Abstract
The content of sulfur in soils has been declining. Possible option to supply sulfur into the soil can be utilization of waste sulfur from biogas production. Two pot experiments with identical treatments were established to examine the effect of waste sulfur from biogas plant applied solely and in mixture with other nutrients on the growth and yield of oilseed rape. The included treatments were control, waste elemental sulfur (S), S + boron (B), S + B + humic substances (HS), S + B + HS + ammonium sulfate (AS), S + B + HS + ammonium nitrate (AN). The results from the first experiment showed significant increase in N-tester values and aboveground biomass after the treatments with S enhanced with both mineral fertilizers compared to any other treatments. The content of N and S in plants was also higher after these treatments. This was confirmed by second experiment. The seed yield was highest after the same treatments. The result from both experiments proved, that reutilization of waste S can be an interesting option. The application of sole waste S is a viable possibility. However, the mixture of sulfur with AS and AN is a more optimal alternative. Such combination could lead to better N use efficiency due to the co-application of N and S and also presents an interesting compromise to an overall lower consumption of mineral fertilizers (especially N) while adding more sulfur to the soil, especially in mixture with AS. This treatment resulted in the highest seed yield and production of oil in comparison with any other treatment.
Introduction
In the last decades, crops have often suffered from a lack of sulfur in the soil, as it has been reported by many authors (Feinberg et al. Citation2021; Kulhánek et al. Citation2016; Scherer Citation2009). The deficiency of S is a result of low consumption of conventional sulfur containing fertilizers and organic fertilizers, intensification of crop production yields mostly via low-S or S-free fertilizers like Urea, leaching sulfates out of the soil and especially decrease of air pollution. Since 1990, SO2 emissions in EU have been reduced by almost 80% (Degryse et al. Citation2016) and the sulfur deposition should further decrease until 2050 (Engardt et al. Citation2017). The atmospheric deposition of S in Czech Republic is about 5 kg ha−1 per year (CHMI Citation2019). Zbíral, Smatanová, and Němec (Citation2018) have described a highly statistically significant decrease of S in our soil from 33 mg kg−1 in year 1981 to average 8 mg kg−1 in year 2017 caused by the reduction of sulfur emissions. Similar decline in the atmospheric deposition of S from 100 kg ha−1 to 5-20 kg ha−1 per year in United Kingdom is described by Campbell and Smith (Citation1997). Therefore, it is crucial to fertilize by sulfur in addition to the other essential nutrients, especially because of the increased cultivation of high yielding crops (and cultivars) with high sulfur requirements (Zenda et al. Citation2021).
Sulfur has a key role in the synthesis of cysteine, methionine, and some vitamins (Schoenau and Sukhdev Citation2008). Sulfur is also necessary for the vegetative growth (Narayan et al. Citation2022). Plants take up sulfur from the soil solution, mainly as sulfate ion SO42−. Sulfur is commonly applied in the form of mineral fertilizers, and the co-application with nitrogen is often recommended as these nutrients have been proved to have good synergy (Dubousset, Etienne, and Avice Citation2010; Fismes et al. Citation2000). Salvagiotti and Miralles (Citation2008) described the increase of biomass and grain yield after S fertilization and the positive interaction between N and S, which resulted in a greater nitrogen use efficiency. Sulfur deficiency also lowers the utilization of N and results in a deterioration in crop quality (Carciochi et al. Citation2017). As sulfur is an essential constituent of enzymes involved in N metabolism, its deficiency could decrease N assimilation and effectiveness of N2 fixation (Grzebisz, Niewiadomska, and Przygocka-Cyna Citation2022). The accumulation of nitrates in S-deficient plants (Salvagiotti et al. Citation2009) have been also described, similar as accumulation of trace elements (Na and Salt Citation2011) and heavy metals stress response or increased susceptibility do diseases (Dubuis et al. Citation2005). In addition, Haneklaus, Bloem, and Schnug (Citation2007) reported that each kg of S deficit causes up to 15 kg of nitrogen to be lost in the environment. Oilseed rape (Brassica napus L.) is one of the major crops worldwide (Shahzadi et al. Citation2015) with the high demand for sulfur fertilization (Sienkiewicz-Cholewa and Kieloch Citation2015). Sulfur, together with boron, are essential for a synthesis of glucosinolates and sulfur amino acids (Zhao et al. Citation1993). Approximately 16 kg of sulfur per ha is required to produce 1 t of rape seeds according to the Varényiová, Ducsay, and Ryant (Citation2017). Because of this high demand for S, oilseed rape is particularly sensitive to sulfur deficiency compared to the other crops such as cereals or legumes (Zhao et al. Citation1997). For the oilseed rape, S has an important role in production of oil and total oil content (Egesel, Gül, and Kahrıman Citation2009; Jankowski Citation2008). Besides common sulfur containing fertilizers, some wastes from industrial production can be used as a source of this plant nutrient. A possible source of such waste sulfur is a biogas production. By application of this waste, it is possible to return at least part of the sulfur consumed by biomass utilized in the biogas plant back into the agroecosystem.
Biogas production is a currently promoted source of renewable energy, which can produce sulfur as a waste product. The European Green Deal sets to lower the greenhouse emission by at least 55% by the year 2030 and the biogas and biomethane should play a key role in Europe transition to the cleaner energy utilization according to the European Biogas Association (Arnau et al. Citation2020). Biogas contains hydrogen sulfide (H2S), which is removed in the biogas purification process while producing waste sulfur. The reutilization (Dong et al. Citation2022) of waste sulfur obtained from the biogas pre-treating process seems to be promising from the point of view of plant nutrition (Fontaine, Eriksen, and Sørensen Citation2021) and especially from the economic aspect of biogas production. It also provides a possible decrease in the use of common mineral fertilizers (which often requires an input of nonrenewable resources), decrease in the amount of waste deposited in the landfills and decrease in sulfur deficiency in the environment (Lisowska et al. Citation2022). Another possible positive effect of waste sulfur is the elemental form of sulfur, which acts much slower in the soil and have to be oxidize before it becomes available to the plants (Fontaine, Eriksen, and Sørensen Citation2021). Therefore, it can provide the plant-available sulfate more gradually, especially in combination with mineral fertilizers.
The aim of this study was to examine the effect of fertilization with waste elemental sulfur from biogas production solely and in combination with additional sources of nutrients. The waste sulfur from biogas plant is still an unconventional source of sulfur in common agriculture, therefore, our goal was to evaluate its positive or negative effects on oilseed rape growth and yield in comparison with the untreated plants. The main hypothesis was, that application of waste sulfur obtained from biogas plant after desulphurization process is going to have a positive effect on plant yield and growth in comparison with untreated plants. Second hypothesis was, that the application of other nutrients in the combination with waste S is going to enhance the possible effect of solely applied waste sulfur. The plant-growth experiment (2019) was established in smaller pots to examine the effect of waste sulfur treatments during the early vegetation stages of oilseed rape, the plant-yield experiment (2019-2020) with the identical treatments was established in Mitscherlich pots to verify the effect of waste sulfur treatments on the yield and quality of oilseed rape. Such reutilization of waste sulfur back in agriculture is suitable from the economic aspect of biogas purification and waste management. The application of this sulfur could help to reduce the consumption of mineral fertilizers and, at the same time, address the deficient sulfur content in the soil and plants.
Materials and methods
The two pot experiments were established in the greenhouse (2019) and vegetation hall (2019-2020) of the Biotechnological house at Mendel University in Brno, Brno, Czech Republic (49◦12′36.94” N and 16◦36′49.95” E).
