ABSTRACT
Widely available digital technologies are empowering citizens who are increasingly well informed and involved in numerous water, climate, and environmental challenges. Citizen science can serve many different purposes, from the “pleasure of doing science” to complementing observations, increasing scientific literacy, and supporting collaborative behaviour to solve specific water management problems. Still, procedures on how to incorporate citizens’ knowledge effectively to inform policy and decision-making are lagging behind. Moreover, general conceptual frameworks are unavailable, preventing the widespread uptake of citizen science approaches for more participatory cross-sectorial water governance. In this work, we identify the shared constituents, interfaces, and interlinkages between hydrological sciences and other academic and non-academic disciplines in addressing water issues. Our goal is to conceptualize a transdisciplinary framework for valuing citizen science and advancing the hydrological sciences. Joint efforts between hydrological, computer, and social sciences are envisaged for integrating human sensing and behavioural mechanisms into the framework. Expanding opportunities of online communities complement the fundamental value of on-site surveying and indigenous knowledge. This work is promoted by the Citizens AND HYdrology (CANDHY) Working Group established by the International Association of Hydrological Sciences (IAHS).
Editor A. CastellarinAssociate editor Not assigned
1 Introduction
Interest in citizen science is growing in several disciplines (Kullenberg and Kasperowski Citation2016, Njue et al. Citation2019), including the hydrological sciences (). State-of-the-art citizen science approaches, achievements, and caveats in hydrology and geosciences are currently being discussed and reviewed (Conrad and Hilchey Citation2011, Assumpção et al. Citation2018, Zheng et al. Citation2018, Njue et al. Citation2019, Paul et al. Citation2019, See Citation2019). Crowdsourcing, a specific type of citizen science (Haklay Citation2013, Paul et al. Citation2018), is expected to gain momentum as well as play a bigger role in water resource and risk monitoring (Mazzoleni et al. Citation2017, Citation2018), management (Uprety et al. Citation2019), communication, and awareness-raising (Mukhtarov et al. Citation2018).
Figure 1. Bibliometric analysis using the Scopus database (for the period 1960–2018) filtering journal papers referring to hydrological studies [Scopus search string TITLE-ABS-KEY (hydrology OR hydrological OR floods OR droughts OR water)] (briefly referred to as “Hydrology”) or papers where citizen science and hydrology (briefly referred to as “Citizens and Hydrology”) are investigated by filtering keywords that are used as title, abstract or keyword (Scopus search string for citizens and hydrology is [TITLE-ABS-KEY (hydrology OR hydrological OR floods OR droughts OR water) AND TITLE-ABS-KEY (“citizen science” OR crowdsourced OR crowdsourcing OR citizen)]. The curves shown for “Citizens & Hydrology” (blue) and “hydrology” (green) represent the ratio of the number of papers published in each year as respect to the overall average number of publications in the reference period (1960–2018), expressed as a percentage increase. The horizontal bar chart inset shows the breakdown of percentages of publications per scientific sector (as categorized by Scopus) related to the blue curve.
![Figure 1. Bibliometric analysis using the Scopus database (for the period 1960–2018) filtering journal papers referring to hydrological studies [Scopus search string TITLE-ABS-KEY (hydrology OR hydrological OR floods OR droughts OR water)] (briefly referred to as “Hydrology”) or papers where citizen science and hydrology (briefly referred to as “Citizens and Hydrology”) are investigated by filtering keywords that are used as title, abstract or keyword (Scopus search string for citizens and hydrology is [TITLE-ABS-KEY (hydrology OR hydrological OR floods OR droughts OR water) AND TITLE-ABS-KEY (“citizen science” OR crowdsourced OR crowdsourcing OR citizen)]. The curves shown for “Citizens & Hydrology” (blue) and “hydrology” (green) represent the ratio of the number of papers published in each year as respect to the overall average number of publications in the reference period (1960–2018), expressed as a percentage increase. The horizontal bar chart inset shows the breakdown of percentages of publications per scientific sector (as categorized by Scopus) related to the blue curve.](/cms/asset/011da400-d419-4ba1-9c77-035a7000b3c6/thsj_a_1849707_f0001_oc.jpg)
Citizens’ involvement is beneficial for studies assessing human impacts on the hydrological cycle (Abbott et al. Citation2019), as well as for assessing the interlinkages between hydroclimatic dynamics, especially extremes, and society. This is a relevant and timely societal challenge, as evidenced by the International Association of Hydrological Sciences (IAHS) launching the hydrological decade 2013–2022 with the theme “Panta Rhei: Change in Hydrology and Society” (Montanari et al. Citation2013, McMillan et al. Citation2016). Coupled water–human socio-hydrology studies interlinking the environmental, social, and economic sciences have matured, providing additional clues to achieving water security and sustainable development (Di Baldassarre et al. Citation2013b, Citation2019, Young et al. Citation2015, Brondizio et al. Citation2016, Ceola et al. Citation2016, Mård et al. Citation2018). At the same time, awareness of major water, climatic, and environmental issues has stressed the importance of citizens’ engagement (Hayward Citation2012, Liu et al. Citation2014, O’Brien et al. Citation2018).
Hydrology is therefore poised to become a major field of activity for citizen science, which may lead both scientists and citizens to better understand the complexity of hydrological phenomena. Citizen science constitutes, for hydrologists, an opportunity to join efforts integrating scientific, social, economic, cultural, political, and administrative processes. Moreover, citizens’ observations and behaviour are not bounded and biased by disciplinary preconceptions. Crowdsourcing can fill the need for more distributed and diverse observations of the multiple sets of land, water, atmosphere, and energy variables (e.g. geology, soil type, vegetation, soil moisture, surface and groundwater discharge, sediment yields, soil chemistry, microbial community, air temperature, and energy consumption). In this regard, transfer of hydrological knowledge, data, and tools is particularly important for addressing knowledge gaps linked to most studies that have investigated only one of the Earth’s spheres (i.e. hydrosphere, biosphere, lithosphere, or atmosphere). Citizen science projects could address scientific challenges prompted by Critical Zone (CZ) investigations (White et al. Citation2015, Brantley et al. Citation2016, Bui Citation2016), which require the interfacing of hydro-meteorologic, hydrologic, hydrogeologic, ecohydrologic, and biogeochemical sciences (Banwart et al. Citation2013, Cudennec et al. Citation2016, Loiselle et al. Citation2016, Guswa et al. Citation2020). Moreover, pressing scientific and societal challenges linked to water, energy and food security cannot be dissociated from humans and the role they play in the water–energy–food–ecosystem (WEFE) nexus (Liu et al. Citation2017, Carmona-Moreno et al. Citation2018, Cudennec et al. Citation2018, D’Odorico et al. Citation2018, Connor et al. Citation2020, Rosa et al. Citation2020) or in any attempt to address global environmental challenges in the Anthropocene (Palsson et al. Citation2013, Wu et al. Citation2013, Brondizio et al. Citation2016).
The following section introduces the main definitions and concepts and the challenges and opportunities linked to citizen science for hydrological sciences.
1.1 Citizen involvement in hydrology: from knowledge to action
The heterogeneous nature of citizen participation in water research projects allows multiple ways for citizens and scientists to interact. As a consequence, there are also multiple definitions of citizen science (See et al. Citation2016, Eitzel et al. Citation2017). Most literature defines citizen science as a method applied in research, designed and coordinated by scientists, which includes the involvement of citizens. Common theoretical schemes and definitions of citizen science are based on varying levels of participation by citizens. For instance, Haklay (Citation2013) proposed four increasing levels of involvement: (1) crowdsourcing, (2) distributed intelligence, (3) participatory science, and (4) extreme citizen science. Another consequence of this heterogeneity is that citizen science overlaps with community-based participatory research, public participation in science and research, participatory research, or participatory action research (Eitzel et al. Citation2017). summarizes the most relevant definitions used in this manuscript. We also introduce definitions of human sensors and citizen science for hydrological sciences, based on concepts explained later in this work.
Table 1. Definitions of major concepts or newly introduced (in bold) used in this manuscript.
Citizen science is dedicated to developing scientifically organized data–information–knowledge workflow performed through scientist–citizen cooperation (Wiggins and Crowston Citation2011, Aulov et al. Citation2014, Geiger and Schader Citation2014, Morschheuser et al. Citation2016, Smith et al. Citation2017, Vicari et al. Citation2019) and may also include citizen-designed experiments of scientific interest (Puri and Sahay Citation2003). The value of public engagement in research projects goes beyond the pure extension of the scientific observation capacity (Gura Citation2013). Directly involving citizens is an effective way to account for social, economic, educational, and behavioural dynamics (Chawla and Cushing Citation2007, Palsson et al. Citation2013, Jollymore et al. Citation2017). Together with more informed communication strategies (Aulov et al. Citation2014, Rutten et al. Citation2017, Smith et al. Citation2017, Pandya and Dibner Citation2019, Vicari et al. Citation2019), citizen science leverages multiple voices and local culture and conditions, which bring critical indigenous knowledge to hydrological studies (Sivapalan Citation2005). Citizens’ engagement may, thus, have multiple beneficial outcomes, from knowledge development and awareness raising to encouraging more informed actions by concerned citizens. A large number of citizen science projects in environmental and hydrological sciences have demonstrated their positive impacts on the sustainability and safety of natural and anthropogenic ecosystems (Buytaert et al. Citation2014, Njue et al. Citation2019, Federal Crowdsourcing and Citizen Science Catalog Citation2020, Joint Research Centre Citation2020).
1.2 Citizens’ intelligence for hydrological observations
Technological advances enabled scientists to capture hydrological processes at finer spatial and temporal scales. However, hydrology remains a data-scarce science, with many important variables (such as river flow, water quality, sediments, rainfall/snow depths, and groundwater levels) being severely under-sampled. This has decisive implications for our ability to assess and manage water resources, deal with challenges, and forecast events (Beven et al. Citation2020, Cudennec et al. Citation2020, Pecora and Lins Citation2020). Also, variables that are less used in practical applications can be crucial for the scientific understanding of complex processes and systems. Examples include interception volumes and their partitioning, snowmelt and sublimation fluxes, and phenomenological and social indicators affected by hydrological processes.
Citizens using mobile devices in urban and non-urban ecosystems are generating an unprecedented amount of data. Opportunistic sensing (definition in ) is an emerging frontier for the hydrological sciences, gaining novel insights from citizens’ distributed monitoring networks of hydrological processes (McCabe et al. Citation2017, Tauro et al. Citation2018). Social and demographic, behavioural, and human dynamics data are also voluntarily (or non-voluntarily) produced and shared by citizens through their handheld devices or digital platforms (Smith et al. Citation2019). Those data are commonly referred to as crowdsourced data (Howe Citation2006) or volunteered geographic information (VGI) (Goodchild Citation2007, Haklay Citation2013) (definition in ). The terms “informal” or “unstructured” data are also used to refer to data produced by citizens, since this data often does not conform to existing standards (Stork Citation2001, Melville et al. Citation2012, Gandomi and Haider Citation2015).
Participatory monitoring approaches and crowdsourcing (definition in ) of citizen scientists are increasingly tested to fill this data gap in hydrology and related disciplines, thanks to distributed volunteers using manual instruments such as the raingauge or more complete personal weather stations and other affordable environmental sensors (Buytaert et al. Citation2014, Follett and Strezov Citation2015, Kullenberg and Kasperowski Citation2016, Cunha et al. Citation2017, Mao et al. Citation2019, Trouille et al. Citation2019). Widely accessible technologies allow non-experts to easily gather, analyse, visualize, and share a wealth of Earth system data (Breuer et al. Citation2015, Michelsen et al. Citation2016, Starkey et al. Citation2017, Tkachenko et al. Citation2017, Njue et al. Citation2019, Sermet et al. Citation2019) that complements those from traditional monitoring networks and field surveys (Starkey et al. Citation2017, Etter et al. Citation2018, Davids et al. Citation2019). The interest and proactive attitude of the general public can lead to new discoveries and improved modelling of hydrologic phenomena (Yang and Ng Citation2017).
