation66,Citation67] 1 wt% Pd/C with small Pd particles (2.7 nm) (Table S1, entry 5) gave only the desired amine as a product in HMF amination (, entry 19), while over 1 wt% Pd supported on Al2O3 95% yield of the amine together with the over-hydrogenated [5-(anilinomethyl)-oxolan-2-yl]methanol was obtained. On the other hand, interactions of the furan ring with Al2O3 in furfural were more prominent than in case of HMF, and it was confirmed by infrared spectroscopy that in reductive amination of HMF interactions of the furan ring with Pd(111) surface were suppressed due to presence of a hydroxyl group in HMF when compared to furfural amination.[Citation66] In the amination of AMF, over Pt/Al2O3 in methanol only 81% yield of the secondary amine was obtained due to formation of the corresponding alcohol (10%) and imine (9%) (, entry 28).[Citation24] On the other hand, when using 2-propanol as a solvent the main product was alcohol, 68%, and only 20% of the secondary amine was formed together with 12% imine (, entry 29).[Citation24]
Ketone aminations have also been largely investigated (, entries 30–45). Even amination of cyclohexanone with ammonia was reviewed because of its prominent solvent effect (see Section 2.1.3).[Citation25] Reductive amination of cyclohexanone for production of secondary amines was performed over monometallic Pd[Citation17] and bimetallic Pd-Fe catalysts[Citation11] as well as over Au/TiO2.[Citation10] High yields can also be obtained in amination of ketones, for example 91% yield of the desired amine was reported in in amination of cyclohexanone with aniline over polystyrene embedded N-heterocyclic carbene-linked (NHC) Pd particles.[Citation17] In several studies, relatively high pressures and temperatures were used[Citation10,Citation11,Citation17] in comparison to aldehyde amination. In amination of cyclohexanone with benzylamine, Au/TiO2 with gold particles of ca. 3 nm size was producing 72% yield of the desired amine at 100°C under 30 bar hydrogen in cyclohexane as a solvent (, entry 35, Table S1, entry 7).[Citation10]
On the other hand, acetophenone with electron donating methoxy- and electron-withdrawing fluoro groups at para position could be rather selectively transformed to the corresponding amines in the reaction with benzylamine at 100°C under 1 bar hydrogen[Citation69] and at 90°C under 10 bar hydrogen,[Citation61] yielding 86% and 90%, amine, respectively (, entries 41 and 42). In the former case, the other products were imine (Y = 2%) and 1-phenylethanol (Y = 6%) formed by hydrogenation of the carbonyl bond.
When a sterically more hindered ketone, e.g. propiophenone, was used as a reactant, the best result was obtained over Au/TiO2 with 2.9 nm Au particles with only 11% yield of the desired amine under the same reaction conditions when propiophenone conversion was only 20% (, entry 44).[Citation10] Similar results were obtained in propiophenone amination with R-1-phenyl ethaneamine over Pd supported on polyethyleneimine exhibiting 2 nm Pd particles giving only 12% amine yield at 50°C under 3 bar hydrogen in 21 h (, entry 43).[Citation21] The main product in Ref. [Citation21] was imine indicating that electronic effects of the substrate are highly important in reductive amination of ketones.[Citation21]
Formation of N-alkylated α-methyltryptamine derivatives with 0–78% yield depending on the carbonyl structure was reported in reductive amination of α-methyltryptamine derivative (, , entry 45) with different aldehydes and ketones under 3 bar of hydrogen pressure using 10% Pd/C catalyst in methanol ().[Citation27]
Figure 4. Reductive amination of α-methyltryptamine derivative into N,N-dialkyl-α-methyltryptamine derivative adapted from.[Citation27] Copyright permission from Elsevier Ltd.
![Figure 4. Reductive amination of α-methyltryptamine derivative into N,N-dialkyl-α-methyltryptamine derivative adapted from.[Citation27] Copyright permission from Elsevier Ltd.](/cms/asset/85cf496b-d5e9-4240-83df-2882b2ba022b/lctr_a_1942689_f0004_b.gif)
Table 3. Solvent effect in reductive amination of furfural with aniline over Pt/SiO2–SO3 catalyst. Reaction conditions: furfural, 6 mmol; aniline, 5 mmol; catalyst, 0.15 mol%; ethyl acetate, 40 mL; H2 pressure, 5 MPa; room temperature; 8 h, adapted from Ref .[Citation26] Copyright from Elsevier Ltd.
2.1.2. Effect of catalyst properties in reductive amination of aldehydes and ketones
Metal loading and structure sensitivity are also important parameters in reductive amination of carbonyl compounds with amines.[Citation61,Citation67] For example, amination of 4-fluoroacetophenone with benzylamine was studied over different Pd-MIL-101(Cr) catalysts exhibiting the same metal particle size.[Citation61] Due to different Pd loading, the only difference in these catalysts is the ratio between Pd and Lewis acid sites, which are present in MIL-101 support. The results revealed that the selectivity to the desired amine increased with decreasing the metal loading. In addition, Pd loading on carbon was systematically studied in reductive amination of HMF with aniline in trifluorotoluene (TFT) as a solvent at 100°C under 3 bar hydrogen.[Citation67] The results showed that with larger Pd particles both activity and selectivity of the catalysts decreased. Highly dispersed Pd particles in Pd/C were the most efficient for amination of HMF with aniline, while for larger Pd particles complete imine conversion was not obtained.[Citation67]
Effect of the support has been investigated in comparative experiments using Pd/C and Pd/Al2O3 with the same metal particle size illustrating that Pd/C was more selective. The reason for this was explained by CO adsorption experiments revealing that a larger amount of Pd(111) facilitates stronger interactions of the furan ring in Pd/Al2O3 in comparison to Pd/C.[Citation67] Acidity of the support has been investigated in Ref. [Citation61,Citation69], when Cu was supported on alumina, silica, SiO2-TiO2 and SiO2-Al2O3 in amination of 4-methoxyacetophenone with aniline at 100°C under 1 bar hydrogen in toluene. The highest yields of the desired amine were obtained with SiO2-TiO2 and SiO2-Al2O3 as supports being 97% and 96%, respectively. In addition, an acidic MIL-101 was used as a support for Pd in the amination of 4-fluoroacetophenone to produce nearly 90% yield of the corresponding secondary amine under 10 bar hydrogen at 90°C (, entry 42, Table S1, entry 12).[Citation61] However, MIL-101 containing Cr3+ ions with only Lewis acid sites, confirmed by ammonia TPD,[Citation65] was able to only catalyze formation of imine, while activation of ketone was not efficient.[Citation61] Furthermore, under comparative conditions, i.e. 90°C under 10 bar hydrogen, the yields of the desired amine were 90% and 28% for 0.2 wt% Pd-MIL-101 and 5 wt% Pd/C, respectively, while a large amount of hydrogenated 1-(4ʹ-fluorophenyl)ethanol was formed also over 5 wt% Pd/C demonstrating that the metal loading and the support are crucial for selective amination of this ketone.[Citation61] It was also observed that the unwanted hydrogenolysis of the formed desired amine is promoted with a high Pd loading especially at a high temperature.[Citation61]
2.1.3. Effect of reaction conditions
2.1.3.1. Effect of reaction temperature
Optimization of the reaction temperature and hydrogen pressure in reductive amination of aldehydes and ketones has been scarcely studied.[Citation11,Citation15,Citation61] The highest yield of N-benzylcyclohexanamine, 94%, was obtained over Fe@Pd/C catalyst in 8 h (, entry 3, Table S1, entry 3) and this catalyst was active and selective at the highest studied temperature 80°C and the highest pressure 30 bar. The yields of products under different reaction conditions were not reported. In addition, several mono- or bimetallic Pd catalysts supported on NiO,[Citation15] polymer-N-containing heterocyclic carbene (NHC)[Citation17] and β-cyclodextrin[Citation66] gave more than 90% yield at 50°C, 80°C and 180°C, respectively. Especially, Pd/NiO was very active and selective even at 25°C under atmospheric hydrogen pressure in transforming benzaldehyde with cyclohexylamine (, entry 1, Table S1, entry 1).[Citation15] As a comparison, the effect of temperature on the amine yield was studied in benzaldehyde amination with aniline in water under 30 bar hydrogen over Fe-Pd core shell structured catalyst on active carbon (denoted as Fe@Pd/C)[Citation11] and over Pd/NiO at 1 bar hydrogen in ethanol.[Citation15] In the latter case, the amine yield decreased at a higher temperature due to hydrogenation of benzaldehyde to toluene,[Citation15] while the amine yield increased over Fe@Pd/C[Citation11] at a higher temperature. It should, however, be pointed out here that in water a very high pressure was applied as hydrogen solubility in water is low.[Citation76]
High temperature also promoted decomposition of the desired secondary amine in the amination of 4-fluoroacetophenone with benzylamine to toluene and 4-fluoro-(1-acetophenone) over Pd-MIL-101 catalyst at 90°C under 10 bar hydrogen, while 50°C was the optimal temperature giving ca. 91% yield of the desired amine.[Citation61]
Furfural amination could be selectively performed over supported Pd catalysts in the temperature range of 80−100°C under 3–35 bar hydrogen (, entries 12, 13)[Citation17,Citation67] and over Me/SiO2–SO3H (Me = Au, Pt, Ir) at 90°C under 50 bar hydrogen (, entries 14–17, Table S1, entries 13–15).[Citation26] Especially, in ethyl acetate as a solvent high yields of imine were obtained over gold, iridium and platinum supported on SiO2-SO3H support lowering the yield of the amine (, entry 14).[Citation26] It should be pointed out that hydrogen solubility in solvents has not been addressed when different solvents were tested.[Citation17,Citation26] Furthermore, reductive amination of 5-acetoxymethylfurfural with primary amines (e.g. aniline) in methanol could be efficiently carried out at low temperatures, i.e. 10–25°C and 5 bar of hydrogen pressure providing 99% and 86% yield of the secondary amine over Pt/Al2O3 or Pd/Al2O3 as well as Pd/C catalysts, correspondingly.[Citation24]
Ketone amination with amine has also been successfully performed over various supported metal catalysts under varying reaction conditions.[Citation10,Citation17,Citation21,Citation61,Citation69] Note that in these studies pressure and temperature have not been typically optimized.[Citation10,Citation17,Citation21] The results demonstrated that ketone amination can be performed in the temperature and pressure range of 50–100°C and 1–30 bar hydrogen, respectively (, entries 35–45). A rather long reaction time has also been often needed (, entries 39–43).
2.1.3.2. Effect of hydrogen pressure
The effect of hydrogen pressure on the amine yields has been also studied.[Citation11,Citation17,Citation68] The results show that for benzaldehyde amination relatively high pressure promote amine formation (, entry 2, 3, 8, 9).[Citation11,Citation17] At the same time, Pd/NiO was active and selective in benzaldehyde amination already at 25°C under 1 bar hydrogen (, entry 1),[Citation15] while higher reaction temperatures gave lower amine yields and more toluene was formed by hydrogenation of benzaldehyde. In Ref. [Citation17], it was reported that the highest yields of the desired amine were obtained at high pressures, when the hydrogen pressure was varied in the range of 25–40 bar in amination of benzaldehyde with aniline at 80°C over PS-Pd-NHC in water. On the other hand, Pd supported on metal organic framework UiO modified with p-phenylenediamine was an efficient catalyst in this reaction in ethanol as a solvent at 50°C when more than 5 bar hydrogen was applied (, entry 20, Table S1 entry 6).[Citation68] The highest applied hydrogen pressure, 30 bar was efficient for production of N-benzylaniline from benzaldehyde and aniline over Fe@Pd/C in water,[Citation11] but no information about other products at lower pressures was given. Low hydrogen pressures were also applied to facilitate HMF amination with aniline over supported Pd catalysts giving high yields of the corresponding amine (, entry 19, 20).[Citation67,Citation68] It should be pointed out that such aspect as low hydrogen solubility in water[Citation76] has not been discussed at all indicating that more systematic physico-chemical approach is required in this research area.
2.1.3.3. Solvent effect
Several studies are available addressing the solvent effect in the synthesis of secondary amines by reductive amination of carbonyl compounds.[Citation15,Citation17,Citation25,Citation26,Citation65,Citation67] Typically 2-propanol and ethanol gave the highest amine yields,[Citation15] while rather comparative amine yields over Pd-β-cyclodextrine catalyst were achieved in ethanol and water.[Citation66] Water was reported as the best solvent for benzaldehyde amination with aniline .[Citation17] No clear correlation of the amine yield and the solvent dielectric constant could be established, which in part can be related to not considering solvent dependent hydrogen solubility. It should be mentioned in Ref. [Citation17] that a low yield of the desired amine (48%) in benzaldehyde amination with aniline over a Pd supported catalyst was reported without mentioning the yields of the side products.
Reductive amination of furfural with aniline was studied in methanol, water, ethyl acetate and THF over Pt/SiO2–SO3 (, entries 14–17)[Citation26] indicating that the highest amine yield was obtained in ethyl acetate (, entry 14).[Citation26] Lower amounts of side products in ethyl acetate is related to inhibition of the imine formation due to a decrease of aniline nucleophilic ability because of aniline. Alcoholic solvents exhibit more side reactions, in particular the reaction in methanol with furfural gave furfuryl acetal, competing with the imine formation. Reversibility of imine formation as well as generation of a hydroxylamine derivate is a feature of water as a solvent, which hinders utilization of the latter despite such positive characteristics as low costs, environmental friendless and safety. In THF there is no protonation of aniline which becomes available as a nucleophile. Subsequently, THF gave the highest imine yield despite condensation of the secondary amine ().[Citation26]
Table 4. Reductive amination of AMF with primary amines R-NH2 over Pt/Al2O3 catalyst adapted from Ref. [Citation24].