Plant-growth experiment
The pilot experiment was established in smaller plastic pots (ø = 10 cm, height 12 cm) with 2.8 kg of soil per pot with the idea to observe the effect of waste sulfur and its possible combinations with boron, humic substances, and mineral fertilizers on oilseed rape during the early vegetation stages. The soil was imported in large quantities from the locality Žabčice (49◦1′9.009” N and 16◦35′29.64” E) before the start of the first experiment. Then, it was air dried and homogenized by hand using an expanded mesh panel throwing screen sieve (2 cm diameter sieve) at our university. Then, it was analyzed and used in the experiment. The agrochemical properties of used soil determined according to the certified methodology (Zbíral, Malý, and Váňa Citation2011) are given in . The oilseed rape (intensive hybrid variety DK Excellium; Dekalb) was sown on July 29, 2019. The application of fertilizers was performed in the form of a solution (240 ml per pot) applied to the soil surface after the sowing. The treatments included in the experiments were: 1. unfertilized control (Control), 2. waste elemental sulfur (S), 3. S + boron (B), 4. S + B + humic substances (HS), 5. S + B + HS + ammonium sulfate (AS), 6. S + B + HS + ammonium nitrate (AN). The examined treatments and fertilizers doses are described in . The fertilizers rates used in the pot experiments were converted from the field fertilizers rates: 75 and 100 kg ha−1 S, 1 kg ha−1 B, 1.05 kg ha−1 of HS, 25 kg ha−1 S in AS and 22 kg ha−1 of AN. The suspension of waste elemental sulfur (12% S0) was obtained by the desulfurization of biogas using the Thiopaq®scrubber (Paques, Balk, The Netherlands), which works by washing the raw biogas with a slightly alkaline solution (pH 8–9) and the subsequent biological oxidation of sulfides to elemental sulfur. The elemental sulfur particle size in the suspension was less than 60 μm (96.9% of the particles). The waste sulfur was mixed with other nutrients according to , every treatment had four repetitions. The following were used in mixtures with elemental sulfur: boric acid (B; 17.5% B, CAS: 10043-35-3, Sigma Aldrich, St. Louis, MO, USA), humic substances (HS; FK-7; 7% w/v humic substances in the form of water-soluble fulvic acids, VUCHT, Bratislava, Slovakia), ammonium sulfate (AS; 21% N and 24% S, CAS: 7783-20-2, Sigma Aldrich, St. Louis, MO, USA), and ammonium nitrate (AN; 35% N, CAS: 6484-52-2, Sigma Aldrich, St. Louis, MO, USA). The germination and emergence of oilseed rape took place in a climabox (PlantMaster, CLF Plant Climatics GmbH, Wertingen, Germany) at Mendel University in Brno (Brno, Czech Republic) to ensure the most uniform result. Ten days after the emergence, the oilseed rape was thinned down to 5 plants per pot and randomly moved to the temperature-controlled greenhouse (day/night: 12 h/12 h, min. light intensity 550 μmol m−2 s−1/0 μmol m−2 s−1; temperature 22 °C/15 °C; relative humidity 55-60%/90-95%) with drip irrigation. The chlorophyll content in plants were measured by Yara N-tester chlorophyll meter at the BBCH 19 (October 24, 2019) according Skarpa et al. (Citation2023). The aboveground biomass (AGB) of the rape plants were subsequently manually harvested, dried, and weighed that day. The results of dry matter (DM) of AGB were expressed as a 1 plant per pot (weight from pot/5), as the first, plant-growth experiment was mainly focused on the growth.
Table 1. Agrochemical properties of soil used in both experiments.
Table 2. Experimental treatments of waste sulfur in combination with another nutrients.
Plant-yield experiment
The subsequent, plant-yield experiment was established in Mitscherlich pots (ø = 20 cm, height 17.5 cm, STOMA GmbH, Siegburg, Germany) filled with 6.5 kg of air-dried and sieved soil. Soil from the same spot and locality as in the previous experiment was used, as it was prepared before the start of the first experiment in large quantity (). The examined treatments were also the same (). The experiment was established on the December 4, 2019, and the fertilization in form of solution was performed immediately after the sowing, identically to the first experiment. Each treatment had six repetitions. Main goal of this plant-yield experiment was to evaluate the effect of waste sulfur on the yield and quality of oilseed rape. The germination and emergence of oilseed rape took place in temperature-controlled greenhouse (day/night: 12 h/12 h, min. light intensity 550 μmol m−2 s−1/0 μmol m−2 s−1; temperature 22 °C/15 °C; relative humidity 55-60%/90-95%) at Mendel University in Brno (Brno, Czech Republic) to reduce the risk of freeze damage and ensuring the optimal progress of growth. Ten days after the emergence, the oilseed rape was thinned down to a final count of 5 plants per pot. The experimental pots were moved to the open vegetation hall with semi-natural climate conditions (under a rain shelter) and randomly (in terms of treatments and repetitions) placed on the aluminum table in the spring of 2020. A minimum spacing of 15 cm between pots was ensured. The average daily temperatures and the daily relative humidity in the vegetation hall are shown in . A controlled watering regime identical for all treatments (pots) was used in this experiment. Plants were watered to 70% of the maximum water holding capacity throughout the growing season. The watering regimes were maintained on gravimetric basis (Nachabe Citation1998). The pots were hand-watered with demineralized water on the soil surface. The two pots from each treatment were used for plants analysis during the vegetation (April 14, 2020; BBCH 55-57), the rest (4 pots) were manually fixed with a string to prevent lodging and they were harvested in full maturity of oilseed rape on the July 14, 2020. The biomass obtained in BBCH 55-57 and after the harvest (straw) were analyzed from two repetitions, the results presented in and are expressed as an average of these two analyses.
Figure 1. The average daily temperature (°C) and relative humidity (%) in the vegetation hall during the experiment.
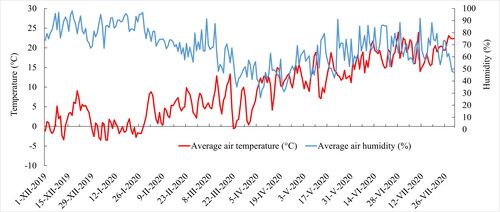
Table 3. The average content of N and S in the plants and their uptake by AGB of 1 plant with the N:S ratio.
Analytical methods
Chlorophyll content in plant leaves (N-tester values)
The chlorophyll content of oilseed rape leaves was measured using a Yara N-tester chlorophyll meter (Yara International ASA, Oslo, Norway). The chlorophyll content was expressed as ‘N-tester value.' Measurement was performed at a wavelength range of 650–940 nm. Using red and NIR values, the N-tester calculates a numeric, dimensionless value that is proportional to the amount of total chlorophyll present in the leaf. Five plants were assessed in each treatment and the value of the chlorophyll content of each plant was the mean of 60 measurement.
Plant analysis
The plants were manually harvested by cutting above the soil surface from each pot. The AGB of oilseed rape plants was oven- dried at 60 °C for the first two hours. The temperature was then reduced to 45 °C where the samples were kept for 72 h. The dry weight of AGB was determined using a laboratory-scale precision balanced Kern PCB (KERN & Sohn GmbH, Balingen, Germany). Then, the dried AGB was homogenized by the grinder Grindomix GM200 (Retsch GmbH, Haan, Germany). The HNO3/H2O2 digestion of biomass was achieved using a microwave digestion systemin ETHOS 1 (Milestone Srl, Sorisole, Italy). Subsequently, the nitrogen content in AGB of rape was determined by Kjeldahl method (Kjeltec 2300 device, Foss Analytical, Hillerød, Denmark) and other nutrients (S, P, K, Mg, Ca, and B) by Optical emission spectrometry (ICP–OES (Spectro, Kleve, Germany). Same analyses were used for determination the nutrient content in the rapeseed straw. The N/S uptake was calculated as (weight of AGB*content of N/S)/100 and then expressed as mg.
Seed yield and quality
The rape seeds in the plant-yield experiment were purified from coarse impurities by repetitive sifting. Rapeseed yield was weighed from five plants within each pot, and the value was adjusted to 8% of moisture. Rapeseed yield was than expressed as gram per pot (g pot−1). The oil content was determined gravimetrically after the extraction of the samples with diethyl ether using the Soxhlet method based on the NMR extraction of seeds in a continuous flow extractor Minispec mq series TD-NMR (Bruker Corporation, Ettlinger, Germany).
