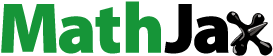
ABSTRACT
An agent-based model (ABM) is developed to explore the socio-hydrological and economic impacts of a water transfer project, as a supply enhancement strategy, on the cap-and-trade scheme, as a demand-side management practice, in Rafsanjan Plain, Iran. The modelling scheme benefits from a distributed groundwater model coupled with a socio-economic ABM to explore the social and economic impacts of the proposed supply enhancement and demand management strategies. The main goal of the integrated model is to investigate how the supply enhancement strategies and the cap-and-trade policy can bring about more favourable hydrological, social, and economic results in the recipient basin. Findings indicate that integration of the supply augmentation and the cap-and-trade policy can facilitate the emergence of collective action in the farmer society. It is shown that the combination of these two policies, when implemented to mitigate water scarcity, reduces economic costs incurred on both farmers and the government and brings about more favourable socio-hydrological outcomes.
Editor S. Archfield Associate editor S. Pande
1. Introduction
Arid and semi-arid regions face significant challenges of water supply insecurity due to population growth, socio-economic developments, and climate change (Wheeler et al. Citation2014, Gu et al. Citation2017, Alamdarlo et al. Citation2019). Globally, more than 90% of consumptive water use relates to agriculture, which raises major concerns about unsustainable use and over-consumption of limited freshwater resources, particularly in arid regions (Nouri et al. Citation2019). Therefore, designing and implementing effective strategies for sustainable management and reallocation of water resources between different users deserves serious concern (Chen et al. Citation2019).
Demand management and supply enhancement strategies are often used for reallocating water when water demand exceeds water supply, aiming to mitigate water scarcity. While the first type of strategy attempts to come up with measures for operating within the limits of current water supplies, the second aims to harness and make use of new water resources. Demand management strategies incorporate educational measures and economic incentives such as water pricing and trade of water rights. On the other hand, supply enhancement strategies aim to increase water supplies through desalination, construction of new dams, inter-basin water transfer projects, etc. These two management practices can be undertaken jointly, which is normally an ideal solution for improving water security (Wheeler et al. Citation2017, Loch et al. Citation2020).
Market-based allocation of freshwater resources is a demand management practice that is considered an effective tool for addressing water scarcity (Gill et al. Citation2017, Seidl et al. Citation2020). A cap-and-trade policy is a market-based water allocation strategy that facilitates sustainable use of water resources and engenders water security. This policy limits water extraction to facilitate sustainability and provides users with the opportunity to trade their water permits to improve the economic situation of the region (Connor et al. Citation2016, Aghaie et al. Citation2020a).
Many studies have analysed the environmental and economic impacts of water markets. In particular, Du et al. (Citation2017) developed an agent-based model (ABM) to analyse a hypothetical water market, looking at the ways that farmers’ behaviours affect productivity and market performance. In a highly relevant and recent study, Aghaie et al. (Citation2020a) evaluated the hydro-economic outcomes of different market mechanisms built on the basis of the cap-and-trade policy. They concluded that discriminatory price double-auctions (markets where every transaction takes place at a different price) surpasses uniform price double-auctions and water markets with equilibrium price discovery in terms of hydrological and economic performance.
Inter-basin water transfer projects are a type of supply enhancement strategy aiming to increase water security by transferring water from one basin with abundant resources to another basin (Sinha et al. Citation2020). This strategy may involve the construction of long tunnels, reservoir operation, mass water pumping, and water diversions. Numerous studies have assessed the socio-economic and hydrological impacts of inter-basin water transfer projects (Feng et al. Citation2007, Citation2018, Rey et al. Citation2016, Peng et al. Citation2017, Zhou et al. Citation2017, Nasiri-Gheidari et al. Citation2018, Sinha et al. Citation2020). Feng et al. (Citation2007), in particular, developed a decision support system (DSS) based on a computable general equilibrium (CGE) model to analyse the socio-economic effects of the great south–north water transfer project in China. They analysed the environmental impacts of the water transfer project under different scenarios by incorporating numerical models into the proposed DSS. They concluded that the incremental water supply from the water transfer project facilitates the development of the recipient region.
Rey et al. (Citation2016) proposed a new method to assess various sectoral water allocation strategies to mitigate water shortage problems in an area of northeastern Spain. Finally, Nasiri-Gheidari et al. (Citation2018) developed a multi-objective optimization model for minimizing environmental pollution and maximizing the economic benefits of a water transfer project under uncertainty. Their results show that higher levels of uncertainty result in greater reduction in water allocation to the receiving basin.
Numerous top-down simulation-optimization or bottom-up approaches can be used to understand possible impacts of policy implementation. While there are many top-down studies on market-based allocation of water (Manjunatha et al. Citation2011, Erfani et al. Citation2014, Li et al. Citation2019), and inter-basin water transfer projects (Karamouz et al. Citation2009, Gurung and Bharati Citation2012, Maknoon et al. Citation2012, Rani et al. Citation2016, Nasiri-Gheidari et al. Citation2018), it is claimed that bottom-up methods, such as agent-based modelling, are capable of addressing the dynamic complexity of water markets, water transfer projects, and micro-level interactions (Du et al. Citation2017, Zhang et al. Citation2018). Agent-based modelling is a bottom-up approach belonging to generative science (Epstein Citation2006), which is capable of exploring macro-level patterns and structures from micro-level interactions (Tong et al. Citation2018, Pouladi et al. Citation2019, Michaelis et al. Citation2020).