These results indicate that more systematic studies are required to unravel the effect of solvents on synthesis of secondary amines. For example, in amination of cyclohexanone with ammonia over Ru/C catalyst under hydrogen atmosphere[Citation25] in different solvents condensation of cyclohexanone with cyclohexylamine resulted in imine formation in methanol with the highest rate.[Citation25] The solvent effect in the synthesis of primary amine was studied in more detail considering formation of both imine and the Shiff base via interactions of synthesized primary amine again with the initial ketone. No clear correlations between the cyclohexylamine yield and the Hildebrand-Hansen and Kamlet-Taft parameters often used to explain the solvent effects were found.[Citation77,Citation78] Instead, the solvent has a profound influence on the catalyst reactivity and product distribution. Thus, in aprotic polar solvents, such as dioxane and THF, the main product was imine indicating inhibition of imine hydrogenation. Such inhibition effect in hydrogenation is plausibly due to the strong adsorption of the solvent on the catalysts blocking accessibility of the active sites. Solvent–catalyst interactions are weaker for protic and aprotic apolar solvents inhibiting hydrogenation. In protic solvents the reactions between the ketone and ammonia and the imine was more prominent than in aprotic apolar solvents. The lowest cyclohexylamine selectivity in water can be explained by instability of the imine and the Schiff base in water, and, faster C = O double bond hydrogenation. DFT calculations for C = O hydrogenation under basic conditions suggested involvement water in the reaction as a hydrogen donor. Not only on Ru but also over catalysts (), selectivity to cyclohexylamine in methanol was substantially higher than in water suggesting a general validity of the solvent effect.[Citation25]
Figure 5. Product distributions of reductive amination of cyclohexanone over Group VIII metal-based catalysts in methanol and water. Reaction conditions: Cyclohexanone 1 mmol, catalyst 0.02 g, solvent 3 mL, under 9 bar hydrogen, T = 25°C, agitator speed = 1000 rpm, time = 2 h adapted from Ref. [Citation25] Notation: 1 methanol, 2 water as a solvent. Copyright from Elsevier Ltd.
![Figure 5. Product distributions of reductive amination of cyclohexanone over Group VIII metal-based catalysts in methanol and water. Reaction conditions: Cyclohexanone 1 mmol, catalyst 0.02 g, solvent 3 mL, under 9 bar hydrogen, T = 25°C, agitator speed = 1000 rpm, time = 2 h adapted from Ref. [Citation25] Notation: 1 methanol, 2 water as a solvent. Copyright from Elsevier Ltd.](/cms/asset/533c5865-3b1a-4236-a8dd-609f859f2055/lctr_a_1942689_f0005_b.gif)
Continuous synthesis of N-substituted 5-(acetoxymethyl)-2-furfuryl amines was also shown to be efficient in methanol, which is a suitable solvent for condensation of aromatic aldehydes with primary aromatic amines as well as for hydrogenation of imines.[Citation71,Citation79,Citation80] Much lower yields of the imine in the first stage were observed in ethanol and isopropanol eventually retarding formation of the desired product (, entries 28, 29). Subsequently, 5-(acetoxymethyl)-2-furanmethanol was generated by hydrogenation of the initial aldehyde – 5-acetoxymethylfurfural.[Citation24]
2.1.3.3.1. Effect of reactant concentration
The effect of the initial reactant concentration has been very scarcely studied.[Citation69] It has been already known for a long time that the production of secondary amines from carbonyl compounds can be facilitated with a large excess of amine.[Citation81] The decreasing ratio of aniline/ketone in amination of 4-methoxyacetophenone with aniline over Cu/SiO2 gave less alcohol as a side product.[Citation69] At the same time, however, the yield of the desired amine decreased as well. Furthermore, it was stated in Ref. [Citation69] that the excess of amine in comparison to ketone suppressed formation of the dialkylated product.
2.1.4. Catalyst recycling
Typically catalyst recycling has been very successful in reductive amination of carbonyl compounds with amines[Citation11,Citation67] as there was no metal leaching.[Citation67] The yield of the desired amine decreased due to catalyst poisoning.[Citation69] For example, in benzaldehyde amination with aniline over Pd supported on polystyrene embedded N-containing heterocyclic carbene in water at 80°C at 35 bar hydrogen the yield of amine 93–94% remained the same in four cycles.[Citation17]
Pd supported on active carbon has been ra ather promising catalyst in reductive amination of aldehydes most probably due to rather mild reaction conditions.[Citation67,Citation69] For example, Pd/C catalyst was also successfully recycled in HMF amination with aniline under 3 bar hydrogen at 100°C when it was washed and dried at 100°C between the cycles.[Citation69] In addition, Pd/C catalyst was very active and selective in HMF amination with aniline at 100°C under 3 bar hydrogen giving 98% yield and only a minor decrease in the yield was observed in the third cycle.[Citation67] In Ref. [Citation67], the Pd/C catalyst was only filtrated and washed three times with ethanol after which it was reused without a separate reduction step prior to the second experiment. This result is very promising and most probably Pd remains stable due to rather mild reaction conditions. Furthermore, the filtrate test showed that the reaction was heterogeneously catalyzed.
On the other hand, degradation of the MOF support was observed in amination of HMF with aniline in ethanol at 50°C under 5 bar hydrogen. At the same time HMF conversion decreased by nearly 25%[Citation68] when the catalyst was only washed with ethanol and dried. It was also stated that aggregation and Pd leaching were observed. Analogously, the yield of amine decreased from 93% to 86% in the fourth cycle in benzaldehyde amination with aniline in water over Fe@Pd/C catalyst at 80°C under 30 bar hydrogen.[Citation11] Ru/C could be recycled after cyclohexanone reductive amination with ammonia in methanol resulting in a conversion decrease from ca. 95% to ca. 88% and indicating thus a reasonable catalyst stability. An increase of the imine yield was concomitant with lower yields of the Schiff base, cyclohexylamine and cyclohexanol.[Citation25] In recycling Cu/SiO2 catalyst in amination of 4-methoxy-acetophenone with aniline the yield of the product decreased from 86% to 71% during recycling,[Citation69] although no Cu leaching occurred. The reason for the lower yield of amine was stated to be catalyst poisoning rather than coking.
The yield of aminomethylhydroxymethylfuran (99%) did not change within 2.5 h time-on-stream in the reductive amination of 5-acetoxymethylfurfural (AMF) with aniline on Pt/Al2O3, which did not display any visible changes. Nevertheless, ca. 4.5 wt% of hydrogen-enriched carbonaceous species were formed according to TG-DSC-MS[Citation24] not affecting catalytic activity.
2.1.5. Kinetics and modeling in amination of carbonyl compounds with amines using molecular hydrogen
Reaction kinetics was investigated in HMF amination with aniline over Pd supported on UiO-67 in the presence of polymer (poly-para-phenylenediamine, PpDA) UiO-67/PpPDA/Pd at 50°C under 5 bar hydrogen.[Citation68] The results showed that the main product was imine, while also amine was already formed after first 5 min (). Then the maximum concentration of imine was reached already after 15 min and thereafter it reacted completely further to amine in 2 h.[Citation68]
Figure 6. Concentration profiles in HMF amination with aniline over Pd supported on poly-para-phenylenediamine modified metal organic framework catalyst UiO-67 in ethanol at 50°C under 5 bar hydrogen adapted from.[Citation68] Notation: (■) HMF conversion, (▲) imine and (●) amine yield. Copyright permission from Royal Society of Chemistry.
![Figure 6. Concentration profiles in HMF amination with aniline over Pd supported on poly-para-phenylenediamine modified metal organic framework catalyst UiO-67 in ethanol at 50°C under 5 bar hydrogen adapted from.[Citation68] Notation: (■) HMF conversion, (▲) imine and (●) amine yield. Copyright permission from Royal Society of Chemistry.](/cms/asset/638f7338-98a9-4ae4-999e-720bef655747/lctr_a_1942689_f0006_b.gif)
Modeling of the reaction kinetics in amination of 4-fluoroacetophenone with benzylamine was demonstrated.[Citation61] The reaction network contained as the first step imine formation followed by its hydrogenation. In addition, decomposition of the formed amine was also observed under the applied reaction conditions. Furthermore, it is known that the imine formation over Lewis acidic sites is a reversible reaction, which was also included in the reaction scheme:
in which A, B and U denote ketone, amine and the undesired amine, while I and D are imine and the secondary amine. Both hydrogenation and hydrolysis occurring on Pd are modeled with the first order reactions. It was observed that k2 and k3 were correlating with Pd loading.
2.1.6. Continuous amination of carbonyl compounds with amines over heterogeneous catalysts with molecular hydrogen
Reductive amination of aldehydes and ketones with amines has been demonstrated over Pd and Ni supported on a static mixer[Citation20] and over Ni supported on different supports.[Citation74] A comparative study of continuous amination of several amines with aldehydes and ketones was performed over Ni supported on NaX zeolite, carbon and magnesium oxide in the temperature range of 60–240°C under atmospheric pressure.[Citation74] Synthesis of several secondary amines was successfully demonstrated over these catalysts, however, time-on-stream behavior was not reported.[Citation74] Interestingly even dehydroaromatization of cyclohexylamine occurred over Ni/MgO in its amination with butylamine forming N-butylaniline instead of N-butylcyclohexylamine.[Citation74]
Continuous reductive amination in a tubular reactor containing a static mixer printed with Ni or Pd was demonstrated.[Citation20] The metals were loaded either via cold spraying (Ni) or electroplating (Pd). In Ref. [Citation20], amination of benzaldehyde with butylamine was optimized revealing that the reaction was efficient under 24 bar hydrogen pressure giving at 120°C in ethyl acetate as a solvent 77% yield of the desired amine over Ni supported on 3D printed catalytic static mixer (CSM) with a long residence time. After lowering the hydrogen pressure to 20 bar and increasing the liquid flow rate twofold, the main product was imine with 53% yield with the amine being the rest. As a part of scaling up a larger solution volume (1 L) was analyzed indicating only minor leaching of the metals (at ppb level). For Pd-CMS maximally 93% yield of N-benzylbutylamine was obtained under optimized conditions, 20 bar hydrogen at 120°C. In addition to benzaldehyde also other aldehydes and ketones were used as reactants including HMF, 5-methylfurfural, cinnamaldehyde, vanillin, 2-heptanone as well as nitrogen sources (4-anisaldehyde, α-methylbenzylamine, piperidine, morpholine). Catalyst fouling and humins formation was confirmed in the spent catalyst after using 5-methylfurfural and 5-(chloromethyl)furfural as reactants. Time-on-stream behavior was, however, not described.
Continuous mode reductive amination of 5-acetoxymethylfurfural (AMF) or HMF with aniline was performed in methanol as a solvent over packed bed catalysts including platinum, palladium and gold supported on carbon and alumina.[Citation24] The process includes non-catalytic condensation of AMF (or HMF as the initial aldehyde) with primary amines giving an imine and subsequent hydrogenation of the latter. Platinum catalysts afforded high yields of different N-substituted 5-(acetoxymethyl)-2-furfuryl amines at 25–55◦C and 5 bar H2, while low selectivity was seen on Pd and Au supported on γ-alumina and carbon (, entries 21–30).
The effect of the primary amine structure on formation of aminomethylhydroxymethylfuran (AMHMF) derivatives was studied using the reaction between AMF and different aromatic and aliphatic amines over Pt/Al2O3 catalyst according to ().
Figure 7. Scheme of reductive amination of AMF with different aromatic and aliphatic amines adapted from.[Citation24] Copyright permission from Elsevier Ltd.
![Figure 7. Scheme of reductive amination of AMF with different aromatic and aliphatic amines adapted from.[Citation24] Copyright permission from Elsevier Ltd.](/cms/asset/a5053073-7135-4eb8-a125-14ed28b241a1/lctr_a_1942689_f0007_b.gif)
High yields of secondary amines up to 99% were obtained[Citation24] with AMF and aromatic amines containing electron-donating substituents (i.e. methyl and methoxy-) in m- and p-positions (, entries 2, 3 and 6). Steric hindrance of the methyl group in o-position inhibited imine formation in the condensation of AMF and o-toluidine. Electron-withdrawing substituents (F, Cl, Br, I, C(O)CH3) in the para-position of aniline (, entries 7 − 13) resulted in weaker nucleophilic properties of the corresponding aromatic amines in comparison, thereby giving lower imine yields at the first stage.[Citation24] The order of the nucleophilicity decrease was in line with the desired product yield. The yield of aminomethyl hydroxymethylfuran (AMHMF) derivatives yield in the reaction between AMF and chloroaniline isomers was dependent on the position of an electron-withdrawing substituent in the aromatic amine (, entries 8, 14 − 16). Steric hindrance created by Cl substituent influences also hydrogenation of the imine, namely imines formed from m-chloroaniline and o-chloroaniline are less reactive than the imine obtained from p-chloroaniline (, entries 8, 14, 16).[Citation24] Significantly lower yields of AMHMF derivatives were observed for aliphatic amines (n-hexylamine, cyclohexylamine and n-dodecylamine) than for aniline (, entries 17 − 21) due to hydrogenation of the acetyl group in the desired products.
2.1.7. Mechanism
Mechanistic aspects of one-pot reductive amination of carbonyl compounds with amines under molecular hydrogen have been scarcely studied.[Citation15,Citation67,Citation69,Citation82] In one-pot synthesis of secondary amines from aldehydes and amines the corresponding imine is formed in situ and then reduced to amine.[Citation67] In this reaction the acid sites are required for the amine adsorption providing imine formation while hydrogenation of the latter to amine requires the metal sites. Several studies concluded that the Lewis acid sites catalyze imine formation.[Citation61,Citation68] In addition to formation of a secondary amine, several side reactions can also occur, for example furan ring hydrogenation and opening,[Citation67] hydrogenation of aldehyde or ketone.[Citation15,Citation69] It has also been observed that the formed imine from the reaction between 4-fluoroacetophenone and benzylamine can decompose to toluene and 4-methoxy-ethyl-2-amine.[Citation61]
In the reductive amination of a carbonyl compound with a primary amine the first step, imine formation is also crucial (). The forthcoming hydrogenation of an imine requires a suitable metal, a support and temperature, which were discussed in previous Sections. The role of solvent in selective imine formation was recently emphasized.[Citation82] In the first step a carbinol amine is formed after a nucleophilic attack of an amine group on carbon in the C = O bond.[Citation83] Subsequent water elimination, required to form an imine, is dependent on the solvent polarity. Because aldehyde condensation, for example with aniline is an equilibrium reaction, several parameters promote imine formation including low pH, high temperature and the use of polar solvents.