Statistic analysis
The effect of the waste sulfur treatments on the observed parameters (N-tester value, average DM weight of AGB of 1 plant, oilseed rape yield, straw yield, oil content and oil production) of oilseed rape was statistically analyzed using the STATISTICA 14 software (TIBCO Software, San Jose, CA, USA) by the method of analysis of variance with the treatment such as fixed effect and the pot used as the random effect to take into account the grouping of individuals in the same pot. The differences between the means were determined according to the Fisher’s LSD test, at the 95% level of significance (p ≤ 0.05). The normality and homogeneity of variances were verified, respectively, by Shapiro-Wilk and Levene values at p ≤ 0.05. The influence of the monitored factors was analyzed via analysis of variance (level of significance p ≤ 0.05). The statistical evaluation methods used were identical for both experiments.
Results and discussions
Plant-growth experiment
The first parameter observed in the pilot experiment with small pots was the chlorophyll content in oilseed rape leaves expressed as N-tester values. The results obtained from the measurements are given in . The effect of fertilization with waste sulfur proved to be statistically significant, the highest content of chlorophyll was observed after both treatments supplemented with mineral fertilizers, as the content of chlorophyll in plants is directly dependent on the amount of nitrogen in the tissues (Gianquinto et al. Citation2004; Mu et al. Citation2017). Ammonium nitrate same as ammonium sulfate both provided a quickly available nitrogen to the plants, which resulted in almost 42% and 44% increase in observed values in comparison with control treatment. Evaluation of the nutritional status after the joint fertilization with N and S by using the N-tester device was performed by Lacroux et al. (Citation2008), and Škarpa et al. (Citation2021), and their results also showed a significant increase in measured N-tester values after the joint treatment with N and S compared to the control. The application of sole waste sulfur from biogas plant also provided an increase in N-tester value in comparison with control treatment without fertilization, possibly because of the well-known synergy between sulfur fertilization and nitrogen efficiency.
Figure 2. The average N-tester values (BBCH 19, October 24, 2019). Columns marked by different letters indicate significant differences p < 0.05 (fisher’s LSD test). The error bars present the mean standard deviation. 1. unfertilized control (control), 2. waste elemental sulfur (S), 3. S + boron (B), 4. S + B + humic substances (HS), 5. S + B + HS + ammonium sulfate (AS), 6. S + B + HS + ammonium nitrate (AN).
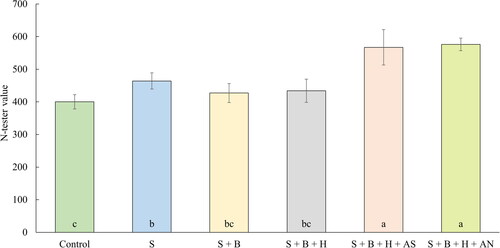
The average weigh of DM of AGB of oilseed rape plant after the harvest are in correlation with N-tester values as it is evident from the similar trend in the results presented in . The results presented in were used to calculate correlation, which represents a strong dependency (r = 0.98, p < 0.001). Both treatments of waste sulfur supplemented with mineral fertilizers provided the highest production of plant biomass, which can be again explained by the nitrogen addition to the fertilizer mixture. This result is in agreement with our previous work (Škarpa et al. Citation2021), where the waste sulfur was applied in mixtures with mineral nitrogen fertilizer (urea ammonium nitrate) with a positive effect on AGB production. The increase in AGB weight due to the sulfur fertilization was also described by Perveen et al. (Citation2018), as they observed a significant increase in root and shoot biomass and root and shoot length of maize due to the application of different sulfur compounds. An increased barley yield after the fertilization with S0 was described also by Grzebisz and Przygocka-Cyna (Citation2007) in their long-term experiment. A positive effect of the S application on canola pods formation and subsequent seed yield was also demonstrated by Khalid et al. (Citation2016). In our experiment, the fertilization with sole waste sulfur without any supplements (S) also proved to be statistically significant, with the 35.5% increase in comparison with control treatment. Although the importance of boron for the oilseed rape is well documented, neither the N-tester values or weight of AGB were increased after the treatments with the combination of S and B without mineral fertilizers (S + B, and S + B + HS) in comparison with fertilization with sole sulfur, possibly because of the early vegetation stages of measured and harvested plants (BBCH 19). It is also important to remind, that the examined fertilizers were applied to the soil (not on leaves), therefore, the applied boron had possibly less time for uptake and assimilation to the plants in this pilot experiment, although Malhi et al. (Citation2003) are describing incorporation of B into the soil as the most effective application. Other literatures on the contrary, have considered foliar application to be more suitable (Ma et al. Citation2015; Mortvedt Citation1994).
Figure 3. The average DM weight of AGB (g 1 plant−1; BBCH 19, October 24, 2019). Columns marked by different letters indicate significant differences p < 0.05 (fisher’s LSD test). The error bars present the mean standard deviation. 1. unfertilized control (control), 2. waste elemental sulfur (S), 3. S + boron (B), 4. S + B + humic substances (HS), 5. S + B + HS + ammonium sulfate (AS), 6. S + B + HS + ammonium nitrate (AN).
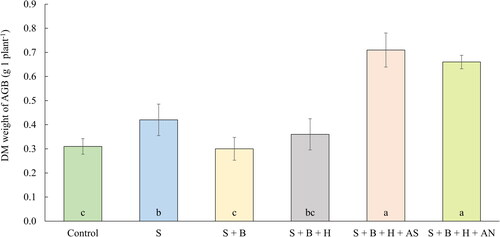
The average contents of nitrogen and sulfur determined in the AGB of the rape harvested at the end of the experiment are described in . The content of nitrogen was significantly highest in the plants treated with waste sulfur in mixture with mineral fertilizers, which can be easily explained by the addition of mineral nitrogen in comparison with other treatments. The content of sulfur after these treatments in plants was also one of the highest, which can be attributed to the great nitrogen-sulfur synergy (Jamal, Moon, and Abdin Citation2014) and to the additional source of sulfur in AS fertilizer. It could be also influenced by increased mobilization of organic sulfur and oxidation rate of sulfur as a result of mineral fertilizers addition. Various researchers have reported a lack of S to be a limiting factor for the efficient utilization of added nitrogen. Thus, for maximal use of added N, fertilization by sulfur to the soil becomes essential. More importantly, these mixed treatments resulted in highest N:S ratios, which can be presented as an interesting indicator of nutritional status according to Sutradhar, Kaiser, and Fernández (Citation2017). The principle behind this assessment is the fact that plants need a proportional amount of N and S for optimal amino acid synthesis. Therefore, nitrogen-to-sulfur ratios above a N:S ratio threshold indicate S deficiency (Haneklaus, Bloem, and Schnug Citation2007). A possible disadvantage of this indicator is the decreasing value of the N:S ratio during the vegetation (Reussi, Echeverría, and Sainz Rozas Citation2012). For oil seed rape, the ideal N:S ratio have been described as 7:1 (Janzen and Bettany Citation1984). The ratios observed from our experiment are lower, mostly because the plant analysis was performed early in the vegetation. The contents of sulfur observed in plants in our experiment were higher in comparison with common field conditions. On the contrary, the contents of nitrogen were lower compared to the common field conditions with high amounts of applied nitrogen. The main goal of our experiment was to evaluate the effect of waste sulfur, therefore, another uniform fertilization with nitrogen was omitted. The uptake of N and S by 1 plant were expressed from the average weight and average contents of nutrients (). It is evident from these results, that both treatments enhanced with mineral fertilizers have resulted in highest uptake of both N and S, the results are slightly in favor of AS instead of AN as it also provides available sulfur. The value of S uptake also helps to explain a lower content of sulfur in plants after the fertilization with solely waste S compared to the control treatment. The weight of average AGB of 1 plant was significantly higher on S treatment in comparison with control, which can possibly result in a lower content of S in plant tissues. However, the uptake of sulfur on both treatments were identical, in addition, the uptake of nitrogen was higher after the sulfur fertilization. The experiment performed by Tabak, Lisowska, and Filipek-Mazur (Citation2020) proved, that the application of waste sulfur increases the content of available form of S in the soil, which can lead to a higher uptake by plants, which is supported by our results (). This effect is more visible with longer time period and the dose of applied S. However, the high amount of waste sulfur can also have a negative effect on soil pH (Lisowska et al. Citation2022).
