Abstract
Mare endometrosis is a major reproductive problem associated with low fertility and is characterized by persistent inflammation, TGFβ-1 signaling, and consequently, extracellular matrix deposition, which compromises endometrial glands. Mesenchymal stem cell-based products (MSCs), such as extracellular vesicles (EVs), have gained attention due to the regulatory effects exerted by their miRNA cargo. Here, we evaluated the impact of preconditioning equine adipose mesenchymal stem cells with TGFβ-1 for short or long periods on the anti-fibrotic properties of secreted extracellular vesicles. MSCs were isolated from six healthy horses and exposed to TGFβ-1 for 4, 24, and 0 h. The expression of anti-fibrotic and pro-fibrotic miRNAs and mRNAs in treated cells and miRNAs in the cargo of secreted extracellular vesicles was measured. The resulting EVs were added for 48 h to endometrial stromal cells previously induced to a fibrotic status. The expression of anti-fibrotic and pro-fibrotic genes and miRNAs was evaluated in said cells using qPCR and next-generation sequencing. Preconditioning MSCs with TGFβ-1 for 4 h enriched the anti-fibrotic miRNAs (mir29c, mir145, and mir200) in cells and EVs. Conversely, preconditioning the cells for 24 h leads to a pro-fibrotic phenotype overexpressing mir192 and mir433. This finding might have implications for developing an EV-based protocol to treat endometrial fibrosis in mares.
Introduction
Endometrosis is a major reproductive problem in mares, defined as peri-glandular fibrosis of the endometrium (Katila and Ferreira-Dias Citation2022) with a loss of tissue architecture, impaired cellular hemostasis, and persistence of inflammation (Buczkowska et al. Citation2014). Endometriosis is multifactorial, with persistent inflammation being one of its main causes (Rebordão et al. Citation2014). TGFβ-1 is pivotal for the transition between inflammation and tissue remodeling by stimulating the migration of neutrophils and inducing macrophage polarization and transcription of ECM-related genes in myofibroblasts (Sanjabi et al. Citation2009; Klingberg et al. Citation2013). However, in chronic inflammation, as in endometrosis, persisting signaling occurs, leading to massive differentiation of other resident cells, provoking the conversion of fibroblasts to myofibroblasts (Szóstek-Mioduchowska et al. Citation2019a) and dysregulation of TIMPS/MMP balance (Szóstek-Mioduchowska et al. Citation2020), interleukin (Szóstek et al. Citation2013; Szóstek-Mioduchowska et al. Citation2019b), and prostaglandin overexpression (Szóstek et al. Citation2012; Szóstek-Mioduchowska et al. Citation2020), leading to the deposition of collagen type I, which compromises endometrium gland functionality (Hoffmann et al. Citation2009).
Endometrosis has no cure since the compromise of tissue functionality is usually irreversible (Trundell Citation2022). Regenerative therapies using mesenchymal stem cells (MSCs) are a potential treatment tool, owing to their immunomodulatory and regenerative capacity (Gugjoo et al. Citation2019). Previously, it was hypothesized that the regenerative activity of MSCs was mediated through migration to the damaged area and subsequent homing and differentiation in the resident tissue (Balaji et al. Citation2012). Paracrine mechanisms have been identified as responsible for therapeutic activity, including soluble factors with immunomodulatory (Huang et al. Citation2022) and anti-fibrotic activity (Huang and Yang Citation2021). MSCs produce a broad range of biomolecules and extracellular vesicles (EVs), which are important tools in tissue repair and homeostasis due to their intrinsic molecular cargoes (Hou et al. Citation2021). EVs are anucleate lipidic bilayer structures with myriad cargoes, including miRNAs, capable of generating phenotypic changes in target cells via post-transcriptional regulation (Makarova et al. Citation2021).
Preconditioning MSCs with cytokines and growth factors, including TGFβ-1, have been used to increase their immunomodulatory potency (Saparov et al. Citation2016; Schäfer et al. Citation2016; Sarsenova et al. Citation2022; Le et al. Citation2024). The resulting phenotype is: activated MSCs with enhanced immunomodulatory and immunosuppressive features (Noronha et al. Citation2019). MSCs in a fibrotic environment, characterized by persistent TGFβ-1 signaling, are an important source of active myofibroblasts, which participate in ECM deposition (Qin et al. Citation2023). To the best of our knowledge, there are no previous reports on short exposition (4h) of cells in vitro to TGF-β1, others tried concentrations from 0.1 to 10 ng/ml for longer periods (24h; Li et al. Citation2021; Qin et al. Citation2023). We hypothesized that short chase with TGF-β1 would mimic the effect of low concentration exposition as in Li et al. (Citation2021) by shifting the cell’s secretory phenotype to an anti-fibrotic one, owing to the miRNA cargo of secreted EVs. This study aimed to analyze the effect of TGFβ-1 preconditioning of horse adipose MSCs on the expression of miRNAs related to fibrosis and how the EVs secreted by such cells, and the miRNAs carried by the EVs, influence the fibrotic phenotype in an in vitro model of fibrotic endometrium stromal cells.
Materials and methods
The experimental design is displayed in supplementary Figure S1
Biological samples, isolation and characterization of adipose MSCs
The animal study was approved by the Ethics Committee of the Faculty of Veterinary Sciences, University of Concepcion, Chile (CB-10-2019). The study was conducted in accordance with the local legislation and institutional requirements.
MSCs positive for CD90/CD44/MHCI and negative for MHCII were isolated in the laboratory from adipose tissue (AT-MSCs) of Chilean Thoroughbred horses (n = 6) and characterized as described by Cabezas et al. (Citation2018), following the criteria stablished by Dominici et al. (Citation2006).
TGFβ-1 preconditioning in at-MSCs
AT-MSCs were pooled and seeded in T175-flask plates at 20,000 cells/cm2 in DMEM High Glucose (HG), 1x Antibiotic-Antimycotic, 1x Glutamax, and 10% Fetal Bovine Serum (FBS). At 80% confluency, serum was reduced to 1% for 12 h. After this, 10 ng/mL of TGFβ-1 type I (PeproTech, USA) was supplemented with culture medium for 4 and 24 h. The control group was not treated with TGFβ-1 (T0). At the indicated time points, the monolayer was detached with 1X Trypsin-EDTA (Sigma-Aldrich, USA), pelleted, and total RNA was extracted. Expression of miRNAs related to fibrosis—anti-fibrotic (mir19, mir29b, mir29c, mir145, mir200, and mir486) and pro-fibrotic (mir17, mir192, mir199, and mir433)—was studied using 2ΔΔCT-qPCR.