At present, affordable electronic devices (smartphones, cameras, microcontrollers, smart watches, drones, etc.) are able to monitor not only the environment, but also biometrics (e.g. temperature, heart rate), geolocation i.e. Global Positioning System (GPS), and communications (Stefanidis et al. Citation2013, O’Grady et al. Citation2016, Yu et al. Citation2017, Tauro et al. Citation2018, Wang et al. Citation2018, Mao et al. Citation2019, Seibert et al. Citation2019, Sermet et al. Citation2019, Dixon et al. Citation2020). Geospatial models, data, and tools are also more accessible to a larger number of users (e.g. researchers, professionals, students) than ever before thanks to open-source licensing (e.g. GNU General Public Licence), volunteer developers, user-friendly interfaces, geospatial data processing, and web platforms (Ames et al. Citation2012, Goodchild et al. Citation2012, Gorelick et al. Citation2017, Dallery et al. Citation2020).
1.3 Online communities: a wealth of data and opportunities
Web-based digital platforms aggregate a growing number of users, enabling scale and performance in crowdsourcing (Fohringer et al. Citation2015, Le Boursicaud et al. Citation2016, Jollymore et al. Citation2017, Li et al. Citation2018, Sit et al. Citation2019). A group of crowdsourcers who share a common platform to cooperate for a common goal form an online community (OC) (definition in ). OCs are used in several settings to manage the relations among organizations and their stakeholders (Dellarocas Citation2006, Leidner et al. Citation2010), for mobilising people to lobby for common interests or causes (von Krogh et al. Citation2012, Braccini et al. Citation2019), for engaging individuals in the cooperative production of knowledge and innovation (Ma and Agarwal Citation2007, Faraj et al. Citation2011, Hutter et al. Citation2011, Majchrzak et al. Citation2013, Braccini et al. Citation2018), or for sharing knowledge and information of public interest (McLure Wasko and Faraj Citation2005). OCs can also increase engagement in and awareness of water-related challenges. At the same time, OCs present the risk of drifts in the understanding and practices of citizens in water management issues. These risks are significant especially when OCs adopt a social media presence which may be influenced by opinion biases, low scientific literacy, and/or organized forms of misinformation (e.g. “fake news”) (Baccarella et al. Citation2018).
Online communities allow citizen science programmes to facilitate the interaction among selected groups of citizens, such as users from a particular geographic region (e.g. country, municipality, river basin, or coastal zone), demographic category (e.g. gender, age, religion, social status), recreational (e.g. river fishing, sailing, hiking), or professional activity (e.g. engineers, educators, sociologists). The number of OCs, empowered by online tools and communities (Federici et al. Citation2015) and digital social networks (Liberatore et al. Citation2018), is significantly increasing. However, offline communities still play a major role in many issues in both developed and developing countries, and the two settings can also overlap.
Online communities can be applied to different aspects of water management. Engagement of OCs in emergencies constitutes one relevant framework in which crowdsourcing of hydrological information could be helpful for early detection, rapid response, and efficient recovery (e.g. See et al. Citation2016, Ernst et al. Citation2017).
1.4 Citizen science for water emergency management and regional planning
Several examples exist where crowdsourcing activities have become pivotal components of real-time emergency and post-disaster management actions (Poser and Dransch Citation2010, Albuquerque et al. Citation2015, Huang and Xiao Citation2015, Le Boursicaud et al. Citation2016, Yadav and Rahman Citation2016). In concordance, crowdsourcing is used in the field of natural hazards (). For instance, during the floods in South India in 2015, citizen science observations were used for real-time emergency management (Naik Citation2016, Pandey and Natarajan Citation2016, Yadav and Rahman Citation2016, Anbalagan and Valliyammai Citation2017). Furthermore, some citizen science projects successfully investigated and tested the technical and procedural implementation of well-informed, educated, and organized groups of citizens (named citizen observatories in most cases) as effective risk mitigation measures (Wehn et al. Citation2015, Montargil and Santos Citation2017, Paul et al. Citation2018).
The added value of citizen science is also leading to the integration of citizen observations and feedback into decision-making frameworks for participatory regional planning (Aretano et al. Citation2013, Kleinhans et al. Citation2015, Kahila-Tani et al. Citation2016). Citizen-driven efforts support effective cooperation and mutual trust among stakeholders. For instance, misunderstandings and conflicts that arise between water users and managers (e.g. disputes in water allocation among different geographic regions or economic sectors, as in the WEFE nexus) can be anticipated and mitigated with citizens’ involvement (IMoMo Citation2018). Citizens’ consensus, behaviour, perceptions, and social dynamics in general – all difficult to measure – can be quantified (or at least assessed) and integrated into environmental and urban resource planning through crowdsourcing (Lee et al. Citation2011, Huang and Xiao Citation2015, Xiao et al. Citation2015, Beigi et al. Citation2016, Michelsen et al. Citation2016, Tkachenko et al. Citation2017, Arthur et al. Citation2018, Witherow et al. Citation2018).
1.5 Citizen science to achieve transdisciplinarity in hydrological sciences
Citizen science approaches can enlarge the scale, impact, and “ground-truthing” of hydrological sciences (Buytaert et al. Citation2014, Afshari et al. Citation2018), fostering unique opportunities to develop transdisciplinary research (definition in ). Transdisciplinary hydrological research is a fundamental asset of studies aiming to address water challenges (e.g. water quality, water accessibility) and extremes (e.g. floods, droughts) while considering the interplay between socio-environmental factors that govern human–water systems (Ceola et al. Citation2016, Di Baldassarre et al. Citation2019).
While the technology that facilitates citizen science is mature with ready-to-use equipment and software broadly used across diverse disciplines (Wan et al. Citation2014, Mazzoleni et al. Citation2017, Tauro et al. Citation2018, Blöschl et al. Citation2019), standardization procedures are not. New policies are also needed that recognize citizen science as a cross-cutting priority, to support and regulate opportunistic sensing and unstructured information gathering, sharing, and use (Palsson et al. Citation2013, Wehn et al. Citation2015, Weber et al. Citation2019).
Conceptual transdisciplinary frameworks that integrate technical, administrative, and societal aspects to support the development of citizen science projects are lacking in the hydrological sciences. Some investigations developed such frameworks for citizen science methods, but, even if general, they lacked some aspects (e.g. administrative and policy assessment in Kieslinger et al. Citation2018), or referred just to data quality (Antelio et al. Citation2012) or were limited to specific disciplines and scopes and lacked generality and flexibility (Chase and Levine Citation2016). This hinders the progress of transdisciplinary research, limits the accumulation of knowledge, hampers a consistent implementation of the research results, and also makes the development of comparative assessments and evaluations of different citizen science projects challenging and inconsistent.
1.6 Outlook
In this study, we identify and introduce a conceptual framework that integrates crowdsourcing and behavioural mechanisms, to enable transdisciplinary interlinkages and assessments. The proposed framework aims to support the development of accumulated knowledge, avoid pitfalls, and maximize the effectiveness of joint efforts between citizen science projects. Such a conceptual scheme, therefore, is intended to foster advancements in the hydrological sciences by supporting the merging of outcomes from various citizen science initiatives and by allowing synergies amongst different sectors dealing with water challenges.
2 The topology of a transdisciplinary framework for citizen science in hydrology
2.1 Conceptualizing the topological framework
The diversity of concepts, definitions, data, models, tools, and procedures poses a challenge to designing a conceptual framework for citizen science in hydrology. Nonetheless, we propose a topological space to aid in the definition of such a framework and to initialize the discussions with the hydrological community. Four main elements, that are commonly shared by citizen science efforts addressing water issues, were identified: (a) citizens, (b) the hydrological sciences (hydrology, for simplicity), (c) technology, and (d) society. These four elements include different components whose interplay governs the development of citizen science projects in hydrology. The proposed elements and components are therefore topological properties shared by citizen science analysis, modelling, and assessment efforts for hydrological applications. The proposed citizen science topological space is schematically depicted in .
Figure 2. Topological space of a transdisciplinary framework applied to citizen science projects in hydrological sciences. The ellipses show the areas of interest and interfaces of the four main sub-spaces (related to the four main elements (bold): citizens, hydrology, technology and society). In bolded white are the four components (education, innovation, human sensors and human behaviours) that lie at the interface of all the four-element sub-spaces. The position of the components and the information included in each sub-space depict the relationships and shared spaces among the components, but are meant to be illustrative, not exhaustive.
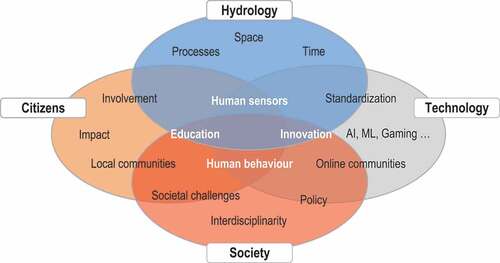
2.2 The dual value of citizens’ involvement: human sensors and human behaviour
Two new major components emerge, together with education and innovation, in the proposed topological space as representative of the main facets of the citizens–hydrology–technology–society interface: human sensors and human behaviour (definitions in ).
In the data–information–knowledge creation and sharing process, citizens are both data producers while participating as observers, and data receivers while working as nodes of a distributed network (Paul et al. Citation2018). The term “human sensor” may, unintentionally, dehumanize the citizen; however, we advocate for this term because in many studies, the human component of citizen observations is largely neglected. It should be noted that, although we choose to use the “human sensors” terminology, we acknowledge that citizens can be engaged in citizen science to varying degrees (see Section 2.3). Nonetheless, to provide a schematic organization of the “human sensors” component, we identify two main categories:
Direct citizen observations: Information produced by involved citizens who utilize custom-made applications for the observation of predetermined phenomena or landscape features to develop specific modelling or monitoring activities. An increasing number of web and smartphone applications were recently established that fall within this category, as in the case of citizens collecting images and videos to monitor water parameters, land cover, or other geophysical parameters (Assumpção et al. Citation2018, Zheng et al. Citation2018, Seibert et al. Citation2019);
Indirect citizen observations: The gathering and processing of information shared by citizens for other purposes, such as citizens spontaneously posting videos and images in online social networks of unusual river conditions (floods, droughts, pollutants, debris, etc.). These pieces of information may then be, instantaneously or subsequently, used by scientists for real-time water monitoring or post-event fluvial studies (Mazzoleni et al. Citation2017, Tkachenko et al. Citation2017, Annis and Nardi Citation2019).
This classification was also introduced by Craglia et al. (Citation2012), as implicitly and explicitly contributed data, and by See et al. (Citation2016) as active and passive crowdsourced geographic information. Thus, the human sensors concept implies that in hydrology, citizen science data are not always generated for a scientific purpose, in opposition to traditional monitoring practices. The human sensors component, representing a human–machine–environment interface, provides qualitative and quantitative observations that can be used within quantitative hydrological studies, with careful attention to the accuracy and scale of these observations (Buytaert et al. Citation2014, Kosmala et al. Citation2016, Seibert and Vis Citation2016, Mazzoleni et al. Citation2019). See for the definition of “human sensors.”
Citizens’ behaviour (i.e. human conduct related to technical and social norms) and actions (i.e. activities performed in developing a task or accomplishing a goal) are a function of social, cultural, educational, psychological, and economic conditions. The “human behaviour” component refers, thus, to the subjectivity of human habits, motivation, perceptions, and dynamics concerning the input citizens receive or concerning the output (or actions) citizens produce. In particular, “human behaviour” refers to how citizens subjectively extract knowledge from the data, modify their actions, or change their behaviour as a result of the gathered knowledge. See for the definition of “human behaviour.”
The “human sensors” component is widely explored in citizen science projects for the hydrological sciences (Zheng et al. Citation2018). In contrast, the “human behaviour” component represents an unexplored potential for hydrology. The interaction of hydrological sciences with social sciences, cognitive sciences, and psychology, among other disciplines investigating human behaviour, represents a pivotal asset of transdisciplinary citizen science projects. Hydrological models, supported by citizen science, should consider the quantitative integration of human behaviour for understanding and simulating water processes influenced by humans. The “human sensors” and “human behaviour” components are both essential for better understanding and forecasting hydrological dynamics in river basins, where human–water interactions govern water cycle variables and determine short- to long-term hydrological change (Di Baldassarre et al. Citation2013b, 2016, Pande and Sivapalan Citation2017, Citation2019).
