ABSTRACT
This study aimed at an ultrastructural characterization of myofibroblasts cultured from different compartments of lung from never-smokers and smokers with or without COPD. In addition, we evaluated the expression of alpha smooth muscle actin (α-SMA), a marker for myofibroblasts, and contractile properties. Stromal cells cultured from central and corresponding peripheral or only from peripheral lung of never-smokers, smokers without COPD and COPD patients were analyzed by transmission electron microscopy (TEM), immunoelectron microscopy (IEM), Western analysis and/or by collagen gel contraction assay. TEM revealed that myofibroblasts cultured from smokers and COPD had less prominent intracellular actin filaments. We also examined fibronexus (FNX), which is a typical ultrastructural feature of myofibroblasts, and observed that patients with COPD more frequently had tandem-like FNX as compared to other samples. Western analysis showed that the samples derived from the central lung of never-smokers expressed higher levels of α-SMA than those of smokers and COPD patients. Cells from central lung were less contractile than those from peripheral lung. We conclude that myofibroblasts have variable ultrastructural and functional properties based on their localization in the lung and, moreover, these properties are affected by both smoking history and COPD.
KEYWORDS:
Introduction
Chronic obstructive pulmonary disease (COPD) is characterized by progressive and non-reversible airflow limitation caused both by fibrosis of airway walls and enlargement of air spaces i.e. emphysema.Citation1 The lung extracellular matrix (ECM) is an important contributor to these changes, similarly as in the progression of several other lung diseases.Citation2,Citation3 It is believed that the chronic inflammation and continuous repair processes trigger an accumulation of ECM components in COPD.Citation4 Fibroblasts and especially myofibroblasts produce most of the ECM proteins and their abnormal activation may lead to pathological tissue remodeling.
Originally myofibroblasts were identified as mediators of wound healing, especially as promoters of scar contraction.Citation5 Since their discovery, myofibroblasts have been identified in various pathological conditions in several tissues.Citation6,Citation7 They are stellate- or spindle-shaped mesenchymal cells typically expressing alpha smooth muscle actin (α-SMA) with a contractile phenotype.Citation8 Myofibroblasts can be only truly identified by their specific ultrastructure as revealed by transmission electron microscopy (TEM), with typical features being a dilated rough endoplasmic reticulum (rER) as well as the presence of gap junctions, peripheral myofilaments and fibronexus (FNX).Citation9,Citation10 FNX is a transmembrane association of α-SMA positive intracellular filaments and fibronectin positive extracellular filaments. FNXs have been classified into three categories, namely 1) track-, 2) plaque- and 3) tandem-like; track-like FNXs can be further subdivided into straight/rigid, curved and fragmentary/scanty subtypes.Citation11 Similarly to the situation in other tissues, track-like FNXs are the most common type in lung myofibroblasts but additionally myofibroblasts derived from interstitial lung diseases (ILD) such as idiopathic pulmonary fibrosis (IPF), asbestosis or hypersensitivity pneumonitis occasionally express also plaque- and tandem-like FNXs.Citation12,Citation13 Tandem- and plaque-like FNXs can also be observed in lung cancer-associated myofibroblasts.Citation13 Disease related differences have also been revealed in other ultrastructural features of myofibroblasts.Citation12,Citation13
Both smoking per se and the presence of airways obstruction, i.e. COPD may have a variable effect on myofibroblasts in distinct locations of the lung. Histological evaluations have shown that in COPD the numbers of myofibroblasts are increased in large airway walls but decreased in small airway walls.Citation14,Citation15 It has also been reported that myofibroblasts were evident also at the endings of the detached alveolar walls in their widened alveolar tips.Citation14 As a consequence of the altered myofibroblast distribution, also other ECM structural elements e.g. tenascin C (Tn-C), fibronectin and collagens have a disturbed expression in COPD.Citation15,Citation16
Even though the stromal cell and ECM modifications associated with smoking and COPD seem to be localization-specific, there are only a few studies comparing the properties of the stromal cells derived from different compartments of the lung. It has been shown that stromal cells derived and cultured from peripheral lung were more contractile than cells sampled from central lung.Citation17 Furthermore, the stromal cells derived from peripheral lung of COPD patient were more contractile also containing more α-SMA than those cells derived from normal peripheral lung.Citation17 TEM analysis has revealed that stromal cells cultured from central lung of smokers displayed fewer myofibroblastic features than cells derived from nonsmokers.Citation13 These abovementioned features as mentioned in some other publications indicated that at least some of the diseases and localization specific properties of the stromal cells seem to be maintained during the culturing of these cells.Citation18
The aim of this study was to compare the properties of cultured stromal cells from peripheral and central lung of never-smokers as well as from smokers with and without COPD. The ultrastructure of cultured lung myofibroblasts and the localization of α-SMA in single cells were analyzed by TEM and IEM. The α-SMA expression and contraction capacity of the whole stromal cell population were also measured.