Figure 8. Reaction mechanism for formation of an imine from a carbonyl compound and an amine.[Citation82]
![Figure 8. Reaction mechanism for formation of an imine from a carbonyl compound and an amine.[Citation82]](/cms/asset/1de4c64c-bdf4-48b4-b51e-fb75f1d6fb66/lctr_a_1942689_f0008_b.gif)
2.2. Amination of carbonyl compounds with amines using various hydrogen sources generated in-situ
Several hydrogen sources other than molecular hydrogen have been used including in-situ generated hydrogen from the water-gas shift reaction,[Citation54,Citation75,Citation84] decomposition of formic acid[Citation13,Citation14,Citation65] or NaBH4 (),[Citation12,Citation16,Citation19] and dimethylformamide over ZrO2[Citation85] as well as using iron powder in CO2/H2O.[Citation2] The main drawback in the use of NaBH4 is that it is expensive and forms toxic waste NaBO2 in water according to the following reaction:
Table 5. Secondary amines from aldehyde/ketones and amines using other hydrogen sources than molecular hydrogen. Notation: FA formic acid, SILP supported ionic liquid phase. Notation: Y yield, AM amine, S selectivity
Under excess of water even NaBO2.xH2O can be formed.[Citation86] Recycling of the waste is also challenging. NaBH4 assisted amination of aldehydes and ketones has been demonstrated in literature.[Citation16,Citation19] Typically, the reaction proceeds under mild conditions over solid acids[Citation12,Citation16,Citation19] giving high yields of the desired amine. Even solvent free amination of benzaldehyde with aniline was demonstrated at 25°C in the presence of an equimolar amount of NaBH4 in comparison to ketone giving 95% amine yield over carbon based solid acid (, entry 9).[Citation12,Citation87] This catalyst, prepared by heating naphthalene with sulfuric acid, and subsequent carbonization, has a surface area of 24 m2/gcat and strong acidity, which was 5.3 fold that of Nafion. The CSBA was claimed to be very stable and non-corrosive.[Citation88] Amination of a large variety of aldehydes and ketones with different amines was successfully tested giving mostly very high amine yields under mild conditions in a short reaction time.[Citation12]
An alternative to sodium borohydride is formic acid, an inexpensive reducing agent,[Citation13] which has also been used together with trimethylamine, forming an azeotropic mixture with the latter (, entry 5).[Citation14] During decomposition of formic acid CO2 is predominantly produced, while over some catalysts CO can be also released along with water. Hydrogen can be also generated from the water gas shift reaction involving CO and water.[Citation75] Despite CO toxicity, this option has some benefits in comparison to utilization of molecular hydrogen, as CO is less flammable.[Citation75] Carbon monoxide assisted reductive amination of carbonyl compounds occurred at different pressures and temperatures, which were varied in the range of 5–50 bar and 60–160°C, respectively (, entries 6–8).[Citation54,Citation75,Citation84] Reaction times have been changed from 2.5 to 20 h. Amination of HMF with aniline using CO in the presence of Au/TiO2 where the support had the rutile phase[Citation84] gave more than 99% yield of the desired product (, entry 8). In this process, hydrogen was generated in-situ because of the water gas shift reaction. The highest amounts of hydrogen confirmed by CO/H2O temperature programmed surface reaction techniques were produced over Au/TiO2 catalyst in comparison with Au/CeO2, Au/ZrO2, Au/Al2O3 and Au/SiO2 exhibiting the same gold particle size.
Au/TiO2 catalyst exhibiting gold particles of the size of 2 nm was very active in HMF amination with aniline at 60°C under 20 bar CO (, entry 8).[Citation84] Recyclability of Au/TiO2 was also demonstrated in six consecutive experiments for which the catalyst was washed with acetone and dried under vacuum prior to the next experiment. A very high yield of amine was obtained after the sixth experiment without any leaching of Au.[Citation84] Concentration profiles of imine, amine and the corresponding alcohol, 2,5-bis-(hydroxymethyl)furan are presented in .
Figure 9. Conversion of HMF (■) and concentration profiles of alkyliminefuran (▲), alkylaminofuran (●) and 2,5-bis-(hydroxymethyl)furan (o) in amination of HMF with aniline over Au/TiO2 at 60°C under 20 bar in 1:1 methanol water mixture as a solvent adapted from Ref. [Citation84]. Copyright permission from Royal Society of Chemistry.
![Figure 9. Conversion of HMF (■) and concentration profiles of alkyliminefuran (▲), alkylaminofuran (●) and 2,5-bis-(hydroxymethyl)furan (o) in amination of HMF with aniline over Au/TiO2 at 60°C under 20 bar in 1:1 methanol water mixture as a solvent adapted from Ref. [Citation84]. Copyright permission from Royal Society of Chemistry.](/cms/asset/8105b20b-da34-48e9-aa95-ec57bf79ca1a/lctr_a_1942689_f0009_b.gif)
The undesired alcohol yield was ca. 12%. Furthermore, it was confirmed that under an inert atmosphere, HMF reacted very fast with aniline to form the imine, while in the presence of CO/water the rate limiting step is imine hydrogenation to amine. In Ref. [Citation84], the scale up experiment was also made for amination of HMF with aniline over Au/TiO2 under 20 bar CO at 60°C for 15 h giving 98% yield of amine (, entry 8). The reaction mechanism was proposed including adsorption of CO on metallic gold. Subsequently, CO reacts with water via the water gas shift reaction forming hydrogen and CO2. HMF forms imine by condensation with aniline followed by the formed imine hydrogenation on the gold surface.
In the amination of 4-fluoroacetophenone with p-anisidine high CO pressure of 50 bar was applied at 160°C over different Rh catalysts in THF, which was found to be the best solvent (, entry 7).[Citation75] The highest yield of the desired amine, 82% was obtained over commercial Rh on a carbon matrix with the highest catalyst loading (1 mol%) after 20 h. This method was also successfully demonstrated for different substrates.[Citation75]
Reductive amination of benzaldehyde, 2-pyridinecarboxaldehyde with different substituted aromatic amines mediated by CO2/water/Fe mixture + Pd/C was reported by Ma et al. (, entries 14–22).[Citation2] Two sequential paths for the hydrogen transfer from water to the imine intermediate were proposed. The first step implies a hydrogen transfer from water to Pd(0), leading to formation of highly active Pd hydrides. Then, the generated Pd hydrides are able to hydrogenate the imine intermediate to the amine product, accompanied by regeneration of Pd(0) catalyst ().
Figure 10. Pd/C-catalyzed reductive amination of aldehydes with Fe in CO2/H2O system adapted from Ref. [Citation2] Copyright of Elsevier Ltd.
![Figure 10. Pd/C-catalyzed reductive amination of aldehydes with Fe in CO2/H2O system adapted from Ref. [Citation2] Copyright of Elsevier Ltd.](/cms/asset/51ea3dfc-d282-4b5c-9622-ae30ba705615/lctr_a_1942689_f0010_b.gif)
Isotope-labeling experiments using D2O confirmed that water acts not only as a solvent, but also as a hydrogen source ().[Citation2]
Figure 11. Isotope labeling experiments adapted from Ref. [Citation2] Copyright permission from Elsevier Ltd.
![Figure 11. Isotope labeling experiments adapted from Ref. [Citation2] Copyright permission from Elsevier Ltd.](/cms/asset/e468ea8a-1626-4512-acd2-a08690f2ad65/lctr_a_1942689_f0011_b.gif)
Yields ranging from 11% to 82% for various amines and aniline derivatives were obtained (). The highest yields were seen for aldehydes with methyl- and methoxy- groups at the para-position (, entries 15, 16).[Citation2,Citation47] Reductive amination with aniline or p-anisidine giving heteroaromatic aldehydes was also reasonably efficient (, entry 17, 18, 19). Similar yields were seen for aniline with an electron-donating group (, entry 17) and reductive amination of benzaldehyde with m-toluidine (, entry 20). Much worse results were noticed for 1-naphthylamine at an elevated temperature (, entry 21), while the steric hindrance in 2,6-dimethylaniline was a reason for an even lower yield of 11% yield of the (, entry 22). Nevertheless, the method utilizing CO2 as a reaction medium and promoter has a good potential for further industrial exploitation.
Reductive amination of ketones and aliphatic aldehydes to N,N-dimethyl derivatives using t-ZrO2 catalyst in anhydrous DMF acting both as the amine source and the solvent afforded selectivity to the desired tertiary amines exceeding 99% under mild conditions (, entry 23).[Citation85] This example is beyond the scope of secondary amine synthesis, which is in the core of the current review, but could be considered as an one of the important synthesis methods.
Tamboli et al. reported a silica supported ionic liquid phase (SILP) as a catalyst in reductive amination of cyclohexanone with formic acid and triethyl amine as a hydrogen source.[Citation14] The catalyst based on (1-carboxymethyl-3-methyl-imidazolium bis(trifluoromethylsulfonyl)imide as the ionic liquid) afforded yields of N-cyclohexyl amine derivatives between 58% and 84% at 30°C. The reaction of benzyl amine and cyclohexanone was studied in different solvents giving after 5 h and full conversion the yields of 74%, 81% and 84% for methanol, DMSO and acetonitrile, respectively.
A slight decrease in the amine product yield was observed with the SILP catalyst after three consecutive cycles, which can be related to the ionic liquid leaching.
2.3. Comparison of different methods to synthesize secondary amines
It is instructive to compare different methods for production of secondary amines. For example, N-benzylcyclohexylamine, which is an intermediate for synthesis of pharmaceuticals,[Citation89] can be prepared via reductive amination of cyclohexanone with benzylamine in hydrogen,[Citation10] or alternatively using NaBH4 as a reductant over solid carbon catalyst ().[Citation12] The third method is to use formic acid as a reducing agent together with Au/TiO2,[Citation13] acidic ionic liquid supported on silica[Citation14] or sulfonic acid supported on hydroxyapatite encapsulated γ-Fe2O3 [γ-Fe2O3@HAP-SO3H].[Citation19] Furthermore, N-benzylcyclohexylamine can be prepared by amination of benzaldehyde with cyclohexylamine.[Citation19] These comparative studies are collected in . The results demonstrate that chemical reducing agents require low temperatures and that especially NaBH4 was very active already within 10 min.[Citation12] Higher temperatures and relatively high hydrogen pressures were required for transforming cyclohexanone and benzaldehyde to the corresponding amine,[Citation10,Citation17] mild reaction conditions and a non-metallic catalyst can be used with formic acid.
Table 6. Comparison of different methods of N-benzylcyclohexylamine preparation. Notation: Y yield, AM amine, IM imine.
3. Synthesis of secondary amines from aldehyde and ketones reacting with nitroarenes over heterogeneous catalysts
3.1. Molecular hydrogen as a hydrogen source
3.1.1. Catalyst selection
Recent literature on amination of aldehydes and ketones with nitrocompounds over different catalysts is summarized in .[Citation1,Citation23,Citation28–30,Citation46,Citation47,Citation51,Citation52,Citation56,Citation59–61,Citation65]
Table 7. The highest yields of secondary amines resulted from amination of aldehydes and ketones with nitrocompounds over heterogeneous catalysts using molecular hydrogen. Notation: nN:nAld and nN:nAld denote the molar ratio of nitro compound to aldehyde and ketone, respectively, cAld and cket denote initial concentrations of aldehyde and ketone, respectively.
In amination of aldehydes and ketones with nitro-compounds non acidic catalysts, such as Pd/C, Pd/Al2O3,[Citation23,Citation65] Au/Al2O3[Citation63,Citation92] and Pd/SiO2[Citation47] were shown not to be active, because acid sites are required to transform the formed amine to imine.[Citation72] For example, in amination of benzaldehyde with nitrobenzene over Pd/C and Pd/Al2O3 catalysts benzyl alcohol was the main product.[Citation65] Analogously, 1-phenylethanol was the main product in the reaction between acetophenone and nitrobenzene over Pd/C, Pd/Al2O3 and Pt/Al2O3.[Citation30] On the other hand, bifunctional catalysts promote one-pot amination of aldehydes and ketones with nitrobenzene.[Citation1,Citation23,Citation28–30,Citation46,Citation47,Citation52,Citation59–61,Citation65,Citation70] For example, Pd supported on Lewis acidic MIL-101[Citation30,Citation65] containing Cr3+ ions and Zr containing MOFs, such as amino modified UiO-66 loaded with Ir complexes,[Citation1] were selective toward amination in the reaction of benzaldehyde with nitrobenzene (, entries 2, 6 and 23). For example, a PdAg alloy@MIL-101, reduced in the presence of H2/Ar was very selective (90%) toward amine formation starting from nitrobenzene and benzaldehyde at 99% conversion. On the other hand, Pd/Al2O3 gave lower selectivity, 53% at 67% conversion.[65] Over PdAg alloy@MIL-101 the other products were the corresponding imine (S = 3%) and benzylalcohol (S = 7) (, entry 6).[Citation65] This catalyst contained zirconium aminobenzendicarboxylate MOF Zr(BDC-NH2) formed by contacting a mixture of ZrCl4, acetic acid in DMF with the linker, 2-aminoterephtalic acid.[Citation1] In the subsequent step the prepared Zr6O4(OH)4(BDC-NH2)6 (denoted as UiO-66-NH2) is transformed to the corresponding ligand L and further modified to form the corresponding Ir-modified structure (UiO-66-NH2-LIr).[Citation1] These catalysts possessed in addition to not quantified Lewis acidity[Citation30] also small catalyst particle sizes (Table S2, entries 1, 2), In general MIL-101 is known to exhibit some acidity.[Citation65] Another bimetallic catalyst, Pd2Ag1MIL-101, prepared by the double solvent approach,[Citation93] facilitated quantitative incorporation of the aqueous metal precursor solution into the hydrophilic MIL-101 cavities. This catalyst was selective in amination due to a lower hydrogenation activity of Ag in comparison with Pd. Moreover, presence of Ag also diminished aggregation of Pd nanoparticles.[Citation93]
Some magnetic heterogeneous catalysts, facilitating easy catalyst separation, were found to be active in amination of aldehydes.[Citation23,Citation52,Citation60] In[Citation52] Pd supported on magnetic composite Fe3O4@SiO2/EDAC prepared via formation of covalent bonds between ethylene diamine functionalized cellulose (EDAC) and silica coated Fe3O4 was shown to be a very efficient catalyst in transformation of benzaldehyde and its derivatives to secondary amines under mild conditions at 25°C and 1 bar hydrogen in a ethanol-water mixture (, entry 5, Table S2 entry 5).[Citation52] The reaction time was also quite short over Pd-Fe3O4/SiO2 (ethylene diamine), 2.5 h[Citation52] in comparison to Pd/Fe3O4/C for which 8 h was required (, entry 10, Table S2, entry 10).[Citation90] Moreover, separation and recycle was reported to be facile. Analogously Pd/Fe3O4-NH2/starch (Pd@Fe3O4-NH2-starch) catalyst with the average diameter of Pd particles of 8.4 nm was efficient in benzaldehyde amination with nitrobenzene giving 93% yield at 25°C in the ethanol-water mixture under hydrogen atmosphere already after 2 h (, entry 9, Table S2, entry 9).[Citation60] This catalyst was prepared by contacting iron chloride hexahydrate in sodium acetate and 1,6-hexanamine in ethylene glycol as a solvent to form magnetic Fe2O3-NH2 nanoparticles, which were further functionalized with starch chloride in the presence of trimethylamine in chloroform. Such method facilitated formation of metallic Pd particles inside starch-amine coated magnetic Fe2O3.[Citation60] Magnetic hollow mesoporous spheres composed of Fe3O4 modified polystyrene, after calcination were transformed to hollow spheres followed by loading with Pd. The catalyst prepared in this way was active giving 94.2% yield of benzylaniline at 8 h under hydrogen atmosphere at 25°C (, entry 11, Table S2, entry 11).[Citation23]
The role of Ag in Pd-Ag alloys in amination of benzaldehyde with nitrobenzene is to decrease the hydrogenation ability of Pd and to suppress the alcohol formation, while MIL-101 metal organic framework provides the Lewis acidity (, entry 6). Amination of a ketone, cyclohexanone, with nitrobenzene was also successful giving 83% yield at 25°C under 1 bar hydrogen over the same Pd@Fe3O4-NH2-starch catalyst mentioned above which exhibited 8.4 nm Pd particles (, entry 24, Table S2, entry 9).[Citation60]
Interestingly nitrone was the main product in reductive amination of 4-methylfurfural with nitrocyclohexane over Au/TiO2 in ethanol at 60°C under 10 bar hydrogen.[Citation94] Only 3% of N-[(5-methylfuran-2-yl)methyl]cyclohexamine was formed after 14 h apparently because dehydration of nitrone formed in the reaction between N-hydroxyaniline (hydroxylamine) and benzaldehyde was retarded ().