Harvest and characterization of EVs
Monolayers of cells coming from 5 T175 flasks for each time point were used to generate EVs. After treatment as above, monolayers were washed twice with PBS and changed to culture medium with 1% FBS, previously depleted of EVs using ultracentrifugation protocol of Shelke et al. (Citation2014). After an additional 48 h, the medium was collected and clarified using a 10 kDa Amicon filter (UFC901024, Merck-Millipore, Germany). As per the manufacturer’s instructions, the retentate was used to isolate the EVs using the ExoLutE Exosome Isolation Kit (Rosetta Exosome, Korea). The EVs were resuspended in 100 µl of 0.22 µm-filtered commercial PBS and subjected to nanoparticle tracking analysis at 10 to 100 particles per frame using a Nano Sight NS300 (Malvern Instruments, Malvern, UK). PBS was used as a negative control, and samples were diluted 1:1000 in PBS and injected into a 5 µl/min continuous flow. Results were analyzed according to Gerritzen et al. (Citation2017) using the built-in software NTA 3.2 (Malvern Instruments, UK).
For transmission electron microscopy (TEM), five µl of EVs in PBS were fixed with 4% paraformaldehyde (1:1). The solution was placed on carbon-formvar-coated copper electron microscopy grids for 20 min and washed with PBS. Then, 1.5% glutaraldehyde was applied to the grid for 5 min and then washed thrice with molecular-grade water. Finally, 0.5% uranyl oxalate pH 7.0 (Electron Microscopy Sciences, USA) was applied for 5 min to facilitate contrast. The grid was dried, placed, and images were taken at 40,000× to 80,000× magnification on the Talos™ F200C transmission electron microscope (Thermo Fisher Scientific, USA).
For the evaluation of miRNA cargo in EVs, 20 µL of EV suspension was mixed with 20 µl of cell lysis buffer from the Cells-to-cDNA kit (Thermo Fisher ScientificTM, USA), and 25 fmol of synthetic spike (cel-mir39, NorgenTM, Canada) was added, heated at 75 °C, and finally frozen at −80 °C until qPCR analysis. The miRNAs measured were: mir29c, mir145, and mir200 (anti-fibrotic), and mir192 and mir433 (pro-fibrotic).
Exposition of EVs to endometrial stromal cells in fibrosis in vitro system
To measure the anti-fibrotic capacity of preconditioned AT-MSCs medium, we used an in vitro endometrial stromal cell (EndSCs) fibrosis system previously validated in our laboratory (Wong et al. Citation2023). Briefly, 800,000 endometrial stromal cells/well from mares in the follicular phase of the estrus cycle were seeded in 12-well plates in DMEM HG supplemented with 1% AAM, 10% FBS, 0.5 ng/mL progesterone, and 30 pg/mL estradiol. After attaching, FBS was reduced to 1%, and the medium was supplemented or not (naïve cells) with a fibrotic cocktail of 10 ng/ml each of IL1β, IL6, TNFα, and TGFβ-1 for 24 h. The monolayers were then gently washed with PBS, and the serum was changed to 1% EV-free FBS. Culture was prolonged for another 48 h. The following experimental design was used: endometrial cells primed or not with the fibrotic cocktail were exposed to 1010 EVs from AT-MSCs challenged with TGFβ-1 for 0, 4, or 24 h. The exposure of endometrial cells to the EVs coming from the AT-MSCs was allowed for 48 h, after that, the monolayers were washed with PBS, tripsinized, pelleted, and subjected to mRNA extraction, followed by conversion to cDNA and qPCR gene expression analysis of fibrotic gene markers (SMA, COL1A1, COL3A1, and CTGF). Samples from the best anti-fibrotic conditions were used for a global scan of the transcriptome using next-generation sequencing (NGS). To track the internalization of EVs, they were labeled with PKH-67 fluorescent dye (PKH67LG, Sigma-Aldrich, USA) according to the manufacturer’s instructions and added for 24 h to EndSCs cultured in medium supplemented with 1% FBS-depleted. At that point, the nuclei of the cells were stained with 1 µl of NucBlue dye (Thermo Fisher ScientificTM, USA), and internalization was analyzed in the EVOS FL Imaging System (Thermo Fisher ScientificTM, USA).
qPCR analysis for gene expression
Total RNA was isolated from cells using EZNA Total RNA Kit I (OMEGATM, USA) and resuspended in 30 µl of molecular-grade water. The quality was assessed by a 260/280 nm ratio using a spectrophotometer (BioTek EPOCH, Agilent TechnologiesTM, USA). For cellular miRNA analysis, cDNA was synthesized from 500 ng of RNA incubated with 2 µl of 0.1 mM ATP (New England BiolabsTM, USA), 2 µl of 10 µM RT primer, 2 µl of 10x poly (A) polymerase buffer (New England BiolabsTM), 0.2 µl of 1 mM of dNTP mix (Thermo Fisher ScientificTM, USA), 1 µl of SuperScript IVTM Reverse transcriptase (Thermo Fisher ScientificTM, USA), 0.4 µl of poly(A) polymerase (New England BiolabsTM, USA), and 0.6 µl of RiboLock (Thermo Fisher ScientificTM, USA) at 37 °C for 30 min, then 52 °C for 10 min, and finally 95 °C for 5 min for inactivation of enzymes. For miRNA cargo in EVs, 20 µl of EV suspension was mixed 1:1 with 20 cell lysis buffer (Cells-to-cDNA kit, Thermo Fisher ScientificTM, USA), and 25 fmol of synthetic spike (cel-mir39, NorgenTM, Canada) was added and heated at 75 °C. The cDNA was synthesized as described above.
To analyze mRNA gene expression, total RNA from cells was converted to cDNA using a high-capacity reverse transcription kit (Thermo Fisher ScientificTM, USA). Briefly, 500 ng of cDNA were mixed with 2 µl of 10x RT Buffer, 0.8 µl 25x dNTP mix, 10x RT Random primers, 1 µl MultiScribe Reverse Transcriptase, 1 µl RiboLockTM, and 3.2 µl of molecular-grade water. The reverse transcription was performed at 25 °C for 10 min, 37 °C for 120 min, and 85 °C for 5 min.
The qPCR reaction was performed in a Real-Time PCR System (MxPro 3000, Thermo Fisher ScientificTM, USA) with 5 µL of KiCqStart SYBR Green qPCR Ready Mix with ROX (Sigma-Aldrich, USA), 2.5 µL molecular-grade water, 0.5 mix of forward and reverse primers at 10 nM, and 2 µL of cDNA. Each reaction was performed in triplicate, and the resulting CT was collected. The data were processed using the 2ΔΔCT method (Livak and Schmittgen Citation2001), and the relative gene expression was expressed in fold change using SNORD43 (for miRNA related to fibrosis in MSCs), the geometric mean of SNORD43 and cel-mir39 for miRNA cargo evaluation in EVs, and the mRNA gene expression of GAPDH and B2M housekeeping genes. The time-zero group was used as a normalizer.