Such bottom-up approaches can help policymakers to assess whether a policy is environmentally sustainable, economically feasible, and socially acceptable (Castilla-Rho et al. Citation2017, Pouladi et al. Citation2020). Previous works have asserted that policies that limit farmers’ groundwater withdrawal, such as cap-and-trade policies, can cause farmers’ non-compliance behaviour, depending heavily on institutional and cultural factors (Castilla-Rho et al. Citation2017, Aghaie et al. Citation2020b). These works assert that compliance behaviours of water users depend substantially on monitoring level and enforcement power when restrictive water management policies are adopted. However, an open question remains as to how such policies should be adopted to be socially acceptable in developing countries, given that monitoring millions of water users is not feasible considering the high costs.
In this paper, agent-based modelling is used to provide insights into this question and to explore the socio-hydrological and economic impacts of a supply enhancement strategy (a water transfer project) on a demand-side management practice (the cap-and-trade scheme) in an arid region in Iran, which currently lacks effective monitoring capabilities. Particularly, we investigate how farmers’ social behaviours evolve through time in response to the proposed policies. The cap-and-trade policy and the water transfer project are modelled independently in an arid region, and the conditions on which the implementation of these two strategies may result in the emergence of collective action are explored. To this end, two different expressions are proposed accounting for human behaviour towards water conservation, the joint impacts of which form social norms. This is in line with the key aspect of socio-hydrology, the science of people and water, which is the explicit incorporation of two-way feedbacks and interactions between human and water systems (Sivapalan et al. Citation2014, Jeong et al. Citation2020, Ponnambalam and Mousavi Citation2020). The integrated model benefits from a distributed groundwater model for simulating the hydrological processes in the aquifer and estimation of the fluctuations of the groundwater level.
Furthermore, a socio-economic ABM is linked to the groundwater model to explore the social and economic impacts of the proposed supply enhancement and demand management strategies. The main goal of the integrated model is to provide insights into the ways that supply enhancement strategies and the cap-and-trade policy can bring about more favourable hydrological, social, and economic results in the recipient basin.
2. Case study
Rafsanjan Plain is located in the central part of Iran and includes more than 60 000 hectares of pistachio orchards. This plain is located in Kerman Province and its main aquifer is Rafsanjan Aquifer, which incorporates more than 1400 agricultural, industrial, and domestic wells, supplying the majority of the region’s water demand. Due to water scarcity and economic losses, some policymakers proposed a to transfer water from a basin located in the western part of Iran (). Another solution for facilitating sustainable use of groundwater resources in the region is a cap-and-trade policy, which has recently received considerable attention. Therefore, there is a need to investigate the socio-hydrological and economic impacts of these supply-side and demand-side management practices before any decision is made, which is the aim of this study.
3. Methodology
The developed ABM aims to assess supply augmentation and demand-side policies to find a way to ameliorate the hydrologically unfavourable condition of the aquifer without creating negative social impacts in the recipient basin. The model is intended to assess the socio-hydrological condition of the region to help transition towards groundwater sustainability. It includes a groundwater flow simulator named FlowLogo, which is a distributed groundwater model, and evaluates the social and economic impacts of the policies by a coupled socio-economic ABM.
As illustrated in , the integrated modelling approach assesses social impacts of and evaluates hydrological conditions affected by the cap-and-trade policy and the water transfer project supplying water to the households in the farmer society of the region. The environment of the developed ABM includes a hydrological environment (i.e. the aquifer) and an agricultural environment (i.e. farms), and it has one agent type (i.e. farmer agents) and one proto-agent type (i.e. households). Proto-agents are agents that do not have individual adaptation processes, properties, and states (Wilensky and Rand Citation2015). Because this study aims to investigate how farmers’ behaviours evolve through time due to policy implementation, farmers are agents and have states and behaviours. However, there is no need to make households full agents, as they will simply change their pumping rates due to the water transfer project’s conveyance capacity and make no other changes to the environment. Therefore, the transferred water is supplied to the household proto-agents, and the cap-and-trade policy is implemented in the farmer society. Depending on the volume of water transferred between basins, the household proto-type agents adjust their pumping from groundwater and contribute to the groundwater hydrology through their return flow to the aquifer. The socio-economic ABM and the groundwater simulation model interact with each other and evolve through time; the socio-economic ABM sends farmers’ and households’ pumping rates to the groundwater model (i.e. FlowLogo), and the FlowLogo model sends water tables to the socio-economic ABM.
3.1. Groundwater flow simulator
To simulate the fluctuations of groundwater tables of Rafsanjan Aquifer due to agricultural and domestic groundwater pumping, we used a distributed groundwater flow simulator named FlowLogo, which is established in the NetLogo programmable modelling environment (Castilla-Rho et al. Citation2015) and which was calibrated and validated in our previous study (Aghaie et al. Citation2020a). The FlowLogo modelling platform enables researchers to integrate ABMs with a distributed groundwater model. The FlowLogo groundwater simulator incorporates various types of boundary conditions and a variety of sinks and sources, which are the building blocks of a groundwater simulator.
