ABSTRACT
Robot grippers based on the jamming of granular material have been studied widely in previous years. Recently, also other benefits and challenges of granular material have emerged for robotics. We discuss various functions of granular matter in robotic actuation, sensory processing, locomotion, and manipulation. We also provide a review of the design and methods of robots for moving in or on challenging granular environments. Drawing on the properties of granular material and their potential applications, we propose our unique perspectives and innovative ideas for future research and development in this field.
1. Introduction
Granular material is made up of solid particles which can be small-grained such as flour or sand, or larger pieces like fruits, cobbles, or the tetrapods that are used to reduce coastal erosion. A key point for the suitability of granular matter in robotics and beyond is the capability of switching between liquid-like behaviour and a quasi-solid state. Namely, if the individual particles are cushioned from each other by a layer of gas or liquid, they can slide across each other, while in the solid case, the particles are jammed against each other and can hardly move.
The transformation of soft and flowable material into customisable rigid shapes, is very promising for the increased demand for versatile and adaptive robotic components, in particular in the context of the emerging field of soft robotics.
Similar to the phenomenon of the freezing of ice, the behaviour of granular materials can be described in terms of a phase transition from liquid to solid. When the phase transition happens the flexible material becomes hard and retains its form which can be exploited in grippers that first adapt to an object and then ‘freeze’ to cling to its surface according to the ideal shape that was assumed before. This jamming transition can be considered as a break-through in robotics to realise universal grippers, and has been discussed and reviewed before [Citation1].
Granular material also possesses potential for robotic sensing and morphological information processing via phenomena such as directional responses, nonstandard friction, domed pressure profiles, efficient damping, and controllable plasticity, all of which may enable new designs in modern robotics.
Granular material is not an entirely new subject: The study of the dynamics of sand dunes, for example, goes back several decades [Citation2]. By noticing the similarity of the dynamics of sand and of powder snow, Bangold anticipated already a more general approach to granular matter. By 1958 a good understanding of the mechanics of packages of spheres, elastic or rigid, under various kinds of stress was obtained [Citation3]. In 1968, based on the example of the North London retaining walls failures, Schofield and Wroth studied the interaction of granular material with water in their introduction to soil mechanics [Citation4]. In particular, considering interlocking among particles enabled a description of practically relevant phenomena by providing an explanation for the transition between quasi-solid and quasi-fluid behaviour. The related critical phenomena can be seen as a precursor to the jamming transition that is important also here. The study of the dynamics of granular flows was advanced in the 1980s by Savage [Citation5,Citation6] helped to popularise the complexity of the phenomena of granular matter that can behave in completely different way in the static equilibrium case and under small noise. The advent of a universal [Citation7] granular-matter-based gripper [Citation8] was also not without precursors, such as adaptive jaws for grippers [Citation9] and robotic fingers [Citation10]. Since then a much wider range of applications of granular materials in robotics has begun to emerge owing to the potential of the discovered methods related to flexibility, adaptability, dexterity, and resilience in robotics.
We will provide an overview of the current state of research on granular material that is relevant for applications in robotics. As principles (see Figure ) and applications of granular jamming technology have been reviewed already elsewhere [Citation1], we will touch this subject only briefly and will shift the focus to research on granular material in other areas inasmuch as there is a relation to robotics. We will also consider designs of robots for use in granular environments. The discussion chapter will highlight some of the challenges and opportunities associated with the typical techniques of granular material, and point to future directions for research in this field.
2. Universal robotic grippers
Many studies have been conducted to investigate the factors that affect the grasping effectiveness of jamming grippers [Citation1,Citation11–13]. Currently, universal grippers are fairly well-developed for various applications, for more detail see [Citation1]. Their performance has been evaluated based on various factors, including technical parameters as well as the effect of factors such as temperature [Citation14] and vibration [Citation15]. It is anticipated that the focus of research will shift further towards more sophisticated structural designs and more flexible operations that go beyond the scope of traditional grasping.
Although granular jamming has the potential to induce substantial stiffness variations, its effect on deformation is generally modest. Therefore, in hybrid jamming a combination of jamming with other actuation techniques is used in order to achieve the desired motion, especially in components with complex structures or dynamics.
A typical application to finger-like jamming grippers is to utilise pneumatic deformation directly [Citation16]. This method can employ passive jamming of particles to stabilise the designed shape and increase stiffness [Citation17,Citation18]. Applications with this method can include intricate internal structures [Citation19] or the effect of specific materials [Citation20] for better controllability and more versatile deformation modes. Using instead wire control for the actuated portion in conjunction with the reinforced portion of granular jamming can provide higher control precision [Citation21]. If jamming is used only for braking, multi-chamber structures seem preferable, such as a structure consisting of three stiff chambers that function as bones connected by two chambers filled with particles to serve as joints [Citation22]. Granular jamming is thus used as an auxiliary technique for stiffness modulation, working in conjunction with the primary actuator to achieve the desired functionality of the soft robot.
The comparison of different jamming designs [Citation23] yields slight advantage for layered designs over granular designs for the task of stiffening a finger-type effector in a button-pushing task. While the stiffness changes by a factor of 8 were measured, theory [Citation24] predicts that there is no limit for ideal hard-sphere material that is self-locking when bent.
Further work includes an actuator consisting of a jamming pad in combination with stiff claws [Citation25], and may enable rock-climbing using the ability to conform to a variety of shapes. For the moment, robotic assembly appears to be a more manageable task [Citation26]. Also other combinations of jamming pads and traditional mechanisms appear promising, e.g. with chains [Citation21,Citation27], or with ball joints as kind of backbone spine within a jamming cushioning [Citation28].
The retention forces resulting from geometric interlocking and suction are considerably more substantial than those attributed to static friction. Soft particles, in lieu of rigid particles, induces a squeezing effect that produces substantial frictional and holding forces [Citation29]. However, it should be noted that the augmentation of the clamping force through soft particles is restricted, while they make the gripper as a whole deformable, so there is a trade-off between the two effects.
3. Robotic interaction using granular material
3.1. Fingers and arms
In addition to the popular spherical universal gripper, granular jamming technology is also used for long, strip-like components to gain flexibility and to enable more complex operations.
The study of finger-like granular components focuses on the degree of bending of the component and the change in stiffness. Through membrane coupling [Citation30] and inter-particle interactions, the stiffness of particle structures can change with external pressure variations. Building on this foundation, finger-shaped structures for gripping actions via passive jamming have been proposed [Citation17]. Furthermore, by improving the structure of the membrane, such as increasing crease areas, the performance of passive jamming can be further enhanced [Citation18]. Active fingers based on jamming are typically paired with pneumatic actuators to achieve soft gripping. Its feasibility and generality is discussed in Ref. [Citation16]. A related study tested the effect of finger stiffness for various pressure levels on gripping performance [Citation20]. Another approach is to push solid particles directly via a piston rather than by controlling the medium as in a pneumatic or hydraulic system. Although the stiffness changes are less dramatic, fingers can be controlled thus to grasp and bend reliably [Citation31]. At this point, it should be noted that in jamming studies, finger-coordinated gripping behaviour is always achieved through three-finger gripping, which may mean that simple bending is not used to its full potential.
Inspired by the muscle fibre patterns of the human hand, fibre threads were used in conjunction with a chain-like granular jamming chamber [Citation21] to simulate various hand movements which increased the stiffness range. Textile ropes can simulate tendons which interact with modules composed of granular material to form a skeleton [Citation24] which realises more general shapes than even a human hand.
However, realistic finger simulation depends on the design of the chamber morphology and the actuation of the joints. Pneumatic components can be used as joints here as well to connect multiple independent granular jamming chambers to simulate finger morphology [Citation32], which preserves the advantages of granular jamming while more accurately adjusting finger shape. Another bio-mimetic design involves using integrated layer jamming as bones and particle jamming as joints, where the layer regions and particle regions are interlocked with each other to ensure load transfer from the fixed finger end to the fingertip [Citation22]. These design innovations cater to particle interference technology for some of the more demanding soft robotics applications.