The proposed characterization of the “human sensors” and “human behaviour” components suggests the depiction of an iterative cycle associated with citizens’ engagement: data–information–knowledge–behaviour–action. This concept is derived from the data–information–knowledge–wisdom framework (Bernstein Citation2011). Considering that actions also support data production, this looping iteration applies whenever citizens’ behavioural mechanisms are triggered by citizen science (i.e. observation) methods, supporting information and knowledge production and exchange. We posit that this iterative cycle is involved for all citizen science-driven workflows, and therefore also includes hydrological studies ().
2.3 Thematic areas of a transdisciplinary framework for advancing hydrological sciences
In this section, we explore the most relevant research prospects and gaps that should be addressed for advancing and connecting the hydrological sciences with academic and non-academic disciplines. Seven thematic areas are proposed, and the related transdisciplinary interlinkages are identified in and described below.
Figure 4. The Citizens and Hydrology (CANDHY) transdisciplinary research framework, with the disciplines of interest categorized into the four main elements (citizens, hydrology, technology and society) of the topological model and the seven selected thematic areas (in the coloured boxes).
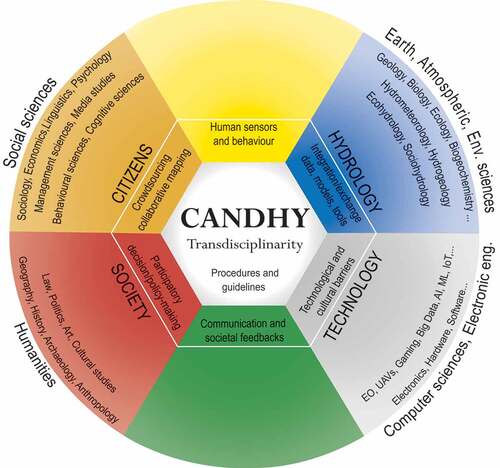
2.3.1 Theme 1. Crowdsourced data and collaborative monitoring/mapping tools
This theme is discussed in terms of: (a) the tools and methods used by citizens for crowdsourced data gathering and processing; and (b) the crowdsourced data accuracy, quality, and specifications (e.g. spatial and temporal scale, resolution).
The first factor deals with existing or novel technologies used for monitoring that are available to citizens. While it is still possible to use analogue tools (Breuer et al. Citation2015) (e.g. interviews performed by volunteers, notes taken during field surveys, etc.), crowdsourcing tools are now mainly related to the use of mobile devices and web-based applications. In particular, significant advancements in the field of data collection and processing, involving the exploitation of information derived from crowdsourced data, are rapidly progressing in computer sciences. These also include methods for crawling indirect citizen observation data from online sources or methods for transforming qualitative or indirect data into quantitative hydrologically relevant information (Le Boursicaud et al. Citation2016, Michelsen et al. Citation2016, Restrepo-Estrada et al. Citation2018). Both tools and methods are gaining momentum provided by artificial intelligence (AI), and, in general, the rapidly advancing field of big data science, mining, and analytics (Kamar et al. Citation2012, Jeschke et al. Citation2018, Sermet et al. Citation2019).
The second factor pertains to the accuracy, quality, and technical specifications associated with crowdsourced data. The technical specifications associated with crowdsourced data define the reliability, robustness, flexibility, and scalability of the data. All these properties need to be considered and evaluated (Jollymore et al. Citation2017, Kieslinger et al. Citation2018). The spatial and temporal scales, the resolution, and several other parameters should also be defined before using such informal datasets. The main trade-off is having increasing volumes and frequency of data, but having to characterize crowdsourced data, vs using standard monitoring stations that can lead to limited discrete records (Davids et al. Citation2017). The data gathering, processing, production, and dissemination chain of crowdsourced data should follow quality standards, and these should be analysed and reported (Antelio et al. Citation2012). Standardization procedures that actually characterize traditional monitoring networks require innovations to include and exploit the opportunity offered by such data. Standard metadata structures are required to accommodate the heterogeneity of specifications associated with crowdsourced data. Finally, the design of online platforms to share and visualize crowdsourced data, implemented independently or jointly with traditional monitoring networks, should include optimal procedures for combining and/or assimilating each specific dataset, taking into account its quality and properties (see Theme 3).
2.3.2 Theme 2. Human sensors and behaviour analytics
Effective implementation of the “human sensors” and “human behaviour” components, to be jointly considered, represents the main value but also the major challenge of citizen science in hydrology. Citizens’ observations are influenced by diverse non-technical (e.g. social, cultural, psychological) conditions pertaining to the “human behaviour” component. Analogously, research aiming to examine or influence human behaviour should always consider environmental factors, as perceived and observed by citizens, and thus, interface with the “human sensors” component.
As a result, the joint “human sensors–behaviour” characterize the multiple and diverse instances of citizens’ participation and the varying influence of citizens’ behaviour on the data collection or on the participation process itself. At the same time, a change of human behaviour as a reaction to the gathered observation or knowledge will be analysed as well. It is, thus, crucial to analyse interactions between the “human sensors” and “human behaviour” components to understand their combined effects on the data–information–knowledge–behaviour–action workflow.
Nonetheless, while the randomness and subjectivity of human-derived observations and actions are valuable for citizen science projects in hydrology, obtaining different information from the same crowdsourcing methodology also represents a challenge, as hydrological data require consistency over space and time (Strobl et al. Citation2019a). This thematic area is, thus, also suitable to investigate how the hydrological sciences should connect with knowledge, expertise, and scientific reasoning from other disciplines. For example, transdisciplinary studies are needed to understand how conditions of observations vary with changing climatic and demographic settings such as country, education, gender, age, income, and migration status or with fatigue conditions and other biases linked to diverse socio-cultural conditions. This theme also promotes research on cognitive and psychological sciences, to analyse individual life conditions especially related to people at risk, under stress, and in critical situations, which is the case for both water security and hydrological risk management challenges (Sheth Citation2009, Resch et al. Citation2015).
The interface between “human sensors–behaviour” and hydrology represents a cross-cutting topic that requires transdisciplinarity, where natural, social, and computer science experts should join efforts. In particular, we see the behavioural and cognitive sciences, focusing on motivation, learning, and communications, as relevant to this theme (Oliveira et al. Citation2017). Moreover, gaming technologies, which can integrate advanced hydrodynamic simulations (Zadick et al. Citation2016, Jeschke et al. Citation2018), are also expected to provide transdisciplinary testing environments and favour the engagement of non-traditional audiences (Newman et al. Citation2012, Morschheuser et al. Citation2016, Radchuk et al. Citation2017, Aubert et al. Citation2018, Citation2019, Den Haan and Van der Voort Citation2018).
Citizen science faces several challenges related to the integration of the human sensors and human behaviour. Theme 2 inherits the issues identified in Theme 1, in particular those affecting the operational use of crowdsourced data. Additionally, the confidentiality and the heterogeneity of data related to behavioural mechanisms provide further complications. Privacy and security concerns become more relevant and impactful in establishing long-lasting, consistent, generalized, and scalable citizens’ engagement processes (Quinn Citation2018, Anhalt-Depies et al. Citation2019).
2.3.3 Theme 3. Integration and exchange of hydrological data, models, and tools
Although on-ground and remote sensing monitoring contribute to an increasing volume of hydrological data (Tomsett and Leyland Citation2019), large parts of the world suffer from water data scarcity, e.g. due to the degradation of traditional gauge networks and a lack of resources and commitment (McCabe et al. Citation2017, Manfreda et al. Citation2018, Tauro et al. Citation2018, World Bank Group Citation2018, Dixon et al. Citation2020). The usefulness of this scarce amount of data, if any, strongly relies on the accessibility, organization, and distribution of derived information for supporting research and innovations.
The availability and performances of water information systems have flourished in recent times (Demir and Krajewski Citation2013, Swain et al. Citation2015, Vitolo et al. Citation2015, Shukla et al. Citation2019). Water information systems are generally equipped with GIS and web–GIS interfaces implementing geospatial data models representing morphometric, environmental, hydrological, and socio-economic parameters associated with river basins and networks (Singh and Fiorentino Citation1996, Maguire et al. Citation2005, Whiteaker et al. Citation2006, Silberbauer Citation2019).
A number of regional and local water management agencies are investing relevant economic resources to extend their monitoring networks and develop ad hoc hardware and software for data gathering and sharing (e.g. web GIS, dashboards). Notably, examples exist of hydrological information systems covering large spatial scales and a wide variety of data sources (Addor et al. Citation2020), such as WMO Hydrological Observing System (WHOS), United States Geological Survey National Water Information System (USGS Nwis), Consortium of Universities for the Advancement of Hydrologic Science (CUAHSI) HydroShare, Water Information System for Europe (WISE), UNESCO-IHP Water Information Network System, World Resources Institute, and Global Runoff Data Centre (GRDC) databases. However, these systems rely solely on existing institutionalized data collection, and therefore reflect the uneven global distribution of observations, with significant gaps especially in low- and middle-income countries (World Bank Group Citation2018, de Bruijn et al. Citation2019, Crochemore et al. Citation2020). Moreover, satellite-based Earth observation (EO) systems are also improving considerably, capturing high-resolution spatial and temporal coverages of major Earth and water dynamics, with major efforts from governmental agencies (e.g. European Space Agency The Soil Moisture and Ocean Salinity (ESA SMOS), National Aeronautics and Space Administration Surface Water and Ocean Topography Mission (NASA SWOT)). EO services also provide readily available expert-use solution-oriented data and models for managing, monitoring, and mapping floods, droughts, land use, and urban change, among other things (Hewitt et al. Citation2012, Demeritt et al. Citation2013). EO platforms could also benefit from the integration of citizens’ observations (Fritz et al. Citation2017), from global to local crowdsourcing projects. Nonetheless, major obstacles and issues still impact the integration of unconventional citizen-driven information and tools with standard hydrological and EO information systems.
This theme describes the role of citizen science in the integration and exchange of heterogeneous data sources and in the development of software for hydrological sciences and across the wide spectra of disciplines interested in water issues.
2.3.4 Theme 4. Technological, institutional, and psychological/cultural barriers for the uptake of citizen science projects
Implementing citizen science projects for innovation in hydrological sciences is a scientific challenge, but psychological and cultural barriers also need to be considered (Elliott and Rosenberg Citation2019). The water resource and risk management sector is bound by diverse laws and norms developed by international, national, regional, and local authorities. They are designed to make the different parties (e.g. managers, professionals, scientists, governments, etc.) cooperate consistently and efficiently to monitor, protect, and allocate water resources while guaranteeing sustainable and safe human activities (Kallis and Butler Citation2001, Bubeck et al. Citation2017). However, such rules often do not include citizen science activities explicitly. The use of informal data and community engagement in policy and decision-making may even contrast with some of the current approaches, where government authorities are not used to cooperating and interacting with laypeople. As a result, in existing highly hierarchical top-down approaches, a psychological and cultural shift is needed to overcome and move towards greater collaboration and participation (see also Theme 6). It is also necessary to investigate how to benefit from existing local participatory methods that are not related to citizen science.
This theme investigates regulatory frameworks that support the effective integration of new technical and administrative specifications, including citizen science. Adaptive and flexible, yet consistent and robust, regulations are needed to support the transfer of hydrological science innovations in operational water information, policy, and decision-making systems empowered by citizen science. Three major factors are investigated in this theme: (a) regulations and norms about the tools and methods for collecting, sharing, using, and validating hydrological data from informal sources; (b) evaluation of participatory approaches as mechanisms for more inclusive decision-making; and (c) cost–benefit analyses of citizen science projects.
The first factor deals with the issue that specifications of crowdsourced data and crowdsourcing platforms for hydrological monitoring and modelling are not consistent with existing standards. Pictures taken from cell phones as compared to water level measurements gathered from standard flow gauges is a good example of the lack of consistency that hampers the impacts of citizen science on fostering technological innovations for hydrological sciences. For example, evacuation decision-making during flooding conditions only considers validated data from official standard flow monitoring gauges. Crowdsourced data may complement standard data used for disaster risk management, especially if supported by proper legal frameworks, norms, and quality control.