3.2. Cap-and-trade policy in the agricultural sector
The cap-and-trade policy is an effective tool that facilitates the sustainable use of limited resources by putting a cap on resource extractions and providing the opportunity to trade resource permits. As shown in , the proposed policy in the agricultural sector works as follows. The traditional water extractions of farmers are capped at a sustainable level defined based on regional groundwater levels, and a farmer’s total allowable water extraction is his/her water permit. As a result of the permit caps, farmers may desire to extract more water than their water cap permits. Furthermore, they may be willing to participate in an existing (or proposed) water market to trade their water permits. Also, farmer agents can report their neighbours who violate the permit cap to a central market institution, depending on their understanding of pumping cost externalities.
In each market round, which is on a yearly basis, farmers may send their offer price, offer amount (volume of water), and violation reports to the central institution, which is responsible for transferring water permits from sellers to buyers based on a discriminatory-price double auction (Du et al. Citation2017) and issuing fines attempting to curb violations. After matching the bids and transferring water permits from successful sellers to successful buyers, the central institution releases trade and fine results, based on which farmers update their pumping rates, social behaviours, rent-seeking and irrigation strategies. Finally, FlowLogo is run based on updated pumping rates, after which the central institution caps water permits if needed to facilitate sustainability.
Farmers’ water permits are capped, every year, based on groundwater level and/or drawdowns to ensure sustainability, as follows:
where (m3/year) is the ith farmer water permit in time step t,
(m3/year) is the ith farmer water permit before implementing the cap-and-trade policy (without any caps), and
is the permit cap coefficient, defined based on Equation (2):
where (m) is the maximum allowable water table,
(m) is the water table of the region in which the farm of the ith farmer agent is located, and
(m) is the sustainable water table that the cap-and-trade policy aims to reach; 42 polygonal regions are defined in the aquifer corresponding with 42 piezometers, and
is the water table of the piezometer corresponding to ith farmer agent region.
Accordingly, farmer agents estimate their water demand/supply ) from/to the water market. Farmers’ water demand can be satisfied by participating in the market and/or by violation. Similarly, farmers’ water supply to the market can come from their water permit and/or their violation. Farmers’ water demand/supply is the difference between their water permit
and their actual irrigation requirements
:
An agent’s actual irrigation requirement () for a selected irrigation strategy and cropping pattern is a function of their stress-avoidance irrigation requirement
and the agent’s irrigation strategy parameter (
(Aghaie et al. Citation2020a):
The agent’s irrigation strategy parameter ( is a behavioural measure that accounts for heterogeneous behaviours of farmers for crop irrigation. The bounding value of
0 refers to rain-fed farming, and a decision of
1 denotes irrigation with no water stress. Farmer agents adapt their irrigation behavioural parameters using an algorithm to achieve more profit through time (Aghaie et al. Citation2020a).
Finally, farmer agents estimate their bid amount (volume of water to be traded) in each year as follows, based on the difference between their water demand/supply () and their violation amount (
: water they are going to withdraw illegally):
where (m3/year) is the ith farmer violation amount from the agent’s valid permit, estimated based on EquationEquation (6)
(6)
(6) (Aghaie et al. Citation2020b):
where and
(m3/year) are the ith farmer water permit before implementing the cap-and-trade policy and in time step t, and
and
are two dimensionless variables showing the severity of fines for violators and the percentage of wells that are monitored. Finally,
stands for the ith agent’s boldness, which is a social behavioural parameter representing the agent’s tendency towards violation and pumping water illegally (estimated by EquationEquation (12)
(12)
(12) ). In fact, the ith farmer agent pumps water illegally with a likelihood equal to
and to a maximum level of
, attempting to proceed towards its water permit before implementing the cap-and-trade policy
.
As mentioned, farmer agents willing to participate in the cap-and-trade market send two independent variables to the market institution: bid amount (estimated using EquationEquation (5)(5)
(5) ) and offer price (estimated using EquationEquation (7)
(7)
(7) ). Two main factors affecting farmer agents’ bidding prices are (a) farmers’ rent-seeking (
), which represents their greediness, and (b) their reservation price,
(IRR/m3), which is the maximum price a buyer would be willing to pay or the minimum price a seller would require for its water supply (Du et al. Citation2017). Accordingly, farmers’ bid prices (IRR/m3) are calculated as follows:
The farmer agents adapt their rent-seeking behaviour based on their success in trade (Cliff and Bruten Citation1997):
where is a reference price that farmers attempt to reach. A farmer agent with a successful trade would use the last transaction price as reference trading price. Otherwise, with no successful trade, the farmer uses the average market prices as the reference price. Furthermore,
is a behavioural parameter that accounts for farmers’ learning rate distributed between agents by a uniform distribution (Aghaie et al. Citation2020a).
A market participant’s surplus, which is a measure of his/her welfare, can be calculated based on the difference between his/her traded price and the participant’s reservation price
. The sum of buyers’ surplus is the total consumer surplus (
), and the sum of sellers’ surplus is the total producer surplus
, the sum of which is social welfare in the farmer society (Aghaie et al. Citation2020a):
where and
(m3/year) are agent i’s sold and bought permits, and
and
(IRR/m3) are the agent’s reservation price and traded price, respectively.