All the primers are listed in Table S1 of the Supplementary Section, and the mRNA primers were designed in-house using AmplifXTM software. The miRNA primer set was designed with miRprimerTM software.
Next-generation sequencing
EndSCs displaying the best anti-fibrotic profile were subjected to global transcriptomic analysis (NGS), alongside with EndSCs induced to fibrosis or not (naïve) non-treated with EVs. The isolated RNA was quantified, and RNA integrity was checked using an RNA pico-chip kit (AgilentTM, USA). The mRNA was isolated using the NEBNext® Poly(A) mRNA Magnetic Isolation Module (NEBTM, USA). The libraries were created using the Ultra™ II Directional RNA Library Prep Kit (NEBTM, USA) and sequenced using the Illumina NextSeq 500 platform (IlluminaTM, USA). The results were paired-end (PE) reads with 150 bp of length each. Subsequently, using FASTP software, the quality of the resulting raw reads was checked, the remaining adapters were trimmed, and low-quality reads (Phreads < 25 or length < 50 bp) were cleaned out. The filtered reads were mapped using HISAT2 software against the Equus caballus reference genome (EquCab3.0), and the produced SAM files were converted into BAM files using SAM tools. The individual gene quantification was performed using feature counts (R-subread package), contrasting the BAM files to the EquCab3.0 GTF annotation file (Ensembl version 104). The differential expression analysis was performed on the R platform using the EdgeR package, the results (PCA, Heatmap, and Volcano plots) were visualized using the ggplot2 package, and gene ontology prediction was realized using the clusterProfiler package.
Statistical analysis
Data were analyzed using R platform and expressed as means ± standard deviation. ANOVA was used to compare different conditions and post-hoc Tukey was used for pairwise comparisons. A p value < 0.05 was considered significant.
Results
Characterization of at-MSC
The isolated cells fulfilled the criteria of Dominici et al. Citation2006, i.e.: differentiation towards three mesodermal lineages. Adipogenic differentiation was evidenced by the formation of intracellular lipid droplets, which were stained with Oil Red. The formation of calcium mineralized deposits showed a differentiation towards the osteogenic lineage and the formation of a micro mass rich in glycoaminoglycans showed a capacity of differentiation towards the chondrogenic lineage. In addition, the immune phenotyping showed positivity for CD44, CD90 and MHCI and almost no expression of MHCII (supplementary Figure S2).
Expression of miRNAs related to fibrosis in at-MSCs
Expression of cellular miRNAs with anti-fibrotic or pro-fibrotic action was studied by qPCR after TGFβ-1 challenge.
Anti-fibrotic miRNAs: miR19: downregulated at 4 h of TGFβ-1 exposition, its expression abolished at 24 h; miR29, miR 29c, and miR486: upregulated at 4 h, downregulated at 24 h. Micro-RNAs 145 and 200 were highly (8-fold) upregulated at 4 h, then decreased at 24 h, but still higher than T0. The expression of pro-fibrotic miRNAs (mir17, mir192, mir199, and mir433) throughout the exposure time compared to the control showed a significant increase only at 24 h, with mir433 being the most expressed. In contrast, mir214 peaked at 4 h of exposure. All differences were statistically significant (p < 0.05; ).
Figure 1. Analysis by RT-qPCR of anti and pro-fibrotic miRNAs relative gene expression in at-MSC preconditioned with TGFβ-1 at time points 0h: red bars; 4h: blue bars; 24h hours: green bars.
Y-axes: fold change of the relative expression, the error bar is the standard deviation. Different letters indicate statistically significant differences between groups (p < 0.05). Three replicates per treatment. Housekeeping: SNORD43.
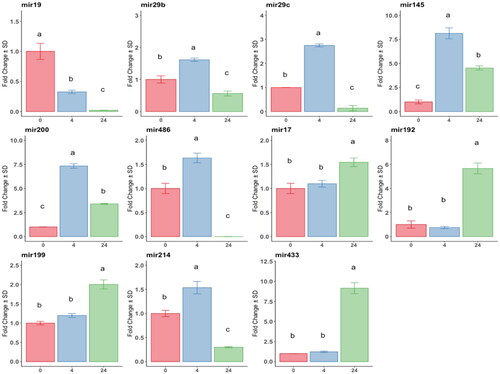
Changes in EVs and their cargo after preconditioning
We analyzed the vesicle cargo of AT-MSCs exposed to the treatments, and EVs were characterized (). TEM showed the classical cup-shaped morphology of EVs, and NTA analysis displayed a change in size of EVs, with 166 nm at T0 and a single peak in the histogram. At 4 h and 24 h, there was an increment in size (195.5 and 210 nm, respectively) with multiple peaks. Expression of EV protein markers was the same at all tested times.
Figure 2. Characterization of extracellular vesicles secreted by at-MSC and isolated from culture media. A to C, histograms of nanoparticle tracking analysis at time zero, 4, and 24h respectively, size is indicated on the X-axis, and concentration on the Y-axis. D: representative image from TEM showing the morphology of EV. E: Bottom right: Western blot analysis of selected EV markers, molecular weight is displayed.
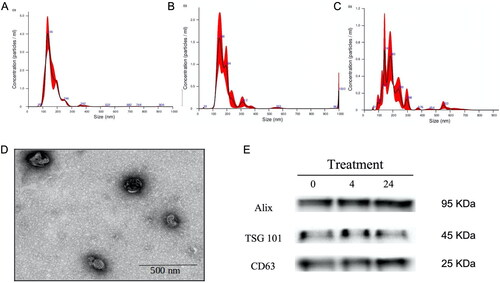
At the miRNA level, the anti-fibrotic miRNAs (mir29c, mir145, and mir200) were significantly highly expressed at 4 h compared to the control and 24-h groups; mir145 was the most upregulated. Conversely, the pro-fibrotic miRNAs mir192 and mir433 were significantly upregulated at 24 h of exposition compared to 0 h, and no difference was registered between 0 h and 4 h ().
Figure 3. Analysis by RT-qPCR of anti and pro-fibrotic miRNAs relative gene expression in EV, derived from at-MSC preconditioned with TGFβ-1 at time points 0h: red bars; 4h: blue bars; 24h hours: green bars. Y-axes: fold change of the relative expression, the error bar is the standard deviation. Different letters indicate statistically significant differences between groups (p < 0.05). Three replicates per treatment. Housekeeping: geometric mean of cel-mir39 and SNORD43.