Materials and methods
Study subjects
Lung tissue samples were derived from 26 patients who had undergone surgery for lung cancer during 2008 to 2012 in Oulu University Hospital. Each patient provided their informed consent and the study protocol had a favorable statement from the local ethical committee. Patients were divided according to the clinical data and lung function parameters into never-smokers (n = 7), smokers without COPD (n = 10) and smokers with COPD (n = 9). Group of COPD patients included 2 ex-smokers while the rest 7 were current smokers. COPD was diagnosed and classified based on the GOLD criteria. Demographic data of the patients is described in . Some of the same samples have been analyzed also in another context and those previously published results are used partially also in this publication.Citation13,Citation14
Table 1. Patient data and number of donors for both peripheral and central lung samples or only for peripheral lung samples. (SD = standard deviation)
Cell culture
Tissue samples from tumor-free central airways and tumor-free peripheral lung were collected for the culture of stromal cells. An experienced pulmonary pathologist collected lung tissue specimens for cell culture during the diagnostic sample preparation. Samples were taken only if it did not disturb the routine diagnostics, and thus the samples from tumor-free central lung were not available from all patients (). The cells were cultured as described previously.Citation12,Citation18 Briefly, the cells were cultured in Minimum essential medium Eagle α modification (Sigma-Aldrich, Inc, St Louis, MO, USA) supplemented with 13% heat-inactivated fetal bovine serum (PromoCell, Heidelberg, Germany), 10 mM 4-(2-hydroxyethyl)-1-piperazine-ethanesulfonic acid (HEPES, Sigma), 100 U/ml penicillin (Sigma), 100 μg/ml streptomycin (10 mg/ml, Sigma), 2.5 μg/ml amphotericin B (Sigma) and 2 mM L-glutamine (Sigma). The cells were passaged at near confluence and they were examined after 2, 3, or 4 passages. Because the samples used in this study are untransformed primary cell-derived cultures having limited proliferation capacity, some samples were analyzed only in certain assays while some samples were used for all assays. Samples from 3 never-smokers, 4 healthy smokers and 4 COPD patients were analyzed by all of the methods described in this study. In addition, due to material limitations, some samples were analyzed only by some method(s) as described below. Samples from 2 never-smokers, 5 smokers without COPD and 3 COPD lung were analyzed by TEM, IEM and Western analysis but not by collagen gel contraction assay. Samples from 1 never-smoker, 1 smoker without COPD and 2 COPD patients were analyzed only by collagen gel contraction assay.
Transmission electron microscopy
Cultured stromal cells from peripheral lung tissue of never-smokers (n = 5), smokers without COPD (n = 9) and smokers with COPD (n = 7) were prepared for TEM. COPD group included one patient who had given up smoking and the rest were current smokers. From the same patients, the specimens were derived central lung tissue of never-smokers (n = 5), smokers without COPD (n = 6) and smokers with COPD (n = 2). As described above, the central tissue was not obtained for every patient due to the location of the tumor. The cultured cells were fixed, prepared, and sectioned as previously described.Citation19 The sections were stained with uranyl acetate and lead citrate and visualized by Philips CM 100 TEM (FEI, Eindhoven, The Netherlands) utilizing Olympus Soft Imaging System (Munich, Germany).
