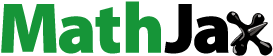
ABSTRACT
Background
Learning communities is a collaborative framework that can assist the adoption of the educational innovation of digital technologies, through reflective discourse and student-centred pedagogy.
Purpose
Framed within this framework, the study aimed to examine: a) the nature of elementary preservice teachers’ interactions, collaboration, and discussion during the learning community meetings concerning the design of science experiments with the use of digital technologies, and, b) the ways (if any) did the collaborative learning communities framework support preservice elementary teachers in developing digital technology-integrated science experiments.
Sample
The participants of the study were 12 preservice elementary teachers.
Design and Methods
The study took place during a university training course and data were collected over a period of six months. As part of this course, the participants engaged in the following: lectures and practicals about the use of digital technologies in science education and a series of collaborative tasks in which the participants, as a learning community, had to design science curriculum materials with the use of digital technologies. The data analysis included: a) mixed methods analysis of the learning community discussions regarding the content and nature of the discussion and b) network analysis of the design influences regarding the developed teaching material.
Results
The findings showed that the participants made extensive use of participatory and on-task discussions in the learning community and benefited greatly from the collaborative framework, especially through peer interactions and negotiations.
Conclusions
Findings speak to the importance of collaborative and dialogical environments, such as learning communities in designing technology-enhanced science teaching material.
Introduction
Cultivating design practices features centrally as a core goal of the science curriculum in reform documents around the world in general and in Europe in particular (European Commission Citation2021). The design process is an open-ended and student-centred activity of constructing artefacts, as for example, online courses and digital videos (Mishra and Koehler Citation2006) as well as science experiments that address content knowledge and practices (Stammes et al. Citation2020; van Riesen et al. Citation2018). When engaging in design practices, trainee teachers take an agentic role as they become practitioners themselves in authentic contexts. In doing so, they are not only provided with the opportunity to develop an understanding of how to incorporate technology effectively, but they are also provided with the opportunity to apply that understanding in the complex domains of real-world practice (Mishra and Koehler Citation2006). As welldocumented in the literature, the act of designing science experiments can also serve as a productive means for meaningful learning of science content and knowledge-building practices for teachers (Oshima et al. Citation2004). Similarly, regarding school students, their engagement with design and research courses can also result in significantly higher positive self-efficacy, increased enjoyment and less anxiety towards doing design tasks (Vossen et al. Citation2018).
However, design practices are considered quite a challenging task for beginning teachers given the high demand in knowledge, skills and values embedded in the design and implementation of inquiry-based practical work (Stammes et al. Citation2020). Hence, supporting frameworks and interventions need to be implemented in teacher training programmes concerning laboratory teaching (Duit and Tesch Citation2010). One such approach is the use of digital technology tools, such as Microcomputer-Based Laboratory (MBL) tools, also known as datalogging systems/dataloggers, which have been widely used for science experiments since the 80s. The diffusion of digital technologies in science classrooms has received increased attention in the past few years since innovative Information and Communication Technology (ICT) tools with innovative features arise by concurrently adding additional layers of complexity and additional demands on teachers (Waight and Abd-El-Khalick Citation2012). Collaboration with peers and experts comes as an approach that can facilitate the adoption and successful implementation of the educational innovation of digital technology (Bell, Maeng, and Binns Citation2013). This is precisely what the purpose of this study was through the use of Learning Communities in the context of a teacher preparation program.
Learning community is typically used to refer to small groups of teachers, students, researchers, and experts working together through a participatory, collaborative and reflexive approach that aims to facilitate the adoption of the educational innovation (Couso Citation2016). Empirical evidence points to the potential of learning communities to cause change in teachers’ attitudes and practices (Elster Citation2010) as well as to enhance their confidence and sharing of resources (Jones et al. Citation2013). This is achieved through opportunities for inquiry-oriented dialogue, critical reflection and participation needed; hence, the effectiveness of learning communities is not predefined (Couso Citation2016; Jones et al. Citation2013; Wenger Citation1998).
Grounded within these claims, for the purpose of the study reported in this paper we implemented a collaborative learning community framework that aimed at supporting groups of elementary preservice teachers to design and develop science experiments with the use of datalogging systems. Hence, the purpose of the study was to examine the ways in which a learning community setting supported preservice teachers to participate and integrate digital technologies in their instructional practices. In doing so, the study aims to contribute to an existing gap in the knowledge base regarding the nature of the interaction, collaboration, and discussion that takes place among teachers during the design and development process. Instead, the vast majority of studies in the context of technology education have treated collaboration mainly as a mediating factor while the data are mostly drawn through self-reported interviews and questionnaires (Bell, Maeng, and Binns Citation2013; Kafyulilo, Fisser, and Voogt Citation2015), while there is a lack of studies that include the analysis of teachers’ collaborative discourse when designing technology-enhanced instruction (Yeh, Chan, and Hsu Citation2021). More narrowly, the study aims to address the following research questions:
What is the content and the nature of elementary preservice teachers’ discussion and collaboration during the learning community meetings concerning the design of science experiments with the use of digital technology?