3.2.1. Cap-and-trade and social norms
The cap-and-trade policy restricts farmers’ water pumping, which can cause the emergence of compliance or non-compliance behaviours representing farmers’ attitudes towards the policy (Aghaie et al. Citation2020b). In the case of compliance behaviour, farmers tend to play a positive role in the water conservation policy by reporting violators and obeying the regulations, and when it comes to non-compliance behaviour, they show a propensity to violate their water permit and exhibit a disregard for violations by other farmers. The probability that a farmer agent takes water illegally is addressed by its boldness (), and the punitiveness (
) defines the probability of reporting a breaching neighbour agent. The joint impacts of boldness and punitiveness form social norms
in a farmer society with N individuals (Castilla-Rho et al. Citation2017):
When the majority of the agents comply with permit caps (i.e. ,
and
), a norm of compliance emerges throughout the farmer society. Conversely, if the emerged norm moves towards −1, non-compliance behaviour dominates in the farmer society. Boldness of social behaviour is estimated using a mathematical equation that is dependent on institutional frameworks and permit caps (Aghaie et al. Citation2020b):
where and
(m3/year) are the agent’s water permits before and after permit caps at time step t,
is a variable showing the magnitude of fines incurred by violators, and
is the percentage of wells that are equipped with meters and are monitored at time step t. It is worth noting that the region’s economic situation does not allow for strict monitoring by equipping wells with meters, so it is assumed that authorities allocated a budget to equip 10% of wells with meters on a yearly basis, and that every 2 years 6% of the total meters cease working.
Similarly, the punitiveness social behaviour for each farmer agent is estimated using the following equation (Aghaie et al. Citation2020b):
where is the ith farmer’s punitiveness regarding its jth neighbour at time step t (i.e. the likelihood of reporting the neighbour j as a violator),
(kg/ha) is the average crop yield of all of the agent’s neighbours, and
(kg/ha) is the crop yield of neighbour j. Consequently, the punitiveness for each agent,
, that is used in EquationEquation (11)
(11)
(11) , is assumed to be equal to the maximum of the agent’s punitiveness towards its neighbours. As can be seen in EquationEquations (11
(11)
(11) –Equation13
(13)
(13) ), these formulas are in line with Ostrom’s core ideas relevant to the argument that tragedies of the commons can be avoided through locally emerging institutions, leading to the sustainable use of a shared resource in the long run (Ostrom Citation1990). That is, in the formulation of punitiveness, farmers’ reactions to the pumping cost externalities are taken into account; they report their neighbours who had a higher crop yield than their locally averaged crop yield, meaning that farmers react to their neighbours’ over-pumping. Thus, when punitiveness increases, social norm-following increases, leading to a decline in boldness – meaning that people are locally cooperating towards the sustainable use of water resources, forming collective action.
It is worth noting that due to the lack of reliable data, all of the behavioural parameters (rent-seeking, learning rate, irrigation strategy parameter, boldness, and punitiveness) are initially distributed between farmer agents according to a uniform distribution.
3.3. Water transfer project for the drinking sector
The water transfer project through the conveyance line shown in is considered the main strategy for long-term drinking water supply to Rafsanjan Plain, Iran. This project consists of a 650-km-long water transfer line. The cost of the line highly depends on the system’s capacity. The primary objective of this project is to increase water supply security by supplying water to the drinking water sector (i.e. households) in Rafsanjan Plain, with an annual demand of 160 × 106 m3 (million cubic metres, MCM). Notwithstanding its high costs, which will require substantial financial resources to meet, there are major concerns about its socio-hydrological impacts.
We attempt to estimate the costs of this project and use transaction costs earned in the agricultural market, i.e. the cap-and-trade policy in the agricultural sector, as a financial resource for the water transfer project. It is worth mentioning that transaction costs are expenses incurred by farmers when participating in the market as sellers or buyers. In addition, the socio-hydrological impacts of the water transfer project on the efficiency of the cap-and-trade policy in the receiving basin are evaluated. To this end, four main scenarios are designed: (1) the status quo scenario that evaluates the current hydrological and economic conditions of the region; (2) the WT scenario, where WT stands for the water transfer project for the drinking sector; (3) the C&T scenario that represents the implementation of the cap-and-trade policy in the agricultural sector; and (4) the WT+C&T scenario, which is a combination of scenarios 2 and 3, i.e. the cap-and-trade policy in the farmer society and the water transfer project for improving drinking water supply security. Furthermore, a sensitivity analysis is performed to explore the socio-hydrological impacts of different conveyance capacities.
3.4. Calibration and validation
There is currently no cap-and-trade water market or water transfer project in the case study area of Rafsanjan Plain, and these two strategies have been proposed to the government as a means to mitigate water scarcity. Therefore, we calibrated and validated the groundwater flow simulator under the present conditions without any of the aforementioned strategies being in play (Aghaie et al. Citation2020a). Regarding the calibration of the socio-economic ABM, no calibration is performed as the supply enhancement and demand management strategies proposed in this study have not been yet implemented, so no empirical data exist. On the other hand, the validation of the socio-economic ABM can be explained as follows.