Plant-yield experiment
The aboveground biomass of oilseed rape was analyzed twice for the nutrient contents during the plant-yield experiment established in Mitscherlich pots. First results were obtained after the plants collection during the vegetation (the beginning of bud formation, BBCH 55-57) on April 14, 2020, second analysis was performed after the oilseed rape harvest three months later (July 14, 2020). The content of nutrients in the first term is described in . It is evident, that the addition of boron to the fertilizer mixture rapidly increased the content of B in the plants, as they were more mature (compared to the first experiment) and therefore had more time to uptake the nutrient from the soil. Almost every treatment has also provided an increased content of sulfur in comparison with unfertilized control. An increase in mobility and availability of sulfur after the application of waste sulfur is described by several authors (Lisowska et al. Citation2022; Tabak, Lisowska, and Filipek-Mazur Citation2020). The highest increase by 53% of S in plants in our experiment was observed after the fertilization with mixture of S + B + HS + AS. The dose of waste sulfur on this treatment enhanced with mineral fertilizers was lower (in comparison with the treatment without mineral fertilizers), but the addition of readily available ammonium sulfate provided the additional amount of S. On the contrary, the second treatment supplemented with mineral fertilizer (ammonium nitrate) provided a lower supply of S to the plants, as the content of sulfur in plants was by 30% lower in comparison with control. This result can be explained again by lower dosage of applied waste sulfur, which was enhanced only by mineral fertilizer without sulfur (ammonium nitrate) in this treatment. Interestingly, the content of nitrogen in plants were also lower after this treatment with ammonium nitrate in comparison with the treatment supplemented with ammonium sulfate, which again points out to more optimal nitrogen efficiency when the sulfur content is abundant (Carciochi et al. Citation2019). An increase in N concentration in leaves after the sulfur fertilization was also observed by Rossini et al. (Citation2018). The content of other nutrients was primarily based on their content in the soil since these nutrients were not supplied via examined treatments. However, the content of phosphorus in the plants was slightly higher after both treatments supplemented with mineral fertilizers. This result supports the idea, that the nitrogen, especially in ammonium form, is frequently increasing the P absorption. Nitrogen also supports the root growth, which is also essential for the uptake of low mobile phosphorus in the soil. As the provision of nitrogen to the agriculture is relatively infinite thanks to the Haber-Bosch synthesis, the phosphorus is predicted to be a limited source worldwide. Thus, a positive interaction between nitrogen and phosphorus is a welcome result. In addition, there has also been reported a positive interaction between sulfur and phosphorus (Yadav Citation2011).
Table 4. Average content of nutrients in plants at the start of bud formation (April 14, 2020).
The is describing the content of nutrients in the straw of rape after the harvest of oilseed rape and seed separation. The content of sulfur in the plant biomass was higher in every treatment fertilized with sulfur (compared to the control), which is an optimal prerequisite for high yield and oil content in the seeds. However, this result is in contrary with Varényiová, Ducsay, and Ryant (Citation2017), where the crop fertilized with sulfur showed lower content of sulfur in tissues. Treatments based on the application of waste sulfur (elemental sulfur) provided slightly higher content of sulfur in comparison with treatment supplemented with mineral fertilizers. This can be explained by a slower availability of elemental sulfur, which provided more optimal amount of available sulfate in later vegetation stages. Unfertilized plants (Control) showed the lowest AGB production (), straw (), and seed production (). It is likely that the nitrogen content was not diluted in their tissues (due to low carbohydrate productions) and therefore the N content was relatively highest (). Similar trends were observed for other nutrients (P, Mg, and Ca). The boron content in straw of rape under treatments with B fertilization were significantly higher than Control and S (no-B application).
Figure 4. The average straw yield of oilseed rape (g pot−1) (July 14, 2020). Columns marked by different letters indicate significant differences p < 0.05 (fisher’s LSD test). The error bars present the mean standard deviation. 1. unfertilized control (control), 2. waste elemental sulfur (S), 3. S + boron (B), 4. S + B + humic substances (HS), 5. S + B + HS + ammonium sulfate (AS), 6. S + B + HS + ammonium nitrate (AN).
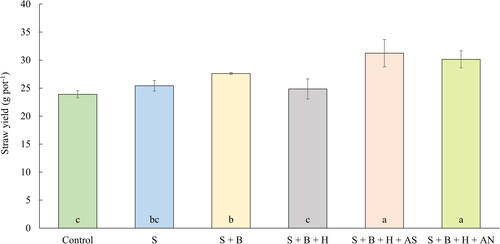
Figure 5. The average seed yield of oilseed rape (g pot−1) (July 14, 2020). Columns marked by different letters indicate significant differences p < 0.05 (fisher’s LSD test). The error bars present the mean standard deviation. 1. unfertilized control (control), 2. waste elemental sulfur (S), 3. S + boron (B), 4. S + B + humic substances (HS), 5. S + B + HS + ammonium sulfate (AS), 6. S + B + HS + ammonium nitrate (AN).
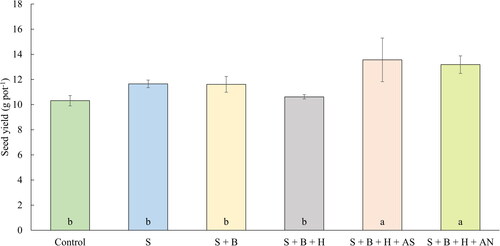
Table 5. Average post-harvest content of nutrients in straw of rape.
The weight of aboveground biomass (straw) of oilseed rape after the harvest and seed separation is described in . The highest, statistically significant, yield of straw was observed after both treatments supplemented with mineral fertilizers, which is in correlation with previous plant-growth experiment, and it can be similarly explained by the combination of waste sulfur in a slower elemental form with the quickly available nitrogen (ammonium nitrate) and even sulfur (ammonium sulfate) in mineral fertilizers. Similar availability of different form of sulfur are described by Riley, Zhao, and Mcgrath (Citation2000), a slower effect of fertilization with S0 is also published by Malhi, Solberg, and Nyborg (Citation2005). The control treatment without fertilization provided straw yield by 30.7% lower in comparison with the treatment S + B + HS + AS and by 26.1% lower in comparison with treatment S + B + HS + AN. Treatments based on the application of waste sulfur without mineral N-fertilizers also provided higher yield in comparison with control, the highest increased was observed after the mixture of waste S with boron (by 15.5%). The role of boron is often associated with a participation in the formation, structure and stability and cell walls. The optimal supply of B also ensures the strength and elasticity of plants tissues via lignification. Another important function of boron is the involvement in synthesis of proteins, nucleic acids, carbohydrates, and hormones, especially IAA. The addition of humic substances did not provide an increase in comparison with application of sole S or S + B. On the contrary, the straw yield was slightly lower. An interesting result was published by Šerá and Novák (Citation2022). Their research points out, that the effect of humic substances is strongly dependent on their origin (Nardi et al. Citation2002), structural, physical, and chemical properties, and on the taxonomy of examined plant species. In their experiment, the effect of humic substance on the germination and initial growth of oilseed rape was also not significant, the positive effect was observed with different plant species, Papaver somniferum L. The studies of HS on seed germination capacity with any positive effect was also published by Türkmen et al. (Citation2004). Important factors of applied HS are also a pH (Chen, Clapp, and Magen Citation2004) and content of potassium. However, even the adjusting of tested HS on the same level of pH and concentration of K may not resulted in positive effect of HS, as published by Šerá and Novák (Citation2011). They are also promoting the origin of HS as the most important factor, even compared to the application dose. According to their study, another important factors to fully examine the interactions of HS with plants are natural plant species and large ecological plasticity, as the high seed vitality and germination or low dormancy of model crops may obscure the effect of HS application. The different concentrations of humic acid were tested in the experiment performed by Dulaimy and Fahdawi (Citation2020). It is evident from their results, that some concentrations also had a negative effect on the examined parameters. On the contrary, the positive effect of HS is described by several authors (Al-Eezzi and Al-Alawy Citation2022; Zhang et al. Citation2021).