The second factor pertains to research on the impacts of including public participation in decision-making. Some studies indicate positive correlations between participation, awareness, compliance with the law, and improved management (Von Korff et al. Citation2012, Buchecker et al. Citation2013); therefore, European directives (e.g. Water Framework Directive, Floods Directive) incentivize participatory approaches. Nevertheless, most national and regional regulations do not consider participatory approaches, and significant political and cultural barriers impact citizen science uptake for more inclusive water governance and decision-making. To overcome these barriers, official authorities may benefit, by means of citizen science projects, from increased and wider involvement of citizens and stakeholders.
The third factor is linked to studies that address other reasons hindering the uptake of regulations supporting citizen science. These reasons are rooted not only in the reliability of the data but also in the lack of understanding by authorities of the costs of citizen science projects and how to adapt existing practices. Understanding the technologies used by scientists and citizens is fundamental, but not sufficient. Indeed, cost–benefit analyses that consider the entire technological and administrative burden associated with citizen science projects are needed. This theme, thus, also includes research on how to create locally adapted citizen science projects, to facilitate adoption, paving the way for parsimonious citizen science project implementation (Assumpcao et al. Citation2019).
2.3.5 Theme 5. Communicating water science and societal feedbacks
Scientific communication (definition in ) has moved from being solely the dissemination of scientific knowledge and research outcomes to the general public to encompassing public awareness, scientific literacy, and culture (Burns et al. Citation2003). This new purpose may stimulate behaviour change and actions from affected citizens and stakeholders. The scientific communication workflow includes multi-directional feedback between scientists and society, which can be further enhanced by citizen science (Bonney et al. Citation2009, Le Coz et al. Citation2016, Montargil and Santos Citation2017).
Communication and dissemination have become an integral part of research projects addressing hydrological issues. Citizen science constitutes a valid method for testing novel ways to exchange information between scientists and communities, particularly those affected by societal, climatic, and hydrological challenges. Citizen science projects represent a testbed for processing and conveying, to both experts and the general public, the transdisciplinary value of water as a resource, and disaster risk management.
This theme extends the proposed transdisciplinary framework to include studies on communication strategies for knowledge exchange and public feedback. It seeks to investigate, test, and discover new forms of visualization, infographics, and mapping, as well as new engagement models (e.g. demographics-targeted communication campaigns, online communities and social networks, and gaming technologies) that are explored and tested by scientists to reach a wider audience, from children to senior citizens (Schwabish Citation2020).
2.3.6 Theme 6. Collaborative and participatory efforts supporting decision-making and policymaking
Citizen science can help decision-making in many forms, most obviously by providing relevant data to authorities or by increasing participation levels in addressing societal challenges linked to water issues. The level and scale of citizens’ participation (Haklay Citation2013) determine the effectiveness and impact of citizen science projects in supporting or prompting a change in water governance (Buytaert et al. Citation2016), but are not the only possibilities. Challenges due to the segmentation and diversity of participatory approaches are identified. These have various implications for the uptake of citizen science for decision- and policy-making. Four major factors to be investigated include: (a) diversity of approaches and scales; (b) diversity of participants; (c) the working conditions (behaviour, safety, productivity, etc.) characterizing citizens when deploying data as human sensors; and (d) the trustworthiness and authoritativeness of citizen science data and projects.
The first factor relates to the collaborative process. Differences in citizen science approaches depend on the spatial and temporal scale of the phenomenon of interest. For example, different scales apply to participatory projects aiming to manage extreme events, that usually require high frequency and low data latency (i.e. real-time conditions), as in disaster risk management and emergency response (Eilander et al. Citation2016, Ernst et al. Citation2017, Sy et al. Citation2019). This last example contrasts with urban and regional monitoring activities that are characterized by low-frequency and long-term response times (Albano et al. Citation2015, Lisjak et al. Citation2017). The dynamics of the phenomena of interest also affect the behaviour component, since different citizen science approaches are implemented for emergency management, such as extreme river flows vs water sampling of regular river flows.
The second factor is linked to the diversity and often random, unpredictable behaviour of the participants. In this regard, we emphasize that human sensors–behaviour interactions not only vary from citizen to citizen but also vary in time and depend on the specific context. The same citizen in the same time frame may behave and operate differently depending on motivation, availability, and the technical and engagement methods used for prompting and implementing their involvement. In this regard, uncertainty analyses in crowdsourcing benefit from transdisciplinarity. In particular, the hydrological sciences are linking with statistical and demographic studies, as well as with social and psychological sciences, to deal with the randomness and bias associated with observations of natural and human phenomena (Seibert et al. Citation2019, Strobl et al. Citation2019b).
The third factor refers to the working conditions of humans “working as sensors”. In particular, there are diverse sensing conditions and processes that affect the quality and quantity of crowdsourcing records. The behaviour, safety, and productivity that impact the “human sensors” component need to be appropriately designed and managed. Research investigations should also consider the behaviour related to the use of dedicated tools for crowdsourced data production (e.g. the different effectiveness of information generated by citizens previously properly and purposely trained or by the volunteer data-gathering of untrained participants) (Strobl et al., Citation2019a). Further working conditions are represented by the analysis of potentially hazardous situations affecting citizens when sensing extreme phenomena and the potential risks of implementing citizen networks that may involve improper use of mobile devices in dangerous conditions.
The fourth factor deals with the operational use of crowdsourced data and crowdsourcing programmes for policy- and decision-making in water resource and disaster risk management. The previous factors, once addressed, may support the inclusion of crowdsourced data for integrating (or surrogating) traditional monitoring data. Nevertheless, policymakers and decision-makers may still be impacted by bounding conditions linked to actual standardized procedures that often avoid the full exploitation of the value of crowdsourcing. This bounding factor generally relies on the trustworthiness and authoritativeness of data gathering using citizen science methods. As an example, data gathered from a citizen science project supporting hydrological observations using a mobile application (e.g. Crowdwater – Strobl et al., Citation2019b or Scent project – Tserstou et al. Citation2017), even if consistent with standard river flow observations, may be disregarded for policy- and decision-making. In the context of flooding, direct observations, even if transmitted in a timely manner, cannot be used for evacuation planning (with some exceptions, e.g. Naik Citation2016, Pandey and Natarajan Citation2016, Yadav and Rahman Citation2016, Anbalagan and Valliyammai Citation2017).
It is expected that policymakers and decision makers will increasingly integrate citizen science in their decision-making, but how exactly this will happen remains unclear. This theme explores how hydrological scientists will collaborate with jurisdictional and policy experts, as well as with communication experts, psychologists, and other non-hydrologists, to support the operational use of crowdsourced data. It is therefore crucial to test shared procedures (i.e. shared among the plethora of heterogeneous components discussed earlier) and, consequently, identify and test novel guidelines allowing trans-sectorial participatory decision- and policymaking.
2.3.7 Theme 7. Procedures and guidelines for the integration of citizen science into hydrological research
New procedures and guidelines are needed for valuing citizen science in hydrology. Citizen science projects are lacking comparable data gathering, modelling, and integrated assessment schemes (Haywood and Besley Citation2014, Burgess et al. Citation2017). The many examples of diverse citizen science projects confirm that these schemes are hard to find. It is difficult to even analyse and compare different methodologies within similar projects sharing the same goal (e.g. water level monitoring) in hydrological sciences. The integration of the full range of heterogeneous and diverse water-related citizen science data in a robust assessment framework is challenging, but essential to achieve. From a technical point of view, comparative considerations on the quality and quantity of data, plus quality assurance, should be made to support transdisciplinary research programmes that aim to use such diverse data and tools. Also, it is necessary to evaluate data models and fuzzy methods that can incorporate multiple datasets into modelling frameworks (Malczewski Citation2006). Comparative assessments are fundamental to building knowledge from diverse citizen science models and outcomes. Additionally, heterogeneity, gaps, flexibility, interoperability, scalability, and data assimilation should be evaluated. Investigations on model calibration and validation procedures are also needed to accommodate the varying formats, uncertainties, and availability of crowdsourcing data. In turn, models optimized for crowdsourced data can be compared with models built with expert-sourced observed data, standard observations, or previously validated simulated variables.
This theme seeks to define and develop procedures and guidelines – as set out in the proposed conceptual transdisciplinary framework – and establish synergies among, as well as take into account the opportunities and caveats characterized in, the previous six themes. It constitutes a synthesis of the technical and non-technical, methodological, and procedural challenges and solutions introduced in this section. It aims to develop consistent assessments of citizen science projects and frameworks and accumulated knowledge towards the maturing of transdisciplinary citizen science projects in the context of finding integrative solutions to water challenges.
3 The CANDHY Working Group at the International Association of Hydrological Sciences
The Citizens and Hydrology (CANDHY; logo in ) Working Group (WG) was established in July 2017 by the International Association of Hydrological Sciences (IAHS). The principal aim of the CANDHY WG is to support the use of citizen science in hydrology and harmonize research in this context, promoting the value of citizen science for advancing the hydrological sciences and finding answers to the most pressing open scientific, technical, and societal challenges in this field of expertise. This paper identifies the fundamental components, thematic areas, and specifications of citizen science projects giving them structure and direction to advance research and achieve scientific breakthroughs in hydrology.
Figure 5. The logo of the International Association of Hydrological Sciences (IAHS) Citizens AND HYdrology (CANDHY) working group.
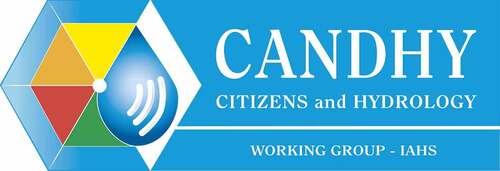
The citizens and hydrology topic is aligned with major programmes and efforts of the IAHS. IAHS launched and catalysed the Predictions in Ungauged Basins (PUB) Decade (2003–2012) (Blöschl et al. Citation2013, Hrachowitz et al. Citation2013) and the ongoing Panta Rhei Decade (2013–2022) (Montanari et al. Citation2013, McMillan et al. Citation2016) for promoting and coordinating scientific efforts for achieving improved hydrological data and models. CANDHY WG cooperates with the IAHS Measurements and Observations in the XXI century (MOXXI) WG, whose mission and goals are to address Panta Rhei science questions 1 and 5 that focus, respectively, on the identification of key gaps in the understanding of hydrological change, and on advancing monitoring and data analysis capabilities, also through opportunistic sensing, to predict and better manage hydrological change (Tauro et al. Citation2018). The CANDHY WG contributed to the Unsolved Problems in Hydrology (UPH) initiative, defining the UPHs of the “Measurement and data” section (UPHs 16, 17, 18) as well as the questions about “Interfaces with society” (UPHs 21, 22, 23) (Blöschl et al. Citation2019).
The CANDHY WG aims to promote the development of citizen science projects linking Earth, environmental, atmospheric, and hydro-sciences as well as humanities, social, and computer sciences, to synergistically define methods, procedures, and guidelines for the effective use of informal data and to foster participatory solutions for water challenges.
4 Citizen science in hydrological sciences: the way forward
While citizen science initiatives show promising results, there are still several challenges to be addressed. Evaluations of effective costs and benefits of citizen science projects need to be critically debated. Technology becomes obsolete very quickly and data infrastructure needs to be maintained. Short-term financing schemes and fragmentation affect the sustainability and long-term achievements of citizen science projects. Intellectual property, licensing, and data protection are serious challenges that need to be managed (Quinn Citation2018). Relevant opportunities offered by citizens’ distributed monitoring networks and affordable opportunistic sensing require government and financial support, multi-sectoral coordination, and long-term vision (Dixon et al. Citation2020).
The impact of citizen science in hydrology depends on drivers and involvement from actors and stakeholders of participatory outcomes. It is not only a matter of extending the participation. What is needed is a commitment to co-generate citizen science programmes so that stronger links, mutual trust, and shared beliefs among researchers, citizens, and policymakers are developed (Stahl et al. Citation2017, Njue et al. Citation2019).