Figure 12. Reductive amination of 4-methylfurfural with nitrocyclohexane over Au/TiO2 adapted from Ref. [Citation94] Copyright permission from Elsevier Ltd.
![Figure 12. Reductive amination of 4-methylfurfural with nitrocyclohexane over Au/TiO2 adapted from Ref. [Citation94] Copyright permission from Elsevier Ltd.](/cms/asset/a618b777-013d-4c90-9631-14132498c46a/lctr_a_1942689_f0012_b.gif)
Transition metal catalysts, such as Co3O4 encapsulated by nitrogen-enriched graphene exhibiting a core-shell structure (denoted as Co3O4/NGrC),[Citation29] cobalt nanoparticles supported on N-doped mesoporous carbon (denoted as Co/mCN),[Citation61] and Fe2O3 supported on nitrogen containing carbon (denoted as Fe2O3/NGrC)[Citation28] have also been applied as catalysts in reductive amination of aldehydes and ketones with nitro compounds (, entries 12–14, 28). For example, Co3O4 supported on nitrogen modified graphene gave 95% yield of N-benzyl aniline at 110°C under 50 bar of hydrogen (, entry 12),[Citation29] thus requiring a high hydrogen pressure. This catalyst exhibited metallic cobalt inside Co3O4 supported in nitrogen enriched graphene layers, mesopores and both small and large metal particles (Table S2, entry 12). Analogously to Co3O4 supported on nitrogen doped graphite, Fe3O4 supported on nitrogen doped graphite exhibited also a core-shell structure bearing superparamagnetic Fe2O3 particles as confirmed by Mössbauer spectroscopy (Table S2, entry 14).[Citation28]
3.1.2. Effect of reaction conditions in amination of aldehyde and ketones with nitroarenes over heterogeneous catalysts and catalyst recycling
Noble metal catalysts have been very active in reductive amination of aldehydes with nitro-compounds already at relatively low temperatures.[Citation59,Citation63] For example, an optimum reaction temperature, 60°C was obtained in amination of n-heptaldehyde with 3-nitrostyrene over Au/Al2O3 catalyst in a flow reactor under 50 bar hydrogen for production of the corresponding unsaturated amine giving 74% yield of the desired amine, N-(3-vinylbenzyl)-amine,[Citation63] while at a higher temperature the C = C double bond in the side chain was also hydrogenated. Under optimum conditions the amount of the hydrogenated side chain product was 8.5%.[Citation63] Benzaldehyde amination with nitrobenzene was successfully performed at 25°C giving 92% amine yield[Citation59] in ethanol over Pd nanoparticles supported in Cherry tree gum in 20 min (, entry 8). At higher temperatures, the yield of amine was reported to decrease.[Citation59] Interestingly, this catalyst was inactive for amination of acetophenone with nitrobenzene under mild conditions, i.e. at 25°C under 1 bar hydrogen (, entry 22),[Citation59] while Pd@MIL-101[Citation30] and Pd2Ag1 alloy supported on metal organic framework MIL-101[Citation65] worked well at 110°C under 5 bar hydrogen (, entry 25, 26). This result indicates that reductive amination of ketones requires more harsh conditions than aldehyde amination.
Over transition metal catalysts, higher reaction temperatures have been applied even for amination of aldehydes[Citation28,Citation61] in comparison to noble metal catalysts. The highest studied temperature, 150°C, was found to be the best one for amination of benzaldehyde with nitrobenzene over Co supported on nitrogen doped carbon catalyst under 10 bar hydrogen in ethanol ().[Citation62] Analogously, the highest studied temperature, 150°C was the best one in the same reaction over Fe2O3 supported on nitrogen doped graphite under 50 bar hydrogen giving 46% yield of N-benzylaniline in 30 h ().[Citation28] It can also be noted that hydrogenation of imine proceeds more slowly at lower temperatures. For amination of ketones very harsh conditions, 160°C and 50 bar were required for Co3O4 nitrogen doped graphitic carbon (denoted as NGr@C) (Table S2 entry 11).[Citation29]
Figure 13. Effect of temperature in reductive amination of benzaldehyde with nitrobenzene over Co supported on mesoporous nitrogen doped carbon (square) adapted from Ref. [Citation62] and over Fe2O3 supported on nitrogen doped graphitic carbon (circle) adapted from Ref. [Citation28] Notation: open symbol imine, solid symbol amine. Copyright permissions from John Wiley & Sons and from Elsevier Ltd.
![Figure 13. Effect of temperature in reductive amination of benzaldehyde with nitrobenzene over Co supported on mesoporous nitrogen doped carbon (square) adapted from Ref. [Citation62] and over Fe2O3 supported on nitrogen doped graphitic carbon (circle) adapted from Ref. [Citation28] Notation: open symbol imine, solid symbol amine. Copyright permissions from John Wiley & Sons and from Elsevier Ltd.](/cms/asset/e14a402e-8299-45bc-96b7-9383f0927953/lctr_a_1942689_f0013_b.gif)
3.1.2.1. Effect of hydrogen pressure
Low hydrogen pressure has been applied in reductive amination of aldehydes with nitrocompounds over Pd supported catalysts (, entries 3–11),[Citation23,Citation46,Citation47,Citation51,Citation52,Citation56,Citation59,Citation60,Citation65] whereas transition metal catalysts operated under harsher conditions (, entries 12–14, 28).[Citation28,Citation29,Citation62] Higher hydrogen pressures are beneficial for hydrogenation of the formed imine in amination of aldehydes with nitro-compounds over Cu/Al2O3 and Co supported on nitrogen doped carbon,[Citation29,Citation62] while a low hydrogen pressure retarded imine hydrogenation over Co supported on mesoporous N-doped carbon (denoted as Co/m-CN) in the amination of benzaldehyde with nitrobenzene.[Citation62] This catalyst was prepared in one-pot synthesis pyrolyzing cobalt nitrate hexahydrate, melamine and polyacrylonitrile under inert atmosphere.[Citation62] Higher hydrogen pressure, 70 bar, promoted amine formation in the same reaction at 160°C over Fe2O3 supported on nitrogen doped graphite (NGr) in THF water mixture.[Citation28] It was also observed that with a lower hydrogen pressure the formed aniline in n-heptanal amination with p-nitrotoluene over Cu/Al2O3 was not reacting further and 50 bar at 115°C was required to maximize the amine yield of the desired amine over this catalyst.[Citation72] The yield of amine with 9 wt% Cu/Al2O3 was 85% and 15% n-heptanol was also formed.[Citation72] In amination of ketones even over Pd catalysts in some cases higher than atmospheric pressure was required (, entries 23, 26).[Citation30,Citation65]
3.1.2.2. Effect of solvent
The effect of solvents has been intensively studied in amination of carbonyl compounds with nitro compounds.[Citation28,Citation29,Citation51,Citation59–61] Relatively polar solvents gave high yields of secondary amines in aldehyde amination with nitro compounds. For example, the best solvent in the amination of benzaldehyde with nitrobenzene was a mixture of ethanol–water (3:1) over inorganic/organic Pd loaded amine functionalized composite catalyst denoted as Pd-Fe3O4-SiO2-EDAC.[Citation52] This catalyst was prepared via coprecipitation FeCl2 and FeCl3 to form Fe3O4 nanoparticles followed by formation of silica layer on the surface of Fe3O4 using a sol–gel process. Thereafter, cellulose modified by 1,2-ethylene diamine was covalently bonded with silica coated Fe3O4 particles. The highest yield of N-benzylaniline (above 99%) was obtained in ethanol over Co supported on nitrogen doped carbon (denoted as CoCN) catalysts under 10 bar hydrogen at 150°C,[Citation62] while at 130°C more imine was also observed (6%). Furthermore, a THF–water mixture was a suitable solvent for production of N-benzylaniline over Co3O4 supported on nitrogen modified graphite (NGr) catalyst at 110°C under 50 bar hydrogen in 24 h giving 95% amine yield.[Citation29] It was also observed that the presence of more water in the dioxane–water solvent mixture in the amination of benzaldehyde with nitrobenzene lowered the yield of the desired amine by inhibiting the further imine hydrogenation over Co3O4/NGr catalyst at 110°C under 50 bar hydrogen.[Citation29] It was stated that catalyst poisoning can also be inhibited via applying more hydrophobic solvents. Triethylamine and water were not appropriate solvents over Co supported on nitrogen doped mesoporous carbon (mCN-900, pyrolyzed at 900°C) giving large amounts of unreacted imine and aniline as products in reductive amination of benzaldehyde with nitrobenzene.[Citation62]
3.1.2.3. Molar feed ratio
Changes in the molar ratio between the reactants, i.e. nitroarene/aldehyde, have been scarcely studied.[Citation28–30,Citation51] Typically a slight excess of nitroarene was used in amination of benzaldehyde.[Citation51] It was observed in Ref. [Citation28] that an excess of benzaldehyde in its amination with nitrobenzene causes a slower reaction rate as well as formation of a higher amount of benzylalcohol over Fe2O3 supported on nitrogen modified graphite (NGr) catalyst[Citation28] and over Co3O4 supported on nitrogen doped graphitic carbon (NGr@C).[Citation29] In addition with a three-fold excess of nitrobenzene N,N-dibenzylaniline (see ) was formed over Pd-MIL-101 catalyst.[Citation30]
3.1.2.4. Catalyst recyclability
Several catalysts exhibited very good recyclability in amination of benzaldehyde with nitrobenzene such as Pd/polymer,[Citation46] PdCu supported on β-cyclodextrin,[Citation66] Pd/Fe3O4,[Citation51] Pd supported on Fe3O4 carbon composite catalyst,[Citation90] Pd@MIL-101,[Citation30] Pd2Ag1@MIL-101,[Citation65] Pd/Fe2O3@C,[Citation90] Fe2O3 supported on nitrogen doped graphitic carbon composite catalyst (NGr@C).[Citation28]
Metal leaching can be a problem in the liquid phase reductive aminations, for example in HMF amination in ethanol over Pd/C, 76% of Pd was leached out from the commercial catalyst after 6 h.[Citation67] In addition, Pd leaching occurred during HMF amination also from Pd/UiO-67 (ca. 65% of Pd was leached).[Citation68] On the other hand, no metal leaching was reported from iron – fenantroline complex immobilized on a carbon support, [Citation28] from a polymer supported Pd catalyst,[Citation46] and from Pd supported on Fe3O4-carbon composite,[Citation90] However, the presence of polymer retarded catalyst deactivation of Pd supported polyparaphenylene diamine modified metal organic framework UiO-67 in HMF amination with aniline in ethanol, at 50°C under 5 bar hydrogen[Citation68] and ca. 95% of Pd remaining in the catalyst.
In general, aggregation of metal particles can be one of reasons for the activity decline.[Citation30] Under mild conditions, no aggregation of Pd supported on gum acacia occurred. This catalyst exhibited the same metal particle size of ca. 9 nm after the fifth reaction cycle in amination of benzaldehyde with nitrobenzene at 25°C under 1 bar hydrogen.[Citation47] The yield of N-benzylaniline, however, decreased slightly from the fresh catalyst giving 88% to 85% in the fifth cycle. On the other hand, a minor increase of the Pd particle size was observed in Pd@MIL-101 when it was used in benzaldehyde amination with nitrobenzene at 110°C under 5 bar hydrogen.[Citation30]
A very promising catalyst exhibiting excellent recyclability in reductive amination of benzaldehyde with nitrobenzene was Pd/Fe3O4@C[Citation90] prepared by solvothermal synthesis (Table S2, entry 10). The yield of secondary amine decreased only form 92% in the first cycle to 88% in the sixth cycle while no Pd leaching was observed. Furthermore, it was stated that the catalyst synthesis is simple and convenient. In addition, this solvothermal method to synthesize catalyst is attractive for large-scale industrial synthesis. On the other hand, it was shown in several studies[Citation28,Citation29,Citation51] that the yield of N-benzylaniline is decreasing during recycling. Thus, the yield of benzylaniline decreased from 92% to 87% in the fourth cycle with Pd/Fe3O4 catalyst in amination of benzaldehyde with nitrobenzene under hydrogen atmosphere in ethanol at 25°C[Citation51] when the catalyst was washed with ethanol between experiments. For Fe2O3 supported on nitrogen modified graphitic carbon the yield of N-benzylaniline decreased from 63% to 55% from the first to the fifth cycle at 170°C under 50 bar hydrogen during 30 h.[Citation28] Analogously for Co3O4 supported on nitrogen modified graphite – carbon composite (NGr@C) catalyst used in amination of benzaldehyde with nitrobenzene the yield of the desired amine decreased after the first use from 95% to 60% for the sixth recycle,[Citation29] even if no leaching neither catalyst structural changes were detected. The reason for catalyst deactivation might be accumulation of carbonaceous species on the catalyst surface.