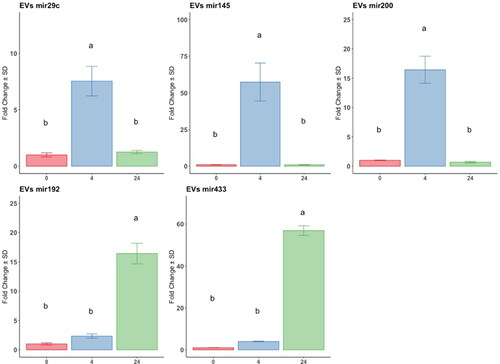
Evaluation of the anti-fibrotic properties of EVs in a cellular model
To evaluate the anti-fibrotic properties of EVs triggered by preconditioning MSCs with TGFβ-1 for 0, 4, and 24 h, we exposed EndSCs to EVs derived from AT-MSCs. The uptake was visualized using PKH-67-labeled EVs; stain was detected at all time points, while no staining was detected in control cells (). EndSCs were subjected to the fibrosing cocktail described earlier and exposed to EVs derived from AT-MSCs treated with TGFβ-1 for 0, 4, or 24 h. EVs from AT-MSCs pretreated for 4 h induced a decrease in the expression of SMA, CTGF, and COL1A1 compared to Induced, T0 and non-treated EndSCs (naïve), (p < 0.05). Conversely, after 24 h of TGFβ-1-preconditioning, expression of SMA and CTGF increased (p < 0.05) compared to non-treated EndSCs (naïve), while expression of COL1A1 and COL3A1 did not change ().
Figure 4. Uptake of EV labelled with PKH. EVs were derived from at-MSC preconditioned with TGFβ-1. Endometrial stromal cells were co-cultured with 1x1010 PKH-67 labelled EV for 48 h at 38.5 °C and visualized 24h later. Blue: nuclei stained with Nuc-Blue; green stained EVs with PKH-67 fluorescent dye. A) background control; B to D: internalization of EVs at time 0, 4 and 24h respectively.
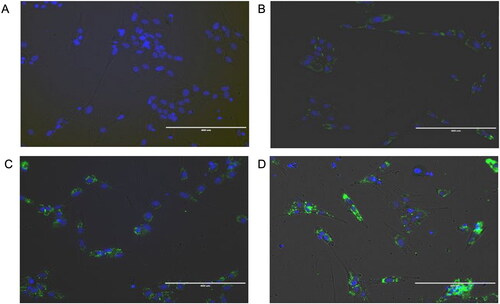
Figure 5. Analysis by RT-qPCR of the relative gene expression of fibrotic markers in an endometrial stromal in vitro fibrotic model. Endometrial stromal cells untreated (naïve) or primed with the fibrotic cocktail (induced), exposed to EV derived from at-MSC preconditioned with TGFβ-1 at time points 0h: green; 4h: purple and 24h hours: orange bars) as described in M&M. Y-axes: fold change of relative expression using the geometric mean of GAPDH and B2M as housekeeping. Different letters indicate statistically significant differences between groups (p < 0.05) and the error bar is the standard deviation. Three replicates per treatment.
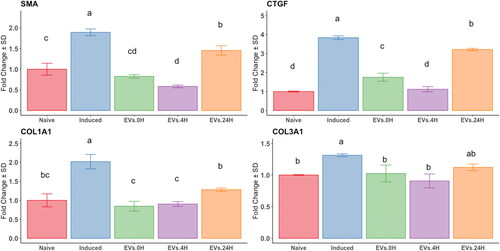
Global transcriptome analysis
At the PCA plot, an evident grouping according to the treatments was formed (). A total of 13394 genes were detected with a threshold > 0.5 counts per million (cpm) in at least two samples. For differentially expressed genes (DEG) analysis, we compared the following groups: (i) induced vs. naïve, (ii) EV.4H vs. induced group, and (iii) naïve vs. EV.4H and log2FC > 0.5 or log2FC < −0.5 and -Log10FDR < 0.05 as cut-off criteria (supplementary Table S2). Volcano plots and gene ontology(GO) analysis associated with DEG are depicted in supplementary Figures S3 and S4. In the first comparison, 1230 genes were upregulated in the induced condition compare to naïve condition. Gene onthology (GO) indicated an enrichment in SMAD-R binding and inflammatory response. In the second comparison, 1526 genes were upregulated in EV.4H condition compared to induced condition. GO indicated Interferon type I response. The third comparison 225 genes were upregulated in EV.4H condition vs naïve.
Figure 6. Principal component analysis (PCA) of naïve, induced and EVs.4H. PCA was executed in edgeR software with the normalized Log2-transformed count per million gene values.
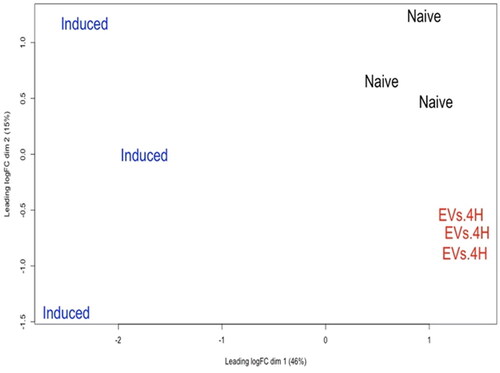
To have a clearer view of genes involved in pro-fibrotic or anti-fibrotic events in our experiments, we used an enrichment analysis based on the fibrosis-related gene list of Sun et al. (Citation2022). The top 100 expressed transcripts in the different experimental conditions were analyzed using an assemblage algorithm, which yielded two branches: A (induced) and B (naïve and EV.4H), clearly separated (). In addition, the algorithm grouped the pattern of genes into two clusters, where cluster 1 contained the most highly expressed genes in the induced group. The clusters of genes are listed in supplementary Table S3. In the heat map, SMA, COL1A1, and COL3A1 genes were upregulated in the induced group and downregulated in the naive and EV.4H groups, confirming our PCR results.
Discussion
This study aimed to investigate the impact of TGFβ-1 preconditioning on AT-MSC’s potential use as an anti-fibrotic therapeutic tool in mare endometrosis. In our experiments, a 4-h treatment of AT-MSCs with TGFβ-1 resulted in a notable increase of anti-fibrotic miRNAs in EVs. Conversely, if cells are exposed for longer periods (24 h), there was a shift towards a fibrosis-related mRNA and miRNA pattern. These findings were substantiated by exposing endometrial stromal cells to EVs from preconditioned AT-MSCs, leading to decreased expression of main fibrosis markers.