In general, ABMs face difficulties with validation due to insufficient empirical data. To address this issue, there are two types of model validation: (a) outcome validation, which assesses the fitness of the modelling results and empirical data; and (b) structural validation, which examines whether the model structure agrees with expert views and theories (Manson Citation2003). Owing to the lack of observed data and because the aim of the study is to understand human behaviours when implementing policies, we focus on structural validation, ensuring that the behavioural rules defined for farmer agents are based on validated theories of farmers’ decisions and behaviours, such as social behaviours (Castilla-Rho et al. Citation2017, Aghaie et al. Citation2020b), bidding behaviour determining farmers’ bid amounts and price (Du et al. Citation2017, Gonzales and Ajami Citation2019), and irrigation behaviour (Aghaie et al. Citation2020a). Furthermore, the model has been run with extremely high and low values of several parameters (such as monitoring percentage, fine magnitude, and households’ demands) to ensure that reasonable solutions and changes occur. In the future, if the cap-and-trade market is implemented, or if the water transfer project is completed, the outcome validation can be performed when observed data become available.
4. Results and discussion
As described in Section 3.3, four main scenarios were designed to assess the cap-and-trade policy in the agricultural sector and the water transfer project for the drinking sector: the status quo scenario, the WT scenario, the C&T scenario and the C&T+ WT scenario.
shows the economic, social, and hydrological outcomes of the water transfer project (WT), which satisfies households’ water demand completely, and the cap-and-trade policy (C&T). Although the cap-and-trade policy and the water transfer project are not directly related, this figure generally suggests that the water transfer project reduces the households’ pumping rates, affecting the cap-and-trade policy development. When the households reduce their pumping rates, the groundwater is restored, the permit cap is refined, violations are reduced, and a stronger social norm emerges. This interaction continues until the most desirable results are achieved. This result can provide insights into how the cap-and-trade scheme can be adopted in developing countries to be socially acceptable given that monitoring millions of water users is impractical and costly.
Figure 4. Outcomes of the water transfer (WT) project and the cap-and-trade policy (C&T): time series of (a) unit hydrograph of Rafsanjan Aquifer, (b) changes in farmers’ profit (net benefit), (c) social norm, (d) water price, (e) cumulative farmers’ surplus, and (f) volume of traded water. The intervals show 95% confidence bands
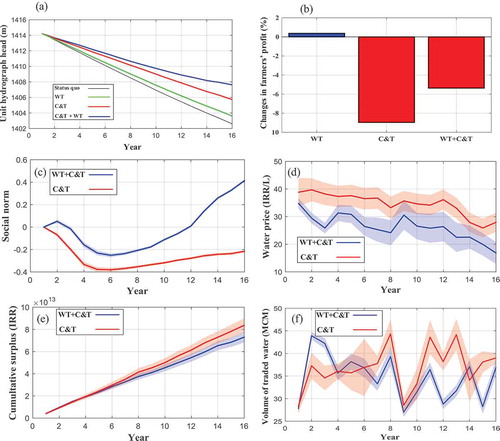
It can be seen in ) that the status quo of the aquifer is hydrologically undesirable, since the groundwater level drops 80 cm/year. As the main consumer of the groundwater resource is agriculture in the study region, the C&T policy seems an essential strategy for reducing the groundwater drawdown from 80 to 62 cm/year. Besides, notwithstanding bringing about drinking water security, the water transfer project alone could not substantially restore the aquifer because the drinking sector places only minor stress on the aquifer compared to agriculture. Finally, the combination of the demand-side policy and the supply augmentation strategy leads to a more favourable hydrological condition, where the yearly drawdown is reduced to 37 cm/year.
In the case of the water transfer project, no significant change occurs in the economic situation of the farmers ()). This is because the contribution of the WT project to reducing the groundwater level drawdown is quite small ()); hence the farmers’ savings in pumping costs resulting from the WT project may not significantly improve their economic status. On the other hand, the C&T scenario has resulted in a significant reduction (by 9%) in farmers’ profits due to permit caps. This reduction in farmers’ net benefit can be compensated for to some extent when the C&T scenario is combined with the WT scenario, due to a decrease in farmers’ pumping costs and water level drawdown, resulting in less severe permit caps ()). From a socio-hydrological perspective, an interesting insight here is that ) shows the attractiveness of multiple coordinated interventions. That is, combining two interventions (WT+C&T) provides more favourable impacts than simply the sum of the individual interventions (i.e. the outcome of WT added to the outcome of C&T in )).
) shows the time series of social norms emerging due to policy implementation. It is obvious that in the C&T scenario, due to the weak institutional framework and monitoring percentage, a norm of non-compliance has emerged. As mentioned in section 3.2.1, the region’s economic situation does not allow further monitoring by equipping additional wells with meters, so it is assumed that the authorities allocated a budget to equip 10% of wells with meters on a yearly basis, and that every 2 years 6% of the total meters cease working. The number of wells equipped with meters are fewer at the beginning of the simulation period, so social norms drop until time step 5. However, after time step 5, the monitoring reaches a level (49.5% of wells) that leads to an increasing trend in social norms ()). This fact has resulted in an increasing social norm through time, after time step 5, in both the C&T and WT+C&T scenarios. It can be seen that while the C&T scenario has resulted in the formation of a weak social norm, adding the water transfer project facilitates compliance and collective action. This is because the aquifer is substantially restored in the WT+C&T scenario, so the severity of permit caps is reduced to the extent that they are dependent on regional drawdowns. As a result of the reduction in permit cap severity from the water transfer project, the tendency in the farmer society to take water illegally is reduced. Therefore, it can be deduced that every supply enhancement strategy, such as the water transfer project, can facilitate the emergence of collective action and farmers’ compliance when the cap-and-trade policy is implemented.