Stiffness changes based on jamming are typically influenced by only small volume changes, and tests have shown that their deformation range still exceeds that of shape-memory metals [Citation30]. The advantage of incorporating particles into pneumatic-based actuators is that they can achieve greater force and more controllable curvature angles [Citation33]. Granular components have been used in the medical applications, such as endoscopes with variable stiffness arms with a camera at the end [Citation34]. The variable stiffness and non-metallic properties make granular material highly desirable for medical applications. In proposed surgical manipulators each granular module has omnidirectional bending, extension, and stiffening capabilities [Citation19,Citation35]. Together with other components made of magnetic material, components with variable stiffness can also be used for soft tissue retraction during surgery [Citation36].
In addition, this type of granular component can also be used in designing larger structures to replace traditional rigid robot arms [Citation37]. Similar to other soft robots, the precision of these granular arms may be relatively limited, and the weight of the device may affect its performance, as addressed in their research. Nevertheless, granular soft arms have been demonstrated to perform complex actions successfully. Related research also suggests that elongated granular jamming components can be utilised as robot legs [Citation38] or other types of limbs.
To use a universal gripper for climbing, a deformable skirt generates an airtight seal a suction cup even on uneven surfaces as concrete, tile, and riveted structures. The combination of gripping force and adhesion provide the universal vacuum gripper [Citation40] with the ability to climb vertical walls. Similarly humanoid robots robots can be made to climb vertically [Citation41]. A design of a hand for climbing uses granular material in the palm to distribute the load evenly across four fingers. This is combined with a number of spiny phalanges to work on rocky surfaces [Citation25]. This research spins outs also to object manipulation, where using merely fingertip-sized components can provide strong suction to fix objects [Citation42].
3.2. Damping and energy dissipation
Granular material also possesses notable advantages when used in feet or climbing appendages for robots. Jamming components in robotic feet can improve the mobility of legged robots on uneven and deformable terrains by adaptation of both foot shape and stiffness. A suggestive approach is to maintain a jamming component in a loose state while the foot is not in contact with the ground. This allows the particles inside the membrane to flow freely and to fully adapt to the terrain. At the moment of impact, positive pressure jamming is utilised to stiffen the foot, so that the component dampens the impact and provides support [Citation37]. A bio-mimetic design [Citation43] imitated soft paw pads of dogs or cats by high-traction and soft underlying tissue using granular material within suitable membranes. Experimental tests of general characteristics such as friction, damping, and deformation on a quadruped robot demonstrated the advantages of this soft-foot system. The same method of terrain adaptation was tested in a quadruped robot, now with a focus on the ability to rapidly switch the state of jamming in order to achieve faster movement on uneven terrain [Citation44]. Also more complex terrains were studied, e.g. soft flowable ground such as sand [Citation45].
Due to the discrete damping effect of particles, which refers to their ability to absorb and dissipate energy by moving relative to each other when subjected to external vibrations, particle systems exhibit excellent performance in reducing vibration. This property has led to their use as vibration-damping material in engineering structures [Citation46], as well as in machinery such as vehicle suspensions [Citation47] to improve their seismic performance. Previous research has focused on specific applications, such as spring systems [Citation48], while others have explored ways to improve energy dissipation efficiency [Citation49] through the use of granular binary mixtures [Citation50] or the application of microgravity [Citation51]. Of particular interest is the potential impact of active vibration fluidisation on granular jamming performance [Citation15]. By controlling the frequency and amplitude of the vibration waveform, the gripping strength of soft interference fixtures can be effectively enhanced.
The use of particles for buffering and vibration reduction has many advantages, such as simple equipment, low manufacturing costs, and wide applicability, such as applying damping effects to torque [Citation52]. The greatest advantage of vacuum packed particles lies in the fact that the damping level can be controlled smoothly by vacuum level. The dissipation characteristics are provided by the frictional phenomena that occur between loose-particle material, with the damper operation characteristics being close to linear [Citation49]. Granular dampers can also be used in parking buffers as practical tests have shown [Citation53]. Further explorations are required for leveraging the performance of granular material in terms of energy dissipation.
Loose granular media possess acoustic properties that can mitigate noise [Citation54]. Relevant investigations have delved into the effective mass of granular media [Citation55] in the field of acoustics, as well as the mechanisms for acoustic absorption in dry and weakly wet granular media [Citation56]. In this application, the moisture content between the granular medium is crucial, as the dampening of acoustic modes is primarily dominated by water films adsorbed at grain-grain contacts, as opposed to global viscous dampening or attenuation within the grains [Citation57]. Although this application has yet to be implemented in robotics, it may be a worthwhile consideration for certain mechanical systems with specific requirements.
3.3. Body structure for robots
Elastogranular mechanics [Citation58] can be utilised for shape adaptation and locomotion of robotic bodies. Already before the arrival of jamming grippers, granular material has been proposed as content for robotic skin. A purely soft and liquid-filled robot [Citation59,Citation60], can alter its exterior shape to induce rolling motion by controlling the jamming of the granular segments. The material can even serve ass a backbone for robots [Citation28], if the parts are connected by spherical joints. Additionally, it also has been incorporated into wings for the changeable stiffness for morphing aerostructures [Citation61]. Despite granular material is less commonly used in robotic bodies than in end-effectors, partitioning control of granular material represents a promising method for joint-like components in various robotic scenarios.
3.4. Granular material for sensing
The human inner ear contains otoconia (analogous to otoliths in other animals), a fine granular material embedded in a gel matrix, that is coupled to the hair cells to transduce sound information to the midbrain. By an optimal choice of the properties of the transducer is it possible to achieve noise reduction at the same time as amplification of interesting frequency ranges and combinations.
Currently, the research on the manipulation capability of granular material is relatively mature while few applications possess sensing capabilities. Stress measurements for continuous and deformable media like granular material are typically achieved through measuring deformation. Current research mainly utilises optical and magnetic methods for signal transmission [Citation62]. The application of optical signals is used in haptic displays mentioned in previous chapters, where the deformation caused by external forces results in changes in reflected light beams that can be quantified to measure contact forces. In contrast, magnetic signals are measured using Hall sensors as receivers, which detect the displacement of permanent magnets with soft media deformation. Thanks to the support of machine learning algorithms, perceptual measurements of continuous soft media [Citation63] have achieved considerable accuracy now and are being wider used in this field.
In recent research, the skin of the fingertips is formed by mixing liquid silica gel [Citation64] and permanent magnetic particles in a beaker at equal mass ratio and solidifying them with permanent magnets. Particle silicone skin, the sensor is at the root of the finger and can more accurately measure the position and strength of the contact. The thickness of the magnetic skin layer may have an impact on the performance of jamming when grasping extremely irregular objects, but this design basically realises the simulation of human fingers grasping and touching objects.
3.5. Interaction devices
The inherent deformability arising from the flowability of granular material and tunable stiffness due to jamming are useful for the generation of tactile information in interaction devices, which can be based on pneumatic or hydraulic jamming media [Citation65]. The detection of deformation can rely on optical and capacitive sensing.
The two-dimensional haptic jamming display [Citation66] was meant to illustrate the function of a tactile interaction devices. Later the number of independently controllable cells increased to 12 by using a embedded vacuum lines structure to improve the geometric resolution [Citation67]. The focus shifted then to closed-loop control [Citation68] and recently algorithmic support was provided by experiments adapting the device output to a desired shape [Citation69].
Two-dimensional haptic displays can provide tactile feedback in medicine [Citation70], and were also assessed from the perspective of user adaptation [Citation71]. In medical research, the focus has been on practical multi-finger palpation [Citation72] with independent multiple cells to better simulate tissue stiffness and investigate the experiential differences of tactile feedback with different numbers of fingers. This type of application is usually fixed to larger interaction devices, such as KineSys MedSim [Citation73], a cable-driven platform of the kinesthetic system for medical simulation. Current research explores how three-dimensional systems can fully utilise tactile interaction capabilities in a portable setting.
An early design of a three-dimensional tactile display, the HoverMesh [Citation74], was based on a mechanical structure linked by prismatic joints. Other studies have utilised the shape design of a spherical granular jamming gripper for interaction [Citation75] or directly used the spherical component without any other parts [Citation76]. This type of design may find applications in advanced medical palpation, such as to mimic the shape and mechanical properties of organs to achieve a fully immersive experience shapes and elasticity [Citation77]. Recent haptic shape displays have mainly focused on shape design and embedded surface sensing control algorithms [Citation78] to achieve more precise shape matching and more effective closed-loop interaction.