The obtained yield of oilseed rape is given in . The highest, statistically significant, yield was again achieved after both treatments supplemented with mineral fertilizers. The mixture of waste sulfur with ammonium sulfate provided an increase by 31.5% in comparison with control, the addition of ammonium nitrate has resulted in increase by 27.8%. The seed yield was significantly correlated with straw production (r = 0.97, p < 0.001). The mixture of waste sulfur and mineral fertilizers have provided the highest AGB also in the first, plant-growth experiment in smaller pots. The results of several studies (Ahmad et al. Citation2011; Jackson Citation2000) have also described the highest seed yield at treatments with sulfur and nitrogen combination. It has been reported that the grain yield can be significantly boosted by the combined application of N and S, which is related to the close link between the uptake of SO42- and NO3-. N and S have exhibited strong interdependence on effecting significant yield and quality improvements in several crop species, including maize (Xie et al. Citation2003), oilseed rape (McGrath and Zhao Citation1996), oilseed crops (Fazili et al. Citation2010), soybean (Jamal and Abdin Citation2005), wheat (Salvagiotti and Miralles Citation2008), sunflower (Hocking, Randall, and Pinkerton Citation1987), or sesame (Raza et al. Citation2018). The treatments based on fertilization with sole waste sulfur and waste S in mixture with boron provided a similar yield (Malhi, Gan, and Raney Citation2007; Pużyńska et al. Citation2018). The seed yield provided by the treatments with S + B + HS was again lower in comparison with the treatments without humic acids, although not significantly. This is partially in the contrary with the results published by Al-Bourky et al. (Citation2021). In this work with Oryza sativa L., the addition of HS resulted in higher yields, weight of grains, number of grains, number of panicles, leaf area and even plant height. Neither parameter was, however, statistically significantly higher compared to the control treatment. The effect of humic acid and the nitrogen fertilization was also tested by Delfine et al. (Citation2005). Their results are in agreement with ours, as the treatment with the application of humic acid provided a statistically significantly lower yield of Triticum durum compared to the soil fertilization by nitrogen in two experimental years. It is evident from , that the addition of mineral fertilizers to the mix have similar, significant effect.
The most important factor of oilseed rape quality is the oil content in seeds (Brennan, Mason, and Walton Citation2000). The average oil content in the seed of oilseed rape is described in the . There are several studies (Ahmad et al. Citation2011; Rehman et al. Citation2013) describing the positive effect of sulfur fertilization on the oil content. On the contrary, Ma et al. (Citation2015) reported, that the oil content is not dependent on the dose of sulfur, unlike the crop yield. Withers and O’Donnell (Citation1994) are described a decreased oil content in treatments fertilized by sulfur. It is evident from our results, that the established trend from previous examined parameters (highest values after both treatments with mineral fertilizers) is different. From the minerally enhanced treatments, only the treatment supplemented with ammonium sulfate have provided more oil content in comparison with control treatment. The second treatment based on the addition of mineral fertilizer supplemented with ammonium nitrate did provide lower content of oil in comparison with control treatment by 10.0%. This result can be possibly explained by the ‘dilution effect' (Jarrell and Beverly Citation1981). The younger the plants, the higher the nutrient concentrations of nutrients. The more the plants grow, the lower the concentration of nutrients. This is evident from , especially for the nitrogen content in plants tissues. The plants fertilized with mixture of waste sulfur and ammonium sulfate did provide high yield of straw and seeds, probably due to the addition of nitrogen contained in mineral fertilizer. However, the nitrogen efficiency of solely N from mineral fertilizers is relatively small (around 33-40% according to Barłóg, Grzebisz, and Łukowiak (Citation2022), so the nitrogen was probably depleted and utilized for the mentioned parameters. The full potential of nitrogen fertilization cannot be achieved with suboptimal amount of sulfur, as it was presented by several authors (Dubousset, Etienne, and Avice Citation2010; Fismes et al. Citation2000; Haneklaus, Bloem, and Schnug Citation2007). On the contrary, the mixture of waste sulfur and ammonium sulfate have provided slightly less nitrogen to the plants, but more amount of quickly available sulfur, which resulted in higher nitrogen utilization in plants and higher oil content in seeds probably due to the mentioned N-S synergy and addition of quickly available NS mineral fertilizer. Treatments without mineral fertilizers based only on waste sulfur have also provided statistically (except for S + B + HS) higher content of oil in comparison with control treatment, probably because of mentioned dilution effect. The observed yield of straw and seed was lower after these treatments; therefore, more nutrient was available for creating a sufficient amount of oil. The addition of solely HS did not result in higher quality product, which is similar to the finding by Delfine et al. (Citation2005). In this two years experiment, the bulk density, weight of thousand grains and protein content of Triticum durum were not significantly different compared to the control treatment. Not entirely unambiguous are also the findings published by Danyaei et al. (Citation2017). In their work, the combined application of sulfur-containing humic acid in different rates resulted in the highest fresh and dry weight of olives after the fertilization, however, the highest fruit length, chlorophyll a content and content of total chlorophyll were highest after the control treatment.
Figure 6. The average oil content of oilseed rape (July 14, 2020). Columns marked by different letters indicate significant differences p < 0.05 (fisher’s LSD test). The error bars present the mean standard deviation. 1. unfertilized control (control), 2. waste elemental sulfur (S), 3. S + boron (B), 4. S + B + humic substances (H), 5. S + B + HS + ammonium sulfate (AS), 6. S + B + HS + ammonium nitrate (AN).
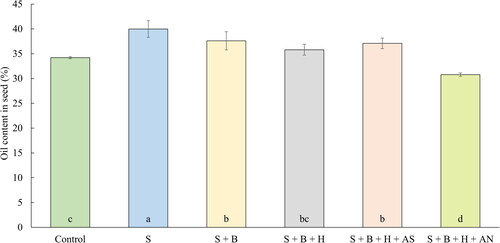
The average seed yield of rape and the average oil content were used for the expression of oil production (g pot−1). It is evident from the results (), that the treatment with mineral fertilizer containing sulfur (AS) have provided the highest production of oil from each pot. The treatment with sole waste sulfur application is another treatment with high oil production. The seed yield was lower after this fertilization compared to the minerally enhanced treatments (), however, the oil content () in seed fertilized with elemental sulfur was the highest compared to every other treatment. The second treatment enhanced with mineral fertilizer (AN) have on other side provided one of the highest seed yields, the oil content was however lower. These results are supporting the more balanced application of N and S as a more suitable option for oilseed rape.
Figure 7. The average production of oil (g pot−1) (July 14, 2020). Columns marked by different letters indicate significant differences p < 0.05 (fisher’s LSD test). The error bars present the mean standard deviation. 1. unfertilized control (control), 2. waste elemental sulfur (S), 3. S + boron (B), 4. S + B + humic substances (HS), 5. S + B + HS + ammonium sulfate (AS), 6. S + B + HS + ammonium nitrate (AN).
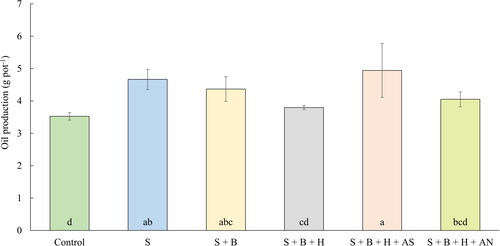
Conclusions
The result from both experiments had proved, that the reutilization of waste sulfur obtained from the biogas plants can be a viable option in agriculture. The reutilization of such waste as a fertilizer represents at least partial return of some nutrients depleted from the soil by the crops utilized in biogas plants, which is important from the point of view of nutrient balance. It is evident from presented results, that the application of sole waste sulfur is certainly a possibility, which confirmed initial hypothesis. However, the combination of waste S with additional nutrients is a more optimal alternative, which confirmed the second hypothesis. The mixture of waste sulfur and mineral nitrogen fertilizers (ammonium nitrate or ammonium sulfate) proved to have the greatest influence on the examined parameters of oilseed rape, such as weight of aboveground biomass, N/S content in the plant tissues and seed and straw yield. The mixture of waste sulfur with mineral fertilizers also represents an interesting compromise, that could lead to a lower consumption of common mineral fertilizers (especially N or NS) usually applied in agriculture. The mixture of waste sulfur in elemental form with ammonium sulfate also represent a prerequisite for optimal supply of S during the vegetation of crops. This treatment resulted in the highest overall seed and straw yield. Although the oil content in seeds was slightly lower probably due to the dilution effect, the production of oil was the highest due to the highest seed yield. In conclusion, the examined combination of waste sulfur with AS, resulted in the most quantitative and qualitative product.