When supply increases in a market, the unit price of water decreases. ) concurs with this fact as it shows that the WT+C&T scenario had a market price approximately 24% lower than that of the WT scenario, due to less groundwater level drawdown and smaller caps on permits (i.e. an increase in supply). ) shows that farmers’ surplus participation in the water market is smaller in the WT+C&T scenario, as it had lower prices and volume of traded water in comparison with the WT scenario.
Finally, ) shows the volume of water traded between farmer agents. Notwithstanding the first 3 years, it can be seen that in the face of water scarcity, the traded volume of water is higher (C&T scenario), but there is less participation in the market when water level drawdown is reduced (WT+C&T scenario). It is worth mentioning that the WT+C&T scenario has a decreasing trend of traded volume of water because the aquifer is restored through time ()), which mitigates water scarcity. This is in line with the results of previous research stating that agricultural water markets perform more successfully in terms of volume of traded water when encountering water shortage problems, which facilitates transferring water from low-value uses to high-value uses in agricultural sectors (Du et al. Citation2017). Regarding time steps 1–3, however, the market has a higher volume of traded water in the WT+C&T scenario compared to the C&T scenario. The reason for this may lie in the fact that the aquifer is significantly depleted in the first few time steps ()) – a reduction in supply – so farmers’ demand is at a high level without a water transfer project (i.e. the C&T scenario). However, when WT comes into play, an abrupt increase occurs in the traded amounts ()), due to an increase in supply, despite its declining trend owing to aquifer restoration in time (decreasing demand).
All in all, the results show that the integration of a supply enhancement strategy with the cap-and-trade scheme would bring about more favourable socio-hydrological and economic outcomes in agricultural sectors. Furthermore, the cap-and-trade scheme can be socially acceptable in developing countries, where insufficient monitoring capabilities exist, when combined with a supply augmentation strategy, notwithstanding the economic costs incurred by governments or authorities. Furthermore, the cap-and-trade scheme complements supply enhancement strategies, as the transaction costs incurred by farmers compensate for the economic costs of the water transfer project by 4% in the first 16 years of C&T implementation. Finally, the project indirectly reduces permit caps due to a reduction in drawdowns, thus lessening the severity of the reduction in farmers’ net benefits due to the permit caps. Therefore, these two strategies in combination can compensate for each other’s drawbacks to some extent.
The economic cost of the water transfer project is heavily dependent on its conveyance capacity. Thus, as mentioned in section 3.3, it is essential to perform a sensitivity analysis to assess the socio-hydrological effects of this strategy for different conveyance capacities. shows the results of a sensitivity analysis that was performed for different conveyance capacities supplying water to the households. It can be deduced that if the capacity is smaller than 40% of the households’ water demands, the social norm that emerges in the farmer society would be one of non-compliance, due to severe groundwater withdrawal and its related permit caps. However, if the conveyance capacity is more than 40% of the households’ demand, a norm of compliance would emerge through time in the farmer society. Thus, not only can a conveyance capacity approximately equal to 50% of the households’ demand bring about favourable socio-hydrological results, it can also reduce the economic costs of the water transfer project to half of the economic cost of a conveyance line satisfying the demand of all domestic users. ) shows the time series of the unit hydrograph head of Rafsanjan Aquifer for different conveyance capacities for the water transfer project. It is obvious that the more the water transfer project satisfies the demand of households, the smaller is the groundwater drawdown through time. Hence, if the water transfer project is combined with the cap-and-trade policy, and the convenience capacity is designed equal to 50% of the households’ demands, the economic costs of the project would be reduced (through lower costs of the conveyance line and the revenue obtained from transaction costs incurred by farmers), and the socio-hydrological impacts of the policy implementation would be more favourable.
5. Conclusion
Arid and semi-arid regions face significant challenges of water supply insecurity in the face of climate change and population growth, which highlights the need to adopt effective policies to improve water security. Thus, this paper studies the socio-hydrological and economic effects of a demand-side practice (i.e. the cap-and-trade policy) and a supply enhancement strategy (i.e. the water transfer project).
The results of the study indicate that either of the C&T or WT scenarios is solely capable of restoring the hydrological condition of Rafsanjan Aquifer to some extent. However, the combination of these supply enhancement and demand management strategies substantially reduces groundwater drawdowns from 80 to 32 cm/year. From a social perspective, a norm of non-compliance emerged in the farmer society in the C&T scenario due to the weak institutional framework of the region. However, adding the water transfer project to the cap-and-trade policy led to collective action and compliance behaviour in the farmer society. Furthermore, the project to transfer water to households reduces groundwater drawdowns, leading to less severe permit caps, which lessens the severity of reduction in farmers’ profits due to permit caps, and the revenue earned from transaction costs imposed on market participants thus compensates for 4% of the water transfer project in the short run. This study shows, in particular, that the combination of supply enhancement strategies and the cap-and-trade policy can be a socially, hydrologically, and economically ideal solution for improving water supply security in the recipient basin.