In healthy tissues, the overproduction of extracellular matrix components (ECM) and the onset of fibrosis are limited by anti-fibrotic miRNAs, like the mir29 family, which targets 16 genes related to ECM (Cushing et al. Citation2011). Others reported upregulation of mir29 expression in macrophages (Domingo-Gonzalez et al. Citation2015) via inhibition of H4K20me3 (Lyu et al. Citation2018). Other miRNAs, like mir145, regulate TGFBRII and SMAD3 (Megiorni et al. Citation2013), and their downregulation is observed in various types of fibrosis; mir200 plays a crucial role in regulating ZEB1/SMA expression (Yang et al. Citation2012); and mir486 directly targets SMAD2 (Douvris et al. Citation2022). In this work, these miRNAs were upregulated at 4 h with a decline at 24 h, suggesting a negative feedback of TGFβ-1 on its effectors to dampen the myofibroblast phenotype of AT-MSCs. Melling et al. (Citation2018) propose a similar negative feedback loop as a regulator of the cancer-associated fibroblast phenotype.
In fibrotic tissues, differentiation into myofibroblasts is accompanied by changes in miRNA levels, which target genes controlling disease progression (Fu et al. Citation2021). In the case of endometrosis, a specific pattern of miRNA is found for each stage of the pathology, implicating that miRNA are active participants in the processes of focal adhesion and extracellular matrix-receptor interaction (Wójtowicz et al. Citation2023).
In our work, at 24 h, there was an upsurge of pro-fibrotic miRNAs mir17, mir192, mir199, and mir433. miR 17 targets SMAD7, which negatively regulates the TGFβ-1/SMAD2/3 pathway and is linked to cystic and renal fibrosis (Oglesby et al. Citation2015; Fu et al. Citation2021). In turn, SMAD7 inhibition leads to increased expression of mir192 (Chung et al. Citation2010), which acts directly on the ZEB2-E-box repressor of COL1A2 (Kato et al. Citation2007). High levels of expression of mir199 are related to bleomycin-induced lung fibrosis in sheep and murine models and myofibroblast differentiation in oral submucous fibrosis with an increment of COL1A1 and COL3A1 (Yuan et al. Citation2019; Perera et al. Citation2021), and dysregulation of mir433 results in inhibition of AZIN1, a regulator of ECM production, with subsequent amplification of the TGFβ-1 signaling pathway (Li et al. Citation2013). The exact role of type I collagen in equine endometrial fibrosis remains unclear, but it is thought that its excessive deposition impairs endometrial gland functionality by disrupting proper tissue structure and function which compromises the integrity and function of the endometrial gland, promotes the development of cicatricial tissues with major tensile strength and ultimately reduced glandular functionality (Rebordão et al. Citation2018; Alpoim-Moreira et al. Citation2022).
In our experiments, endometrial stromal cells exposed to EVs released by AT-MSCs pretreated with TGFβ-1 for 4 h reduced the expression of fibrotic genes such as SMA, CTGF, and COL1A1. Notably, EVs coming from T0 preconditioning (basal levels, not exposed to TGFβ-1) led to a similar phenotype of fibrotic genes. Other reports support the anti-fibrotic properties of the AT-MSCs secretome and highlight the key action of miRNAs in reverting the myofibroblast phenotype (Liu et al. Citation2023). Basalova et al. Citation2020 note that human AT-MSCs significantly inhibited SMA expression in myofibroblasts by transferring anti-fibrotic miR29 within secreted EVs. In endometrial fibrosis, EV-enclosed mir145 ameliorated the disease in humans (Zhou et al. Citation2023). Noticeably, we found overexpression of pro-fibrotic miR199 in equine AT-MSCs (Navarrete et al. Citation2020). Therefore, MSCs may promote or fight fibrosis, depending on their culture or niche conditions. In vitro, this may be attained by fine-tuning the TGFβ-1 preconditioning of AT-MSCs, like in our experiments. At 4 h of TGFβ-1 preconditioning, there is an anti-fibrotic cargo of miRNAs in EVs, while the opposite is observed if cells are treated for 24 h. These results are consistent with those of Salehipour Bavarsad et al. (Citation2022), who observed a reduction of SMA and COL1A1 levels and inhibition of phosphorylated SMAD2/3 complex proteins in activated hepatic stellate cells using EVs derived from Wharton jelly MSCs pretreated with TGFβ-1 at low doses.
In this research, NGS revealed a different transcriptomic pattern in cells induced with the fibrotic cocktail compared to the control. The DEGs in these cells were related to TGFβ-1 stimulation, ECM organization, and regulation of the inflammatory response. Similar changes have been reported for endometriosis in mares (Szóstek et al. Citation2012; Szóstek-Mioduchowska et al. Citation2019a, Citation2019b). Of note was the high expression of MMP13, a proteolytic protein related to the progression of chronic inflammation pathologies, and POSTN, an ECM protein related to TGFβ-1 stimulus, which has been involved in the induction of the production of collagen type I and myofibroblast differentiation in lung fibrosis (Alzobaidi et al. Citation2022). Other relevant genes in fibrosis overexpressed in the induced condition were CCN2 or CTGF, ACTA2, COL1A2, and COL3A1.
Our results are in agreement with those of Szóstek-Mioduchowska et al. (Citation2023), who showed a differential expression of genes related to inflammation, abnormal metabolism and production of connective tissue in advanced degree of endometrosis. These results validate our endometrial in vitro model, characterized by chronic inflammation and ECM deposition in stromal cells (Trundell Citation2022).
When these cells were exposed to EVs from TGFβ-1-pretreated AT-MSCs, several interferon I-related genes were upregulated. Type I interferons are stimulators of antiviral genes, act as regulators of the immune response, and might antagonize TGFβ-1 action (Okada et al. Citation2018). Activation of the TL3 gene, a specific inducer of type-I interferons, mitigates the pro-fibrotic phenotype of skin myofibroblasts through autocrine interferon signaling, with disruption of SMAD2/3 phosphorylation and upregulation of their inhibitor, SMAD 7 (Fang et al. Citation2013). A similar mechanism is observed in interferon-gamma interference with TGFβ-1 response through JAK/STAT in hepatic stellate cells, leading to a reduction of SMA and fA1 expression via inhibition of the SMAD3/4 complex and overactivation of SMAD 7 (Weng et al. Citation2007). Also, the failure of inflammation control in fibrotic diseases has been related to the axis COX2/IFN-lambda, which is controlled by mir29 via its direct target of the DNA methyltransferase 3a gene, a COX2 inhibitor (Fang et al. Citation2012). Accordingly, we found an increase in mir29 expression in the 4 h EV-treated group and high expression of SMAD7, suggesting a possible mechanism of anti-fibrotic regulation caused by this miRNA cargo.
Conclusions
Here we showed that TGFβ-1 preconditioning of AT-MSCs for 4 h yields EVs whose miRNA cargo can be of use for treating fibrotic diseases such as mare endometriosis. These vesicles could inhibit the expression of genes related to the myofibroblast phenotype and ECM proteins while increasing the expression of interferon-related genes. To our knowledge, this is the first report on the anti-fibrotic action of EVs from MSC with short-term preconditioning using TGFβ-1.