The ultrastructural properties were analyzed as described previously.Citation12 Briefly, the amount of intracellular actin filaments, the amount of extracellular component of FNX and the amount of pericellular ECM were quantified as low (+), moderate (++) or strong (+++). The type of FNX was categorized according to Singer classification into tandem, plaque, or track-like,Citation11 and the subtype of the track-like FNX as follows: straight/rigid, curved or fragmentary/scanty.Citation13 The occurrence of adherens- and gap-type junctions and dilated rER was evaluated as the numbers of positive cases. Two investigators separately assessed the semi-quantitative TEM findings and there was almost perfect agreement in their evaluations (p < .001, Fisher; kappa coefficient = 0.947).
Immunoelectron microscopy
All the cell lines analyzed by TEM were also processed for IEM as previously described.Citation12 Ultrathin sections were first incubated in 0.1% glycine in phosphate-buffered saline (PBS) and then in 1% bovine serum albumin in PBS. Monoclonal anti-human α-SMA antibody clone 1A4 (Dako, Glostrup, Denmark) incubation was followed by anti-mouse IgG (Zymed Invitrogen, Carsbad, USA) incubation, after which the samples were incubated with Protein A-gold conjugate (Department of Cell Biology, University of Utrecht, The Netherlands) prior to visualization. The sections were embedded in methylcellulose and studied by Philips CM 100 TEM. The amount of gold particles was counted in four rectangles, 0.26 µm2 each, diagonally marked starting from the upper left corner of the image. Ten images per sample were counted, i.e., the total analyzed area was 10.4 µm2 for each sample.
Western analysis
Cultured stromal cells from central and peripheral lung compartments were analyzed by Western analysis for α-SMA expression as described previously.Citation12 The samples analyzed were the same as those used in TEM and IEM. After blotting, the membranes were incubated with monoclonal anti human α-SMA antibody clone 1A4 and polyclonal anti-human GAPDH (Abcam, Cambridge, UK) followed by IRDye800 Conjugated affinity-purified anti-mouse IgG (Rockland Immunochemicals, Gilbertsville, PA, USA) and IRDye700 Conjugated affinity-purified anti-rabbit IgG (Rockland Immunochemicals). The intensities of the bands were analyzed by Odyssey infrared imager (LI-COR Biosciences, Lincoln, NE, USA). The α-SMA intensity was related to the corresponding GAPDH intensity of the same sample. One control sample was used for normalization of the variation between distinct membranes.
Collagen gel contraction assay
In order to study the functional properties, cells from tumor-free peripheral lung of 5 never-smokers, 5 smokers without COPD and 6 smokers with COPD were evaluated in the gel contraction assay. 4 COPD patients were current smokers and 2 were ex-smokers. Central lung samples were derived from the same patients, i.e., from 4 nonsmoking cases, 3 smokers without COPD and 4 smokers with COPD. Central sample was not available in all cases as described above. Contraction assays were conducted in four replicates as previously described.Citation13 Briefly, a mixture of collagen (extracted from rat tail, 0.75 mg/ml) gel and cell suspension (300 000 cells per ml) in serum-free medium was cast into 24-well plates and allowed to set for 15 min in the cell culture incubator. The gels were detached from the walls of the wells with a spatula and subsequently 1 ml of serum-free medium was added into each well. The areas of the gels were measured every day (days 0–4) by MCIDTM Core Image Analysis System software. The results are represented as percentages compared to the original size of collagen gel.
Statistical analysis
Statistical analyses were performed by Statistical Package for the Social Sciences (IBM SPSS statistics 25, IBM Inc. Chicago, IL) using Chi-Square test or Fisher’s Exact Test for categorized data and Mann-Whitney U – test, Independent Samples t-test, Paired Samples T-test, Kruskal-Wallis test or ANOVA for continuous data. Values of p < .05 were considered as significant and indicated as * < 0.05, ** < 0.01 and *** < 0.001.
Results
Ultrastructural features of myofibroblasts
Stromal cells from 21 patients (5 never-smokers, 9 smokers without COPD and 7 smokers with COPD) were cultured and analyzed by TEM. From 13 patients, the cells were derived and analyzed from both peripheral and central lung, and from 8 patients only from peripheral lung. The cultured cell populations contained both fibroblasts and myofibroblasts as described earlier,Citation12,Citation18 but TEM analysis was used to reveal the ultrastructural features typical of myofibroblasts. The ultrastructural features of myofibroblasts from peripheral () and central lung () were evaluated in a semiquantitative manner. Representative TEM images can be seen in . Statistically significant differences between the groups are displayed in .