The use of particles in wearable devices predates jamming technology. Initially, these devices consisted of deformable soft vinyl tubes worn on the arms to provide a sensation of contacting a wall or moving in water with viscosity [Citation79]. To address the weight issue of large wearable devices, lighter particle material like Styrofoam beads are commonly used. In order to combine precise tactile feedback and joint stabilisation, clothes with variable stiffness [Citation80], upper-limb orthotics that constrain shoulder and elbow movements [Citation81], and robotic exoskeletons [Citation82] were tested.
In Ref. [Citation83] inspiration from octopus suckers is taken for the design of a multiple-holed surface to generate a shape-adaptive structure that serves a the robot as an anchor ball. Jamming-based adjustable stiffness enables the ball to stick to surfaces providing support for a robot that moves towards and from the anchor by a fluid-powered ropeway to navigate in cluttered environments.
Recent research has aimed for more nuanced sensation expression, such as for gloves [Citation84] as tactile interaction devices. A versatile sensing gripper [Citation85] uses transparent granular material to enable optical sensing. In this way the deformation of the membrane can be detected and also the object being grasped can be identified. Recently, this research was shown to perform precise assembly operations including re-grasping and pose correction which is serviceable in general-purpose assembly using robots [Citation86].
4. Granular environments
4.1. Legged robots on granular environments
The difficulty of moving on granular media arises not only from the unevenness of the ground but also from the fluidity of the granular environment under pressure, making it difficult to achieve stable support for the movement [Citation87]. Legged robots have demonstrated a distinct advantage in such environments compared to their wheeled counterparts (compare also Exodus 14:25a), but achieving the same level of mobility on solid ground is still quite challenging. Following the structure outlined in Table , we will review here current research on legged and biomimetic robotic locomotion in granular media.
Table 1. Overview of challenges for robots in granular environments (see Ch. 4).
The design of the foot is the most important part for legged robots walking on loose granular media. The fluidity of the granules makes it difficult to stabilise the walking motion, and feet sinking into the granular medium can also be a problem that creates additional resistance. In bipedal robots, larger footprints can result in more stable walking, see Figure . Based on research on granular reaction forces, dynamic bipedal walking has been achieved on deformable terrain dry granular media [Citation39]. Using soft granular material in feet may improve walking performance on soft media [Citation45].
Figure 2. Time-lapse photograph of a planar biped walking on a bed of poppy seeds, an experimental surrogate for granular terrain. The robot walked at 10 cm/s. Photo credit: Rob Felt, GaTech, see also Ref. [Citation39].
![Figure 2. Time-lapse photograph of a planar biped walking on a bed of poppy seeds, an experimental surrogate for granular terrain. The robot walked at 10 cm/s. Photo credit: Rob Felt, GaTech, see also Ref. [Citation39].](/cms/asset/a10d3c21-1d0f-4005-a1e8-a438f2743f66/tadr_a_2348544_f0002_oc.jpg)
A popular animal model is the zebra-tailed lizard [Citation88], whose paws have a natural advantage for walking in the sand. This served as an inspiration for the design of robots with six C-shaped legs [Citation89–91]. This type of leg can be seen as a compromise between easily controllable wheel and legs, known as whegs. On granular material, this chimera proves useful. During the off-duty phase, each limb is removed from the granular material, and during the duty phase the flat shape sinks in less than a pointed leg, while the robot can retain good flexibility and excellent speed. However, the animal original can achieve the same benefits by exerting a considerably lower pressure on the surface [Citation92].
In addition to standard walking, legged robots can also move by hopping. A brief contact causes the granular ground to jam [Citation93], providing controllable support momentarily. Relevant research proposed the model for motion planning of this locomotion mechanism and analysed various considerations in the design of hopping movements on soft ground [Citation94]. Subsequent research has focused on reducing the depth of penetration upon landing in order to minimise energy loss by a legged robot impacting yielding terrain [Citation95]. Currently, this hopping mode of locomotion can be considered a controlled movement on granular media.
4.2. Terrestrial swimming
Quite opposite to jamming, vibrations can lead to a liquefaction of the ground which tends to facilitate penetration [Citation96]. In some animals this has been traditionally observed as a kind of swimming. [Citation97] To avoid complications due to foot penetration, limbless robots were designed to move on granular media [Citation98] mimicking the way snakes travel, see Figure . Snake-like movement patterns were also studied [Citation99] as well as the way sea turtles move on the beach [Citation100].
Figure 3. Javelin sand boa (Eryx jaculus) moving on granular matter (Image taken on Pylos (Peloponnese/Greece) by Benny Trapp, CC BY-SA 3.0.)
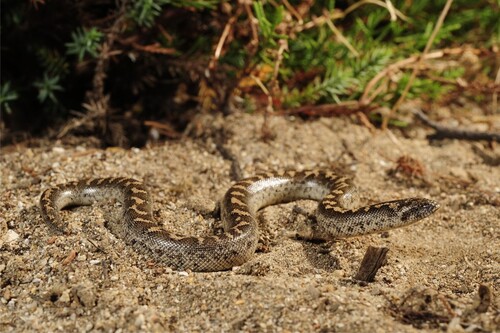
Sandfish (scincus scincus) or skinks are scaled reptiles that use swimming patterns for movement on sand surfaces [Citation101] Their movement in the desert was studied using MRI, 3D laser scanning studied the body shape of the sandfish and its movement in the loose desert was explored using fast nuclear magnetic resonance (NMR) imaging. Local decomposition of sand around moving skinks is likely. Sand thus behaves locally as a viscous fluid rather than as a solid material. In this fluidised sand, skinks can ‘swim’ with their limbs (Figure ). As the animals swam deeper into the material, the intensity of muscle activation increased, but did not change with the frequency of fluctuations, because the resistance of the material increases with depth but not with speed.
Figure 4. Sandfish (scincus scincus) ‘swimming’ on granular matter. (Image by The Great Mule of Eupatoria, CC BY-SA 3.0.)
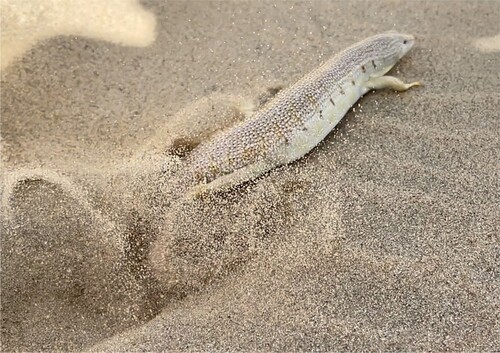
Other papers have studied the effects of the environmental conditions [Citation102], movement patterns for subsurface mobility [Citation103,Citation104], as well as the skink biomechanics [Citation105]. The same team has also produced and investigated a robotic sandfish model [Citation106].
4.3. Movement within granular material
A very interesting view [Citation107] on the broader implications of coping with the instability of granular media, is taken with a focus on various snake locomotion behaviours (in addition to skinks), which can provide insights into the co-evolution of neuromechanical control templates with morphology and behaviour, see also [Citation108]. The suggestive earthworm approach to subterranean movement can be realised by peristaltic soft actuators [Citation109].
In order to move through granular matter, the fluidisation effect can be used to reduce the resistive forces of the environmental material. It can be enabled by passing pressurised water or airflow [Citation110] through the material, which can be applied to create a conduit for an electrical line. The robot can steer horizontally and vertically [Citation111], and the pullout is nearly drag-free, because of the invertability of its outer skin, solving a problem the was discussed for plant-like root systems [Citation112].
A similar burrowing effect is realised by razor clams (Ensis directus) [Citation113] by opening and closing their shell while providing anchorage with the muscular foot in order to break the symmetry between downwards and upwards motion. This mechanism has inspired a simulated model system that uses cavity expansion theory and discrete elements to study burrowing in dry sand [Citation114]. A related approach treats the particles as a flow medium, like a hydraulic pump, and actuates them by changing the internal volume of the container [Citation115].
Polychaetes, a class of annelids, are bristled worms that have been modelled by a soft-robot system in order to explore its capability of locomotion in granular substrates [Citation116]. Other options for locomotion in granular media is the use of compliant fins [Citation117], or flagella [Citation118]
5. Design of granular material
5.1. Wet granular material and suspensions
Humidity and surface tension can make the surface of particles adsorb certain water molecules to produce a pendular state [Citation119], resulting in a cohesive attraction or adhesion force between particles. [Citation120,Citation121]. To study this theoretically, it is an option to use a discrete element method in order to determine the properties of wet granular material [Citation122]. For example the shear modulus of wet granular matter [Citation123], which should the interesting result that optimal strength is achieved at very low liquid volume fractions of 1–3%.