Disclosure statement
The authors declare no conflict of interest.
Data availability statement
Presented data in this study are available on request from the corresponding author.
Additional information
Funding
References
- Ahmad, G., A. Jan, M. Arif, M. Tariq Jan, and H. Shah. 2011. Effect of nitrogen and sulfur fertilization on yield components, seed and oil yields of Canola. Journal of Plant Nutrition 34 (14):2069–82. doi: 10.1080/01904167.2011.618569.
- Al-Bourky, R. h., M. A. Manshood, M. R. Mahmoud, and S. T. Al-Mousawy. 2021. Effect of humic acid on growth and yield several genotypes of rice (Oryza Sativa L.). IOP Conference Series 923 (1):012059. doi: 10.1088/1755-1315/923/1/012059.
- Al-Eezzi, Y., and H. Al-Alawy. 2022. Effect of fulvic acid and seaweed on the growth and yield of broccoli. Journal of Pharmaceutical Negative Results 13 (S01):583–87. doi: 10.47750/pnr.2022.13.S01.71.
- Arnau, Á. S., S. Pflüger, M. Giacomazzi, M. Decorte, and M. Genua. 2020. Annual Report 2020 European Biogas Association. Brussels, Belgium: European Biogas Association. Accessed September 7, 2022. https://www.europeanbiogas.eu/eba-annual-report-2020/.
- Barłóg, P., W. Grzebisz, and R. Łukowiak. 2022. Fertilizers and fertilization strategies mitigating soil factors constraining efficiency of nitrogen in plant production. Plants (Basel, Switzerland) 11 (14):1855. doi: 10.3390/plants11141855.
- Berger, K. C., and E. Truog. 1939. Boron determination in soils and plants. Industrial & Engineering Chemistry Analytical Edition 11 (10):540–5. doi: 10.1021/ac50138a007.
- Brennan, R. F., M. G. Mason, and G. H. Walton. 2000. Effect of nitrogen fertilizer on the concentrations of oil and protein in canola (Brassica Napus) seed. Journal of Plant Nutrition 23 (3):339–48. doi: 10.1080/01904160009382020.
- Campbell, G. W., and R. I. Smith. 1997. Spatial and temporal trends in atmospheric sulphur deposition to agricultural surfaces in the United Kingdom. In Fertiliser society. London: Fertiliser Society.
- Carciochi, W. D., G. A. Divito, L. A. Fernández, and H. E. Echeverría. 2017. Sulfur affects root growth and improves nitrogen recovery and internal efficiency in wheat. Journal of Plant Nutrition 40 (9):1231–42. doi: 10.1080/01904167.2016.1187740.
- Carciochi, W. D., N. I. Reussi Calvo, N. Wyngaard, G. A. Divito, M. Eyherabide, and H. E. Echeverría. 2019. Prognosis and diagnosis of sulfur status in maize by plant analysis. European Journal of Agronomy 108:1–10. doi: 10.1016/j.eja.2019.04.008.
- Danyaei, A., S. Hassanpour, M. A. Baghaee, M. Dabbagh, and M. Babarabie. 2017. The effect of sulfur-containing humic acid on yield and nutrient uptake in olive fruit. Open Journal of Ecology 07 (04):279–88. doi: 10.4236/oje.2017.74019.
- Degryse, F., B. Ajiboye, R. Baird, R. C. da Silva, and M. J. McLaughlin. 2016. Oxidation of elemental sulfur in granular fertilizers depends on the soil-exposed surface area. Soil Science Society of America Journal 80 (2):294–305. doi: 10.2136/sssaj2015.06.0237.
- Delfine, S., R. Tognetti, E. Desiderio, and A. Alvino. 2005. Effect of foliar application of n and humic acids on growth and yield of durum wheat. Agronomy for Sustainable Development 25 (2):183–91. doi: 10.1051/agro:2005017.
- Dong, Y.-N., W.-C. Chen, L.-L. Zhang, B.-C. Sun, G.-W. Chu, and J.-F. Chen. 2022. Sulfur recycle in biogas production: Novel higee desulfurization process using natural amino acid salts. Chemosphere 297:134215. doi: 10.1016/j.chemosphere.2022.134215.
- Dubousset, L., P. Etienne, and J. C. Avice. 2010. Is the remobilization of s and n reserves for seed filling of winter oilseed rape modulated by sulphate restrictions occurring at different growth stages? Journal of Experimental Botany 61 (15):4313–24. doi: 10.1093/jxb/erq233.
- Dubuis, P.-H., C. Marazzi, E. Stadler, and F. Mauch. 2005. Sulphur deficiency causes a reduction in antimicrobial potential and leads to increased disease susceptibility of oilseed rape. Journal of Phytopathology 153 (1):27–36. doi: 10.1111/j.1439-0434.2004.00923.x.
- Dulaimy, J., and W. Fahdawi. 2020. Effect of humic acid on growth and yield of barley humic acid as interacted with row spacing. https://www.researchgate.net/publication/340936001.
- Egesel, C. Ö., M. K. Gül, and F. Kahrıman. 2009. Changes in yield and seed quality traits in rapeseed genotypes by sulphur fertilization. European Food Research and Technology 229 (3):505–13. doi: 10.1007/s00217-009-1067-3.
- Engardt, M., D. Simpson, M. Schwikowski, and L. Granat. 2017. Deposition of sulphur and nitrogen in Europe 1900–2050. Model calculations and comparison to historical observations. Tellus B 69 (1):1328945. doi: 10.1080/16000889.2017.1328945.
- Fazili, I. S., M. Masoodi, S. Ahmad, A. Jamal, J. S. Khan, and M. Z. Abdin. 2010. Interactive effect of sulfur and nitrogen on growth and yield attributes of oilseed crops (Brassica campestris l. and Eruca Sativa mill.) differing in yield potential. Journal of Plant Nutrition 33 (8):1216–28. doi: 10.1080/01904161003765745.
- Feinberg, A., A. Stenke, T. Peter, E. Lyn, S. Hinckley, C. T. Driscoll, and L. H. E. Winkel. 2021. Reductions in the deposition of sulfur and selenium to agricultural soils pose risk of future nutrient deficiencies. Communications Earth & Environment 2 (1):101. doi: 10.1038/s43247-021-00172-0.
- Fismes, J., P. C. Vong, A. Guckert, and E. Frossard. 2000. Influence of sulfur on apparent n-use efficiency, yield and quality of oilseed rape (Brassica Napus L.) grown on a calcareous soil. European Journal of Agronomy 12 (2):127–41. doi: 10.1016/S1161-0301(99)00052-0.
- Fontaine, D., J. Eriksen, and P. Sørensen. 2021. Sulfur from biogas desulfurization: Fate of S during storage in manure and after application to plants. The Science of the Total Environment 754 (February):142180. doi: 10.1016/j.scitotenv.2020.142180.
- Gianquinto, G., J. P. Goffart, M. Olivier, G. Guarda, M. Colauzzi, L. Dalla Costa, G. Delle Vedove, J. Vos, and D. K. L. Mackerron. 2004. The use of hand-held chlorophyll meters as a tool to assess the nitrogen status and to guide nitrogen fertilization of potato crop. Potato Research 47 (1–2):35–80. doi: 10.1007/BF02731970.
- Grzebisz, W., A. Niewiadomska, and K. Przygocka-Cyna. 2022. Nitrogen hotspots on the farm—A practice-oriented approach. Agronomy 12 (6):1305. doi: 10.3390/agronomy12061305.