Citizen science is expected to reinforce the value and mission of socio-hydrology in understanding and addressing sustainable development and environmental issues (Pande and Sivapalan Citation2017, Di Baldassarre et al. Citation2019, Fritz et al. Citation2019). Approaches for connecting citizen science and socio-hydrology are still not well defined, but initial investigations on this topic are emerging (Buarque et al. Citation2020).
The next steps needed to allow citizen science to reach its full potential for hydrological sciences are through the following major research lines of action:
Understand how crowdsourced data and actions generate hydrological knowledge and how this should be formalized in models and hydrological studies. Research and standardization efforts are needed to address data quality, data validation, and data interoperability.
To date, most of the hydrologic information systems seem to be developed as top-down programmes (e.g. USGS Hydrosheds; Australian Water Observations from Space – WOfS; EU Global Surface Water Explored – GSWD) and do not allow for the integration of informal data processing methods resulting from citizen science. It is necessary to learn from successful examples, such as collaborative mapping projects like OpenStreetMap, involving thousands of volunteer mappers daily updating street/urban features. Hydrologists should seek a common, inclusive, and open platform fostering the integration of crowdsourcing with standard hydrologic monitoring and geospatial mapping data.
A growing number of hydrological studies use data from social networks, but most of the crowdsourced data used in research are still indirect citizens’ observations. Hydrological scientists may need to focus on creating efficient tools and resources (e.g. smartphone apps, web platforms, training, awareness, and communication campaigns) to incorporate the data collected through social networks. Novel paradigms and disciplines may be needed to support an increasing volume and effectiveness of social media content for hydrological sciences.
Gaming technologies have successfully integrated numerical algorithms developed by hydrologists. Advanced hydrodynamic simulations of water dynamics are, in fact, now embedded in commercial gaming console applications and used daily by millions of users of any nationality and age. Hydrologists should take advantage of the growing interest, audience, and skills raised by the gaming industry, big data, artificial intelligence, machine learning, and augmented reality technologies. Collaborative virtual gaming and big data environments represent a great opportunity for citizen science in support of hydrological sciences.
Citizen science represents an opportunity for discovery and testing novel educational programmes to be proposed at different levels (from doctoral research to elementary schools). Hydrological sciences should focus on the opportunities offered by citizen science for innovating and proposing transdisciplinary multi-level education methods and programmes.
Engaged, informed, and concerned citizens are essential for managing the crucial natural resource that is water. Eventually, in this era of increased risk of disinformation, citizen science also represents a way to rebuild trust between citizens, science, and authorities. Trustworthiness and authoritativeness issues are, and will continue to be, a critical topic where scientific knowledge and methods should play a governing role in support of well informed decision- and policymaking.
There are close analogies between the diversity, heterogeneity, and complexity of human beings and hydrologic phenomena. Human and water systems will continue to shape each other and interplay. The knowledge, studies, and solutions that have separately emerged from social and hydrological sciences will need to merge. Disciplinary boundaries of any further discipline able to understand, monitor, and influence the coupled human–water sensors and behaviour should be more permeable. Novel water governance solutions and strategies can be investigated through citizen science projects, and this represents an opportunity for scientists, stakeholders, decision makers and policymakers to develop and learn together.
We think that the proposed transdisciplinary framework may pave the way for a conceptualization and assessment model for citizen science projects addressing water challenges. The proposed CANDHY framework, with its elements, components, and interfaces, may be used to evaluate and compare the completeness, effectiveness, scalability, and replicability of citizen science projects. The CANDHY transdisciplinary framework is a first step towards new knowledge and the integration of crowdsourced data, tools, procedures, and policies produced in diverse academic and non-academic settings. We posit that the CANDHY transdisciplinary framework is a suitable means for valuing citizen science projects in hydrological sciences, with even broader applicability across many natural and social sciences.
Acknowledgements
Two anonymous reviewers provided ideas and comments that greatly helped to improve this paper. The IAHS CANDHY Working Group is continuously welcoming new members, citizens and hydrologists, interested in joining efforts and contributing to the CANDHY mission. To become a CANDHY friend – a member of the working group – and stay up to date, please visit the webpage https://iahs.info/Commissions--W-Groups/Working-Groups/Candhy.do
Disclosure statement
No potential conflict of interest was reported by the authors.
References
- Abbott, B.W., et al., 2019. Human domination of the global water cycle absent from depictions and perceptions. Nature Geoscience, 12 (7), 533–540. doi:10.1038/s41561-019-0374-y
- Addor, N., et al., 2020. Large-sample hydrology: recent progress, guidelines for new datasets and grand challenges. Hydrological Sciences Journal, 65 (5), 712–725. doi:10.1080/02626667.2019.1683182
- Afshari, S., et al., 2018. Comparison of new generation low-complexity flood inundation mapping tools with a hydrodynamic model. Journal of Hydrology, 556, 539–556. doi:10.1016/j.jhydrol.2017.11.036
- Ajzen, I., 1991. The theory of planned behavior. Organizational behavior and human decision processes. Journal of Psychology & Health, 26 (9), 1113–1127. doi:10.1016/0749-5978(91)90020-T
- Albano, R., Sole, A., and Adamowski, J., 2015. READY: A web-based geographical information system for enhanced flood resilience through raising awareness in citizens. Natural Hazards and Earth System Sciences, 15 (7), 1645–1658. doi:10.5194/nhess-15-1645-2015
- Albuquerque, J.P., et al., 2015. A geographic approach for combining social media and authoritative data towards identifying useful information for disaster management. International Journal of Geographical Information Science, 29 (4), 667–689. doi:10.1080/13658816.2014.996567
- Ames, D.P., et al., 2012. HydroDesktop: web services-based software for hydrologic data discovery, download, visualization, and analysis. Environmental Modelling and Software, 37, 146–156. doi:10.1016/j.envsoft.2012.03.013
- Anbalagan, B. and Valliyammai, C., 2017. #ChennaiFloods: leveraging human and machine learning for crisis mapping during disasters using social media. In: Proceedings - 23rd IEEE International Conference on High Performance Computing Workshops, HiPCW 2016, 19 December 2016 to 22 December 2016, Hyderabad, India.
- Anhalt-Depies, C., et al., 2019. Tradeoffs and tools for data quality, privacy, transparency, and trust in citizen science. Biological Conservation, 238, 108195. doi:10.1016/j.biocon.2019.108195
- Annis, A. and Nardi, F., 2019. Integrating VGI and 2D hydraulic models into a data assimilation framework for real time flood forecasting and mapping. Geo-spatial Information Science, 22 (4), 223–236. doi:10.1080/10095020.2019.1626135
- Antelio, M., et al., 2012. Qualitocracy: A data quality collaborative framework applied to citizen science. In: Proceedings of IEEE International Conference on Systems, Man and Cybernetics, 931–936. doi:10.1109/ICSMC.2012.6377847
- Aretano, R., et al., 2013. People perception of landscape change effects on ecosystem services in small Mediterranean islands: A combination of subjective and objective assessments. Landscape and Urban Planning, 112 (1), 63–73. doi:10.1016/j.landurbplan.2012.12.010
- Arthur, R., et al., 2018. Social sensing of floods in the UK. PLoS One, 13 (1). doi:10.1371/journal.pone.0189327
- Assumpcao, T.H., et al., 2019. Citizens’ campaigns for environmental water monitoring: lessons from field experiments. IEEE Access, 7, 134601–134620. doi:10.1109/ACCESS.2019.2939471
- Assumpção, T.H., et al., 2018. Citizen observations contributing to flood modelling: opportunities and challenges. Hydrology and Earth System Sciences, 22 (2), 1473–1489. doi:10.5194/hess-22-1473-2018
- Aubert, A.H., Bauer, R., and Lienert, J., 2018. A review of water-related serious games to specify use in environmental multi-criteria decision analysis. Environmental Modelling and Software, 105, 64–78. doi:10.1016/J.ENVSOFT.2018.03.023
- Aubert, A.H., Medema, W., and Wals, A.E.J., 2019. Towards a framework for designing and assessing game-based approaches for sustainable water governance. Water, 11 (4), 869. doi:10.3390/w11040869
- Aulov, O., Price, A., and Halem, M., 2014. AsonMaps: a platform for aggregation, visualization and analysis of disaster related human sensor network observations. In: Proceedings of the 11th International Conference on Information Systems for Crisis Response and Management, May 2014, University Park, PA, 802–806.
- Baccarella, C.V., et al., 2018. Social media? It’s serious! Understanding the dark side of social media. European Management Journal, 36 (4), 431–438. doi:10.1016/j.emj.2018.07.002
- Banwart, S.A., et al., 2013. Sustaining earth ’s critical zone basic science and interdisciplinary solutions for global challenges. Sheffield: University of Sheffield, 48.
- Beigi, G., et al., 2016. An overview of sentiment analysis in social media and its applications in disaster relief. Studies in Computational Intelligence, 639, 313–340. doi:10.1007/978-3-319-30319-2_13
- Bernstein, J.H., 2011. The data-information-knowledge-wisdom hierarchy and its antithesis. NASKO. doi:10.7152/nasko.v2i1.12806
- Beven, K., et al., 2020. Developing observational methods to drive future hydrological science: can we make a start as a community? Hydrological Processes, 34 (3), 868–873. doi:10.1002/hyp.13622
- Blöschl, G., et al., 2013. Runoff prediction in ungauged basins: synthesis across processes, places and scales. Runoff predict. Ungauged basins synth. Across process. Places scales. Cambridge: Cambridge University Press.