When granular material such as sand is mixed with a certain amount of liquid, the surface tension of the latter imparts considerable stiffness to the material [Citation124], which makes it possible to build sandcastles.
Mechanical and geometrical properties of jammed wet granular material [Citation125] numerically investigates the mechanical and geometric properties of dense wet particles with irreversible attractive interactions. Interstitial liquid can also be used in hydraulic jamming grippers. This is a promising research area that may generate a significant increase in holding force [Citation126]. Hydraulics can help to achieve a higher stiffness, silent operation, and faster actuation. [Citation65]
5.2. Customisation of controllable particles
By customising particles it is possible to design properties of the material to realise an improved functionality, such as controllability or robustness. The customisation relies on the solution of the inverse problems of producing particles towards specified material properties of the granular matter. The artificial evolution of particular towards a fitness function specifying the granular matter properties seems natural and, given the statistical variability within good solutions, also realistic. Successful particle design still leaves the necessity to actually produce them. Of particular interest is the substitution of metal components for granular material of similar properties.
One way to affect the material properties flexibly during use is magnetic control [Citation36] which, however, needs to be optimised by the design of the particles in order to be efficient. Computational approaches helped to design non-trivial particle shapes in order to affect force chain network, contact number, and pressure evolution of jamming systems [Citation127].
Such a similar graph-based description of the interaction dynamics of a material and the rigid bodies to be manipulated, can be represented by graph neural networks [Citation128]. Minimise the distance between a predicted distribution of particles and a desired configuration allows the planning of operational trajectories to achieve the desired material configuration. Learning-based approaches may benefit from data, e.g. relating grip strength and shock absorption: [Citation129]. Although other methods may be preferable in many applications, the production of designed particles is largely dependent on 3D printing, which can produce different types of particles [Citation130], although it is known there is no generally optimal set of particle, e.g. for jamming grippers [Citation131].
5.3. Particle-like robots: programmable matter or gray goo?
Ultimately, it is imaginable to have robotic abilities at the level of particles, which in fact was discussed in the field of programmable matter already near the end of the previous century [Citation132,Citation133] or wrapped particles [Citation134], or the robots act as the particles [Citation135]. Despite the theoretical analysis, these applications had not been tested in practical problems. Whether the robots can function as intelligent ‘particles’ with sufficiently simple structures remains an area that warrants further investigation.
More recently, earlier ideas have found interest in the contact of cyber-physical systems, such as utility fog [Citation136], smart matter [Citation137], or even dust systems for wind dispersal [Citation138,Citation139]. Self-reproducibility was seen as a main danger of nanomaterials, but many other risks can neither be excluded today, such as non-consensual surveillance, or allergic or intolerance reactions or other forms of biological incompatibility in all life forms, which is amplified if a large number of such devices is used.
6. Perspectives
The advent of the jamming gripper was not predicted by researchers in the community. Also in future the field will benefit from surprises, breakthroughs, and serendipity. We will nevertheless ask the following questions: What developments can be realistically expected for robotics from granular matter research in the next decade? What questions are waiting for an answer today? What progress do we hope to see in future?
6.1. Perspectives for robotics
The flowability of granular material enables them to assume a wide range of shapes which consequently supports a large variety of applications. The jamming transition can also be used in the membrane design itself. For example a layer of powder between an inner rubber core and an outer rubber membrane increases the safety when handling fragile objects [Citation140]. In addition to expanding the range of forms available to robot manipulators, the fixation effect and sensory simulation capabilities afforded by jamming render it highly suitable for use in wearable devices. The potential of morphological design includes adjustable wearable exoskeletons for shaping or devices that simulate various levels of tactile feedback in virtual reality games. Furthermore, it can be expected to be the external auxiliary device for robots to introduce a kind of wariness.
Recent studies have increasingly focused on simulating the shape of objects to achieve specific effects, such as tactile granular fingertips [Citation64] that fully imitate human finger shape, attempting to perform any operation like real human fingers. For more accurate tactile displays in medical applications, granular material can be fashioned into organ shapes with greater precision than using ellipsoidal templates [Citation77]. Theoretically, any component with variable stiffness could potentially be implemented using granular material, provided that the benefits of this approach are considered. The imitation of specific structures will make them more specialised for certain tasks.
6.2. Current trends
The manifest mathematical approach to granular matter, particularly of use in simulations is geometric mechanics. The large-scale behaviour can be understood in terms of statistical mechanics, while experimental work is the key to provide insight into the large variety of observable behaviour.
Biological granular matter complex geometries have evolved that shape every single one of a relatively small number of particles (e.g. tarsal bones). In order to mimic functional links and joints of a hand, but also more generally to generate a certain function, non-isotropic material can turn out to be advantageous, once form-function relations are better understood.
The inverse problem is the design of granular material, so that desired observations can be produced by a suitable choice of particle properties [Citation141]. We expect here a wealth of new results in near future, as earlier work was mainly limited to simulations.
Not all applications benefit in the same way from the complexity of granular matter. For example, the pressure profiles (see e.g. [Citation142,Citation143], stalks, roots, tubers, bulbs, or fruit; interaction with large numbers of farm animals, breeding of edible insects; or precision irrigation, fertilisation, and melioration. Similarly, applications will be found in construction and underground engineering, mining in terrestrial pits as well as perspectively on planetary missions and repair of streets and civic structures. Biorobotics and prosthetics posed a further set of interesting challenges. Waste management is dealing with granular material of high complexity and may offer the chance of surprising use cases [Citation144].
6.3. Open questions
Among the many open questions related to granular material, we want to focus only on a few ones that are particularly burning for applications in robotics. Jamming effects depend largely on friction and related small-scale properties of the material that, however, may degrade when being used over long periods. Although resetting procedures may help [Citation145], more work on the resilience and regeneration of granular material is clearly needed.
Physics approaches often assume a separation of scales, i.e. the number of particles in granular matter is assumed to be very large, so that random effects average out and a clear description of the material properties becomes possible. Successful engineering applications, on the other hand, work with a few components, such as ball bearings. The question is thus not only how much material is needed and how many layers of particles are implied by a desired function, but also how granular material are to be understood: Is granular material a complex contraption where every piece plays its prescribed role, or is the idea of a highly redundant bulk behaviour already sufficient for the realisation of applications?
Will we be able to customise granular material, either per application type, per application case and ultimately down to the level of single particles?
Robots are mostly made of steel and plastics. To what extent can granular material substitute such components? Is it possible to control reliably the switching between damping and actuation? Can morphological designs of robots incorporate flexible partitioning of the body into still components and joints? Different modes of the material will require complex control methods in order to realise particular functions based on the variety of behaviours offered by the material.
6.4. Outlook
While we know already about wet granular matter, much can be learned and gained from experiments with magnetic materials, from interactions of polarisable particles via van der Waals forces, complex effects of surface tension, cohesion, which become even more fascinating, if nanoscale processes are relevant.
We have mentioned a few examples from biology such as the use of granular material for sensing, damping, and processing of food, as well as the active interaction with granular material in the environment in fish, skinks, antlions, and many others. There is still much to learn from the locomotion behaviours of animals on granular matter, from the complexity of biological materials and their regenerative properties.
While granular material has shown clear benefits in mechanical interaction with the environment, other benefits can also be explored. Granular material could consist of particles with adaptive or regenerating shapes to increase flexibility and robustness against wear. One could imagine robots that harvest energy from the environment by suitable processes within layers of granular material that utilise heat, pressure, or vibrations. Although, we have decided not to focus too much on smart matter here, this research area where particles are controllable or in control of their behaviour is still very promising for the future of the field.
The rich repertoire of behaviours that gives rise to the fascinating perspective of granular matter for robotics requires more theoretical and applied research. Granular material in robotics could be thought of as a form of programmable matter that has so far not shown success at the same level as predicted over the last three decades. We have aimed to show here that physical materials can be of high interest already without control at the material level, although an adaptive high-level control will in many cases be required.
Disclosure statement
No potential conflict of interest was reported by the author(s).