3.1.3. Continuous amination of carbonyl compounds with nitroarenes
Continuous amination of carbonyl compounds was demonstrated over Ag, Au and Cu catalysts,[Citation63,Citation72,Citation92] which were relatively stable.[Citation63,Citation71–73,Citation92] The amine yield in the reaction between n-heptaldehyde and benzylamine at 80°C over Au/Al2O3 decreased with time-on-stream from 95% to 90% in 140 min due to carbon deposition on the catalyst surface. It was, however, reported that the gold particle size remained the same.[Citation63] Analogously a slight catalyst deactivation occurred in the amination of heptanal with nitrobenzene at 100°C under 30 bar hydrogen for Ag/Al2O3 catalyst. In this case the carbonaceous species were removed by regenerating the catalyst in air at 330°C for 20 h almost restoring the catalyst activity.[Citation92] The authors compared the space time yields of the amine obtained over Ag/Al2O3[Citation92] and Au/Al2O3[Citation63] and concluded that the gold catalyst was ca. twofold more efficient. The yield of the desired amine over Ag/Al2O3 under 30 bar hydrogen at 100°C in toluene was 85% with the imine yield of 9%.[Citation93]
Continuous amination of n-heptanal with p-nitrotoluene was also successfully demonstrated over Cu/Al2O3 catalyst under 50 bar hydrogen at 115°C in toluene as a solvent giving 82% yield of the desired amine and 18% aniline.[Citation73] On the other hand, THF and isopropanol caused severe deactivation of Cu/Al2O3 completely retarding the reaction.[Citation73] The yield of amine decreased slightly with increasing time-on-stream due to deposition of carbonaceous products on the catalyst surface. The catalyst could, however, be regenerated after washing with toluene/2-propanol and treatment in air at 330°C for 2 h.[Citation73] The use of methanol as a solvent produced more aniline as an intermediate over Au/Al2O3, moreover, as reported in Ref. [Citation63], aldehydes are easily hydrogenated in alcohols. It was also pointed out that the space time yield of the desired amine was only 3.3 times lower over Cu/Al2O3 in comparison with a more expensive Au/Al2O3 in reductive amination of n-heptanal with nitrobenzene.[Citation63,Citation73]
3.1.4. Kinetics and modeling of amination of carbonyl compounds with nitroarenes
Kinetics of aldehydes and ketones amination with nitrocompounds has been very scarcely studied.[Citation30,Citation70] Concentration profiles as a function of time were shown, for example in,[Citation70] for amination of benzaldehyde with nitrobenzene, which was performed over a Pt nanowire catalyst at 100°C under 1 bar hydrogen. Experimental data corresponded to a typical consecutive reaction. Already after 1 h the main product was N-benzylaniline. The second major product was N-benzylidineaniline, which reacted further after a prolonged reaction time. Over this catalyst also traces of benzyl alcohol were formed originating from benzaldehyde hydrogenation.
A simple kinetic model was developed also for benzaldehyde amination with nitrobenzene over several catalysts, such as Au@TiO2, MIL-101-SI-Pt, MIL-101-SI-Pd modified with salicylaldehyde (SI) (preparation method for MIL-101-SI-Pt catalyst is given in Table S2, entry 2) as well as Pd loaded on molybdophoshoric acid modified SiO2 at 110°C under 5 bar hydrogen. In Ref. [Citation30], a consecutive reaction network involving nitrobenzene transformations to imine and further hydrogenation to amine was considered while nitrobenzene hydrogenation to aniline and its further reaction to imine were of minor importance because the aniline concentration in the reaction mixture was very low. The results revealed that the slowest reaction varied depending on the catalyst type. For the best catalyst MIL-101-SI-Pd such reaction was nitrobenzene hydrogenation, while for MIL-101-SI-Pt imine hydrogenation was slower. In addition, it was stated that the slowest process in one-pot amination of benzaldehyde with nitrobenzene is imine hydrogenation over Co3O4 supported on nitrogen modified graphite (denoted as Co3O NGr@C) at 110°C under 50 bar hydrogen.[Citation29]
These results show[Citation30,Citation70] that there is a clear need to further study reaction kinetics in a systematic way considering a potential influence of hydrogen pressure, temperature and initial reactant concentration, as such data are largely missing.
3.1.5. Reaction mechanism for amination of carbonyl compounds with nitroarenes
The mechanism of carbonyl compounds amination has been quite intensively studied.[Citation72,Citation82] It was proposed in Ref. [Citation72] that the nitro group is first adsorbed on the active metal sites followed by hydrogenation. Thereafter, the formed amine reacts with an aldehyde giving the imine,[Citation72] which in turn was hydrogenated on the metal sites. It should, however, be pointed out that the amine can also react with aldehyde in the liquid phase in the absence of any catalyst. The major side reaction in reductive amination of carbonyl compounds is alcohol formation from aldehydes and ketones,[Citation70] which is typically inhibited in the presence of large amounts of amine formed in the first step.[Citation72] Mechanistic studies were performed for benzaldehyde amination with nitrobenzene.[Citation70] The imine, N-benzylideneaniline, was used as a reactant in the presence of 1 bar hydrogen at 110°C over Pt nanowire, giving only ca. 5% yield of N-benzylaniline after 24 h. Furthermore, the authors using in-situ infrared spectroscopy demonstrated that nitrobenzene was reduced in the first step to nitrosobenzene, which in turn forms phenyl(phenylamino)methanol from the reaction between benzaldehyde giving a dihydroxy intermediate. This intermediate is dehydrated to N-benzylaniline. It was also confirmed that phenyl(phenylamino)methanol is easier to be dehydrated to N-benzylaniline than to N-benzylideneaniline.[Citation70] According to DFT calculations, the bonding energy of N-H is higher than that for C-OH explaining why N-benzylaniline is formed easier than N-benzylideneaniline.
The reaction mechanism for one-pot reductive amination of a nitroarene under hydrogen atmosphere with an aldehyde was recently proposed in Ref. [Citation82]. In the first step nitroarene is hydrogenated to the corresponding amine (intermediate I) followed by its condensation with an aldehyde to an imine (intermediate II) (). The formed imine is subsequently hydrogenated giving a secondary amine. In addition, when using an excess of the aldehyde, the tertiary amine (by-product 3ʹ) can be formed. On the other hand, with an excess of amine the aminal by-product (III) can be formed. Furthermore, hydrogenation of an aldehyde (reactant 2) can occur generating the corresponding alcohol (by-product 2ʹ). In addition even dehydration of the alcohol has been observed resulting in the corresponding hydrocarbon (by-product 2ʹ).
Figure 14. Mechanism for one-pot reductive amination of nitroarenes with aldehydes.[Citation82].
![Figure 14. Mechanism for one-pot reductive amination of nitroarenes with aldehydes.[Citation82].](/cms/asset/862c8d4c-4533-44ad-9d53-50f3d413e3a4/lctr_a_1942689_f0014_oc.jpg)
3.2. Amination of aldehydes and ketones with nitroarenes over heterogeneous catalysts using alternative hydrogen sources
As alternative hydrogen sources NaBH4,[Citation50] CO-H2O[Citation54,Citation95] and formic acid[Citation49,Citation55,Citation56] have been used for amination of nitroarenes over heterogeneous catalysts. When formic acid is used as a hydrogen source, typically an excess of formic acid is required.[Citation49] Benzaldehyde amination with nitrobenzene was demonstrated using 10 fold excess of formic acid giving 66% yield of the desired amine at 60°C in 6 h over Au/SiO2-NH2.[Citation49] However, even a higher yield, 93% was obtained in THF using a quinuclidine base as an additive together with Au/SiO2-NH2 at 80°C with 15 fold excess of formic acid in 8 h (, entry 1). This catalyst was also very stable without any leaching.[Citation49] It was stated that when amine is adsorbed on the gold surface, the energy barrier to hydrogen heterolytic dissociation decreased.[Citation49] Application of NaBH4 allowed to synthesize benzylamine over Ni/H-mZSM-5 catalyst with high 95% yield at room temperature.[Citation50] Higher temperatures and longer reaction times were required for transition Co metal catalysts to transform benzaldehyde to N-benzylaniline (, entries 3–6, ).[Citation53,Citation54,Citation56] CO/water assisted one-pot reductive amination by transfer hydrogenation was also demonstrated over Co/N-C calcined at 600°C.[Citation90] The highest yield of N-benzylideneaniline was ca. 90% at 170°C under 30 bar CO. In this system hydrogen is provided by the water gas shift reaction: CO+H2O CO2 + H2. The proposed reaction mechanism shown in illustrates formation of as-formed proton (N-H+) and a hydride Co-H−, which are active species in hydrogenation of the nitro group. Furthermore, higher reaction temperatures favored imine hydrogenation to the corresponding amine as in the case of molecular hydrogen as a hydrogen source.
Figure 15. Effect of temperature in reductive amination of benzaldehyde with nitrobenzene over Co supported on nitrogen doped carbon (CN-600, pyrolyzed at 600°C) (ball) adapted from Ref. [Citation53] and over Co supported on nitrogen doped carbon (CN-800, pyrolyzed at 800°C) (rectangular) adapted from Ref. [Citation56] in the presence of formic acid. Notation: open symbol: imine, solid symbol: amine. Copyright permissions from Elsevier Ltd and from American Chemical Society.
![Figure 15. Effect of temperature in reductive amination of benzaldehyde with nitrobenzene over Co supported on nitrogen doped carbon (CN-600, pyrolyzed at 600°C) (ball) adapted from Ref. [Citation53] and over Co supported on nitrogen doped carbon (CN-800, pyrolyzed at 800°C) (rectangular) adapted from Ref. [Citation56] in the presence of formic acid. Notation: open symbol: imine, solid symbol: amine. Copyright permissions from Elsevier Ltd and from American Chemical Society.](/cms/asset/387ab094-2e2c-4e40-8546-f8d89caf4e71/lctr_a_1942689_f0015_b.gif)
Figure 16. The reaction mechanism for one-pot reductive amination of nitrobenzene with benzaldehyde using the CO/H2O assisted system.[Citation95] Copyright Elsevier Ltd.
![Figure 16. The reaction mechanism for one-pot reductive amination of nitrobenzene with benzaldehyde using the CO/H2O assisted system.[Citation95] Copyright Elsevier Ltd.](/cms/asset/32342116-e21a-41da-bf7d-b169fc51281d/lctr_a_1942689_f0016_oc.jpg)
Table 8. Hydrogen sources other than molecular hydrogen used in amination of benzaldehyde with nitrobenzene over heterogeneous catalysts. Notation: N nitro compound, BA benzaldehyde.
An acid leached mesoporous Co catalyst supported on nitrogen doped carbon (Co@CN) ( entry 3) was an active and selective catalyst in formic acid assisted amination of benzaldehyde[Citation56] even in the absence of a base. Formic acid was used 4.5 fold excess in comparison to aldehyde at 150°C in THF over Co supported on nitrogen doped carbon.[Citation56] The effect of the molar ratio of benzaldehyde to nitrobenzene was also studied in benzaldehyde amination in the presence of formic acid over Co supported on nitrogen doped carbon (CN-800, pyrolyzed at 800°C),[Citation56] showing that the yield of the desired amine decreased with decreasing benzaldehyde to nitrobenzene ratio. A rather high reaction temperature, 190°C, was required, since at lower temperatures the imine was the main product indicating that hydrogenation of C = N requires a higher temperature than of the nitro group.[Citation56]
Nickel nanoparticles supported on the proton form of ZSM-5 zeolite (Ni/H-mZSM-5) with a microporous/mesoporous hierarchical structure were used in one-pot reductive amination of aldehydes with nitroarenes comprising various electron-donating and electron-withdrawing groups in the presence of NaBH4 as a mild reducing agent ().[Citation50]
Table 9. One-pot reductive amination of aldehydes with nitroarenes over Ni/H-mZSM-5 with NaBH4 as a reducing agent a.[Citation50].
Presence of an electron-withdrawing group in the nitro aromatic ring decreasing the electron density at the nitro group was beneficial from the kinetic viewpoint (, entry 6), whereas an opposite effect was seen for electron-donating groups (, entries 7 and 8) which can be related to different ability of accepting the hydride ions. The latter increases at a lower electron density at the nitro groups. On the other hand, higher reaction rates were obtained for the compounds containing the electron-donating groups in the benzaldehyde ring (, entries 2–5). In fact, the electron-donating groups stabilize the protonated carbonyl bond intermediate, which is beneficial for reaction kinetics. A study of reusability of the Ni/H-mZSM-5 catalyst demonstrated very low Ni leaching (ca. 3%) and the preserving catalyst structure according to XRD. The recycled catalyst washed with water (10 mL) and ethanol (3 mL × 5 mL) could be reused six times.[Citation50] Catalyst recycling was also investigated in formic acid assisted amination of benzaldehyde.[Citation49,Citation53] No gold leaching was observed from Au/SiO2-NH2 and the hot filtration test indicated that the reaction occurred only over the heterogeneous catalyst.[Citation49] The yield of the desired amine decreased only slightly due to a minor agglomeration of the gold particles.[Citation49] Recycling of Co supported on nitrogen doped carbon (denoted as Co-CN-600-AT, which was acid leached and pyrolyzed at 600°C) was successfully demonstrated in benzaldehyde amination at 190°C under 10 bar nitrogen in the presence of three-fold excess of formic acid.[Citation53] In Ref. [Citation53], separation of Co-CN-600-AT with a magnet and the catalyst reuse after washing it with water and ethanol followed by vacuum drying at 60°C confirmed absence of the metal leaching and an activity decrease.