- Blöschl, G., et al., 2019. Twenty-three unsolved problems in hydrology (UPH)–a community perspective. Hydrological Sciences Journal, 64 (10), 1141–1158. doi:10.1080/02626667.2019.1620507
- Bonney, R., et al., 2009. Citizen science: a developing tool for expanding science knowledge and scientific literacy. Bioscience, 59 (11), 977–984. doi:10.1525/bio.2009.59.11.9
- Brabham, D.C., et al., 2014. Crowdsourcing applications for public health. American Journal of Preventive Medicine, 46 (2), 179–187. doi:10.1016/j.amepre.2013.10.016
- Braccini, A.M., Sæbø, Ø., and Federici, T., 2019. From the blogosphere into the parliament: the role of digital technologies in organizing social movements. Information and Organization, 29 (3), 100250. doi:10.1016/j.infoandorg.2019.04.002
- Braccini, A.M., Za, S., and Sæbø, Ø., 2018. A collaborative discourse or only a collection of voices? An exploratory study of the use of social media in the e-Participation domain. Research Papers, 177. https://aisel.aisnet.org/ecis2018_
- Brantley, S.L., et al., 2016. Designing a suite of measurements to understand the critical zone. Earth Surface Dynamics, 4 (1), 211–235. doi:10.5194/esurf-4-211-2016
- Breuer, L., et al., 2015. HydroCrowd: a citizen science snapshot to assess the spatial control of nitrogen solutes in surface waters. Scientific Reports, 5 (1), 16503. doi:10.1038/srep16503
- Brondizio, E.S., et al., 2016. Re-conceptualizing the anthropocene: A call for collaboration. Global Environmental Change, 39, 318–327. doi:10.1016/j.gloenvcha.2016.02.006
- Buarque, S., et al., 2020. Using historical source data to understand urban flood risk: a socio-hydrological modelling application at Gregório Creek, Brazil. Hydrological Sciences Journal, 65 (7), 1075–1083. doi:10.1080/02626667.2020.1740705
- Bubeck, P., et al., 2017. Explaining differences in flood management approaches in Europe and in the USA – a comparative analysis. Journal of Flood Risk Management, 10 (4), 436–445. doi:10.1111/jfr3.12151
- Buchecker, M., Menzel, S., and Home, R., 2013. How much does participatory flood management contribute to stakeholders’ social capacity building? Empirical findings based on a triangulation of three evaluation approaches. Natural Hazards & Earth System Sciences, 13 (6). doi:10.5194/nhess-13-1427-2013
- Bui, E.N., 2016. Data-driven critical zone science: a new paradigm. Science of the Total Environment, 568, 587–593. doi:10.1016/j.scitotenv.2016.01.202
- Burgess, H.K., et al., 2017. The science of citizen science: exploring barriers to use as a primary research tool. Biological Conservation, 208, 113–120. doi:10.1016/J.BIOCON.2016.05.014
- Burns, T.W., O’Connor, D.J., and Stocklmayer, S.M., 2003. Science communication: a contemporary definition. Public Understanding of Science. doi:10.1177/09636625030122004
- Buytaert, W., et al., 2014. Citizen science in hydrology and water resources: opportunities for knowledge generation, ecosystem service management, and sustainable development. Frontiers in Earth Science, 2 (26), 1–21. doi:10.3389/feart.2014.00026
- Buytaert, W., et al., 2016. Citizen science for water resources management: toward polycentric monitoring and governance? Journal of Water Resources Planning and Management, 142 (4), 01816002. doi:10.1061/(ASCE)WR.1943-5452.0000641
- Carmona-Moreno, C., Dondeynaz, C., and Biedler, M., 2018. Position paper on Water, Energy, Food and Ecosystems (WEFE) nexus and Sustainable Development Goals (SDGs). doi:10.2760/5295
- Ceola, S., et al., 2016. Adaptation of water resources systems to changing society and environment: a statement by the international association of hydrological sciences. Hydrological Sciences Journal, 61 (16), 2803–2817. doi:10.1080/02626667.2016.1230674
- Chase, S.K. and Levine, A., 2016. A framework for evaluating and designing citizen science programs for natural resources monitoring. Conservation Biology, 30 (3), 456–466. doi:10.1111/cobi.12697
- Chawla, L. and Cushing, D.F., 2007. Education for strategic environmental behavior. Environmental Education Research, 13 (4), 437–452. doi:10.1080/13504620701581539
- Connor, R., et al., 2020. Chapter 9 – water-climate-energy-food-environment nexus. The United Nations World Water Development Report 2020 ‘Water and Climate Change’, 118–125. https://en.unesco.org/themes/water-security/wwap/wwdr/2020
- Conrad, C.C. and Hilchey, K.G., 2011. A review of citizen science and community-based environmental monitoring: issues and opportunities. Environmental Monitoring and Assessment, 176, 273–291. doi:10.1007/s10661-010-1582-5
- Craglia, M., Ostermann, F., and Spinsanti, L., 2012. Digital earth from vision to practice: making sense of citizen-generated content. International Journal of Digital Earth, 5 (5), 398–416. doi:10.1080/17538947.2012.712273
- Crochemore, L., et al., 2020. Lessons learnt from checking the quality of openly accessible river flow data worldwide. Hydrological Sciences Journal, 65 (5), 699–711. doi:10.1080/02626667.2019.1659509
- Cudennec, C., et al., 2018. Epistemological dimensions of the water–energy–food nexus approach: reply to discussions of “challenges in operationalizing the water–energy–food nexus.” Hydrological Sciences Journal, 63 (12), 1868–1871. doi:10.1080/02626667.2018.1545097
- Cudennec, C., et al., 2016. Hydrometeorology and hydroclimate. Advances in Meteorology, 1487890, 4. doi:10.1155/2016/1487890
- Cudennec, C., et al., 2020. Towards FAIR and SQUARE hydrological data. Hydrological Sciences Journal, 65 (5), 681–682. doi:10.1080/02626667.2020.1739397
- Cunha, D.G.F., et al., 2017. Citizen science participation in research in the environmental sciences: key factors related to projects’ success and longevity. Anais Da Academia Brasileira De Ciências, 89 (3), 2229–2245. doi:10.1590/0001-3765201720160548
- D’Odorico, P., et al., 2018. The global food-energy-water nexus. Reviews of Geophysics, 56, 456–531. doi:10.1029/2017RG000591
- Dallery, D., et al., 2020. An end user friendly hydrological web service for hydrograph prediction in ungauged basins. Hydrological Sciences Journal, (in press). https://doi.org/10.1080/02626667.2020.1797045
- Davids, J.C., et al., 2019. Citizen science flow – an assessment of simple streamflow measurement methods. Hydrology and Earth System Sciences, 23 (2), 1045–1065. doi:10.5194/hess-23-1045-2019
- Davids, J.C., van de Giesen, N., and Rutten, M., 2017. Continuity vs. the crowd—tradeoffs between continuous and intermittent citizen hydrology streamflow observations. Environmental Management, 60 (1), 12–29. doi:10.1007/s00267-017-0872-x
- de Bruijn, J.A., et al., 2019. A global database of historic and real-time flood events based on social media. Scientific Data, 6 (1), 311. doi:10.1038/s41597-019-0326-9
- Dellarocas, C., 2006. Strategic manipulation of internet opinion forums: implications for consumers and firms. Management Science, 52 (10), 1577–1593. doi:10.1287/mnsc.1060.0567
- Demeritt, D., et al., 2013. The European flood alert system and the communication, perception, and use of ensemble predictions for operational flood risk management. Hydrological Processes, 27 (1), 147–157. doi:10.1002/hyp.9419
- Demir, I. and Krajewski, W.F., 2013. Towards an integrated flood information system: centralized data access, analysis, and visualization. Environmental Modelling and Software, 50, 77–84. doi:10.1016/J.ENVSOFT.2013.08.009
- Den Haan, R.-J. and Van der Voort, M.C., 2018. On evaluating social learning outcomes of serious games to collaboratively address sustainability problems: a literature review. Sustainability, 10 (12), 4529. doi:10.3390/SU10124529
- Di Baldassarre, G., et al., 2013a. Towards understanding the dynamic behaviour of floodplains as human-water systems. Hydrology and Earth System Sciences, 17 (8), 3235–3244. doi:10.5194/hess-17-3235-2013
- Di Baldassarre, G., et al., 2013b. Socio-hydrology: conceptualising human-flood interactions. Hydrology and Earth System Sciences, 17 (8), 3295. doi:10.5194/hess-17-3295-2013
- Di Baldassarre, G., et al., 2019. Sociohydrology: scientific challenges in addressing the sustainable development goals. Water Resources Research, 55 (8), 6327–6355. doi:10.1029/2018WR023901
- Di Baldassarre, G., Brandimarte, L., and Beven, K., 2016. The seventh facet of uncertainty: wrong assumptions, unknowns and surprises in the dynamics of human–water systems. Hydrological Sciences Journal, 61 (9), 1748–1758. doi:10.1080/02626667.2015.1091460
- Dixon, H., et al., 2020. Intergovernmental cooperation for hydrometry – what, why, how? Hydrological Sciences Journal, (in press). doi:10.1080/02626667.2020.1764569
- Eilander, D., et al., 2016. Harvesting social media for generation of near real-time flood maps. Procedia Engineering, 154, 176–183. doi:10.1016/j.proeng.2016.07.441
- Eitzel, M.V., et al., 2017. Citizen science terminology matters: exploring key terms. Citizen Science: Theory and Practice, 2 (1). doi:10.5334/cstp.96
- Elliott, K.C. and Rosenberg, J., 2019. Philosophical foundations for citizen science. Citizen Science: Theory and Practice, 4 (1). doi:10.5334/cstp.155
- Ernst, C., Mladenow, A., and Strauss, C., 2017. Collaboration and crowdsourcing in emergency management. International Journal of Pervasive Computing and Communications, 13 (2), 176–193. doi:10.1108/IJPCC-03-2017-0026
- Estellés-Arolas, E. and González-Ladrón-De-Guevara, F., 2012. Towards an integrated crowdsourcing definition. Journal of Information Science, 38 (2), 189–200. doi:10.1177/0165551512437638
- Etter, S., et al., 2018. Value of uncertain streamflow observations for hydrological modelling. Hydrology and Earth System Sciences, 22 (10), 5243–5257. doi:10.5194/hess-22-5243-2018
- Faraj, S., Jarvenpaa, S.S.L., and Majchrzak, A., 2011. Knowledge collaboration in online communities. Organization Science, 22 (5), 1224–1239. doi:10.1287/orsc.1100.0614
- Federal Crowdsourcing and Citizen Science Catalog, 2020. https://www.citizenscience.gov/catalog [ Accessed 5 August 2020].
- Federici, T., Braccini, A.M., and Sæbø, Ø., 2015. ‘Gentlemen, all aboard!’ ICT and party politics: reflections from a mass-eParticipation experience. Government Information Quarterly, 32 (3), 287–298. doi:10.1016/j.giq.2015.04.009
- Fohringer, J., et al., 2015. Social media as an information source for rapid flood inundation mapping. Natural Hazards and Earth System Sciences, 15 (12), 2725–2738. doi:10.5194/nhess-15-2725-2015
- Follett, R. and Strezov, V., 2015. An analysis of citizen science based research: usage and publication patterns. PLoS One, 10 (11), e0143687. doi:10.1371/journal.pone.0143687
- Fritz, S., et al., 2019. Citizen science and the United Nations sustainable development goals. Nature Sustainability, 2 (10), 922–930. doi:10.1038/s41893-019-0390-3
- Fritz, S., Fonte, C.C., and See, L., 2017. The role of citizen science in earth observation. Remote Sensing, 9 (4), 1–13. doi:10.3390/rs9040357
- Gandomi, A. and Haider, M., 2015. Beyond the hype: big data concepts, methods, and analytics. International Journal of Information Management, 35 (2), 137–144. doi:10.1016/j.ijinfomgt.2014.10.007
- Geiger, D. and Schader, M., 2014. Personalized task recommendation in crowdsourcing information systems - current state of the art. Decision Support Systems, 65, 3–16. doi:10.1016/j.dss.2014.05.007
- Goodchild, M.F., 2007. Citizens as sensors: the world of volunteered geography. GeoJournal, 69 (4), 211–221. doi:10.1007/s10708-007-9111-y
- Goodchild, M.F., et al., 2012. Next-generation digital earth. Proceedings of the National Academy of Sciences, 109 (28), 11088–11094. doi:10.1073/PNAS.1202383109
- Gorelick, N., et al., 2017. Google earth engine: planetary-scale geospatial analysis for everyone. Remote Sensing of Environment, 202, 18–27. doi:10.1016/j.rse.2017.06.031
- Gura, T., 2013. Citizen science: amateur experts. Nature, 496 (7444), 259–261. doi:10.1038/nj7444-259a
- Guswa, A.J., et al., 2020. Advancing ecohydrology in the 21st century: a convergence of opportunities. Ecohydrology, e2208. doi:10.1002/eco.2208
- Haklay, M., 2013. Citizen science and volunteered geographic information – overview and typology of participation. In: D.Z. Sui, S. Elwood, and M.F. Goodchild, eds. Crowdsourcing geographic knowledge: Volunteered Geographic Information (VGI) in theory and practice. Berlin: Springer, 105–122. doi:10.1007/978-94-007-4587-2_7
- Hayward, B., 2012. Children, citizenship and environment: nurturing a democratic imagination in a changing world. London, UK: Routledge. doi:10.4324/9780203106839
- Haywood, B.K. and Besley, J.C., 2014. Education, outreach, and inclusive engagement: towards integrated indicators of successful program outcomes in participatory science. Public Understanding of Science, 23 (1), 92–106. doi:10.1177/0963662513494560
- Hewitt, C., Mason, S., and Walland, D., 2012. The global framework for climate services. Nature Climate Change, 2 (12), 831–832. doi:10.1038/nclimate1745
- Howe, J., 2006. The rise of crowdsourcing. Wired Magazine, 14 (6), 1–4. doi:10.1086/599595
- Hrachowitz, M., et al., 2013. A decade of Predictions in Ungauged Basins (PUB)-a review. Hydrological Sciences Journal, 58 (6), 1198–1255. doi:10.1080/02626667.2013.803183
- Huang, Q. and Xiao, Y., 2015. Geographic situational awareness: mining tweets for disaster preparedness, emergency response, impact, and recovery. ISPRS International Journal of Geo-Information, 4 (3), 1549–1568. doi:10.3390/ijgi4031549
- Hutter, K., et al., 2011. Communitition: the tension between competition and collaboration in community-based design contests. Creativity and Innovation Management, 20 (1), 3–21. doi:10.1111/j.1467-8691.2011.00589.x
- IMoMo, 2018. Non-traditional data collection. https://www.imomohub.com/ [ Accessed 23 April 2020].