Additional information
Funding
Notes on contributors
Han Li
Han Li is a researcher with experience as a Research Assistant at the University of Edinburgh. Holding a Master's degree in Intelligent Robotics from the University of York and an additional Master's degree in Informatics from the University of Edinburgh, he possesses a comprehensive understanding of robotics and artificial intelligence. Specializing in embodied intelligence, human-robot interaction, and reinforcement learning, his research strives to advance the development of various intelligent robots. Han's professional ambition is to foster the creation of more general artificial intelligence in robotics and make emotionally intelligent robots accessible to a wider audience.
Jin Sun
Jin Sun is Professor of Granular Mechanics in the James Watt School of Engineering at the University of Glasgow from 2023. He graduated from Iowa State University in 2007 with a PhD in Mechanical and Chemical Engineering and did postdoctoral research at Princeton University until he joined the University of Edinburgh in 2010. His main research interests lie in rheology and flow of dense granular materials in industrial and natural environments, such as manufacturing of ceramics, food stuff, battery electrodes and pharmaceuticals, and debris and pyroclastic flows. His recent focus has been on design of granular materials with targeted rheological properties for applications in robotics and bioengineering. He has been principal investigator of projects funded by the UK national research councils including EPSRC and NERC; and co-investigator on NERC and EU projects. He has been awarded a Royal Academy of Engineering/Leverhulme Trust Senior Research followship.
J. Michael Herrmann
J. Michael Herrmann is a Lecturer for Robotics in the School of Informatics at the University of Edinburgh. He graduated from Leipzig University in 1993 with a PhD in Informatics and did postdoctoral work in Copenhagen and Tokyo. He was an assistant professor in Göttingen, and joined the University of Edinburgh in 2008. His research interests include computational neuroscience, machine learning, biologically inspired computing, and robotics.
References
- Fitzgerald SG, Delaney GW, Howard D. A review of jamming actuation in soft robotics. Actuators. 2020;9(4):104. doi: 10.3390/act9040104
- Bagnold RA. The physics of blown sand and desert dunes. London: Methuen; 1941.
- Deresiewicz H. Mechanics of granular matter. Adv Appl Mech. 1958;5:233–306. doi: 10.1016/S0065-2156(08)70021-8
- Schofield AN, Wroth P. Critical state soil mechanics. Vol. 310. London: McGraw-hill; 1968.
- Rosato A, Strandburg KJ, Prinz F, et al. Why the Brazil nuts are on top: size segregation of particulate matter by shaking. Phys Rev Lett. 1987;58(10):1038–1040. doi: 10.1103/PhysRevLett.58.1038
- Savage SB. The mechanics of rapid granular flows. Adv Appl Mech. 1984;24:289–366. doi: 10.1016/S0065-2156(08)70047-4
- Perovskii AP. Universal grippers for industrial robots. Russ Eng J. 1980;60(8):3–4. [not available online].
- Brown E, Rodenberg N, Amend J, et al. Universal robotic gripper based on the jamming of granular material. Proc Natl Acad Sci. 2010;107(44):18809–18814. doi: 10.1073/pnas.1003250107
- Schmidt I. Flexible moulding jaws for grippers. Ind Robot An Int J. 1978;5(1):24–26. doi: 10.1108/eb004491
- Rienmüller T, Weissmantel H. A shape adaptive gripper finger for robots. In: 18. International Symposium on Industrial Robots; 1988. p. 241–250.
- Nishida T, Shigehisa D, Kawashima N, et al. Development of universal jamming gripper with a force feedback mechanism. In: 2014 Joint 7th International Conference on Soft Computing and Intelligent Systems (SCIS) and 15th International Symposium on Advanced Intelligent Systems (ISIS); IEEE; 2014. p. 242–246.
- Meuleman S, Balt V, Jarray A, et al. Investigation of particle properties on the holding force in a granular gripper. In: PARTICLES V, Proceedings of the V-th International Conference on Particle-Based Methods, Fundamentals and applications; CIMNE; 2017. p. 588–599.
- Wang Y, Yang Z, Zhou H, et al. Inflatable particle-jammed robotic gripper based on integration of positive pressure and partial filling. Soft Robot. 2022;9(2):309–323. doi: 10.1089/soro.2020.0139
- Bartkowski P, Suwała G, Zalewski R. Temperature and strain rate effects of jammed granular systems: experiments and modelling. Granul Matter. 2021;23(4):79. doi: 10.1007/s10035-021-01138-x
- Coombe C, Brett J, Mishra R, et al. Active vibration fluidization for granular jamming grippers; 2022. Preprint arXiv:2212.06498.
- Al Abeach L, Nefti-Meziani S, Theodoridis T, et al. A variable stiffness soft gripper using granular jamming and biologically inspired pneumatic muscles. J Bionic Eng. 2018;15(2):236–246. doi: 10.1007/s42235-018-0018-8
- Li Y, Chen Y, Yang Y, et al. Passive particle jamming and its stiffening of soft robotic grippers. IEEE Trans Robot. 2017;33(2):446–455. doi: 10.1109/TRO.2016.2636899
- Li Y, Chen Y, Li Y. Distributed design of passive particle jamming based soft grippers. In: 2018 IEEE International Conference on Soft Robotics (RoboSoft); IEEE; 2018. p. 547–552.
- Ranzani T, Cianchetti M, Gerboni G, et al. A soft modular manipulator for minimally invasive surgery: design and characterization of a single module. IEEE Trans Robot. 2016;32(1):187–200. doi: 10.1109/TRO.2015.2507160
- Wei Y, Chen Y, Ren T, et al. A novel, variable stiffness robotic gripper based on integrated soft actuating and particle jamming. Soft Robot. 2016;3(3):134–143. doi: 10.1089/soro.2016.0027
- Jiang Y, Chen D, Liu C, et al. Chain-like granular jamming: a novel stiffness-programmable mechanism for soft robotics. Soft Robot. 2019;6(1):118–132. doi: 10.1089/soro.2018.0005
- Yang Y, Zhang Y, Kan Z, et al. Hybrid jamming for bioinspired soft robotic fingers. Soft Robot. 2020;7(3):292–308. doi: 10.1089/soro.2019.0093
- Wall V, Deimel R, Brock O. Selective stiffening of soft actuators based on jamming. In: 2015 IEEE International Conference on Robotics and Automation (ICRA); IEEE; 2015. p. 252–257.
- Zhao Y, Shan Y, Zhang J, et al. A soft continuum robot, with a large variable-stiffness range, based on jamming. Bioinspir Biomim. 2019;14(6):066007. doi: 10.1088/1748-3190/ab3d1b
- Ruotolo W, Roig FS, Cutkosky MR. Load-sharing in soft and spiny paws for a large climbing robot. IEEE Robot Automat Lett. 2019;4(2):1439–1446. doi: 10.1109/LSP.2016.
- Harada K, Nagata K, Rojas J, et al. Proposal of a shape adaptive gripper for robotic assembly tasks. Adv Robot. 2016;30(17-18):1186–1198. doi: 10.1080/01691864.2016.1209431
- Park W, Lee D, Bae J. A hybrid jamming structure combining granules and a chain structure for robotic applications. Soft Robot. 2022;9(4):669–679. doi: 10.1089/soro.2020.0209
- Wei Y, Chen Y, Yang Y, et al. A soft robotic spine with tunable stiffness based on integrated ball joint and particle jamming. Mechatronics. 2016;33:84–92. doi: 10.1016/j.mechatronics.2015.11.008
- Götz H, Santarossa A, Sack A, et al. Soft particles reinforce robotic grippers: robotic grippers based on granular jamming of soft particles. Granul Matter. 2022;24(1):1–9. doi: 10.1007/s10035-021-01137-y
- Jiang A, Xynogalas G, Dasgupta P, et al. Design of a variable stiffness flexible manipulator with composite granular jamming and membrane coupling. In: 2012 IEEE/RSJ International Conference on Intelligent Robots and Systems; IEEE; 2012. p. 2922–2927.
- Li Y, Chen Y, Yang Y, et al. Soft robotic grippers based on particle transmission. IEEE ASME Trans Mechatron. 2019;24(3):969–978. doi: 10.1109/TMECH.3516
- Zhou J, Chen Y, Hu Y, et al. Adaptive variable stiffness particle phalange for robust and durable robotic grasping. Soft Robot. 2020;7(6):743–757. doi: 10.1089/soro.2019.0089
- Jiang A, Adejokun S, Faragasso A, et al. The granular jamming integrated actuator. In: 2014 International Conference on Advanced Robotics and Intelligent Systems (ARIS); IEEE; 2014. p. 12–17.