Table 2. Transmission electron microscopy analyses of myofibroblasts cultured from peripheral lung of never-smokers, smokers and COPD. Values indicate the numbers of samples that expressed certain features (percentage in parenthesis)
Table 3. Transmission electron microscopy analyses of myofibroblasts cultured from central lung of never-smokers, smokers and COPD. Values indicate the numbers of patient samples that expressed certain features (percentage in parenthesis)
Table 4. The main findings of myofibroblasts and fibroblastic cells from never-smokers, healthy smokers and COPD. The cells were cultured from peripheral and central lung.)
Figure 1. Representative specimens of transmission electron microscopy analysis. Cultured myofibroblasts from never-smokers (A,B), smokers (C,D) and COPD (E,F) were analyzed. On the left is a sample from peripheral lung and on the right, the central sample from the same patient. Dilated rough endoplasmic reticulum (arrow), extracellular component of fibronexus (asterix), intracellular actin belt (arrowhead) and adherens junction (white arrow) are indicated
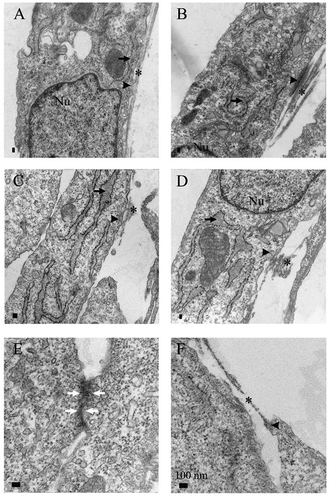
Intracellular actin filaments appeared on the edges of the cells and as expected, they had a mostly faint or moderate appearance (). Myofibroblasts derived from never-smokers had more actin filaments compared to those sampled from smokers including both healthy smokers and COPD patients (p = .034) but in contrast, the strongest expression was seen in one peripheral sample derived from a COPD patient. Interestingly, the sample with the strongest actin filaments was from the youngest donor (53 years) examined in this study and was one of two COPD patients who had given up smoking. Furthermore, the average age of donors for whom we had peripheral samples with mild actin filaments was 66 years; for those having moderate expression, it was 72 years. However, there was no statistically significant correlation between age and the intensity of actin filaments.
The FNX composition of intracellular actin and extracellular fibronectin () has been regarded as the main ultrastructural feature of myofibroblasts. FNXs were identified in 31 out of 34 samples; i.e. only in three samples from smokers without COPD could FNXs not be seen. In the samples from never-smokers, 60% of peripheral lung samples and 100% of central lung samples showed a moderate frequency of FNX. In samples from healthy smokers or COPD patients, the variation of FNX distribution was higher. In most samples, FNX were found at either low or moderate frequencies, while one peripheral cell sample showed a high FNX frequency which was the same specimen as the actin filament outlier described above. There was no statistically significant difference between the groups. As expected, most of FNXs detected were track-like ( and b) but myofibroblasts cultured from lungs of the patients with COPD showed more frequently tandem-like FNXs () than those cells derived from never-smokers or from healthy smokers (p = .007). All subtypes of track-like FNXs were seen in all groups, except for the curved type, which was not seen in samples derived from COPD patients.
The pericellular ECM was very scanty in most samples and only in 4 samples was it estimated to be moderate. Focal densities and dilated rER ( and d) were seen in the majority of the samples when no obvious differences between the study groups were detected. Every sample from COPD patients had adherens junctions (). In the never-smoker group, the adherens junctions were seen in every peripheral sample but only in 40% of central lung samples. In the smokers without COPD, the difference was opposite as in these samples, adherens junctions were seen in all samples from central lung but only in 63% of samples from peripheral lung.
α-SMA expression
The α-SMA analysis in single cells by IEM and in the whole-cultured stromal cell population by Western analysis confirmed the presence of the specific isoform of actin that is considered as a marker of myofibroblast ( and ). IEM analysis showed that myofibroblasts from the central lung compartment exhibited a tendency of more prominent α-SMA expression when compared to the cells derived from peripheral lung, although this was not quite statistically significant (p = .073, ). Western analysis revealed that central cells from never-smokers expressed more α-SMA as compared to central cells from all smokers including both healthy smokers and COPD (p = .027, ). As can be seen from the standard deviations in , there was wide interindividual variation in the extent of α-SMA expression.