- Grzebisz, W., and K. Przygocka-Cyna. 2007. Spring malt barley response to elemental sulphur – the prognostic value of n and s concentrations in malt barley leaves. Plant, Soil and Environment 53 (9):388–94. doi: 10.17221/2295-PSE.
- Haneklaus, S., E. Bloem, and E. Schnug. 2007. Sulfur interactions in crop ecosystems. In Sulfur in plants an ecological perspective, ed. M. J. Hawkesford and L. J. De Kok, 17–58. Germany: Springer. doi: 10.1007/978-1-4020-5887-5_2.
- Hocking, P. J., P. J. Randall, and A. Pinkerton. 1987. Sulphur nutrition of sunflower (Helianthus Annuus) as affected by nitrogen supply: Effects on vegetative growth, the development of yield components, and seed yield and quality. Field Crops Research 16 (2):157–75. doi: 10.1016/0378-4290(87)90005-0.
- Chen, Y., C. E. Clapp, and H. Magen. 2004. Mechanisms of plant growth stimulation by humic substances: The role of organo-iron complexes. Soil Science and Plant Nutrition 50 (7):1089–95. doi: 10.1080/00380768.2004.10408579.
- CHMI 2019. IX. Atmospheric Deposition in the Territory of the Czech Republic Accessed September 11, 2022. https://www.chmi.cz/files/portal/docs/uoco/isko/grafroc/19groc/gr19cz/09_depozice_v1.pdf. (in Czech)
- Jackson, G. D. 2000. Effects of nitrogen and sulfur on canola yield and nutrient uptake. Agronomy Journal 92 (4):644–9. doi: 10.2134/agronj2000.924644x.
- Jamal, A., and M. Z. Abdin. 2005. Effect of sulphur and nitrogen application on growth characteristics, seed and oil yield of soybean cultivars https://www.researchgate.net/publication/230824531.
- Jamal, A., Y.-S. Moon, and M. Z. Abdin. 2014. Sulphur-a general overview and interaction with nitrogen. https://www.researchgate.net/publication/228481225.
- Jankowski, K. J. 2008. Effect of sulfur on the quality of winter rape seeds. Article in journal of elementology. https://www.researchgate.net/publication/282808488.
- Janzen, H. H., and J. R. Bettany. 1984. Sulfur nutrition of rapeseed: I. Influence of fertilizer nitrogen and sulfur rates. Soil Science Society of America Journal 48 (1):100–7. doi: 10.2136/sssaj1984.03615995004800010019x.
- Jarrell, W. M., and R. B. Beverly. 1981. The dilution effect in plant nutrition studies. Advances in Agronomy 34:197–224. doi: 10.1016/S0065-2113(08)60887-1.
- Khalid, S., A. Muhammad, M. Fazal, I. Imranuddin, N. Ullah, and I. Ullah. 2016. Effect of sulphur foliar application on yield and yield components of brassica napus. International Journal of Agricultural AndEnvironmental Research 2 (3):232–6. doi: 10.13140/RG.2.2.33876.45442.
- Kulhánek, M., J. Balík, J. Černý, O. Sedlář, and F. Vašák. 2016. Evaluating of soil sulfur forms changes under different fertilizing systems during long-term field experiments. Plant, Soil and Environment 62 (9):408–15. doi: 10.17221/236/2016-PSE.
- Lacroux, F., O. Trégoat, C. Van Leeuwen, A. Pons, T. Tominaga, V. Lavigne-Cruège, and D. Dubourdieu. 2008. Effect of foliar nitrogen and sulphur application on aromatic Expression of Vitis Vinifera&L. Cv. Sauvignon Blanc. OENO One 42 (3):125. doi: 10.20870/oeno-one.2008.42.3.816.
- Lindsay, W. L., and W. A. Norvell. 1978. Development of a DTPA soil test for zinc, iron, manganese, and copper. Soil Science Society of America Journal 42 (3):421–8. doi: 10.2136/sssaj1978.03615995004200030009x.
- Lisowska, A., B. Filipek-Mazur, M. Komorowska, M. Niemiec, D. Bar-Michalczyk, M. Kuboń, S. Tabor, Z. Gródek-Szostak, A. Szeląg-Sikora, J. Sikora, et al. 2022. Environmental and production aspects of using fertilizers based on waste elemental sulfur and organic materials. Materials (Basel, Switzerland) 15 (9):3387. doi: 10.3390/ma15093387.
- Ma, B., D. K. Biswas, A. W. Herath, J. K. Whalen, S. Q. Ruan, C. Caldwell, H. Earl, A. Vanasse, P. Scott, and D. L. Smith. 2015. Growth, yield, and yield components of canola as affected by nitrogen, sulfur, and boron application. Journal of Plant Nutrition and Soil Science 178 (4):658–70. doi: 10.1002/jpln.201400280.
- Malhi, S. S., E. D. Solberg, and M. Nyborg. 2005. Influence of formulation of elemental S fertilizer on yield, quality and S uptake of canola seed. Canadian Journal of Plant Science 85 (4):793–802. doi: 10.4141/P04-134.
- Malhi, S. S., M. Raza, J. J. Schoenau, A. R. Mermut, R. Kutcher, A. M. Johnston, and K. S. Gill. 2003. Feasibility of boron fertilization for yield, seed quality and B uptake of canola in northeastern saskatchewan. Canadian Journal of Soil Science 83 (1):99–108. doi: 10.4141/S01-081.
- Malhi, S. S., Y. Gan, and J. P. Raney. 2007. Yield, seed quality, and sulfur uptake of brassica oilseed crops in response to sulfur fertilization. Agronomy Journal 99 (2):570–7. doi: 10.2134/agronj2006.0269.
- McGrath, S. P., and F. J. Zhao. 1996. Sulphur uptake, yield responses and the interactions between nitrogen and sulphur in winter oilseed rape (Brassica Napus). The Journal of Agricultural Science 126 (1):53–62. doi: 10.1017/S0021859600088808.
- Mehlich, A. 1984. Mehlich 3 soil test extractant: A modification of mehlich 2 extractant. Communications in Soil Science and Plant Analysis 15 (12):1409–16. doi: 10.1080/00103628409367568.
- Mortvedt, J. J. 1994. Needs for controlled-availability micronutrient fertilizers. Fertilizer Research 38 (3):213–21. doi: 10.1007/BF00749694.
- Mu, X., Q. Chen, F. Chen, L. Yuan, and G. Mi. 2017. A RNA-seq analysis of the response of photosynthetic system to low nitrogen supply in maize leaf. International Journal of Molecular Sciences 18 (12):2624. doi: 10.3390/ijms18122624.
- Na, G. N., and D. E. Salt. 2011. The role of sulfur assimilation and sulfur-containing compounds in trace element homeostasis in plants. Environmental and Experimental Botany 72 (1):18–25. doi: 10.1016/j.envexpbot.2010.04.004.
- Nachabe, M. H. 1998. Refining the definition of field capacity in the literature. Journal of Irrigation and Drainage Engineering 124 (4):230–2. doi: 10.1061/(ASCE)0733-9437(1998)124:4(230).
- Narayan, O. P., P. Kumar, B. Yadav, M. Dua, and A. Kumar Johri. 2022. Sulfur nutrition and its role in plant growth and development. Plant Signaling & Behavior 9:2030082. doi: 10.1080/15592324.2022.2030082.
- Nardi, S.,D. Pizzeghello,A. Muscolo, and A. Vianello. 2002. Physiological effects of humic substances on higher plants. Soil Biology and Biochemistry 34 (11):1527–36. doi: 10.1016/S0038-0717(02)00174-8.
- Perveen, S., N. Iqbal, M. Saeed, S. Zafar, and Z. Arshad. 2018. Role of foliar application of sulfur-containing compounds on maize (Zea Mays L. Var. Malka and Hybrid DTC) under salt stress. Brazilian Journal of Botany 41 (4):805–15. doi: 10.1007/s40415-018-0506-4.
- Pużyńska, K., B. Kulig, W. Halecki, A. Lepiarczyk, and S. Pużyński. 2018. Response of oilseed rape leaves to sulfur and boron foliar application. Acta Physiologiae Plantarum 40 (9):169. doi: 10.1007/s11738-018-2748-y.