Authors contribution
YW: Conceptualization, Data curation, Investigation, Methodology, Writing – original draft. AM: Investigation, Methodology, Validation, Conceptualization, Writing – review & editing. FN: Methodology, Validation, Writing –review & editing. PP: Writing – review & editing, Investigation, Methodology. LM-P: Writing – review & editing, Investigation, Methodology. CJ: Writing – review & editing, Investigation, GR: bioinformatics, cluster supervision. LR-A: Conceptualization, Funding acquisition, Supervision, Writing – review & editing. FOC: Funding acquisition, Methodology, Resources, Supervision, Validation, Writing – review & editing, Conceptualization, Data curation, Formal analysis.
Supplemental Material
Download Zip (1.4 MB)Disclosure statement
No potential conflict of interest was reported by the author(s)
Additional information
Funding
References
- Alpoim-Moreira J, Fernandes C, Pimenta J, Bliebernicht M, Rebordão MR, Castelo-Branco P, Szóstek-Mioduchowska A, Skarzynski DJ, Ferreira-Dias G. 2022. Metallopeptidades 2 and 9 genes epigenetically modulate equine endometrial fibrosis. Front Vet Sci. 9:970003. doi: 10.3389/fvets.2022.970003.
- Alzobaidi N, Rehman S, Naqvi M, Gulati K, Ray A. 2022. Periostin: a potential biomarker and therapeutic target in pulmonary diseases. J Pharm Pharm Sci. 25:137–148. doi: 10.18433/jpps32306.
- Balaji S, Keswani SG, Crombleholme TM. 2012. Mesenchymal stem cells in the regenerative wound healing phenotype. Adv Wound Care (New Rochelle). 1(4):159–165. doi: 10.1089/wound.2012.0361.
- Basalova N, Sagaradze G, Arbatskiy M, Evtushenko E, Kulebyakin K, Grigorieva O, Akopyan Z, Kalinina N, Efimenko A. 2020. Secretome of mesenchymal stromal cells prevents myofibroblasts differentiation by transferring fibrosis-associated micrornas within extracellular vesicles. Cells. 9(5):1272. doi: 10.3390/cells9051272.
- Buczkowska J, Kozdrowski R, Nowak M, Raś A, Mrowiec J. 2014. Endometrosis – significance for horse reproduction, pathogenesis, diagnosis, and proposed therapeutic methods. Pol J Vet Sci. 17(3):547–554. doi: 10.2478/pjvs-2014-0083.
- Cabezas J, Rojas D, Navarrete F, Ortiz R, Rivera G, Saravia F, Rodriguez-Alvarez L, Castro FO. 2018. Equine mesenchymal stem cells derived from endometrial or adipose tissue share significant biological properties, but have distinctive pattern of surface markers and migration. Theriogenology. 106:93–102. doi: 10.1016/j.theriogenology.2017.09.035.
- Chung ACK, Huang XR, Meng X, Lan HY. 2010. miR-192 mediates TGF-β/Smad3-driven renal fibrosis. J Am Soc Nephrol. 21(8):1317–1325. doi: 10.1681/ASN.2010020134.
- Cushing L, Kuang PP, Qian J, Shao F, Wu J, Little F, Thannickal VJ, Cardoso WV, Lü J. 2011. miR-29 is a major regulator of genes associated with pulmonary fibrosis. Am J Respir Cell Mol Biol. 45(2):287–294. doi: 10.1165/rcmb.2010-0323OC.
- Domingo-Gonzalez R, Wilke CA, Huang SK, Laouar Y, Brown JP, Freeman CM, Curtis JL, Yanik GA, Moore BB. 2015. Transforming growth factor-β induces microRNA-29b to promote murine alveolar macrophage dysfunction after bone marrow transplantation. Am J Physiol Lung Cell Mol Physiol. 308(1):L86–L95. doi: 10.1152/ajplung.00283.2014.
- Dominici M, Le Blanc K, Mueller I, Slaper-Cortenbach I, Marini FC, Krause DS, Deans RJ, Keating A, Prockop DJ, Horwitz EM. 2006. Minimal criteria for defining multipotent mesenchymal stromal cells. The International Society for Cellular Therapy position statement. Cytotherapy. 8(4):315–317. doi: 10.1080/14653240600855905.
- Douvris A, Viñas J, Burns KD. 2022. miRNA-486-5p: signaling targets and role in non-malignant disease. Cell Mol Life Sci. 79(7):376. doi: 10.1007/s00018-022-04406-y.
- Fang J, Hao Q, Liu L, Li Y, Wu J, Huo X, Zhu Y. 2012. Epigenetic changes mediated by MicroRNA miR29 activate cyclooxygenase 2 and Lambda-1 interferon production during viral infection. J Virol. 86(2):1010–1020. doi: 10.1128/JVI.06169-11.
- Fang F, Ooka K, Sun X, Shah R, Bhattacharyya S, Wei J, Varga J. 2013. A synthetic TLR3 ligand mitigates profibrotic fibroblast responses by inducing autocrine IFN signaling. J. Immunol. 191(6):2956–2966. doi: 10.4049/jimmunol.1300376.
- Fu H, Chu D, Geng X. 2021. Downregulation of miR-17 suppresses TGF-β1-mediated renal fibrosis through targeting Smad7. Mol Cell Biochem. 476(8):3051–3064. doi: 10.1007/s11010-021-04140-2.
- Gerritzen MJH, Martens DE, Wijffels RH, Stork M. 2017. High throughput nanoparticle tracking analysis for monitoring outer membrane vesicle production. J Extracell Vesicles. 6(1):1333883. doi: 10.1080/20013078.2017.1333883.
- Gugjoo MB, Makhdoomi DM, Sharma GT. 2019. Equine mesenchymal stem cells: properties, sources, characterization, and potential therapeutic applications. J Equine Vet Sci. 72:16–27. doi: 10.1016/j.jevs.2018.10.007.
- Hoffmann C, Ellenberger C, Mattos RC, Aupperle H, Dhein S, Stief B, Schoon H-A. 2009. The equine endometrosis: new insights into the pathogenesis. Anim Reprod Sci. 111(2-4):261–278. doi: 10.1016/j.anireprosci.2008.03.019.
- Hou Y, Li J, Guan S, Witte F. 2021. The therapeutic potential of MSC-EVs as a bioactive material for wound healing. Engineer Regenerat. 2:182–194. doi: 10.1016/j.engreg.2021.11.003.
- Huang Y, Wu Q, Tam PKH. 2022. Immunomodulatory mechanisms of mesenchymal stem cells and their potential clinical applications. IJMS. 23(17):10023. doi: 10.3390/ijms231710023.