Figure 2. Representative specimens of immunoelectron microscopy analysis for alpha smooth muscle actin (α-SMA) expression in myofibroblasts cultured from never-smokers (A, B), smokers (C, D) and COPD (E, F). Peripheral samples are seen on the left and central samples from the same patient on the right. Some gold particles used as the α-SMA stain are indicated by arrows
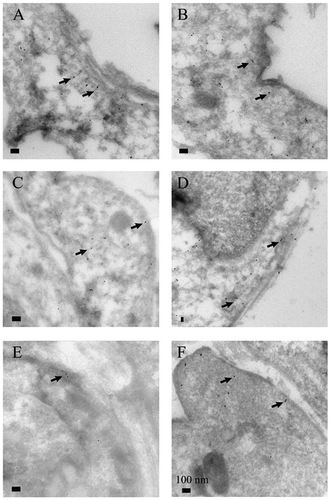
Gel contraction assay
The results of collagen gel contraction analysis of 5 never-smokers, 5 smokers without COPD and 6 COPD patients are shown in . Cells from peripheral () and central () lung of never-smokers were less contractile than the corresponding cells from smokers or COPD patients. However, the differences were not statistically significant. Cells derived from peripheral lung tissue contracted collagen gels more than cells derived from central lung and this difference reached statistical significance when all time points were analyzed in a single-paired comparison (p = .005).
Figure 4. Collagen gel contraction assay of cultured stromal cells derived from peripheral (A) or central (B) lung of never-smokers, healthy smokers or COPD patients and a comparison of all peripheral and central cells (C). In panels A and B, the standard deviations are shown as positive bars for never-smokers, negative bars for smokers and as open circles for COPD
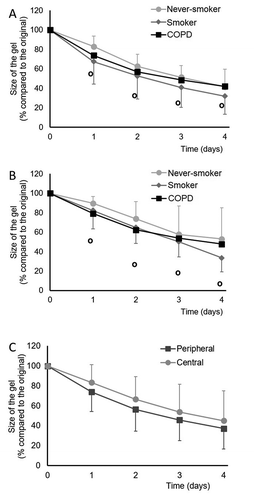
Discussion
This study revealed the ultrastructural features, α-SMA expression analysis, and contraction properties of stromal cells cultured from peripheral or central lung of never-smokers, smokers without COPD and smokers with COPD. This is the first time that an ultrastructural characterization of myofibroblasts has been performed in this context and to this extent. The typical morphological features of myofibroblasts e.g. actin filaments, frequency and phenotype of FNX, adherens junctions, dilated rER, and pericellular ECM were evaluated with differences being detected in many of the ultrastructural properties such as actin filament and FNX appearance and in α-SMA expression and contractile properties.
It has been previously shown that intracellular actin filaments are more prominent in myofibroblasts derived from interstitial lung diseases (ILD) like idiopathic pulmonary fibrosis (IPF) as compared to normal lung.Citation12 Our results revealed a difference in actin filament expression, i.e. we could detect a reduction in the actin bundle load in smokers as compared to never-smokers, suggesting that while ILD induced filaments in the myofibroblasts, smoking exerted the opposite effect. Smoking seems to have complex effect on intracellular actin filaments and there are some confusing reports about its influences. Based on in vitro experiments on cultured cells, it has been suggested that bronchial epithelial cells respond to acute cigarette smoke extract exposure by increasing actin polymerization while other reports have claimed that there is an opposite effect in alveolar epithelial cells.Citation20,Citation21 Our study focused on myofibroblasts that were derived from lung with variable smoking histories, but they were all cultured without in vitro cigarette smoke exposure. It is possible that myofibroblasts from smokers’ lung have a reduced actin polymerization rate that could, in theory, help them to avoid excessive stress fiber formation in the presence of continuous smoke exposure. Further studies will be required to elucidate this complex phenomenon.