Kinetic analysis of the formic acid assisted amination of benzaldehyde with aniline at 150°C in THF showed consecutive formation of imine followed by its hydrogenation to the amine as expected ().[Citation56] In Co supported on nitrogen doped carbon catalyzed amination of benzaldehyde with aniline using six fold excess of formic acid with respect to benzaldehyde at a high temperature promoted formation of amine instead of the imine.[Citation53] The authors[Citation53] also observed that N-benzylidene aniline can be reversibly transformed to benzaldehyde and aniline in a mixture of formic acid–water and especially that the yield of benzyl aniline was suppressed at lower temperatures due to this reaction.
Figure 17. Concentration profiles for the reactant and products in benzaldehyde amination with nitrobenzene over Co supported on nitrogen doped carbon in the presence of formic acid at 150°C in THF as a solvent, adapted from.[Citation56] Notation: Molar percentage of nitrobenzene (■), N-benzylideneaniline (●), aniline (▲) and N-benzylaniline (o). Copyright permission from Elsevier Ltd.
![Figure 17. Concentration profiles for the reactant and products in benzaldehyde amination with nitrobenzene over Co supported on nitrogen doped carbon in the presence of formic acid at 150°C in THF as a solvent, adapted from.[Citation56] Notation: Molar percentage of nitrobenzene (■), N-benzylideneaniline (●), aniline (▲) and N-benzylaniline (o). Copyright permission from Elsevier Ltd.](/cms/asset/82e13666-08ce-4ec1-b728-b24d9f284861/lctr_a_1942689_f0017_b.gif)
The reaction mechanism for formic acid assisted transfer hydrogenation was also discussed. The first step is heterolytic splitting of formic acid to H+ adsorbed on N and Co-HCOO−. Presence of nitrogen in the catalyst is beneficial for the interactions of formate anions with Co nanoparticles. It was confirmed by utilization of deuterated formic acid, DCOOD, that heterolytic cleavage of hydrogen is possible with D+ and D− adsorbing respectively on N and Co.[Citation56] Furthermore, it was stated that formation of Co-HCOO− species is the rate limiting step. In the recycling tests of Co supported on nitrogen modified carbon neither aggregation of the metal nor leaching were observed.
In CO-assisted reductive amination of benzaldehyde with nitrobenzene[95] and 4-methoxynitrobenzene,[Citation54] the effect of CO pressure was also systematically studied (, , entries 4, 5) indicating that a higher CO pressure enhanced formation of amine, while in benzaldehyde amination with 4-methoxynitrobenzene the yield of the corresponding tertiary amine increased. In Ref. [Citation54], the highest yield of the desired secondary amine was obtained after 24 h reaction at 5 bar CO at 120°C. It was also confirmed that CO assisted reductive amination did not proceed in THF as the water gas shift reaction generating hydrogen requires water.
Figure 18. Effect of CO pressure in reductive amination of benzaldehyde with a) nitrobenzene over Co supported on nitrogen modified carbon catalyst in water at 170°C after 10 h adapted from[95] notation: solid symbol – amine, open symbol – imine and b) 4-methoxynitrobenzene over Co2Rh2/C at 120°C after 12 h in THF and a small amount of water adapted from,[Citation54] notation: solid symbol – amine, open symbol – tertiary amine, a and b denote the yield of secondary amine and tertiary amine, respectively after 24 h. Copyright permissions from Elsevier Ltd and from American Chemical Society.
![Figure 18. Effect of CO pressure in reductive amination of benzaldehyde with a) nitrobenzene over Co supported on nitrogen modified carbon catalyst in water at 170°C after 10 h adapted from[95] notation: solid symbol – amine, open symbol – imine and b) 4-methoxynitrobenzene over Co2Rh2/C at 120°C after 12 h in THF and a small amount of water adapted from,[Citation54] notation: solid symbol – amine, open symbol – tertiary amine, a and b denote the yield of secondary amine and tertiary amine, respectively after 24 h. Copyright permissions from Elsevier Ltd and from American Chemical Society.](/cms/asset/721965b6-1526-4016-aa11-0012862b2d79/lctr_a_1942689_f0018_b.gif)
Palladium-catalyzed chemoselective reduction and reductive amination of nitroarenes with water as a hydrogen source mediated by diboronic acid was reported in Ref. [Citation48]. The authors proposed that interactions of Pd with diboronic acid B2(OH)4 resulted in formation of a Pd[B(OH)2]2 complex. Then water was coordinated to one of the Lewis acidic boron atoms of Pd[B(OH)2]2 through an oxygen atom giving followed by a further release of the Pd-hydride complex
and boric acid B(OH)3. This hydride complex (HO)2B-Pd-H in essence plays a role of the hydrogenating agent allowing, first, hydrogenation of a nitroaromatic compound into the corresponding primary amine, and, second, hydrogenation of the giving finally the desired secondary amine. The role of water and diboronic acid was reported to be in recovering palladium and provide formation of (HO)2B-Pd-H complex required for the catalytic cycle.[Citation48]
4. Future outlook
Based on the literature analysis, it can be concluded that there is still a lack of experimental data preventing in depth understanding of the role of the active sites in the catalyst and correlation of the catalytic properties with its performance. In most of the papers, only the yield of the desired amine is given,[Citation1,Citation11–14,Citation17,Citation46,Citation47,Citation51,Citation52,Citation66,Citation68,Citation75] and in several studies the catalyst reuse has been addressed.[Citation11,Citation17,Citation24,Citation28,Citation30,Citation51,Citation65–67,Citation69,Citation90] However, rather few papers have addressed formation of side products,[Citation10,Citation24–26,Citation61,Citation68,Citation84] the reaction mechanism,[Citation82,Citation95] presented kinetic analysis[Citation56,Citation62,Citation68,Citation84,Citation95] or did kinetic modeling.[Citation61] Such studies could, however, give valuable information for the development of reductive amination toward industrial applications. In addition, continuous mode of operation is an attractive option to elucidate catalyst stability and industrial feasibility of the process.[Citation24,Citation63,Citation71,Citation72,Citation74,Citation92] Typically rather diluted reaction mixtures have been used. Subsequently very seldom data are available on amination of more concentrated reaction mixtures[Citation84] and the effect of the initial reactant concentration is addressed.[Citation69] The nature of active sites in the catalyst for reductive amination of carbonyl compounds is typically not adequately addressed because catalyst acidity[Citation10,Citation65] or basicity[Citation10] have been determined very seldom.
Furthermore, a systematic physico-chemical approach has been very scarcely applied in reductive amination of carbonyl compounds. More research is required to systematically study the effect of the initial reactant concentrations[Citation69] and pressure[Citation11,Citation17,Citation68] and to reveal reaction orders with respect to the reactants as well as to determine activation energies for different reaction steps in reductive amination of carbonyl compounds.
5. Conclusions
One-pot reductive amination of aldehydes and ketones for synthesis of secondary amines, important intermediates for production of pharmaceuticals, has been reported for several different types of aldehyde and ketones with high yields obtained in many cases. The feedstock, aldehydes and ketones, are inexpensive and very abundant from biorefinery processes, while nitro compounds are obtained by nitration of benzene. Nitro compounds can also be reduced in situ to the corresponding amines, which then react with aldehydes or ketones in one-pot synthesis of secondary amines. The analysis of literature showed clearly that reductive amination over heterogeneous catalysts is a promising method giving more than 90% yields of secondary amines. For example, in reductive amination of benzaldehyde with cyclohexylamine 94% yield of the secondary amine was obtained at 80°C under 30 bar hydrogen in water and the catalyst recycling was demonstrated in four cycles with conversion slightly decreasing form 93% to 86%. Moreover, reductive amination of ketones has been also demonstrated.
The effect of the aldehyde/ketone structure on the yield of secondary amine has been intensively studied in the literature. The most important catalyst properties are the metal nature and its particle size, loading, support acidity and hydrophobicity, while among the reaction parameter, the source of hydrogen, hydrogen pressure, temperature and solvent are important factors. Both noble and transition metal catalysts have been used in the presence of hydrogen. With NaBH4 as a reducing agent also acidic catalyst in the absence of a metal could be applied, because no hydrogen dissociation occurring on the metal is required. For example, non acidic metal catalysts promote the undesired hydrogenation of aldehydes/ketones instead of forming the imines and in the second step the desired amines.
Industrial feasibility of reductive amination processes needs to be addressed. Although continuous reductive amination has already been demonstrated, the view toward more engineering approach, such as systematic kinetic studies, also with more concentrated feeds and with a complete product analysis are required. In the next phase these systematic results should be combined with kinetic analysis, modeling and proper catalyst characterization including also quantitative acidity/basicity determination.
Acknowledgment
IS is grateful for the support from the Ministry of Science and Higher Education of the Russian Federation under the governmental order for Boreskov Institute of Catalysis (project AAAA-A21-121011390055-8).
References
- Pintado-Sierra, M.; Rasero-Almansa, A. M.; Corma, A.; Iglesias, M.; Sanchez, F. Bifunctional Iridium-(2-aminoterephthalate)-Zr-MOF Chemoselective Catalyst for the Synthesis of Secondary Amines by One-Pot Three-Step Cascade Reaction. J. Catal. 2013, 299, 137–145. DOI: 10.1016/j.jcat.2012.12.004.
- Ma, R.; Zhou, Y.-B.; He, L.-N. Carbon Dioxide Promoted Reductive Amination of Aldehydes in Water Mediated by Iron Powder and Catalytic Palladium on Activated Carbon. Catal. Today. 2016, 274, 35–39. DOI: 10.1016/j.cattod.2016.01.048.
- Wang, C.; Yang, J.; Meng, X.; Sun, Y.; Man, X.; Li, J.; Sun, F. Manganese (Ii)-catalysed Dehydrogenative Annulation Involving C–C Bond Formation: Highly Regioselective Synthesis of Quinolines. Dalton Transact. 2019, 48(14), 4474–4478. DOI: 10.1039/C9DT00647H.
- Blake, L. C.; Roy, A.; Neul, D.; Schoenen, F. J.; Aubé, J.; Scott, E. E. Benzylmorpholine Analogs as Selective Inhibitors of Lung Cytochrome P450 2A13 for the Chemoprevention of Lung Cancer in Tobacco Users. Pharm. Res. 2013, 30(9), 2290–2302. DOI: 10.1007/s11095-013-1054-z.
- Al-Qawasmeh, R. A.; Lee, Y.; Cao, M. Y.; Gu, X.; Viau, S.; Lightfoot, J.; Wrigth, J. A.; Young, A. H. 11-Phenyl-[b, e]-Dibenzazepine Compounds: Novel Antitumor Agents. Bioorg. Med. Chem. Lett. 2009, 19(1), 104–107. DOI: 10.1016/j.bmcl.2008.11.001.
- Wang, J.; Cai, P.; Yang, X. L.; Li, F.; Wu, J. J.; Kong, L. Y.; Wang, X. B. Novel Cinnamamide-dibenzylamine Hybrids: Potent Neurogenic Sgents with Antioxidant, Cholinergic, and Neuroprotective Properties as Innovative Drugs for Alzheimer’s Disease. Eur. J. Med. Chem. 2017, 139, 68–83. DOI: 10.1016/j.ejmech.2017.07.077.
- Hunt-Painter, A. A.; Stocker, B. L.; Timmer, M. S. M. The Synthesis of the Molecular Chaperone 2,5-dideoxy-2,5-imino-Daltritol via Diastereoselective Reductive Amination and Carbamate Annulation. Tetrahedron. 2018, 74, 1307–1312. DOI: 10-1016/j.tet.2018.01.011.
- Hu, L.; Lin, L.; Wu, Z.; Zhou, S.; Liu, S. Recent Advances in Catalytic Transformation of Biomass-derived 5-hydroxymethylfurfural into the Innovative Fuels and Chemicals. Renew. Sust. Energ. Rev. 2017, 74, 230–257. DOI: 10.1016/j.rser.2017.02.042.
- Froidevaux, V.; Negrell, C.; Caillol, S.; Pascault, J.-P.; Boutevin, B. Biobased Amines: From Synthesis to Polymers; Present and Future. Chem. Rev. 2016, 116, 14181–14224. DOI: 10.1021/acs.chemrev.6b00486.
- Kolobova, E.; Mäki-Arvela, P.; Pestryakov, A.; Pakrieva, E.; Pascual, L.; Smeds, A.; Rahkila, J.; Sandberg, T.; Peltonen, J.; Murzin,D. Y. Reductive Amination of Ketones with Benzylamine over Gold Supported on Different Oxides. Catal. Lett. 2019, 149, 3432–3446. DOI: 10.1007/s10562-019-02917-1.
- Patil, N. M.; Bhanage, B. M. Fe@Pd/C: An Efficient Magnetically Separable Catalyst for Direct Reductive Amination of Carbonyl Compounds Using Environment Friendly Molecular Hydrogen in Aqueous Reaction Medium. Catal. Today. 2015, 247, 182–189. DOI: 10.1016/j.cattod.2014.07.057.
- Shokrolahi, A.; Zali, A.; Keshavarz, M. H. Reductive Amination of Aldehydes and Ketones by NaBH4 Using Carbon-based Solid Acid (CBSA) as Catalyst. Green Chem. Lett. Rev. 2011, 4(3), 195–203. DOI: 10.1080/17518253.2010.528051.
- Liang, S.; Monsen, P.; Hammond, G. B.; Xu, B. Au/TiO2 Catalyzed Reductive Amination of Aldehydes and Ketones Using Formic Acid as Reductant. Org. Chem. Front. 2016, 3(4), 505–509. DOI: 10.1039/C5QO00439J.
- Tamboli, A. H.; Chaugule, A. A.; Sheikh, F.-A.; Chung, W. J.; Hern, K. Synthesis, Characterization, and Application of Silica Supported Ionic Liquid as Catalyst for Reductive Amination of Cyclohexanone with Formic Acid and Triethyl Amine as Hydrogen Source. Chin. J. Catal. 2015, 36, 1365–1371. DOI: 10.1016/S1872-2067(15)60848-8.