It is worth mentioning that the socio-hydrological and economic impacts of the water transfer project are evaluated in the water-receiving basin. Future studies should focus on the environmental, social, and economic impacts of this policy in the water-sending basin.
Disclosure statement
No potential conflict of interest was reported by the authors.
References
- Aghaie, V., Alizadeh, H., and Afshar, A., 2020a. Agent-based hydro-economic modelling for analysis of groundwater-based irrigation water market mechanisms. Agricultural Water Management, 234, 106–140. doi:10.1016/j.agwat.2020.106140
- Aghaie, V., Alizadeh, H., and Afshar, A., 2020b. Emergence of social norms in the cap-and-trade policy: an agent-based groundwater market. Journal of Hydrology, 588, 125057. doi:10.1016/j.jhydrol.2020.125057
- Alamdarlo, H.N., Pourmozafar, H., and Vakilpoor, M.H., 2019. Improving demand technology and internalizing external effects in groundwater market framework, case study: Qazvin plain in Iran. Agricultural Water Management, 213, 164–173. doi:10.1016/j.agwat.2018.10.005
- Castilla-Rho, J.C., et al., 2015. An agent-based platform for simulating complex human–aquifer interactions in managed groundwater systems. Environmental Modelling & Software, 73, 305–323. doi:10.1016/j.envsoft.2015.08.018
- Castilla-Rho, J.C., et al., 2017. Social tipping points in global groundwater management. Nature Human Behaviour, 1 (9), 640. doi:10.1038/s41562-017-0181-7
- Chen, S., et al., 2019. A conceptual agricultural water productivity model considering under field capacity soil water redistribution applicable for arid and semi-arid areas with deep groundwater. Agricultural Water Management, 213, 309–323. doi:10.1016/j.agwat.2018.10.024
- Cliff, D. and Bruten, J. (1997) Zero not enough: on the lower limit of agent intelligence for continuous double auction markets. Bristol, England: HP Laboratories.
- Connor, J.D., Bryan, B.A., and Nolan, M., 2016. Cap and trade policy for managing water competition from potential future carbon plantations. Environmental Science & Policy, 66, 11–22. doi:10.1016/j.envsci.2016.07.005
- Du, E., et al., 2017. Evaluating the impacts of farmers’ behaviors on a hypothetical agricultural water market based on double auction. Water Resources Research, 53, 4053–4072. doi:10.1002/2016WR020287
- Epstein, J.M., 2006. Generative social science: studies in agent-based computational modeling. Princeton, NJ: Princeton University Press.
- Erfani, T., Binions, O., and Harou, J.J., 2014. Simulating water markets with transaction costs. Water Resources Research, 50, 4726–4745. doi:10.1002/2013WR014493
- Feng, S., et al., 2007. Assessing the impacts of South-to-North water transfer project with decision support systems. Decision Support Systems, 42, 1989–2003. doi:10.1016/j.dss.2004.11.004
- Feng, T., et al., 2018. Effect of inter-basin water transfer on water quality in an urban lake: a combined water quality index algorithm and biophysical modelling approach. Ecological Indicators, 92, 61–71. doi:10.1016/j.ecolind.2017.06.044
- Gill, B., et al., 2017. Economic, social and resource management factors influencing groundwater trade: evidence from Victoria, Australia. Journal of Hydrology, 550, 253–267. doi:10.1016/j.jhydrol.2017.04.055
- Gonzales, P. and Ajami, N., 2019. Goal-based water trading expands and diversifies supplies for enhanced resilience. Nature Sustainability, 2, 138–147. doi:10.1038/s41893-019-0228-z
- Gu, C., et al., 2017. Effects of climate change and human activities on runoff and sediment inputs of the largest freshwater lake in China, Poyang Lake. Hydrological Sciences Journal, 62, 2313–2330. doi:10.1080/02626667.2017.1372856
- Gurung, P. and Bharati, L., 2012. Downstream impacts of the Melamchi Inter-Basin Water Transfer Plan (MIWTP) under current and future climate change projections. Hydro Nepal: Journal of Water, Energy and Environment, 23–29. doi:10.3126/hn.v11i1.7199
- Jeong, H., et al., 2020. Insights from socio-hydrological modeling to design sustainable wastewater reuse strategies for agriculture at the watershed scale. Agricultural Water Management, 231, 105983. doi:10.1016/j.agwat.2019.105983
- Karamouz, M., Mojahedi, S.A., and Ahmadi, A., 2009. Interbasin water transfer: economic water quality-based model. Journal of Irrigation and Drainage Engineering, 136, 90–98. doi:10.1061/(ASCE)IR.1943-4774.0000140
- Li, K., Huang, G., and Wang, S., 2019. Market-based stochastic optimization of water resources systems for improving drought resilience and economic efficiency in arid regions. Journal of Cleaner Production, 233, 522–537. doi:10.1016/j.jclepro.2019.05.379
- Loch, A., Adamson, D., and Dumbrell, N., 2020. The fifth stage in water management: policy lessons for water governance. Water Resources Research, 56 (5). doi:10.1029/2019WR026714
- Maknoon, R., Kazem, M., and Hasanzadeh, M., 2012. Inter-basin water transfer projects and climate change: the role of allocation protocols in economic efficiency of the project. Case study: Dez to Qomrood Inter-Basin Water Transmission Project (Iran). Wuhan, China: Scientific Research.