- Huang Y, Yang L. 2021. Mesenchymal stem cell-derived extracellular vesicles in therapy against fibrotic diseases. Stem Cell Res Ther. 12(1):435. doi: 10.1186/s13287-021-02524-1.
- Katila T, Ferreira-Dias G. 2022. Evolution of the concepts of endometrosis, post breeding endometritis, and susceptibility of mares. Animals (Basel). 12(6):779. doi: 10.3390/ani12060779.
- Kato M, Zhang J, Wang M, Lanting L, Yuan H, Rossi JJ, Natarajan R. 2007. MicroRNA-192 in diabetic kidney glomeruli and its function in TGF-β-induced collagen expression via inhibition of E-box repressors. Proc Natl Acad Sci U S A. 104(9):3432–3437. doi: 10.1073/pnas.0611192104.
- Klingberg F, Hinz B, White ES. 2013. The myofibroblast matrix: implications for tissue repair and fibrosis. J Pathol. 229(2):298–309. doi: 10.1002/path.4104.
- Le B, Cressman A, Morales D, Fierro FA. 2024. First clinical experiences using preconditioning approaches to improve MSC-based therapies. Curr Stem Cell Rep. 10(1):1–7. doi: 10.1007/s40778-023-00232-5.
- Li R, Chung ACK, Dong Y, Yang W, Zhong X, Lan HY. 2013. The microRNA miR-433 promotes renal fibrosis by amplifying the TGF-β/Smad3-Azin1 pathway. Kidney Int. 84(6):1129–1144. doi: 10.1038/ki.2013.272.
- Li Z-J, Wang L-Q, Li Y-Z, Wang C-Y, Huang J-Z, Yu N-Z, Long X. 2021. Application of adipose-derived stem cells in treating fibrosis. World J Stem Cells. 13(11):1747–1761. doi: 10.4252/wjsc.v13.i11.1747.
- Liu Y-X, Sun J-M, Ho C-K, Gao Y, Wen D-S, Liu Y-D, Huang L, Zhang Y-F. 2023. Advancements in adipose-derived stem cell therapy for skin fibrosis. World J Stem Cells. 15(5):342–353. doi: 10.4252/wjsc.v15.i5.342.
- Livak KJ, Schmittgen TD. 2001. Analysis of relative gene expression data using real-time quantitative PCR and the 2 − ΔΔCT method. Methods. 25(4):402–408. doi: 10.1006/meth.2001.1262.
- Lyu G, Guan Y, Zhang C, Zong L, Sun L, Huang X, Huang L, Zhang L, Tian X-L, Zhou Z, et al. 2018. TGF-β signaling alters H4K20me3 status via miR-29 and contributes to cellular senescence and cardiac aging. Nat Commun. 9(1):2560. doi: 10.1038/s41467-018-04994-z.
- Makarova J, Turchinovich A, Shkurnikov M, Tonevitsky A. 2021. Extracellular miRNAs and cell–cell communication: problems and prospects. Trends Biochem Sci. 46(8):640–651. doi: 10.1016/j.tibs.2021.01.007.
- Megiorni F, Cialfi S, Cimino G, De Biase RV, Dominici C, Quattrucci S, Pizzuti A. 2013. Elevated levels of miR-145 correlate with SMAD3 down-regulation in cystic fibrosis patients. J Cyst Fibros. 12(6):797–802. doi: 10.1016/j.jcf.2013.03.007.
- Melling GE, Flannery SE, Abidin SA, Clemmens H, Prajapati P, Hinsley EE, Hunt S, Catto JWF, Coletta RD, Mellone M, et al. 2018. Corrigendum: a miRNA-145/TGF-β1 negative feedback loop regulates the cancer-associated fibroblast phenotype. Carcinogenesis. 39(8):1094–1094. doi: 10.1093/carcin/bgy083.
- Navarrete F, Wong YS, Cabezas J, Riadi G, Manríquez J, Rojas D, Furlanetto Mançanares AC, Rodriguez-Alvarez L, Saravia F, Castro FO. 2020. Distinctive cellular transcriptomic signature and MicroRNA cargo of extracellular vesicles of horse adipose and endometrial mesenchymal stem cells from the same Donors. Cell Reprogram. 22(6):311–327. doi: 10.1089/cell.2020.0026.
- Noronha NDC, Mizukami A, Caliári-Oliveira C, Cominal JG, Rocha JLM, Covas DT, Swiech K, Malmegrim KCR. 2019. Priming approaches to improve the efficacy of mesenchymal stromal cell-based therapies. Stem Cell Res Ther. 10(1):131. doi: 10.1186/s13287-019-1224-y.
- Oglesby IK, Vencken SF, Agrawal R, Gaughan K, Molloy K, Higgins G, McNally P, McElvaney NG, Mall MA, Greene CM. 2015. miR-17 overexpression in cystic fibrosis airway epithelial cells decreases interleukin-8 production. Eur Respir J. 46(5):1350–1360. doi: 10.1183/09031936.00163414.
- Okada Y, Wang T, Kasai K, Suzuki K, Takikawa Y. 2018. Regulation of transforming growth factor is involved in the efficacy of combined 5-fluorouracil and interferon alpha-2b therapy of advanced hepatocellular carcinoma. Cell Death Discov. 4(1):42. doi: 10.1038/s41420-018-0040-y.
- Perera UE, Derseh HB, Dewage SNV, Stent A, Wijayarathna R, Snibson KJ. 2021. Evaluation of microRNA expression in a sheep model for lung fibrosis. BMC Genomics. 22(1):827. doi: 10.1186/s12864-021-08073-4.
- Qin L, Liu N, Bao C, Yang D, Ma G, Yi W, Xiao G, Cao H. 2023. Mesenchymal stem cells in fibrotic diseases—the two sides of the same coin. Acta Pharmacol Sin. 44(2):268–287. doi: 10.1038/s41401-022-00952-0.
- Rebordão MR, Amaral A, Lukasik K, Szóstek-Mioduchowska A, Pinto-Bravo P, Galvão A, Skarzynski DJ, Ferreira-Dias G. 2018. Impairment of anti-fibrotic PGE2 pathway may influence neutrophil extracellular traps-induced fibrosis in the mare endometrium. Domest Anim Endocrinol. 67:1–10. doi: 10.1016/j.domaniend.2018.10.004.
- Rebordão M, Galvão A, Szóstek A, Amaral A, Mateus L, Skarzynski D, Ferreira-Dias G. 2014. Physiopathologic mechanisms involved in mare endometrosis. Reprod Domest Anim. 49(Suppl. 4):82–87. doi: 10.1111/rda.12397.