- Loeve AJ, van de Ven OS, Vogel JG, et al. Vacuum packed particles as flexible endoscope guides with controllable rigidity. Granul Matter. 2010;12(6):543–554. doi: 10.1007/s10035-010-0193-8
- Cianchetti M, Ranzani T, Gerboni G, et al. Stiff-flop surgical manipulator: mechanical design and experimental characterization of the single module. In: 2013 IEEE/RSJ International Conference on Intelligent Robots and Systems; IEEE; 2013. p. 3576–3581.
- Cavallo A, Brancadoro M, Tognarelli S, et al. A soft retraction system for surgery based on ferromagnetic materials and granular jamming. Soft Robot. 2019;6(2):161–173. doi: 10.1089/soro.2018.0014
- Cheng NG, Lobovsky MB, Keating SJ, et al. Design and analysis of a robust, low-cost, highly articulated manipulator enabled by jamming of granular media. In: 2012 IEEE International Conference on Robotics and Automation; IEEE; 2012. p. 4328–4333.
- Steltz E, Mozeika A, Rembisz J, et al. Jamming as an enabling technology for soft robotics. In: Electroactive Polymer Actuators and Devices (EAPAD) 2010; Vol. 7642; Spie; 2010. p. 640–648.
- Xiong X, Ames AD, Goldman DI. A stability region criterion for flat-footed bipedal walking on deformable granular terrain. In: 2017 IEEE/RSJ International Conference on Intelligent Robots and Systems (IROS); IEEE; 2017. p. 4552–4559.
- Fujita M, Ikeda S, Fujimoto T, et al. Development of universal vacuum gripper for wall-climbing robot. Adv Robot. 2018;32(6):283–296. doi: 10.1080/01691864.2018.1447238
- Hauser S, Mutlu M, Freundler F, et al. Stiffness variability in jamming of compliant granules and a case study application in climbing vertical shafts. In: 2018 IEEE International Conference on Robotics and Automation (ICRA); IEEE; 2018. p. 1559–1566.
- Li L, Liu Z, Zhou M, et al. Flexible adhesion control by modulating backing stiffness based on jamming of granular materials. Smart Mater Struct. 2019;28(11):115023. doi: 10.1088/1361-665X/ab46f3
- Hauser S, Eckert P, Tuleu A, et al. Friction and damping of a compliant foot based on granular jamming for legged robots. In: 2016 6th IEEE International Conference on Biomedical Robotics and Biomechatronics (BioRob); IEEE; 2016. p. 1160–1165.
- Hauser S, Mutlu M, Banzet P, et al. Compliant universal grippers as adaptive feet in legged robots. Adv Robot. 2018;32(15):825–836. doi: 10.1080/01691864.2018.1496851
- Chopra S, Tolley MT, Gravish N. Granular jamming feet enable improved foot-ground interactions for robot mobility on deformable ground. IEEE Robot Automat Lett. 2020;5(3):3975–3981. doi: 10.1109/LSP.2016.
- Zalewski R, Szmidt T. Application of special granular structures for semi-active damping of lateral beam vibrations. Eng Struct. 2014;65:13–20. doi: 10.1016/j.engstruct.2014.01.035
- Makowski M, Zalewski R. Vibration analysis for vehicle with vacuum packed particles suspension. J Theor Appl Mech. 2015;53(1):109–117. doi: 10.15632/jtam-pl.53.1.109
- Zalewski R, Chodkiewicz P, Shillor M. Vibrations of a mass-spring system using a granular-material damper. Appl Math Model. 2016;40(17-18):8033–8047. doi: 10.1016/j.apm.2016.03.053
- Sternberger A, Génevaux JM, Pelat A. Experimental analysis of the vibration dissipation induced by granular materials included into a 1 degree of freedom oscillator. Granul Matter. 2019;21(3):57. doi: 10.1007/s10035-019-0905-7
- Varela-Rosales NR, Santarossa A, Engel M, et al. Granular binary mixtures improve energy dissipation efficiency of granular dampers; 2023. Preprint arXiv:2302.03069.
- Żurawski M, Zalewski R. Experimental studies on adaptive-passive symmetrical granular damper operation. Materials. 2022;15(17):6170. doi: 10.3390/ma15176170
- Rodak D, Zalewski R. Innovative controllable torsional damper based on vacuum packed particles. Materials. 2020;13(19):4356. doi: 10.3390/ma13194356
- Bartkowski P, Bukowiecki H, Gawiński F, et al. Adaptive crash energy absorber based on a granular jamming mechanism. Bull Polish Acad Sci: Tech Sci. 2022;70(1):e139002–e139002.
- Voronina NN, Horoshenkov KV. A new empirical model for the acoustic properties of loose granular media. Appl Acoust. 2003;64(4):415–432. doi: 10.1016/S0003-682X(02)00105-6
- Hsu CJ, Johnson DL, Ingale RA, et al. Dynamic effective mass of granular media. Phys Rev Lett. 2009;102(5):058001. doi: 10.1103/PhysRevLett.102.058001
- Brunet T, Jia X, Mills P. Mechanisms for acoustic absorption in dry and weakly wet granular media. Phys Rev Lett. 2008;101(13):138001. doi: 10.1103/PhysRevLett.101.138001
- Valenza J, Hsu CJ, Ingale R, et al. Dynamic effective mass of granular media and the attenuation of structure-borne sound. Phys Rev E. 2009;80(5):051304. doi: 10.1103/PhysRevE.80.051304
- Schunter Jr DJ, Brandenbourger M, Perriseau S, et al. Elastogranular mechanics: buckling, jamming, and structure formation. Phys Rev Lett. 2018;120(7):078002. doi: 10.1103/PhysRevLett.120.078002
- Steltz E, Mozeika A, Rodenberg N, et al. Jamming skin enabled locomotion. In: 2009 IEEE/RSJ International Conference on Intelligent Robots and Systems; IEEE; 2009. p. 5672–5677.
- Mozeika A, Steltz E, Jaeger HM. The first steps of a robot based on jamming skin enabled locomotion. In: 2009 IEEE/RSJ International Conference on Intelligent Robots and Systems; IEEE; 2009. p. 408–409.
- Brigido-González JD, Burrow SG, Woods BKS. Switchable stiffness morphing aerostructures based on granular jamming. J Intell Mater Syst Struct. 2019;30(17):2581–2594. doi: 10.1177/1045389X19862372
- Wang H, Totaro M, Beccai L. Progress and challenges. Adv Sci. 2018;5(9):1800541. doi: 10.1002/advs.v5.9
- Mohammadi A, Xu Y, Tan Y, et al. Magnetic-based soft tactile sensors with deformable continuous force transfer medium for resolving contact locations in robotic grasping and manipulation. Sensors. 2019;19(22):4925. doi: 10.3390/s19224925
- Fang B, Xia Z, Sun F, et al. Soft magnetic fingertip with particle jamming structure for tactile perception and grasping. IEEE Trans Ind Electron. 2022;70(6):6027–6035. doi: 10.1109/TIE.2022.3201305
- Follmer S, Leithinger D, Olwal A, et al. Jamming user interfaces: programmable particle stiffness and sensing for malleable and shape-changing devices. In: Proceedings of the 25th Annual ACM Symposium on User Interface Software and Technology; 2012. p. 519–528.
- Stanley AA, Gwilliam JC, Okamura AM. Haptic jamming: a deformable geometry, variable stiffness tactile display using pneumatics and particle jamming. In: 2013 World Haptics Conference (WHC); IEEE; 2013. p. 25–30.
- Stanley AA, Okamura AM. Controllable surface haptics via particle jamming and pneumatics. IEEE Trans Haptics. 2015;8(1):20–30. doi: 10.1109/TOH.2015.2391093
- Stanley AA, Hata K, Okamura AM. Closed-loop shape control of a haptic jamming deformable surface. In: 2016 IEEE International Conference on Robotics and Automation (ICRA); IEEE; 2016. p. 2718–2724.
- Stanley AA, Okamura AM. Deformable model-based methods for shape control of a haptic jamming surface. IEEE Trans Vis Comput Graph. 2016;23(2):1029–1041. doi: 10.1109/TVCG.2016.2525788
- Stanley AA, Gwilliam JC, Judkins TN, et al. A haptic display for medical simulation using particle jamming. In: Proc. Medicine Meets Virtual Reality; Vol. 20; 2013. p. 2.