- Manjunatha, A., et al., 2011. Impact of groundwater markets in India on water use efficiency: a data envelopment analysis approach. Journal of Environmental Management, 92, 2924–2929. doi:10.1016/j.jenvman.2011.07.001
- Manson, S., 2003. Validation and verification of multi-agent models for ecosystem management. In: M.A. Janssen, ed. Complexity and ecosystem management: the theory and practice of multi-agent approaches. Cheltenham, UK: Edward Elgar, 63–74.
- Michaelis, T., Brandimarte, L., and Mazzoleni, M., 2020. Capturing flood-risk dynamics with a coupled agent-based and hydraulic modelling framework. Hydrological Sciences Journal, 65 (9), 1458–1473. doi:10.1080/02626667.2020.1750617
- Nasiri-Gheidari, O., Marofi, S., and Adabi, F., 2018. A robust multi-objective bargaining methodology for inter-basin water resource allocation: a case study. Environmental Science and Pollution Research, 25, 2726–2737. doi:10.1007/s11356-017-0527-8
- Nouri, H., et al., 2019. Water scarcity alleviation through water footprint reduction in agriculture: the effect of soil mulching and drip irrigation. Science of the Total Environment, 653, 241–252. doi:10.1016/j.scitotenv.2018.10.311
- Ostrom, E., 1990. Governing the commons: the evolution of institutions for collective action. Cambridge, UK: Cambridge University Press.
- Peng, Y., et al., 2017. Multi-core parallel particle swarm optimization for the operation of inter-basin water transfer-supply systems. Water Resources Management, 31, 27–41. doi:10.1007/s11269-016-1506-4
- Ponnambalam, K. and Mousavi, S.J., 2020. CHNS modeling for study and management of human–water interactions at multiple scales. Water, 12, 1699. doi:10.3390/w12061699
- Pouladi, P., et al., 2019. Agent-based socio-hydrological modeling for restoration of Urmia Lake: application of theory of planned behavior. Journal of Hydrology, 576, 736–748. doi:10.1016/j.jhydrol.2019.06.080
- Pouladi, P., et al., 2020. Socio-hydrological framework for investigating farmers’ activities affecting the shrinkage of Urmia Lake; hybrid data mining and agent-based modelling. Hydrological Sciences Journal, 65 (8), 1249–1261.
- Rani, D., Srivastava, D., and Gulati, T., 2016. A set of linked optimization models for an inter-basin water transfer. Hydrological Sciences Journal, 61, 371–392. doi:10.1080/02626667.2014.986484
- Rey, D., Garrido, A., and Calatrava, J., 2016. An innovative option contract for allocating water in inter-basin transfers: the case of the Tagus-Segura Transfer in Spain. Water Resources Management, 30, 1165–1182. doi:10.1007/s11269-015-1219-0
- Seidl, C., Wheeler, S.A., and Zuo, A., 2020. Treating water markets like stock markets: key water market reform lessons in the Murray-Darling Basin. Journal of Hydrology, 581, 124399. doi:10.1016/j.jhydrol.2019.124399
- Sinha, P., et al., 2020. A new framework for integrated, holistic, and transparent evaluation of inter-basin water transfer schemes. Science of the Total Environment, 721, 137646. doi:10.1016/j.scitotenv.2020.137646
- Sivapalan, M., et al., 2014. Socio‐hydrology: use‐inspired water sustainability science for the Anthropocene. Earth’s Future, 2, 225–230. doi:10.1002/2013EF000164
- Tong, X., et al., 2018. Behaviour change in post-consumer recycling: applying agent-based modelling in social experiment. Journal of Cleaner Production, 187, 1006–1013. doi:10.1016/j.jclepro.2018.03.261
- Wheeler, S., et al., 2014. Reviewing the adoption and impact of water markets in the Murray–Darling Basin, Australia. Journal of Hydrology, 518, 28–41. doi:10.1016/j.jhydrol.2013.09.019
- Wheeler, S.A., et al., 2017. Developing a water market readiness assessment framework. Journal of Hydrology, 552, 807–820. doi:10.1016/j.jhydrol.2017.07.010
- Wilensky, U. and Rand, W., 2015. An introduction to agent-based modeling: modeling natural, social, and engineered complex systems with NetLogo. Cambridge, MA: MIT Press.
- Zhang, E., Xu, Z., and Yang, Z., 2018. Bottom-up quantification of inter-basin water transfer vulnerability to climate change. Ecological Indicators, 92, 195–206. doi:10.1016/j.ecolind.2017.04.019
- Zhou, Y., et al., 2017. Systematic impact assessment on inter-basin water transfer projects of the Hanjiang River Basin in China. Journal of Hydrology, 553, 584–595. doi:10.1016/j.jhydrol.2017.08.039