FNX is a structure on the surface of myofibroblasts that is believed to serve as an intercellular junction between cells; especially it allows for a direct contact between intracellular filaments and the ECM.Citation22 FNX is not an exclusively myofibroblastic phenomenon as similar structures have also been observed in some other cell types such as aortic smooth muscle and endothelium.Citation23 All these cells are contractile and need strong but dynamic adhesion to the ECM. Thus it has been proposed that FNX is a mechano-transducer of ECM transformation that can assist cells in adapting to extracellular stress or changing pressure.Citation23 In our study, FNXs were detected in almost every sample when most of them consisted of the track-like subtype, although myofibroblasts derived from COPD had more tandem-like FNXs than cells derived from non-COPD patients. It is tempting to speculate that the variable distribution of FNX subtypes would reflect the variable role of the myofibroblasts in different pathological conditions or the status of the ECM in these conditions.
We analyzed α-SMA expression by IEM analysis at the single myofibroblast level as well as by Western analysis at the cell population level. Western analysis showed that central cells from never-smokers expressed more α-SMA than central cells from smokers. Even though this was not confirmed by IEM, this result is in line with the TEM observation as we could see less intracellular actin filaments in smokers’ cells. IEM suggested that there might be more α-SMA in myofibroblasts derived from central lung as compared to peripheral lung. This is at odds with the previous report of Hallgren and coworkers; they observed a reverse tendency in their Western analysis.Citation17 However, it is notable that both in their and our studies, the interindividual variation of α-SMA expression was extensive; clearly more samples should be evaluated before drawing more definitive conclusions.
Previously it has been reported that cultured stromal cells from peripheral lung of COPD patients contracted more than those derived from the corresponding central lung or from normal lung.Citation17 The results obtained in our study were in parallel with those studies although we could not detect such large differences. It was, however, notable that the samples examined in the previous study had been collected from lung explants, i.e. from very severe COPD cases (stage IV) while our samples represented mainly a less progressed COPD status (stages I–II). Thus, it is possible that the small tendency of COPD samples to become more contractile seen in our study is more evident in an advanced stage COPD. In fact, in our study, the cells from smokers’ lung without COPD seemed to be as contractile as those from COPD. Our study confirms also the observation that stromal cells derived from peripheral lung are more contractile than cells from central lung.Citation17
The results of our study suggest that stromal cells vary in terms of their ultrastructure, α-SMA expression and contractility depending on their original localization, the disease status, and the smoking history of the donor.
Acknowledgments
The authors thank Raija Sormunen PhD and the Biocenter Oulu EM laboratory staff for their valuable assistance in performing the electron microscopy.
Disclosure statement
The authors report no conflict of interest.
Additional information
Funding
References
- Barnes PJ, Shapiro SD, Pauwels RA. Chronic obstructive pulmonary disease: molecular and cellular mechanisms. Eur.Respir.J. 2003;22(4):672–688. doi:10.1183/09031936.03.00040703.
- Burgess JK, Mauad T, Tjin G, Karlsson JC, Westergren-Thorsson G. The extracellular matrix - the under-recognized element in lung disease? J.Pathol. 2016;240(4):397–409. doi:10.1002/path.4808.
- Hussell T, Lui S, Jagger C, Morgan D, Brand O. The consequence of matrix dysfunction on lung immunity and the microbiome in COPD. Eur.Respir.Rev. 2018;27(148):180032. doi:10.1183/16000617.0032-2018. Print 2018 Jun 30.
- Annoni R, Lancas T, Yukimatsu Tanigawa R, et al. Extracellular matrix composition in COPD. Eur.Respir.J. 2012;40(6):1362–1373. doi:10.1183/09031936.00192611.
- Gabbiani G, Ryan GB, Majne G. Presence of modified fibroblasts in granulation tissue and their possible role in wound contraction. Experientia. 1971;27(5):549–550. doi:10.1007/BF02147594.
- Klingberg F, Hinz B, White ES. The myofibroblast matrix: implications for tissue repair and fibrosis. J.Pathol. 2013;229:298–309.
- Darby IA, Zakuan N, Billet F, Desmouliere A. The myofibroblast, a key cell in normal and pathological tissue repair. Cell Mol.Life Sci. 2016;73(6):1145–1157. doi:10.1007/s00018-015-2110-0.