In what ways (if any) did the collaborative learning community setting support preservice elementary teachers in developing digital technology-integrated science experiments?
Literature review
Digital technologies in science teaching
MBL systems/dataloggers, consist of three main parts: a) electronic sensors and probes, b) a data collection and analysis device, as for example, a computer or more recently, a portable ‘smart’ device e.g. a tablet or mobile phone and c) the necessary data collection and analysis software (Sokoloff, Laws, and Thornton Citation2007). The main affordance of datalogging systems is that they provide the tools to visualise phenomena in real-time (Chen et al. Citation2014) and hence, can facilitate increased understanding (Donnelly-Hermosillo, Gerard, and Linn Citation2020; Sokoloff, Laws, and Thornton Citation2007). In addition, datalogging systems positively affect student motivation and interest while also reducing time for data collection and procedural tasks (Chen et al. Citation2014; Nicolaou et al. Citation2007). Graphs generated from dataloggers assist students in developing graph construction and interpretation skills, which also leads to an improved conceptual understanding of the phenomena (Donnelly-Hermosillo, Gerard, and Linn Citation2020; Nicolaou et al. Citation2007; Rane Citation2018).
Despite these advantages, a review of the literature also showcases various challenges related to the use of datalogging. First, students do not always connect observations with the related science content, focus more on the measuring process, and tend to stop the inquiry process when the measurements are completed (Lavonen, Juuti, and Meisalo Citation2003). Second, students have an ‘unrealistic’ trust in computers or datalogging equipment and have the perception that computers create precise data; therefore, they lack a critical view of the results generated by computers (Chen et al. Citation2014). Similarly, teachers themselves address several difficulties in integrating digital technologies, both because of a lack of knowledge on how to implement digital technologies in classrooms and because of attitudinal barriers (Prestridge Citation2017; Odom et al. Citation2011).
What these findings show is that technology alone will not automatically promote increased student learning. Instead, a deeper understanding of the factors that facilitate technology enactment is needed, away from faith-based approaches (Waight and Abd-El-Khalick Citation2012). Hence, it is imperative to also consider these factors and the general context in which digital technologies are embedded.
First, it is important that the student-centred potentialities and features of digital technology tools, such as the datalogging systems in our case, come along with student-centred pedagogies from the teachers in which the digital technology tools are used as a means to promote inquiry learning (Odom et al. Citation2011; Waight and Abd‐el‐khalick Citation2007). The Technological Pedagogical Content Knowledge (TPACK) framework that Mishra and Koehler (Citation2006) introduced, describes in a more holistic view the basis of effective teaching with technology and comes from the intersection of knowledge about pedagogy, content, and technology, as well as the intersections of them: a) Pedagogical Content Knowledge (PCK), b) Technological Content Knowledge (TCK), and c) Technological Pedagogical Knowledge (TPK). Particularly, PCK was initially introduced by Shulman (Citation1986) as the ‘blending of content and pedagogy into an understanding of how particular topics, problems, or issues are organised, represented and adapted to the diverse interests and abilities of learners, and presented for instruction’. However, more contemporary models of PCK consider it both as a knowledge base that is used to plan a topic-specific lesson in a specific classroom context and as a skill referring to the act of teaching (Gess-Newsome Citation2015). b) Technological Content Knowledge (TCK) is referring to the understanding of how Technology and Content influence and constrain one another, while c) Technological Pedagogical Knowledge (TPK) is referring to the understanding of how teaching and learning is been affected by particular technologies in particular ways. Recent updates of the TPACK framework incorporate the bidirectional influence that educational technology has on systems and cultures (Warr, Mishra, and Scragg Citation2019), while contextual knowledge is also taken into account (Mishra Citation2019). Furthermore, Yeh, Chan, and Hsu (Citation2021) in their literature analysis draw attention to the fact that few studies have analysed the collaborative discourse and the critical role of facilitators in orchestrating the discourse in TPACK development studies. Hence, they propose a collaboration-enriched TPACK framework that diversifies TPACK into three realms: i) personal TPACK, ii) collective i.e. shared/accepted by the community and iii) enacted TPACK when designing technology-enhanced instruction.
Second, assistance from people with specialised knowledge and experience in the domains of technology, but also across pedagogy and content is needed in order to sustain the diffusion of technology in schools. Consequently, teachers could handle the multitude of expectations that are placed on them, whilst the expert(s) can act as ‘translators’ of the educational innovation in several contexts (Waight and Abd-El-Khalick Citation2012).