- Yang, H.; Cui, X.; Deng, Y.; Shi, F. Reductive Amination of Aldehydes and Amines with an Efficient Pd/NiO Catalyst. Synth. Commun. 2014, 44, 1314–1322. DOI: 10.1080/00397911.2013.857690.
- Alinezhad, H.; Tajbakhsh, M.; Salehian, F.; Fazli, K. Reductive Amination of Aldehydes and Ketones to Their Corresponding Amines with N-Methylpyrrolidine Zinc Borohydride. Tetrahedron Lett. 2009, 50(6), 659–661. DOI: 10.1016/j.tetlet.2008.11.102.
- Bagal, D. B.; Watile, R. A.; Khedkar, M. V.; Dhake, K. P.; Bhanage, B. M. PS-Pd-NHC: An Efficient and Heterogeneous Recyclable Catalyst for Reductive Amination of Carbonyl Compounds with Primary/Secondary Amines in Aqueous Medium. Catal. Sci. Technol. 2012, 2, 354–358. DOI: 10.1039/C1CY00392E.
- Ichikawa, S.; Seki, T.; Ikariya, T. Carbon Dioxide-Promoted Selective Reductive Amination of Aliphatic Ketones with Aniline and Hydrogen Using a Pt/C Catalyst. Chem. Lett. 2012, 41, 1628–1629. DOI: 10.1246/cl.2012.1628.
- Deng, J.; Mo, L.; Zhao, F.; Hou, L.; Yang, L.; Zhang, Z. Sulfonic Acid Supported on Hydroxyapatite-encapsulated-γ-Fe2O3 Nanocrystallites as a Magnetically Separable Catalyst for One-pot Reductive Amination of Carbonyl Compounds. Green Chem. 2011, 13(9), 2576–2584. DOI: 10.1039/c1gc15470b.
- Genet, C.; Nguyen, X.; Bayatsarmadi, B.; Horne, M. D.; Gardiner, J.; Hornung, C. H. Reductive Aminations Using a 3D Printed Supported Metal(0) Catalyst System. J. Flow Chem. 2018, 8, 81–88. DOI: 10.1007/s41981-018-0013-6.
- Levi, N.; Neumann, R. Diastereoselective and Enantiospecific Direct Reductive Amination in Water Catalyzed by Palladium Nanoparticles Stabilized by Polyethyleneimine Derivatives. ACS Catal. 2013, 3, 1915–1918. DOI: 10.1021/cs4005453.
- Xing, L.; Cheng, C.; Zhu, R.; Zhang, B.; Wang, X.; Hu, Y. Self-Modulated Highly Chemoselective Direct-Reductive-Amination (DRA) of Benzaldehydes Straightforward to N-Monosubstituted Benzylamine Hydrochlorides. Tetrahedron. 2008, 64, 11783–11788. DOI: 10.1016/j.tet.2008.09.072.
- Zhou, J.; Dong, Z.; Wang, P.; Shi, Z.; Zhou, X.; Li, R. Palladium Supported on Hollow Magnetic Mesoporous Spheres as Recoverable Catalyst for One-Pot Reductive Amination of Aldehydes with Nitroarenes under Mild Conditions. J. Mol. Catal. A: Chem. 2014, 382, 15–22. DOI: 10.1016/j.molcata.2013.10.018.
- Nuzhdin, A. L.; Simonov, P. A.; Bukhtiyarova, G. A.; Eltsov, I. V.; Bukhtiyarov, V. I. Reductive Amination of 5-Acetoxymethylfurfural over Pt/Al2O3 Catalyst in a Flow Reactor. Mol. Catal. 2020, 111297. DOI: 10.1016/j.mcat.2020.111297.
- Song, S.; Wang, Y.; Yan, N. A Remarkable Solvent Effect on Reductive Amination of Ketones. Mol. Catal. 2018, 454, 87–93. DOI: 10.1016/j.mcat.2018.05.017.
- Martínez, J. J.; Nope, E.; Rojas, H.; Brijaldo, M. H.; Passos, F.; Romanelli, G. Reductive Amination of Furfural over Me/SiO2-SO3H (Me: Pt, Ir, Au) Catalysts. J. Mol. Catal. A: Chem. 2014, 392, 235–240. DOI: 10.1016/j.molcata.2014.05.014.
- Karageorge, G. N.; Macor, J. E. Synthesis of Novel Serotonergics and Other N-Alkylamines Using Simple Reductive Amination Using Catalytic Hydrogenation with Pd/C. Tetrahedron Lett. 2011, 52, 5117–5119. DOI: 10.1016/j.tetlet.2011.07.058.
- Stemmler, T.; Surkus, A.-E.; Pohl,M.M.; Junge, K.; Beller, M. Iron-Catalyzed Synthesis of Secondary Amines: On the Way to Green Reductive Aminations. ChemSusChem. 2014, 7, 3012–3016. DOI: 10.1002/cssc.201402413.
- Stemmler, T.; Westerhaus, F. A.; Surkus, A.-E.; Pohl,M.M.; Junge, K.; Beller, M. General and Selective Reductive Amination of Carbonyl Compounds Using a Core-Shell Structured Co3O4/NGr@C Catalyst. Green Chem. 2014, 16, 4535–4540. DOI: 10.1039/C4GC00536H.
- Cirujano, F. G.; Leyva-Pérez, A.; Corma, A.; Xamena, F. X. L. MOFs as Multifunctional Catalysts: Synthesis of Secondary Arylamines, Quinolines, Pyrroles, and Arylpyrrolidines over Bifunctional MIL-101. ChemCatChem. 2013, 5, 538–549. DOI: 10.1002/cctc.201200878.
- Pelckmans, M.; Renders, T.; Van De Vyver, S.; Sels, B. F. Biobased Amines through Sustainable Heterogeneous Catalysis. Green Chem. 2017, 19, 5303–5331. DOI: 10.1039/C7GC02299A.
- Bähn, S.; Imm, S.; Neubert, L.; Zhang, M.; Neumann, H.; Beller, M. The Catalytic Amination of Alcohols. ChemCatChem. 2011, 3, 1853–1864. DOI: 10.1002/cctc.201100255.
- Kimura, H.;. Progress in One-Step Amination of Long-Chain Fatty Alcohols with Dimethylamine: Development of Key Technologies for Industrial Applications, Innovations, and Future Outlook. Catal. Rev. Sci. Eng. 2011, 53, 1–90. DOI: 10.1080/01614940.2011.556913.
- Pera-Titus, M.; Shi, F. Catalytic Amination of Biomass-Based Alcohols. ChemSusChem. 2014, 7, 720–722. DOI: 10.1002/cssc.201301095.
- Simakova, I. L.; Simakov, A. V.; Murzin, D. Y. Valorization of Biomass Derived Terpene Compounds by Catalytic Amination. Catalysts. 2018, 8(9, 365), 1–36. DOI: 10.3390/catal8090365.
- Demidova, Y. S.; Simakova, I. L.; Estrada, M.; Beloshapkin, S.; Suslov, E. V.; Korchagina, D. V.; Volcho, K. P.; Salakhutdinov, N. F.; Simakov, A. V.; Murzin, D. Y. One-Pot Myrtenol Amination over Au Nanoparticles Supported on Different Metal Oxides. Appl. Catal. A. Gen. 2013, 464-465, 348–356. DOI: 10.1016/j.apcata.2013.06.013.
- Demidova, Y. S.; Simakova, I. L.; Wärnå, J.; Simakov, A.; Murzin, D. Y. Kinetic Modeling of One-Pot Myrtenol Amination over Au/ZrO2 Catalyst. Chem. Eng. J. 2014, 238, 164–171. DOI: 10.1016/j.cej.2013.09.007.
- Simakova, I. L.; Demidova, Y. S.; Estrada, M.; Beloshapkin, S.; Suslov, E. V.; Volcho, K. P.; Salakhutdinov, N. F.; Murzin, D. Y.; Simakov, A. Gold Catalyzed One-Pot Myrtenol Amination: Effect of Catalyst Redox Activation. Catal. Today. 2017, 279, 63–70. DOI: 10.1016/j.cattod.2016.01.044.
- Demidova, Y. S.; Suslov, E. V.; Simakova, I. L.; Korchagina, D. V.; Mozhajcev, E. S.; Volcho, K. P.; Salakhutdinov, N. F.; Simakov, A.; Murzin, D. Y. Selectivity Control in One-Pot Amination of Au/ZrO2 by Molecular Hydrogen Addition. J. Mol. Catal. A Chem. 2017, 426, 60–67. DOI: 10.1016/j.molcata.2016.10.034.
- Demidova, Y. S.; Suslov, E. V.; Simakova, I. L.; Volcho, K. P.; Salakhutdinov, N. F.; Simakov, A.; Murzin, D. Y. Promoting Effect of Alcohols and Formic Acid on Au-catalyzed One-pot Alcohol Amination. Mol. Catal. 2017, 433, 414–419. DOI: 10.1016/j.mcat.2017.02.040.
- Demidova, Y. S.; Suslov, E. V.; Simakova, I. L.; Korchagina, D. V.; Mozhajcev, E. S.; Volcho, K. P.; Salakhutdinov, N. F.; Simakov, A.; Murzin, D. Y. One-pot Monoterpene Alcohol Amination over Au/ZrO2 Catalyst: Effect of the Substrates Structure. J. Catal. 2018, 360, 127–134. DOI: 10.1016/j.jcat.2018.01.020.
- Demidova, Y. S.; Simakova, I. L.; Estrada, M.; Beloshapkin, S.; Suslov, E. V.; Volcho, K. P.; Salakhutdinov, N. F.; Simakov, A.; Murzin, D. Y. One-Pot Myrtenol Amination over Au, Au–Pd and Pd Nanoparticles Supported on Alumina. Catal. Lett. 2019, 149(12), 3454–3464. DOI: 10.1007/s10562-019-02958-6.
- Demidova, Y. S.; Simakova, I. L.; Suslov, E. V.; Volcho, K. P.; Salakhutdinov, N. F.; Simakov, A.; Murzin, D. Y.; Catalytic Myrtenol Amination over Gold, Supported on Alumina Doped with Ceria and Zirconia. Catal. Sust. Energy. 2018, 5(1), 49–58. DOI:10.1515/cse-2018-0007.
- De Jong, W.; Marcotullio, G. Overview of Biorefineries Based on Co-Production of Furfural, Existing Concepts and Novel Developments. Int. J. Chem. Reactor Eng. 2010, 8(1). DOI: 10.2202/1542-6580.2174.
- He, J.; Chen, L.; Liu, S.; Song, K.; Yang, S.; Riisager, A. Sustainable Access to Renewable N-containing Chemicals from Reductive Amination of Biomass-derived Platform Compounds. Green Chem. 2020, 22, 6714–6747. DOI: 10.1039/D0GC01869D.
- Dell’Anna, M. M.; Mastrorilli, P.; Rizzuti, A.; Leonelli, C. One-Pot Synthesis of Aniline Derivatives from Nitroarenes under Mild Conditions Promoted by a Recyclable Polymer-Supported Palladium Catalyst. Appl. Catal. A. Gen. 2011, 401, 134–140. DOI: 10.1016/j.apcata.2011.05.010.
- Sreedhar, B.; Reddy, P. S.; Devi, D. K. Direct One-Pot Reductive Amination of Aldehydes with Nitroarenes in a Domino Fashion: Catalysis by Gum-Acacia-Stabilized Palladium Nanoparticles. J. Org. Chem. 2009, 74, 8806–8809. DOI: 10.1021/jo901787t.
- Zhou, H.; Zhou, Y.; Liu, S.; Pi, D.; Shen, G. Water as a Hydrogen Source in Palladium-Catalyzed Reduction and Reductive Amination of Nitroarenes Mediated by Diboronic Acid. Tetrahedron. 2017, 73, 3898–3904. DOI: 10.1016/j.tet.2017.05.056.
- Fiorio, J. L.; López, N.; Rossi, L. M. Gold–Ligand-Catalyzed Selective Hydrogenation of Alkynes into Cis-Alkenes via H2 Heterolytic Activation by Frustrated Lewis Pairs. ACS Catal. 2017, 7(4), 2973–2980. DOI: 10.1021/acscatal.6b03441.
- Kalbasi, R. J.; Mazaheri, O. Synthesis and Characterization of Hierarchical ZSM-5 Zeolite Containing Ni Nanoparticles for One-Pot Reductive Amination of Aldehydes with Nitroarenes. Catal. Comm. 2015, 69, 86–91. DOI: 10.1016/j.catcom.2015.05.016.
- Nasrollahzadeh, M.; Sajadi, S. M. Preparation of Pd/Fe3O4 Nanoparticles by Use of Euphorbia Stracheyi Boiss Root Extract: A Magnetically Recoverable Catalyst for One-Pot Reductive Amination of Aldehydes at Room Temperature. J. Coll. Interf. Sci. 2016, 464, 147–152. DOI: 10.1016/j.jcis.2015.11.020.
- Bhardwaj, M.; Sharma, H.; Paul, S.; Clark, J. H. Fe3O4@SiO2/EDAC-Pd(0) as a Novel and Efficient Inorganic/Organic Magnetic Composite: Sustainable Catalyst for the Benzylic C-H Bond Oxidation and Reductive Amination under Mild Conditions. New J. Chem. 2016, 40, 4952–4961. DOI: 10.1039/C5NJ03413B.
- Jiang, L.; Zhou, P.; Zhang, Z.; Jin, S.; Chi, Q. Synthesis of Secondary Amines from One-Pot Reductive Amination with Formic Acid as the Hydrogen Donor over an Acid-Resistant Cobalt Catalyst. Ind. Eng. Chem. Res. 2017, 56(44), 12556–12565. DOI: 10.1021/acs.iecr.7b03621.
- Park, J. W.; Chung, Y. K. Hydrogen-Free Cobalt–Rhodium Heterobimetallic Nanoparticle-Catalyzed Reductive Amination of Aldehydes and Ketones with Amines and Nitroarenes in the Presence of Carbon Monoxide and Water. ACS Catal. 2015, 5(8), 4846–4850. DOI: 10.1021/acscatal.5b01198.
- Sydnes, M. O.; Isobe, M. One-Pot Reductive Monoalkylation of Nitro Aryls with Hydrogen over Pd/C. Tetrahedron Lett. 2008, 49, 1199–1202. DOI: 10.1016/j.tetlet.2007.12.030.