- Raza, M. A., L. Yang Feng, A. Manaf, A. Wasaya, M. Ansar, A. Hussain, M. H. B. Khalid, N. Iqbal, Z. J. Xi, Y. K. Chen, et al. 2018. Sulphur application increases seed yield and oil content in sesame seeds under rainfed conditions. Field Crops Research 218:51–8. doi: 10.1016/j.fcr.2017.12.024.
- Rehman, H., Q. Iqbal, M. Farooq, A. Wahid, I. Afzal, and S. M. A. Basra. 2013. Sulphur application improves the growth, seed yield and oil quality of canola. Acta Physiologiae Plantarum 35 (10):2999–3006. doi: 10.1007/s11738-013-1331-9.
- Reussi, N., H. E. Echeverría, and H. Sainz Rozas. 2012. Stability of foliar nitrogen: Sulfur ratio in spring red wheat and sulfur dilution curve. Journal of Plant Nutrition 35 (7):990–1003. doi: 10.1080/01904167.2012.671403.
- Riley, N. G., F. J. Zhao, and S. P. Mcgrath. 2000. Availability of different forms of sulphur fertilisers to wheat and oilseed rape. Plant and Soil 222 (1/2):139–47. doi: 10.1023/A:1004757503831.
- Rossini, F., M. Provenzano, F. Sestili, and R. Ruggeri. 2018. Synergistic effect of sulfur and nitrogen in the organic and mineral fertilization of durum wheat: Grain yield and quality traits in the mediterranean environment. Agronomy 8 (9):189. doi: 10.3390/agronomy8090189.
- Salvagiotti, F., and D. J. Miralles. 2008. Radiation interception, biomass production and grain yield as affected by the interaction of nitrogen and sulfur fertilization in wheat. European Journal of Agronomy 28 (3):282–90. doi: 10.1016/j.eja.2007.08.002.
- Salvagiotti, F., J. M. Castellarín, D. J. Miralles, and H. M. Pedrol. 2009. Sulfur fertilization improves nitrogen use efficiency in wheat by increasing nitrogen uptake. Field Crops Research 113 (2):170–7. doi: 10.1016/j.fcr.2009.05.003.
- Shahzadi, T., A. Khan, F. Zafar, A. Ismail, E. Amin, and S. Riaz. 2015. An overview of brassica species for crop improvement. J. Agric. & Environ. Sci 15 (8):1568–73. doi: 10.5829/idosi.aejaes.2015.15.8.12746.
- Scherer, H. W. 2009. Sulfur in soils. Journal of Plant Nutrition and Soil Science 172 (3):326–35. doi: 10.1002/jpln.200900037.
- Schoenau, J. J., and S. M. Sukhdev. 2008. Sulfur forms and cycling processes in soil and their relationship to sulfur fertility. In Sulfur: A missing link between soils, crops, and nutrition, edited by J. Jez, 1–10. Madison: American Society of Agronomy. doi: 10.2134/agronmonogr50.c1.
- Sienkiewicz-Cholewa, U., and R. Kieloch. 2015. Effect of sulphur and micronutrients fertilization on yield and fat content in winter rape seeds (Brassica Napus L.) Plant, Soil and Environment 61 (4):164–70. doi: 10.17221/24/2015-PSE.
- Skarpa, P., J. Jancar, P. Lepcio, J. Antosovsky, D. Klofac, T. Kriska, A. M. Abdel-Mohsen, and M. Brtnicky. 2023. Effect of fertilizers enriched with bio-based carriers on selected growth parameters, grain yield and grain quality of maize (Zea mays L.). European Journal of Agronomy 143:126714. doi: 10.1016/j.eja.2022.126714.
- Sutradhar, A. K., D. E. Kaiser, and F. G. Fernández. 2017. Does total nitrogen/sulfur ratio predict nitrogen or sulfur requirement for corn? Soil Science Society of America Journal 81 (3):564–77. doi: 10.2136/sssaj2016.10.0352.
- Šerá, B., and F. Novák. 2011. The effect of humic substances on germination and early growth of lamb’s quarters (Chenopodium Album Agg.). Biologia 66 (3):470–6. doi: 10.2478/s11756-011-0037-y.
- Šerá, B., and F. Novák. 2022. Stimulation of seed germination and early growth by humic substances on poppy, pepper, rape, and hemp. Biologia 77 (3):641–8. doi: 10.1007/s11756-021-00952-1.
- Škarpa, P., J. Antošovský, P. Ryant, T. Hammerschmiedt, A. Kintl, and M. Brtnický. 2021. Using waste sulfur from biogas production in combination with nitrogen fertilization of maize (Zea Mays L.) by foliar application. Plants (Basel, Switzerland) 10 (10):2188. doi: 10.3390/plants10102188.
- Tabak, M., A. Lisowska, and B. Filipek-Mazur. 2020. Bioavailability of sulfur from waste obtained during biogas desulfurization and the effect of sulfur on soil acidity and biological activity. Processes 8 (7):863. doi: 10.3390/pr8070863.
- Türkmen, Ö., A. Dursun, M. Turan, and Ç. Erdinç. 2004. Calcium and humic acid affect seed germination, growth, and nutrient content of tomato (Lycopersicon Esculentum L.) seedlings under saline soil conditions. Acta Agriculturae Scandinavica Section B: Soil and Plant Science 54 (3):168–74. doi: 10.1080/09064710310022014.
- Varényiová, M., L. Ducsay, and P. Ryant. 2017. Sulphur nutrition and its effect on yield and oil content of oilseed rape (Brassica Napus L.). Acta Universitatis Agriculturae Et Silviculturae Mendelianae Brunensis 65 (2):555–62. doi: 10.11118/actaun201765020555.
- Withers, P. J. A., and F. M. O’Donnell. 1994. The response of double-low winter oilseed rape to fertiliser sulphur. Journal of the Science of Food and Agriculture 66 (1):93–101. doi: 10.1002/jsfa.2740660114.
- Xie, R., S. Dong, C. Hu, and K. Wang. 2003. The role of nitrogen and sulfur interaction in maize Quality(Zea Mays L.) Agricultural Sciences in China 2:527–32.
- Yadav, B. K. 2011. Interaction effect of phosphorus and sulphur on yield and quality of clusterbean in typic haplustept. World Journal of Agricultural Sciences 7 (5):556–60.
- Zbíral, J., M. Smatanová, and P. Němec. 2018. Sulphur status in agricultural soils determined using the mehlich 3 method. Plant, Soil and Environment 64 (6):255–9. doi: 10.17221/142/2018-PSE.
- Zbíral, J., S. Malý, and M. Váňa. 2011. Soil analysis, 3rd ed. Central Institute for Supervising and Testing in Agriculture: Brno, Czech Republic, 18–52.
- Zenda, T., S. Liu, A. Dong, and H. Duan. 2021. Revisiting sulphur—The once neglected nutrient: It’s roles in plant growth, metabolism, stress tolerance and crop production. Agriculture 11 (7):626. doi: 10.3390/agriculture11070626.
- Zhang, P., H. Zhang, G. Wu, X. Chen, N. Gruda, X. Li, J. Dong, and Z. Duan. 2021. Dose-dependent application of straw-derived fulvic acid on yield and quality of tomato plants grown in a greenhouse. Frontiers in Plant Science 12:736613. doi: 10.3389/fpls.2021.736613.
- Zhao, F. J., P. E. Bilsborrow, E. J. Evans, and S. P. McGrath. 1997. Nitrogen to sulphur ratio in rapeseed and in rapeseed protein and its use in diagnosing sulphur deficiency. Journal of Plant Nutrition 20 (4-5):549–58. doi: 10.1080/01904169709365273.
- Zhao, F., E. J. Evans, P. E. Bilsborrow, and J. K. Syers. 1993. Influence of sulphur and nitrogen on seed yield and quality of low glucosinolate oilseed rape (Brassica napus L). Journal of the Science of Food and Agriculture 63 (1):29–37. doi: 10.1002/jsfa.2740630106.