- Eyden B. The myofibroblast: phenotypic characterization as a prerequisite to understanding its functions in translational medicine. J.Cell.Mol.Med. 2008;12(1):22–37. doi:10.1111/j.1582-4934.2007.00213.x.
- Singer II, Kazazis DM, Kawka DW. Localization of the fibronexus at the surface of granulation tissue myofibroblasts using double-label immunogold electron microscopy on ultrathin frozen sections. Eur.J.Cell Biol. 1985;38:94–101.
- Eyden B. The fibronexus in reactive and tumoral myofibroblasts: further characterisation by electron microscopy. Histol.Histopathol. 2001;16:57–70.
- Singer II, Kawka DW, Kazazis DM, Clark RA. In vivo co-distribution of fibronectin and actin fibers in granulation tissue: immunofluorescence and electron microscope studies of the fibronexus at the myofibroblast surface. J.Cell Biol. 1984;98(6):2091–2106. doi:10.1083/jcb.98.6.2091.
- Karvonen HM, Lehtonen ST, Sormunen RT, et al. Myofibroblasts in interstitial lung diseases show diverse electron microscopic and invasive features. Lab.Invest. 2012;92(9):1270–1284. doi:10.1038/labinvest.2012.95.
- Karvonen HM, Lehtonen ST, Sormunen RT, Lappi-Blanco E, Skold CM, Kaarteenaho RL. Lung cancer-associated myofibroblasts reveal distinctive ultrastructure and function. J.Thorac.Oncol. 2014;9(5):664–674. doi:10.1097/JTO.0000000000000149.
- Karvonen HM, Lehtonen ST, Harju T, et al. Myofibroblast expression in airways and alveoli is affected by smoking and COPD. Respir.Res. 2013;14(1):84. doi:10.1186/1465-9921-14-84.
- Lofdahl M, Kaarteenaho R, Lappi-Blanco E, Tornling G, Skold MC. Tenascin-C and alpha-smooth muscle actin positive cells are increased in the large airways in patients with COPD. Respir.Res. 2011;12(1):48. doi:10.1186/1465-9921-12-48.
- Harju T, Kinnula VL, Paakko P, Salmenkivi K, Risteli J, Kaarteenaho R. Variability in the precursor proteins of collagen I and III in different stages of COPD. Respir.Res. 2010;11(1):165. doi:10.1186/1465-9921-11-165.
- Hallgren O, Rolandsson S, Andersson-Sjoland A, et al. Enhanced ROCK1 dependent contractility in fibroblast from chronic obstructive pulmonary disease patients. J.Transl.Med. 2012;10(1):171. doi:10.1186/1479-5876-10-171.
- Lehtonen ST, Karvonen HM, Harju T, et al. Stromal cells can be cultured and characterized from diagnostic bronchoalveolar fluid samples obtained from patients with various types of interstitial lung diseases. APMIS. 2014;122(4):301–316. doi:10.1111/apm.12146.
- Kaarteenaho-Wiik R, Paakko P, Sormunen R. Ultrastructural features of lung fibroblast differentiation into myofibroblasts. Ultrastruct.Pathol. 2009;33(1):6–15. doi:10.1080/01913120802608430.
- D’Anna C, Cigna D, Di Sano C, et al. Exposure to cigarette smoke extract and lipopolysaccharide modifies cytoskeleton organization in bronchial epithelial cells. Exp.Lung Res. 2017;43(9–10):347–358. doi:10.1080/01902148.2017.1377784.
- Schneider DJ, Speth JM, Penke LR, Wettlaufer SH, Swanson JA, Peters-Golden M. Mechanisms and modulation of microvesicle uptake in a model of alveolar cell communication. J.Biol.Chem. 2017;292(51):20897–20910. doi:10.1074/jbc.M117.792416.
- Eyden BP. Brief review of the fibronexus and its significance for myofibroblastic differentiation and tumor diagnosis. Ultrastruct.Pathol. 1993;17(6):611–622. doi:10.3109/01913129309027797.
- Eyden B. Fibronexus junctions associated with in vivo human endothelium. Ultrastruct.Pathol. 2009;33(1):28–32. doi:10.1080/01913120802625822.