- Zhou, P.; Zhang, Z.; Jiang, L.; Yu, C.; Lv, K.; Sun, J.; Wang, S. A Versatile Cobalt Catalyst for the Reductive Amination of Carbonyl Compounds with Nitro Compounds by Transfer Hydrogenation. Appl. Catal. B: Env. 2017, 210, 522–532. DOI: 10.1016/j.apcatb.2017.04.026.
- Murugesan, K.; Senthamarai, T.; Chandrashekhar, V. G.; Natte, K.; Kamer, P. C. J.; Beller, M.; Jagadeesh, R. V. Catalytic Reductive Aminations Using Molecular Hydrogen for Synthesis of Different Kinds of Amines. Chem. Soc. Rev. 2020, 49, 6273–6328. DOI: 10.1039/C9CS00286C.
- Irrgang, T.; Kempe, R. Transition-Metal-Catalyzed Reductive Amination Employing Hydrogen. Chem. Rev. 2020, 120(17), 9583–9674. DOI: 10.1021/acs.chemrev.0c00248.
- Nasrollahzadeh, M.;. Green Synthesis and Catalytic Properties of Palladium Nanoparticles for the Direct Reductive Amination of Aldehydes and Hydrogenation of Unsaturated Ketones. New J. Chem. 2014, 38, 5544–5550. DOI: 10.1039/C4NJ01440E.
- Sharma, H.; Bhardwaj, M.; Kour, M.; Paul, S. Highly Efficient Magnetic Pd(0) Nanoparticles Stabilized by Amine Functionalized Starch for Organic Transformations under Mild Conditions. Mol. Catal. 2017, 435, 58–68. DOI: 10.1016/j.mcat.2017.03.019.
- Anderson, A. E.; Baddeley, C. J.; Wright, P. A. Tuning Pd-nanoparticle@MIL-101 (Cr) Catalysts for Tandem Reductive Amination. Catal. Lett. 2018, 148(1), 154–163. DOI: 10.1007/s10562-017-2208-0.
- Cui, X.; Liang, K.; Tian, M.; Zhu, Y.; Ma, J.; Dong, Z. Cobalt Nanoparticles Supported on N-Doped Mesoporous Carbon as a Highly Efficient Catalyst for the Synthesis of Aromatic Amines. J. Colloid Interface Sci. 2017, 501, 231–240. DOI: 10.1016/j.jcis.2017.04.053.
- Artiukha, E. A.; Nuzhdin, A. L.; Bukhtiyarova, G. A.; Zaytsev, S. Y.; Plyusnin, P. E.; Shubin, Y. V.; Bukhtiyarov, V. I. One-Pot Reductive Amination of Aldehydes with Nitroarenes over an Au/Al2O3 Catalyst in a Continuous Flow Reactor. Catal. Sci. Technol. 2015, 5, 4741–4745. DOI: 10.1039/C5CY00964B.
- Chen, Y. Z.; Zhou, Y. X.; Wang, H.; Lu, J.; Uchida, T.; Xu, Q.; Yu, S.-H.; Jiang, H. L. Multifunctional PdAg@ MIL-101 for One-Pot Cascade Reactions: Combination of Host–Guest Cooperation and Bimetallic Synergy in Catalysis. ACS Catal. 2015, 5(4), 2062–2069. doi:10.1021/cs501953d
- Chen, Y. Z.; Zhou, Y. X.; Wang, H.; Lu, J.; Uchida, T.; Xu, Q.; Yu, S.-H.; Jiang, H. L. Multifunctional PdAg@ MIL-101 for One-Pot Cascade Reactions: Combination of Host–Guest Cooperation and Bimetallic Synergy in Catalysis. ACS Catal. 2015, 5(4), 2062–2069. DOI: 10.1021/cs501953d.
- Gaudino, E. C.; Acciardo, E.; Tabasso, S.; Manzoli, M.; Cravotto, G.; Varma, R. S. Cross-Linked Cyclodextrins Bimetallic Nanocatalysts: Applications in Microwave-Assisted Reductive Aminations. Molecules. 2020, 25(2), 410. DOI: 10.3390/molecules25020410.
- García-Ortiz, A.; Vidal, J. D.; Climent, M. J.; Concepción, P.; Corma, A.; Iborra, S. Chemicals from Biomass: Selective Synthesis of N-Substituted Furfuryl Amines by the One-Pot Direct Reductive Amination of Furanic Aldehydes. ACS Sust. Chem. Eng. 2019, 7, 6243–6250. DOI: 10.1021/acssuschemeng.8b06631.
- Karve, V. V.; Sun, D. T.; Trukhina, O.; Yang, S.; Oveisi, E.; Luterbacher, J.; Queen, W. L. Efficient Reductive Amination of HMF with Well Dispersed Pd Nanoparticles Immobilized in a Porous MOF/polymer Composite. Green Chem. 2020, 22(2), 368–378. DOI: 10.1039/C9GC03140E.
- Santoro, F.; Psaro, R.; Ravasio, N.; Zaccheria, F. Reductive Amination of Ketones or Amination of Alcohols over Heterogeneous Cu Catalysts: Matching the Catalyst Support with the N-Alkylating Agent. ChemCatChem. 2012, 4, 1249–1254. DOI: 10.1002/cctc.201200213.
- Hu, L.; Cao, X.; Ge, D.; Hong, H.; Guo, Z.; Chen, L.; Sun, X.; Tang, J.; Zheng, J.; Lu, J.; Gu, H. Ultrathin Platinum Nanowire Catalysts for Direct C-N Coupling of Carbonyls with Aromatic Nitro Compounds under 1 Bar of Hydrogen. Chem. - Eur. J. 2011, 17, 14283–14287. DOI: 10.1002/chem.201100818.
- Nuzhdin, A. L.; Bukhtiyarova, M. V.; Bukhtiyarov, V. A. Cu-Al Mixed Oxide Derived from Layered Double Hydroxide as an Efficient Catalyst for Continuous-Flow Reductive Amination of Aromatic Aldehydes. J. Chem. Technol. Biotechnol. 2020, 95, 3292–3299. DOI: 10.1002/jctb.6508.
- Artiukha, E. A.; Nuzhdin, A. L.; Bukhtiyarova, G. A.; Gladkii, A. Y.; Bukhtiyarov, V. I. One-Pot Synthesis of Secondary Amines from Nitroarenes and Aldehydes on Supported Copper Catalysts in a Flow Reactor: The Effect of the Support. Kin. Catal. 2018, 59(5), 593–600. DOI: 10.1134/S0023158418050014.
- Nuzhdin, A. L.; Artiukha, E. A.; Bukhtiyarova, G. A.; Derevyannikova, E. A.; Bukhtiyarov, V. I. Synthesis of Secondary Amines by Reductive Amination of Aldehydes with Nitroarenes over Supported Copper Catalysts in a Flow Reactor. Catal. Comm. 2017, 102, 108–113. DOI: 10.1016/j.catcom.2017.09.001.
- Mokhov, V. M.; Popov, Y. V.; Paputina, A. N.; Nebykov, D. N.; Shishkin, E. V. Colloidal and Nanosized Catalysts in Organic Synthesis: XXIII. Reductive Amination of Carbonyl Compounds Catalyzed by Nickel Nanoparticles in a Plug-Flow Reactor. Russ. J. Gen. Chem. 2019, 89(12), 2333–2340. DOI: 10.1134/S1070363219120016.
- Yagafarov, N. Z.; Usanov, D. L.; Moskovets, A. P.; Kagramanov, N. D.; Maleev, V. I.; Chusov, D. Reductive Transformations of Carbonyl Compounds Catalyzed by Rhodium Supported on a Carbon Matrix by Using Carbon Monoxide as a Deoxygenative Agent. ChemCatChem. 2015, 7(17), 2590–2593. DOI: 10.1002/cctc.201500493.
- Wainwright, M. S.; Ahn, T.; Trimm, D. L.; Cant, N. W. Solubility of Hydrogen in Alcohols and Esters. J. Chem. Eng. Data. 1987, 32(1), 22–24. DOI: 10.1021/je00047a006.
- Zhang, J.; Yan, N. Production of Glucosamine from Chitin by Co‐solvent Promoted Hydrolysis and Deacetylation. ChemCatChem. 2017, 9, 2790–2796. DOI: 10.1002/cctc.201601715.
- Khodadadi-Moghaddam, M.; Habibi-Yangjeh, A.; Gholami, M. R. Kinetic Study of Heterogeneous Catalytic Hydrogenation of Cyclohexene to Cyclohexane in Ionic Liquid–Alcohols Mixtures. Appl. Catal. A. Gen. 2008, 341, 58–64. DOI: 10.1016/j.apcata.2008.02.002.
- Cukalovic, A.; Stevens, C. V. Production of Biobased HMF Derivatives by Reductive Amination. Green Chem. 2010, 12, 1201–1206. DOI: 10.1039/C002340J.
- Hizartzidis, L.; Cossar, P. J.; Robertson, M. J.; Simone, M. I.; Young, K. A.; McCluskey, A.; Gordon, C. P. Expanding the Utility of Flow Hydrogenation - a Robust Protocol Restricting Hydrodehalogenation. RSC Adv. 2014, 4, 56743–56748. DOI: 10.1039/C4RA09605C.
- Augustine, R. L.;. Heterogeneous Catalysis for the Synthetic Chemistry; Marcel Dekker: New York, 1996; pp 499.
- Romanazzi, G.; Petrelli, V.; Fiore, A. M.; Mastrorilli, P.; Dell’Anna, M. M. Metal-based Heterogeneous Catalysts for One-Pot Synthesis of Secondary Anilines from Nitroarenes and Aldehydes. Molecules. 2021, 26(4), 1120. DOI: 10.3390/molecules26041120.
- Ciaccia, M.; Di Stefano, S. Mechanisms of Imine Exchange Reactions in Organic Solvents. Org. Biomol. Chem. 2015, 13(3), 646–654. DOI: 10.1039/C4OB02110J.
- Zhu, M. M.; Tao, L.; Zhang, Q.; Dong, J.; Liu, Y. M.; He, H. Y.; Cao, Y. Versatile CO Assisted Direct Reductive Amination of 5-Hydroxymethylfurfural Catalyzed by a Supported Gold Catalyst. Green Chem. 2017, 19(16), 3880–3887. DOI: 10.1039/C7GC01579H.
- Wang, S.; Li, J.; Zhang, M.; Bai, P.; Zhang, H.; Tong, X. The Selective Reductive Amination of Aliphatic Aldehydes and Cycloaliphatic Ketones with Tetragonal Zirconium Dioxide as the Heterogeneous Catalyst. Mol. Catal. 2020, 494, 111–108. DOI: 10.1016/j.mcat.2020.111108.
- Demirci, U. B.; Akdim, O.; Andrieux, J.; Hannauer, J.; Chamoun, R.; Miele, P. Sodium Borohydride Hydrolysis as Hydrogen Generator: Issues, State of the Art and Applicability Upstream from a Fuel Cell. Fuel Cells. 2010, 10(3), 335–350. DOI: 10.1002/fuce.200800171.
- Hara, M.; Yoshida, T.; Takagaki, A.; Takata, T.; Kondo, J. N.; Hayashi, S.; Domen, K. A Carbon Material as A Strong Protonic Acid. Angew. Chem. 2004, 116, 3015–3018. DOI: 10.1002/ange.200453947.
- Nakajima, K.; Hara, M.; Hayashi, S. Environmentally Benign Production of Chemicals and Energy Using a Carbon‐Based Strong Solid Acid. J. Am. Ceram. Soc. 2007, 90(12), 3725–3734. DOI: 10.1111/j.1551-2916.2007.02082.x.
- Vassiliou, S.; Grabowiecka, A.; Kosikowska, P.; Berlicki, Ł. Three Component Kabachnik-Fields Condensation Leading to Substituted Aminomethane-p-Hydroxy-Methylphosphonic Acids as a Tool for Screening of Bacterial Urease Inhibitors. Arkivoc. 2012, 4, 33–43. DOI: 10.3998/ark.5550190.0013.404.
- Zhou, X.; Li, X.; Jiao, L.; Huo, H.; Li, R. Programmed Synthesis Palladium Supported on Fe3O4@ C: An Efficient and Heterogeneous Recyclable Catalyst for One-Pot Reductive Amination of Aldehydes with Nitroarenes in Aqueous Reaction Medium. Catal. Lett. 2015, 145(8), 1591–1599. DOI: 10.1007/s10562-015-1552-1.
- Yamane, Y.; Liu, X.; Hamasaki, A.; Ishida, T.; Haruta, M.; Yokoyama, T.; Tokunaga, M. One-Pot Synthesis of Indoles and Aniline Derivatives from Nitroarenes under Hydrogenation Condition with Supported Gold Nanoparticles. Org. Lett. 2009, 11, 5162–5165. DOI: 10.1021/ol902061j.
- Artyukha, E. A.; Nuzhdin, A. L.; Bukhtiyarova, G. A.; Bukhtiyarov, V. I. Flow Synthesis of Secondary Amines over Ag/Al2O3 Catalyst by One-Pot Reductive Amination of Aldehydes with Nitroarenes. RSC Adv. 2017, 7, 45856–45861. DOI: 10.1039/C7RA08986D.
- Zhu, Q. L.; Li, J.; Xu, Q. Immobilizing Metal Nanoparticles to Metal-Organic Frameworks with Size and Location Control for Optimizing Catalytic Performance. J. Am. Chem. Soc. 2013, 135(28), 10210–10213. DOI: 10.1021/ja403330m.
- Cisneros, L.; Serna, P.; Corma, A. Selective Reductive Coupling of Nitro Aliphatic Compounds with Aldehydes in Hydrogen Using Gold Catalyst. Chin. J. Catal. 2016, 37(10), 1756–1763. DOI: 10.1016/S1872-2067(16)62493-2.
- Zhou, P.; Yu, C.; Jiang, L.; Lv, K.; Zhang, Z. One-pot Reductive Amination of Carbonyl Compounds with Nitro Compounds with CO/H2O as the Hydrogen Donor over non-Noble Cobalt Catalyst. J. Catal. 2017, 352, 264–273. DOI: 10.1016/j.jcat.2017.05.026.