- Jeschke, S., et al., 2018. Water surface wavelets. ACM Transactions on Graphics, 37 (4), 1–13. doi:10.1145/3197517.3201336
- Jiang, S., et al., 2019. Advancing opportunistic sensing in hydrology: a novel approach to measuring rainfall with ordinary surveillance cameras. Water Resources Research, 55 (4), 3004–3027. doi:10.1029/2018WR024480
- Joint Research Centre, 2020. An inventory of citizen science activities for environmental policies. https://data.europa.eu/euodp/en/data/dataset/jrc-citsci-10004 [ Accessed 5 August 2020].
- Jollymore, A., et al., 2017. Citizen science for water quality monitoring: data implications of citizen perspectives. Journal of Environmental Management, 200, 456–467. doi:10.1016/j.jenvman.2017.05.083
- Kahila-Tani, M., et al., 2016. Let the citizens map—public participation GIS as a planning support system in the Helsinki master plan process. Planning Practice & Research, 31 (2), 195–214. doi:10.1080/02697459.2015.1104203
- Kallis, G. and Butler, D., 2001. The EU water framework directive: measures and implications. Water Policy, 3 (2), 125–142. doi:10.1016/S1366-7017(01)00007-1
- Kamar, E., Hacker, S., and Horvitz, E., 2012. Combining human and machine intelligence in large-scale crowdsourcing. Proceedings of the 11th International Conference on Autonomous Agents and Multiagent Systems AAMAS, 12, 467–474.
- Kieslinger, B., et al., 2018. The challenge of evaluation: an open framework for evaluating citizen science activities. SocArXiv. doi:10.31235/OSF.IO/ENZC9
- Kleinhans, R., Van Ham, M., and Evans-Cowley, J., 2015. Using social media and mobile technologies to foster engagement and self-organization in participatory urban planning and neighbourhood governance. Planning, Practice & Research, 30 (3), 237–247. doi:10.1080/02697459.2015.1051320
- Kosmala, M., et al., 2016. Assessing data quality in citizen science. Frontiers in Ecology and the Environment, 14 (10), 551–560. doi:10.1002/fee.1436
- Kullenberg, C. and Kasperowski, D., 2016. What is citizen science? – A scientometric meta-analysis. PLoS One, 11 (1), e0147152. doi:10.1371/journal.pone.0147152
- Le Boursicaud, R., et al., 2016. Gauging extreme floods on YouTube: application of LSPIV to home movies for the post-event determination of stream discharges. Hydrological Processes, 30 (1), 90–105. doi:10.1002/hyp.10532
- Le Coz, J., et al., 2016. Crowdsourced data for flood hydrology: feedback from recent citizen science projects in Argentina, France and New Zealand. Journal of Hydrology, 541, 766–777. doi:10.1016/j.jhydrol.2016.07.036
- Lee, C., Scherngell, T., and Barber, M.J., 2011. Investigating an online social network using spatial interaction models. Social Networks, 33 (2), 129–133. doi:10.1016/j.socnet.2010.11.002
- Leidner, D.E., Kock, H., and Gonzalez, E., 2010. Assimilating generation Y IT new hires into USAA’s workforce: the role of an enterprise 2.0 system. MIS Quarterly Executive, 9 (4), 229–242.
- Li, Z., et al., 2018. A novel approach to leveraging social media for rapid flood mapping: a case study of the 2015 South Carolina floods. Cartography and Geographic Information Science, 45 (2), 97–110. doi:10.1080/15230406.2016.1271356
- Liberatore, A., et al., 2018. Social media as a platform for a citizen science community of practice. Citizen Science: Theory and Practice, 3 (1), 1–14. doi:10.5334/cstp.108
- Lisjak, J., Schade, S., and Kotsev, A., 2017. Closing data gaps with citizen science? Findings from the Danube Region. ISPRS International Journal of Geo-Information, 6 (9), 277. doi:10.3390/ijgi6090277
- Liu, J., et al., 2017. Challenges in operationalizing the water–energy–food nexus. Hydrological Sciences Journal, 62 (11), 1714–1720. doi:10.1080/02626667.2017.1353695
- Liu, X., Vedlitz, A., and Shi, L., 2014. Examining the determinants of public environmental concern: evidence from national public surveys. Environmental Science & Policy, 39, 77–94. doi:10.1016/j.envsci.2014.02.006
- Loiselle, S.A., et al., 2016. Micro and macroscale drivers of nutrient concentrations in urban streams in South, Central and North America. PLoS One, 11 (9), e0162684. doi:10.1371/journal.pone.0162684
- Ma, M. and Agarwal, R., 2007. Through a glass darkly: information technology design, identity verification, and knowledge contribution in online communities. Information Systems Research, 18 (1), 42–67. doi:10.1287/isre.1070.0113
- Maguire, D.J., et al., 2005. GIS, spatial analysis, and modeling. USA: Esri Press, 480.
- Majchrzak, A., Wagner, C., and Yates, D., 2013. The impact of shaping on knowledge reuse for organizational improvement with wikis. MIS Quaterly, 37 (2), 455–469.
- Malczewski, J., 2006. GIS-based multicriteria decision analysis: A survey of the literature. International Journal of Geographical Information Science, 20 (7), 703–726. doi:10.1080/13658810600661508
- Manfreda, S., et al., 2018. On the use of unmanned aerial systems for environmental monitoring. Remote Sensing, 10 (4), 641. doi:10.3390/rs10040641
- Mao, F., et al., 2019. Low-cost environmental sensor networks: recent advances and future directions. Frontiers in Earth Science, 7, 221. doi:10.3389/FEART.2019.00221
- Mård, J., Di Baldassarre, G., and Mazzoleni, M., 2018. Nighttime light data reveal how flood protection shapes human proximity to rivers. Science Advances, 4 (8), 1–8. doi:10.1126/sciadv.aar5779
- Mazzoleni, M., et al., 2017. Can assimilation of crowdsourced data in hydrological modelling improve flood prediction? Hydrology and Earth System Sciences, 21 (2), 839–861. doi:10.5194/hess-21-839-2017
- Mazzoleni, M., et al., 2018. Towards assimilation of crowdsourced observations for different levels of citizen engagement: the flood event of 2013 in the Bacchiglione catchment. Hydrology and Earth System Sciences, 1–40. doi:10.5194/hess-2017-59
- Mazzoleni, M., Amaranto, A., and Solomatine, D.P., 2019. Integrating qualitative flow observations in a lumped hydrologic routing model. Water Resources Research, 55 (7). doi:10.1029/2018wr023768
- McCabe, M.F., et al., 2017. The future of Earth observation in hydrology. Hydrology and Earth System Sciences, 21 (7), 3879–3914. doi:10.5194/hess-21-3879-2017
- McLure Wasko, M. and Faraj, S., 2005. Why should i share? Examining social capital and knowledge contribution in electronic networks of practice. MIS Quaterly, 29 (1), 35–57. doi:10.2307/25148667
- McMillan, H., et al., 2016. Panta Rhei 2013-2015: global perspectives on hydrology, society and change. Hydrological Sciences Journal, 61 (7), 1174–1191. doi:10.1080/02626667.2016.1159308
- Melville, T., Baker, O., and Dolly, D., 2012. Spatial knowledge interchange environment: leveraging Web 2.0 technologies to breach the knowledge divide in agricultural development. In: GEOCROWD ‘12: Proceedings of the 1st ACM SIGSPATIAL International Workshop on Crowdsourced and Volunteered Geographic Information. doi:10.1145/2442952.2442966
- Michelsen, N., et al., 2016. YouTube as a crowd-generated water level archive. Science of the Total Environment, 568, 189–195. doi:10.1016/J.SCITOTENV.2016.05.211
- Montanari, A., et al., 2013. “Panta Rhei—everything flows”: change in hydrology and society—the IAHS scientific decade 2013–2022. Hydrological Sciences Journal, 58 (8), 1256–1275. doi:10.1080/02626667.2013.809088
- Montargil, F. and Santos, V., 2017. Citizen observatories: concept, opportunities and communication with citizens in the first EU experiences. In: A.A. Paulin, L.G. Anthopoulos, and C.G. Reddick, eds. Beyond bureaucracy: towards sustainable governance informatisation. Cham, Switzerland: Springer International Publishing, 167–184. ISBN: 978-3-319-54142-6.
- Morschheuser, B., Hamari, J., and Koivisto, J., 2016. Gamification in crowdsourcing: a review. In: Proceedings of the 49th Annual Hawaii International Conference on System Sciences (HICSS), 5-8 January. Hawaii, USA, 4375–4384 doi:10.1109/HICSS.2016.543
- Mukhtarov, F., Dieperink, C., and Driessen, P., 2018. The influence of information and communication technologies on public participation in urban water governance: A review of place-based research. Environmental Science & Policy, 89, 430–438. doi:10.1016/j.envsci.2018.08.015
- Naik, N., 2016. Flooded streets-A crowdsourced sensing system for disaster response: a case study. In: 2016 IEEE International Symposium on Systems Engineering (ISSE), 1–3. doi:10.1109/SysEng.2016.7753186
- Newman, G., et al., 2012. The future of citizen science: emerging technologies and shifting paradigms. Frontiers in Ecology and the Environment, 10 (6), 298–304. doi:10.1890/110294
- Njue, N., et al., 2019. Citizen science in hydrological monitoring and ecosystem services management: state of the art and future prospects. Science of the Total Environment, 693, 133531. doi:10.1016/j.scitotenv.2019.07.337
- O’Brien, K., Selboe, E., and Hayward, B.M., 2018. Exploring youth activism on climate change: dutiful, disruptive, and dangerous dissent. Ecology and Society, 23 (3). doi:10.5751/ES-10287-230342
- O’Grady, M.J., et al., 2016. Intelligent sensing for citizen science. Mobile Networks and Applications, 21 (2), 375–385. doi:10.1007/s11036-016-0682-z
- Oliveira, N., Jun, E., and Reinecke, K., 2017. Citizen science opportunities in volunteer-based online experiments. In: Proceedings of the 2017 CHI conference on human factors in computing systems, 6800–6812. doi:10.1145/3025453.3025473
- Palsson, G., et al., 2013. Reconceptualizing the ‘anthropos’ in the anthropocene: integrating the social sciences and humanities in global environmental change research. Environmental Science & Policy, 28, 3–13. doi:10.1016/J.ENVSCI.2012.11.004
- Pande, S. and Sivapalan, M., 2017. Progress in socio-hydrology: a meta-analysis of challenges and opportunities. Wiley Interdisciplinary Reviews: Water, 4 (4), e1193. doi:10.1002/wat2.1193
- Pandey, N. and Natarajan, S., 2016. How social media can contribute during disaster events? Case study of Chennai floods 2015. In: 2016 International Conference on Advances in Computing, Communications and Informatics (ICACCI), 1352–1356). doi:10.1109/ICACCI.2016.7732236
- Pandya, R. and Dibner, K.A., 2019. Learning through citizen science: enhancing opportunities by design. National Academies Press. doi:10.17226/25183
- Paul, J.D., et al., 2018. Citizen science for hydrological risk reduction and resilience building. Wiley Interdisciplinary Reviews: Water, 5 (1), e1262. doi:10.1002/wat2.1262
- Paul, J.D., Hannah, D.M., and Liu, W., 2019. Editorial: citizen science: reducing risk and building resilience to natural hazards. Frontiers in Earth Science, 7, 320. doi:10.3389/feart.2019.00320
- Pecora, S. and Lins, H.F., 2020. E-monitoring the nature of water. Hydrological Sciences Journal, 65 (5), 683–698. doi:10.1080/02626667.2020.1724296
- Poser, K. and Dransch, D., 2010. Volunteered geographic information for disaster management with application to rapid flood damage estimation. Geomatica, 64 (1), 89–98. doi:10.5623/geomat-2010-0008
- Preece, J., 2000. Online communities: designing usability and supporting sociability. New York, NY. John Wiley and Sons, Inc.