- Genecov AM, Stanley AA, Okamura AM. Perception of a haptic jamming display: just noticeable differences in stiffness and geometry. In: 2014 IEEE Haptics Symposium; IEEE; 2014. p. 333–338.
- Li M, Ranzani T, Sareh S, et al. Multi-fingered haptic palpation utilizing granular jamming stiffness feedback actuators. Smart Mater Struct. 2014;23(9):095007. doi: 10.1088/0964-1726/23/9/095007
- Stanley AA, Mayhew D, Irwin R, et al. Integration of a particle jamming tactile display with a cable-driven parallel robot. In: Haptics: Neuroscience, Devices, Modeling, and Applications, 9th International Conference, EuroHaptics 2014, Versailles, France, June 24–26, 2014, Proceedings, Part II 9; Springer; 2014. p. 258–265.
- Mazzone A, Spagno C, Kunz A. The hovermesh: a deformable structure based on vacuum cells: new advances in the research of tangible user interfaces. In: Proceedings of the 2004 ACM SIGCHI International Conference on Advances in Computer Entertainment Technology; 2004. p. 187–193.
- Usevitch NS, Khanna R, Carrera RM, et al. End effector for a kinesthetic haptic device capable of displaying variable size and stiffness. In: Haptics: Perception, Devices, Control, and Applications, 10th International Conference, EuroHaptics 2016, London, UK, July 4–7, 2016, Proceedings, Part II 10; Springer; 2016. p. 363–372.
- Sikander S, Biswas P, Kulkarni P, et al. Concept development of fixed geometry tactile display using granular jamming. In: 2019 International Symposium on Medical Robotics (ISMR); IEEE; 2019. p. 1–4.
- He L, Herzig N, de Lusignan S, et al. Granular jamming based controllable organ design for abdominal palpation. In: 2018 40th Annual International Conference of the IEEE Engineering in Medicine and Biology Society (EMBC); IEEE; 2018. p. 2154–2157.
- Koehler M, Usevitch NS, Okamura AM. Model-based design of a soft 3-d haptic shape display. IEEE Trans Robot. 2020;36(3):613–628. doi: 10.1109/TRO.8860
- Mitsuda T, Kuge S, Wakabayashi M, et al. Wearable force display using a particle mechanical constraint. Presence. 2002;11(6):569–577. doi: 10.1162/105474602321050703
- Aihara N, Sato T, Koike H. Highly deformable interactive 3D surface display. In: Adjunct Proceedings of the 25th Annual ACM Symposium on User Interface Software and Technology; 2012. p. 91–92.
- Mitsuda T, Kuge S, Wakabayashi M, et al. Wearable haptic display by the use of a particle mechanical constraint. In: Proceedings 10th Symposium on Haptic Interfaces for Virtual Environment and Teleoperator Systems. HAPTICS 2002; IEEE; 2002. p. 153–158.
- Thompson-Bean E, Steiner O, McDaid A. A soft robotic exoskeleton utilizing granular jamming. In: 2015 IEEE International Conference On Advanced Intelligent Mechatronics (AIM); IEEE; 2015. p. 165–170.
- Tsukagoshi H, Fuchigami K, Watari E, et al. Deformable anchor ball for thrown referring to octopus suckers. J Robot Mechatron. 2014;26(4):477–485. doi: 10.20965/jrm.2014.p0477
- Zubrycki I, Granosik G. Novel haptic device using jamming principle for providing kinaesthetic feedback in glove-based control interface. J Intell & Robot Syst. 2017;85(3-4):413–429. doi: 10.1007/s10846-016-0392-6
- Sakuma T, Von Drigalski F, Ding M, et al. A universal gripper using optical sensing to acquire tactile information and membrane deformation. In: 2018 IEEE/RSJ International Conference on Intelligent Robots and Systems (IROS); IEEE; 2018. p. 1–9.
- Sakuma T, Kiyokawa T, Matsubara T, et al. Jamming gripper-inspired soft jig for perceptive parts fixing. IEEE Access. 2023;11:62187–62199. doi: 10.1109/ACCESS.2023.3285810
- Nedderman RM. Statics and kinematics of granular materials. Vol. 352. Cambridge: Cambridge University Press; 1992.
- Li C, Hsieh ST, Goldman DI. Multi-functional foot use during running in the zebra-tailed lizard (callisaurus draconoides). J Experim Biol. 2012;215(22):4015–4033. doi: 10.1242/jeb.071837
- Li C, Umbanhowar PB, Komsuoglu H, et al. Sensitive dependence of the motion of a legged robot on granular media. Proc Natl Acad Sci. 2009;106(9):3029–3034. doi: 10.1073/pnas.0809095106
- Qian F, Daffon K, Zhang T, et al. An automated system for systematic testing of locomotion on heterogeneous granular media. In: Nature-inspired mobile robotics. World Scientific; 2013. p. 547–554.
- Li C, Zhang T, Goldman DI. A terradynamics of legged locomotion on granular media. Science. 2013;339(6126):1408–1412. doi: 10.1126/science.1229163
- Qian F, Zhang T, Korff W, et al. Principles of appendage design in robots and animals determining terradynamic performance on flowable ground. Bioinspir Biomim. 2015;10(5):056014. doi: 10.1088/1748-3190/10/5/056014
- Aguilar J, Goldman DI. Robophysical study of jumping dynamics on granular media. Nature Phys. 2016;12(3):278–283. doi: 10.1038/nphys3568
- Hubicki CM, Aguilar JJ, Goldman DI, et al. Tractable terrain-aware motion planning on granular media: an impulsive jumping study. In: 2016 IEEE/RSJ International Conference on Intelligent Robots and Systems (IROS); IEEE; 2016. p. 3887–3892.
- Lynch DJ, Lynch KM, Umbanhowar PB. The soft-landing problem: minimizing energy loss by a legged robot impacting yielding terrain. IEEE Robot Automat Lett. 2020;5(2):3658–3665. doi: 10.1109/LSP.2016.
- Darbois Texier B, Ibarra A, Melo F. Low-resistive vibratory penetration in granular media. PLoS ONE. 2017;12(4):e0175412. doi: 10.1371/journal.pone.0175412
- Chong B, Wang T, Erickson E, et al. Coordinating tiny limbs and long bodies: geometric mechanics of lizard terrestrial swimming. Proc Natl Acad Sci. 2022;119(27):e2118456119. doi: 10.1073/pnas.2118456119
- Marvi H, Gong C, Gravish N, et al. Sidewinding with minimal slip: snake and robot ascent of sandy slopes. Science. 2014;346(6206):224–229. doi: 10.1126/science.1255718
- Sharpe SS, Koehler SA, Kuckuk RM, et al. Locomotor benefits of being a slender and slick sand swimmer. J Experim Biol. 2015;218(3):440–450. doi: 10.1242/jeb.108357
- Mazouchova N, Umbanhowar PB, Goldman DI. Flipper-driven terrestrial locomotion of a sea turtle-inspired robot. Bioinspir Biomim. 2013;8(2):026007. doi: 10.1088/1748-3182/8/2/026007
- Sharpe SS, Ding Y, Goldman DI. Environmental interaction influences muscle activation strategy during sand-swimming in the sandfish lizard scincus scincus. J Experim Biol. 2013;216(2):260–274. doi: 10.1242/jeb.070482
- Bergmann PJ, Pettinelli KJ, Crockett ME, et al. It's just sand between the toes: how particle size and shape variation affect running performance and kinematics in a generalist lizard. J Experim Biol. 2017;220(20):3706–3716. doi: 10.1242/jeb.161109
- Maladen RD, Ding Y, Li C, et al. Undulatory swimming in sand: subsurface locomotion of the sandfish lizard. Science. 2009;325(5938):314–318. doi: 10.1126/science.1172490
- Maladen RD, Ding Y, Umbanhowar PB, et al. Mechanical models of sandfish locomotion reveal principles of high performance subsurface sand-swimming. J R Soc Interface. 2011;8(62):1332–1345. doi: 10.1098/rsif.2010.0678
- Ding Y, Sharpe SS, Masse A, et al. Mechanics of undulatory swimming in a frictional fluid. PLoS Comput Biol. 2012;8(12):e1002810. doi: 10.1371/journal.pcbi.1002810
- Maladen RD, Ding Y, Umbanhowar PB, et al. Undulatory swimming in sand: experimental and simulation studies of a robotic sandfish. Int J Rob Res. 2011;30(7):793–805. doi: 10.1177/0278364911402406
- Astley HC, Mendelson III JR, Dai J, et al. Surprising simplicities and syntheses in limbless self-propulsion in sand. J Experim Biol. 2020;223(5):103564. doi: 10.1242/jeb.103564
- Ding Y, Gravish N, Li C, et al. Comparative studies reveal principles of movement on and within granular media. In: Natural locomotion in fluids and on surfaces: swimming, flying, and sliding. Springer; 2012. p. 281–292.