- Salehipour Bavarsad S, Jalali MT, Bijan Nejad D, Alypoor B, Babaahmadi Rezaei H, Mohammadtaghvaei N. 2022. TGFβ1-pretreated exosomes of Wharton jelly mesenchymal stem cell as a therapeutic strategy for improving liver fibrosis. Hepat Mon. 22(1):1–12. doi: 10.5812/hepatmon-123416.
- Sanjabi S, Zenewicz LA, Kamanaka M, Flavell RA. 2009. Anti-inflammatory and pro-inflammatory roles of TGF-β, IL-10, and IL-22 in immunity and autoimmunity. Curr Opin Pharmacol. 9(4):447–453. doi: 10.1016/j.coph.2009.04.008.
- Saparov A, Ogay V, Nurgozhin T, Jumabay M, Chen WCW. 2016. Preconditioning of human mesenchymal stem cells to enhance their regulation of the immune response. Stem Cells Int. 2016:3924858–3924810. doi: 10.1155/2016/3924858.
- Sarsenova M, Kim Y, Raziyeva K, Kazybay B, Ogay V, Saparov A. 2022. Recent advances to enhance the immunomodulatory potential of mesenchymal stem cells. Front Immunol. 13:1010399. doi: 10.3389/fimmu.2022.1010399.
- Schäfer R, Spohn G, Baer PC. 2016. Mesenchymal stem/stromal cells in regenerative medicine: can preconditioning strategies improve therapeutic efficacy. Transfus Med Hemother. 43(4):256–267. doi: 10.1159/000447458.
- Shelke GV, Lässer C, Gho YS, Lötvall J. 2014. Importance of exosome depletion protocols to eliminate functional and RNA‐containing extracellular vesicles from fetal bovine serum. J Extracell Vesicles. 3(1):24783. doi: 10.3402/jev.v3.24783.
- Sun Y-Z, Hu Y-F, Zhang Y, Wei S-Y, Yang B-L, Xu Y-P, Rong Z-L, Wang D, Yang B. 2022. FibROAD: a manually curated resource for multi-omics level evidence integration of fibrosis research. Database (Oxford). 2022:baac015. doi: 10.1093/database/baac015.
- Szóstek AZ, Lukasik K, Galvão AM, Ferreira-Dias GM, Skarzynski DJ. 2013. Impairment of the interleukin system in equine endometrium during the course of endometrosis. Biol Reprod. 89(4):79. doi: 10.1095/biolreprod.113.109447.
- Szóstek-Mioduchowska AZ, Baclawska A, Okuda K, Skarzynski DJ. 2019a. Effect of proinflammatory cytokines on endometrial collagen and metallopeptidase expression during the course of equine endometrosis. Cytokine. 123:154767. doi: 10.1016/j.cyto.2019.154767.
- Szóstek-Mioduchowska AZ, Baclawska A, Rebordão MR, Ferreira-Dias G, Skarzynski DJ. 2020b. Prostaglandins effect on matrix metallopeptidases and collagen in mare endometrial fibroblasts. Theriogenology. 153:74–84. doi: 10.1016/j.theriogenology.2020.04.040.
- Szóstek-Mioduchowska AZ, Lukasik K, Skarzynski DJ, Okuda K. 2019b. Effect of transforming growth factor-β1 on α-smooth muscle actin and collagen expression in equine endometrial fibroblasts. Theriogenology. 124:9–17. doi: 10.1016/j.theriogenology.2018.10.005.
- Szóstek-Mioduchowska A, Słowińska M, Pacewicz J, Skarzynski DJ, Okuda K. 2020. Matrix metallopeptidase expression and modulation by transforming growth factor-β1 in equine endometrosis. Sci Rep. 10(1):1119. doi: 10.1038/s41598-020-58109-0.
- Szóstek-Mioduchowska A, Wójtowicz A, Sadowska A, Moza Jalali B, Słyszewska M, Łukasik K, Gurgul A, Szmatoła T, Bugno-Poniewierska M, Ferreira-Dias G, et al. 2023. Transcriptomic profiling of mare endometrium at different stages of endometrosis. Sci Rep. 13(1):16263. doi: 10.1038/s41598-023-43359-5.
- Szóstek AZ, Siemieniuch MJ, Lukasik K, Galvão AM, Ferreira-Dias GM, Skarzynski DJ. 2012. mRNA transcription of prostaglandin synthases and their products in the equine endometrium in the course of fibrosis. Theriogenology. 78(4):768–776. doi: 10.1016/j.theriogenology.2012.03.024.
- Trundell DA. 2022. Endometriosis in mare; what the mare can teach us when dealing with endometriosis in the woman. In: A. P. Gonçalves, editor. Endometriosis – recent advances, new perspectives and treatments [Internet]. [place unknown]: IntechOpen; [accessed 2023 Jul 10]. doi:10.5772/intechopen.100515.
- Weng H, Mertens PR, Gressner AM, Dooley S. 2007. IFN-γ abrogates profibrogenic TGF-β signaling in liver by targeting expression of inhibitory and receptor SMADS. J Hepatol. 46(2):295–303. doi: 10.1016/j.jhep.2006.09.014.
- Wójtowicz A, Molcan T, Lukasik K, Żebrowska E, Pawlina-Tyszko K, Gurgul A, Szmatoła T, Bugno-Poniewierska M, Ferreira-Dias G, Skarzynski DJ, et al. 2023. The potential role of miRNAs and regulation of their expression in the development of mare endometrial fibrosis. Sci Rep. 13(1):15938. doi: 10.1038/s41598-023-42149-3.
- Wong YS, Mançanares AC, Navarrete FI, Poblete PM, Méndez-Pérez L, Ferreira-Dias GML, Rodriguez-Alvarez L, Castro FO. 2023. Mare stromal endometrial cells differentially modulate inflammation depending on Oestrus cycle status: an in vitro study. Front Vet Sci. 10:1271240. doi: 10.3389/fvets.2023.1271240.
- Yang S, Banerjee S, De Freitas A, Sanders YY, Ding Q, Matalon S, Thannickal VJ, Abraham E, Liu G. 2012. Participation of miR-200 in pulmonary fibrosis. Am J Pathol. 180(2):484–493. doi: 10.1016/j.ajpath.2011.10.005.
- Yuan Y, Li N, Zeng L, Shen Z, Jiang C. 2019. Pathogenesis investigation of miR‐199‐5p in oral submucous fibrosis based on bioinformatics analysis. Oral Dis. 25(2):456–465. doi: 10.1111/odi.13008.
- Zhou L, Dong L, Li H, Liu H, Yang J, Huang Z. 2023. Mesenchymal stem cell-derived exosomes ameliorate TGF-β1-induced endometrial fibrosis by altering their miRNA profile. Am J Transl Res. 15(5):3203–3216.