- Puri, S.K. and Sahay, S., 2003. Participation through communicative action: A case study of GIS for addressing land/water development in India. Information Technology for Development, 10 (3), 179–199. doi:10.1002/itdj.1590100305
- Quinn, P., 2018. Is the GDPR and its right to data portability a major enabler of citizen science? Global Jurist, 18 (2). doi:10.1515/GJ-2018-0021
- Radchuk, O., Kerbe, W., and Schmidt, M., 2017. Homo politicus meets homo ludens: public participation in serious life science games. Public Understanding of Science, 26 (5), 531–546. doi:10.1177/0963662516653030
- Resch, B., et al., 2015. Crowdsourcing physiological conditions and subjective emotions by coupling technical and human mobile sensors. GI_Forum, 1, 514–524. doi:10.1553/giscience2015s514
- Restrepo-Estrada, C., et al., 2018. Geo-social media as a proxy for hydrometeorological data for streamflow estimation and to improve flood monitoring. Computers & Geosciences, 111, 148–158. doi:10.1016/j.cageo.2017.10.010
- Rosa, A.L., Chiarelli, D.D., and Rulli, M.C., 2020. Global agricultural economic water scarcity. Science Advances, 6 (18), eaaz6031. doi:10.1126/sciadv.aaz6031
- Rutten, M., Minkman, E., and van der Sanden, M., 2017. How to get and keep citizens involved in mobile crowd sensing for water management? A review of key success factors and motivational aspects. Wiley Interdisciplinary Reviews: Water, 4 (4), e1218. doi:10.1002/wat2.1218
- Schwabish, J.A., 2020. Elevate the debate: a multilayered approach to communicating your research. Hoboken, NJ: John Wiley & Sons., Ed.
- See, L., et al., 2016. Crowdsourcing, citizen science or volunteered geographic information? The current state of crowdsourced geographic information. ISPRS International Journal of Geo-Information, 5 (5). doi:10.3390/ijgi5050055
- See, L., 2019. A review of citizen science and crowdsourcing in applications of pluvial flooding. Frontiers in Earth Science, 7, 44. doi:10.3389/feart.2019.00044
- Seibert, J., et al., 2019. Virtual staff gauges for crowd-based stream level observations. Frontiers in Earth Science, 7, 70. doi:10.3389/feart.2019.00070
- Seibert, J. and Vis, M.J.P., 2016. How informative are stream level observations in different geographic regions? Hydrological Processes, 30 (14), 2498–2508. doi:10.1002/hyp.10887
- Sermet, Y., et al., 2019. Crowdsourced approaches for stage measurements at ungauged locations using smartphones. Hydrological Sciences Journal, 65 (5), 813–822. doi:10.1080/02626667.2019.1659508
- Sheth, A., 2009. Citizen sensing, social signals, and enriching human experience. IEEE Internet Computing, 13 (4), 87–92. doi:10.1109/MIC.2009.77
- Shukla, A., et al., 2019. Evolution of hydroinformatics at a state water management agency. Hydrological Sciences Journal, 65 (5), 735–748. doi:10.1080/02626667.2019.1661418
- Silberbauer, M., 2019. Internet-based applications for interrogating 50 years of data from the South African national water quality monitoring network. Hydrological Sciences Journal, 65 (5), 726–734. doi:10.1080/02626667.2019.1645334
- Singh, V.P. and Fiorentino, M., 1996. Geographical information systems in hydrology. Hydrology and Water Resources of Africa. doi:10.1007/0-306-48065-4
- Sit, M.A., Koylu, C., and Demir, I., 2019. Identifying disaster-related tweets and their semantic, spatial and temporal context using deep learning, natural language processing and spatial analysis: a case study of Hurricane Irma. International Journal of Digital Earth, 12 (11), 1205–1229. doi:10.1080/17538947.2018.1563219
- Sivapalan, M., 2005. Pattern, process and function: elements of a unified theory of hydrology at the catchment scale. Encyclopedia of Hydrological Sciences. doi:10.1002/0470848944.hsa012
- Smith, A., et al., 2019. New estimates of flood exposure in developing countries using high-resolution population data. Nature Communication, 10 (1), 1–7. doi:10.1038/s41467-019-09282-y
- Smith, L., et al., 2017. Assessing the utility of social media as a data source for flood risk management using a real-time modelling framework. Journal of Flood Risk Management, 10 (3), 370–380. doi:10.1111/jfr3.12154
- Stahl, B.C., et al., 2017. The responsible research and innovation (RRI) maturity model: linking theory and practice. Sustainability, 9 (6), 1–19. doi:10.3390/su9061036
- Starkey, E., et al., 2017. Demonstrating the value of community-based (‘citizen science’) observations for catchment modelling and characterisation. Journal of Hydrology, 548, 801–817. doi:10.1016/J.JHYDROL.2017.03.019
- Stefanidis, A., Crooks, A., and Radzikowski, J., 2013. Harvesting ambient geospatial information from social media feeds. GeoJournal, 78 (2), 319–338. doi:10.1007/s10708-011-9438-2
- Stork, D.G., 2001. An architecture supporting the collection and monitoring of data openly contributed over the World Wide Web. Proceedings Tenth IEEE International Workshop on Enabling Technologies: Infrastructure for Collaborative Enterprises. WET ICE 2001, 380–385. doi:10.1109/ENABL.2001.953448
- Strickland, B.R., 2011. The Gale Encyclopedia of psychology. Simply Psychology, Second Edition. doi:10.4324/9780203720448
- Strobl, B., et al., 2019a. Citizen scientist training through an online game for data quality control. Geoscience Communication, 3 (1), 109–126. doi:10.5194/gc-3-109-2020
- Strobl, B., et al., 2019b. Accuracy of crowdsourced streamflow and stream level class estimates. Hydrological Sciences Journal, 65 (5), 823–841. doi:10.1080/02626667.2019.1578966
- Swain, N.R., et al., 2015. A review of open source software solutions for developing water resources web applications. Environmental Modelling and Software, 67, 108–117. doi:10.1016/J.ENVSOFT.2015.01.014
- Sy, B., et al., 2019. Flood hazard assessment and the role of citizen science. Journal of Flood Risk Management, 12 (S2), 1–14. doi:10.1111/jfr3.12519
- Tauro, F., et al., 2018. Measurements and observations in the XXI century (MOXXI): innovation and multi-disciplinarity to sense the hydrological cycle. Hydrological Sciences Journal, 63 (2), 169–196. doi:10.1080/02626667.2017.1420191
- Tkachenko, N., Jarvis, S., and Procter, R., 2017. Predicting floods with flickr tags. PLoS One, 12 (2), e0172870. doi:10.1371/journal.pone.0172870
- Tomsett, C. and Leyland, J., 2019. Remote sensing of river corridors: A review of current trends and future directions. River and Research Applications, 35 (7), 779–803. doi:10.1002/rra.3479
- Tress, G., Tress, B., and Fry, G., 2005. Clarifying integrative research concepts in landscape ecology. Landscape Ecology, 20 (4), 479–493. doi:10.1007/s10980-004-3290-4
- Trouille, L., Lintott, C.J., and Fortson, L.F., 2019. Citizen science frontiers: efficiency, engagement, and serendipitous discovery with human-machine systems. Proceedings of the National Academy of Sciences, 116 (6), 1902–1909. doi:10.1073/pnas.1807190116
- Tserstou, A., et al., 2017. SCENT: citizen sourced data in support of environmental monitoring. In: 2017 21st International Conference on Control Systems and Computer Science (CSCS), 612–616). doi:10.1109/CSCS.2017.93
- Uprety, M., et al., 2019. Improving water resources management using participatory monitoring in a remote mountainous region of Nepal. Journal of Hydrology: Regional Studies, 23, 100604. doi:10.1016/j.ejrh.2019.100604
- Vicari, R., et al., 2019. Climate risks, digital media, and big data: following communication trails to investigate urban communities’ resilience. Natural Hazards and Earth System Sciences, 19 (7), 1485–1498. doi:10.5194/nhess-19-1485-2019
- Vitolo, C., et al., 2015. Web technologies for environmental big data. Environmental Modelling and Software, 63, 185–198. doi:10.1016/j.envsoft.2014.10.007
- Von Korff, Y., et al., 2012. Implementing participatory water management: recent advances in theory, practice, and evaluation. Ecology and Society, 17 (1). doi:10.5751/ES-04733-170130
- von Krogh, G., et al., 2012. Carrots and rainbows: motivation and social practice in open source software development. MIS Quarterly, 36 (2), 649–676. doi:10.2307/41703471
- Wan, Z., et al., 2014. A cloud-based global flood disaster community cyber-infrastructure: development and demonstration. Environmental Modelling and Software. 58, 86–94. Elsevier. doi:10.1016/J.ENVSOFT.2014.04.007
- Wang, R.Q., et al., 2018. Hyper-resolution monitoring of urban flooding with social media and crowdsourcing data. Computers & Geosciences, 111, 139–147. doi:10.1016/j.cageo.2017.11.008
- Weber, K., Pallas, F., and Ulbricht, M.-R., 2019. Challenges of citizen science: commons, incentives, organizations, and regulations. The American Journal of Bioethics, 19 (8), 52–54. doi:10.1080/15265161.2019.1619862
- Wehn, U., et al., 2015. Citizen observatories as facilitators of change in water governance? Environmental Engineering and Management Journal, 14 (9), 2083–2086.
- White, T., et al., 2015. The role of critical zone observatories in critical zone science. Developments in Earth Surface Processes, 19, 15–78. doi:10.1016/B978-0-444-63369-9.00002-1
- Whiteaker, T.L., et al., 2006. Integrating arc hydro features with a schematic network. Transactions in GIS, 10 (2), 219–237. doi:10.1111/j.1467-9671.2006.00254.x
- Wiggins, A. and Crowston, K., 2011. From conservation to crowdsourcing: A typology of citizen science. In: 44th Hawaii international conference on system sciences, 1–10. doi:10.1109/HICSS.2011.207
- Witherow, M.A., et al., 2018. Analysis of crowdsourced images for flooding detection. European Congress on Computational Methods in Applied Sciences and Engineering, 27, 140–149. doi:10.1007/978-3-319-68195-5_15
- World Bank Group, 2018. Assessment of the state of hydrological services in developing countries. Washington, DC: World Bank, 1–55.
- Wu, P., Christidis, N., and Stott, P., 2013. Anthropogenic impact on Earth’s hydrological cycle. Nature Climate Change, 3 (9), 807–810. doi:10.1038/nclimate1932
- Xiao, Y., Huang, Q., and Wu, K., 2015. Understanding social media data for disaster management. Natural Hazards, 79 (3), 1663–1679. doi:10.1007/s11069-015-1918-0
- Yadav, M. and Rahman, Z., 2016. The social role of social media: the case of Chennai rains-2015. Social Network Analysis and Mining, 6 (1), 1–12. doi:10.1007/s13278-016-0410-5
- Yang, P. and Ng, T.L., 2017. Gauging through the crowd: a crowd-sourcing approach to urban rainfall measurement and storm water modeling implications. Water Resources Research, 53 (11), 9462–9478. doi:10.1002/2017WR020682
- Young, G., et al., 2015. Hydrological sciences and water security: an overview. IAHS-AISH Proceedings of the International Association of Hydrological Sciences, 366, 1–9. doi:10.5194/piahs-366-1-2015
- Yu, Y., et al., 2017. Urban infrastructure safety system based on mobile crowdsensing. International Journal of Disaster Risk Reduction, 27, 427–438. doi:10.1016/j.ijdrr.2017.11.004
- Zadick, J., Kenwright, B., and Mitchell, K., 2016. Integrating real-time fluid simulation with a voxel engine. The Computer Games Journal, 5 (1–2), 55–64. doi:10.1007/s40869-016-0020-5
- Zheng, F., et al., 2018. Crowdsourcing methods for data collection in geophysics: state of the art, issues, and future directions. Reviews of Geophysics, 56 (4), 698–740. doi:10.1029/2018RG000616