- Das R, Babu SPM, Visentin F, et al. An earthworm-like modular soft robot for locomotion in multi-terrain environments. Sci Rep. 2023;13(1):1571. doi: 10.1038/s41598-023-28873-w
- Naclerio ND, Hubicki CM, Aydin YO, et al. Soft robotic burrowing device with tip-extension and granular fluidization. In: 2018 IEEE/RSJ International Conference on Intelligent Robots and Systems (IROS); IEEE; 2018. p. 5918–5923.
- Naclerio ND, Karsai A, Murray-Cooper M, et al. Controlling subterranean forces enables a fast, steerable, burrowing soft robot. Sci Robot. 2021;6(55):eabe2922. doi: 10.1126/scirobotics.abe2922
- Mickovski S, Bengough A, Bransby M, et al. Material stiffness, branching pattern and soil matric potential affect the pullout resistance of model root systems. Eur J Soil Sci. 2007;58(6):1471–1481. doi: 10.1111/ejs.2007.58.issue-6
- Tao JJ, Huang S, Tang Y. SBOR: a minimalistic soft self-burrowing-out robot inspired by razor clams. Bioinspir Biomim. 2020;15(5):055003. doi: 10.1088/1748-3190/ab8754
- Huang S, Tao J. Modeling clam-inspired burrowing in dry sand using cavity expansion theory and dem. Acta Geotechnica. 2020;15(8):2305–2326. doi: 10.1007/s11440-020-00918-8
- Fujita M, Tadakuma K, Takane E, et al. Variable inner volume mechanism for soft and robust gripping–improvement of gripping performance for large-object gripping. In: 2016 IEEE International Symposium on Safety, Security, and rescue robotics (SSRR); IEEE; 2016. p. 390–395.
- Ortiz D, Gravish N, Tolley MT. Soft robot actuation strategies for locomotion in granular substrates. IEEE Robot Autom Lett. 2019;4(3):2630–2636. doi: 10.1109/LSP.2016.
- Li D, Huang S, Tang Y, et al. Compliant fins for locomotion in granular media. IEEE Robot Automat Lett. 2021;6(3):5984–5991. doi: 10.1109/LRA.2021.3084877
- Du Y, Lam J, Sachanandani K, et al. Modeling the locomotion of articulated soft robots in granular medium. IEEE Robot Automat Lett. 2022;7(3):6495–6502. doi: 10.1109/LRA.2022.3173036
- Mitarai N, Nori F. Wet granular materials. Adv Phys. 2006;55(1-2):1–45. doi: 10.1080/00018730600626065
- Huang N, Ovarlez G, Bertrand F, et al. Flow of wet granular materials. Phys Rev Lett. 2005;94(2):028301. doi: 10.1103/PhysRevLett.94.028301
- Artoni R, Loro G, Richard P, et al. Drag in wet granular materials. Powder Technol. 2019;356:231–239. doi: 10.1016/j.powtec.2019.08.016
- Than VD, Khamseh S, Tang AM, et al. Basic mechanical properties of wet granular materials: a dem study. J Eng Mechan. 2017;143(1):C4016001.
- Møller PC, Bonn D. The shear modulus of wet granular matter. Europhys Lett. 2007;80(3):38002. doi: 10.1209/0295-5075/80/38002
- Scheel M, Seemann R, Brinkmann M, et al. Morphological clues to wet granular pile stability. Nat Mater. 2008;7(3):189–193. doi: 10.1038/nmat2117
- Yoshii K, Otsuki M. Mechanical and geometrical properties of jammed wet granular materials; 2022. Preprint arXiv:2209.04709.
- Amend J, Cheng N, Fakhouri S, et al. Soft robotics commercialization: jamming grippers from research to product. Soft Robot. 2016;3(4):213–222. doi: 10.1089/soro.2016.0021
- Bester CS, Zhao Y, Bares J, et al. Jamming transition in granular systems of regular polygons. In: APS March Meeting Abstracts; Vol. 2017; 2017. p. B14–007.
- Tuomainen N, Blanco-Mulero D, Kyrki V. Manipulation of granular materials by learning particle interactions. IEEE Robot Automat Lett. 2022;7(2):5663–5670. doi: 10.1109/LRA.2022.3158382
- Howard D, O'Connor J, Letchford J, et al. A comprehensive dataset of grains for granular jamming in soft robotics: grip strength and shock absorption; 2022. Preprint arXiv:2212.06511.
- Athanassiadis AG, Miskin MZ, Kaplan P, et al. Particle shape effects on the stress response of granular packings. Soft Matter. 2014;10(1):48–59. doi: 10.1039/C3SM52047A
- Howard D, O'Connor J, Brett J, et al. Shape, size, and fabrication effects in 3d printed granular jamming grippers. In: 2021 IEEE 4th International Conference on Soft Robotics (RoboSoft); IEEE; 2021. p. 458–464.
- Karimi MA, Alizadehyazdi V, Busque BP, et al. A boundary-constrained swarm robot with granular jamming. In: 2020 3rd IEEE International Conference on Soft Robotics (RoboSoft); IEEE; 2020. p. 291–296.
- Toffoli T, Margolus N. Programmable matter: concepts and realization. Phys D, Nonlinear Phenom. 1991;47(1-2):263–272. doi: 10.1016/0167-2789(91)90296-L
- Tanaka K, Karimi MA, Busque BP, et al. Cable-driven jamming of a boundary constrained soft robot. In: 2020 3rd IEEE International Conference on Soft Robotics (RoboSoft); IEEE; 2020. p. 852–857.
- Li S, Batra R, Brown D, et al. Particle robotics based on statistical mechanics of loosely coupled components. Nature. 2019;567(7748):361–365. doi: 10.1038/s41586-019-1022-9
- Nath SB, Gupta H, Chakraborty S, et al. A survey of fog computing and communication: current researches and future directions. arXiv preprint arXiv:180404365. 2018.
- Hai A. Smart matter: basic introduction and its potential in the future. In: 2020 5th International Conference on Information Science, Computer Technology and Transportation (ISCTT); IEEE; 2020. p. 148–152.
- Handy R, Richard O. Formulating the problems for environmental risk assessment of nanomaterials [Environmental science & technology]; 2007.
- Iyer V, Gaensbauer H, Daniel TL, et al. Wind dispersal of battery-free wireless devices. Nature. 2022;603(7901):427–433. doi: 10.1038/s41586-021-04363-9
- Fujita M, Tadakuma K, Komatsu H, et al. Jamming layered membrane gripper mechanism for grasping differently shaped-objects without excessive pushing force for search and rescue missions. Adv Robot. 2018;32(11):590–604. doi: 10.1080/01691864.2018.1451368
- Dierichs K, Menges A. Designing architectural materials: from granular form to functional granular material. Bioinspir Biomim. 2021;16(6):065010. doi: 10.1088/1748-3190/ac2987
- Conti L. Propulsion in granular media [Google patents]; 2023. US Patent 11,623,703.
- de Gennes PG. Granular matter: a tentative view. Rev Mod Phys. 1999;71(2):S374–S382. doi: 10.1103/RevModPhys.71.S374
- Roychand R, Kilmartin-Lynch S, Saberian M, et al. Transforming spent coffee grounds into a valuable resource for the enhancement of concrete strength. J Clean Prod. 2023;419:138205. doi: 10.1016/j.jclepro.2023.138205
- Amend JR, Brown E, Rodenberg N, et al. A positive pressure universal gripper based on the jamming of granular material. IEEE Trans Robot. 2012;28(2):341–350. doi: 10.1109/TRO.2011.2171093