Third, it is important to learn how to use digital technologies in authentic contexts, as for example, in interdisciplinary settings where digital technologies are incorporated in order to learn science content and not learning technology for its own sake. Hence, teachers learn how to implement digital technology in meaningful ways for teaching science content under certain pedagogies (Bell, Maeng, and Binns Citation2013; Iliaki et al. Citation2019). The idea of learning with technology rather than about technology is crucial in order to effectively integrate technology in science classrooms (Waight and Abd‐el‐khalick Citation2007).
Fourth, adopting an educational innovation, such as digital technologies is enhanced through social supporting contexts. Collaboration with peers, mentors, researchers, and experts can facilitate the adoption and sustainability of the educational innovation (Sgouros and Stavrou Citation2019), while reflection from peers, discussion and sharing practices can assist teachers to efficiently implement digital technologies (Bell, Maeng, and Binns Citation2013).
Collaborative learning
Collaborating teachers are provided with the opportunity to make their practices explicit to peers, share their experiences and tools and support one another in building productive variations of the instructional practices (Thompson et al. Citation2019). Furthermore, social interaction can promote students’ argumentation skills and support the development of metacognitive skills, since working in groups results in reducing the cognitive load, which can assist participants to focus more on planning, monitoring, controlling and evaluating skills on joint understandings. Interaction between members of the group can also assist them to improve their memory by increasing their information exchange and process information more thoroughly, which results in better performance (Wang, Fang, and Gu Citation2020; Mathabathe and Potgieter Citation2017).
Specifically, regarding the educational innovation of digital technology, implementing metacognitive approaches can make teachers conscious of their beliefs, which in turn informs teachers’ practices (Prestridge Citation2017). Since integrating education technology – as any educational innovation – is an open-ended task, reflection between peers is also imperative in order to understand, but also co-design the innovation so that it can be successfully adopted by the agents in a rather pragmatic approach. This process is continuous and iterative (Psillos and Kariotoglou Citation2016) and includes interactions with all biotic and abiotic factors of the ‘ecosystem’ –teachers, students, curriculum and technology (Waight and Abd-El-Khalick Citation2012).
However, scaffolding is needed so that all collaborating members engage in inquiry stages, interact with peers and make productive use of technological tools (Lämsä et al. Citation2018). Support from the expert is crucial in the explication and negotiation of concepts, in driving the discussion on-task and connecting the related science content as well as in regulating peer interaction (Strømme and Furberg Citation2015).
The imperative role of discussion
According to Gee (Citation2015), through discussion, participants do not only integrate communication skills, but also their actions, beliefs and values in a given social network. The content of the discussion topics as well as the nature of the discourse (open-ended or structured) are considered important factors which, among others, determine the ‘realisations’ of technology in classrooms (Waight and Abd‐el‐khalick Citation2007). Even though both dialogic and authoritative ways of discussion may be essential for teacher orchestration of whole-class discussions (Lehesvuori et al. Citation2019), it is still important to engage students in critical and philosophical modes or discussions in an inquiry-rich environment in order to gain a deep understanding of technology and how it is perceived by students (Waight and Abd‐el‐khalick Citation2007).
In a review of the literature, Bennett et al. (Citation2010) provided a set of general guidelines aimed at improving the effectiveness of the discussion. First, greater improvements in understanding occur when dissimilarity or conflict in understanding or views takes place, in contrast to the common trend that many students follow the ‘majority rule’ or tend to agree passively when some member(s) exert authority. Second, an administrator/leader of the discussion is useful in order to adopt an inclusive style, share tasks equitably around a group, motivate engagement by a number of participants and improve the quality of the discussion. Third, the discussion seems to be more productive if students are provided with specific, inquiry-focused tasks where assistance is given to students to develop understanding through the construction of arguments, as well as when students are given a fixed set of concepts to delimit the content of the discourse.
Summing up, the collaborative settings, as well as the discussion that takes place within those settings should be carefully examined in order to implement and maximise the efficacy of collaboration. This is precisely what we aimed to do in this study.
Methods
Theoretical framework
The study is theoretically framed within the Model of Educational Reconstruction for Teacher Education (ERTE, Van Dijk and Kattmann Citation2007), as it was adapted for the needs of a technology integration study (Nipyrakis and Stavrou Citation2022) and represented in . Central to the model are three domains that are closely integrated and dynamically interrelated: a) the design of learning environments from the preservice teachers, which in our case concerns datalogging learning environments, including studies of students’ learning and the clarification and analysis of the science content as well as the features and usability of the technological tools in order to be reconstructed/modified for science teaching, b) empirical studies on teacher education regarding their PCK and TPACK, studies about how teachers integrate technology and teacher training in the school laboratory and c) guidelines for preservice teacher education concerning the implementation of digital technologies in science education.
Figure 1. Model of Education Reconstruction for Teacher Education (ERTE) (Van Dijk and Kattmann Citation2007), adapted to the needs of the present study.
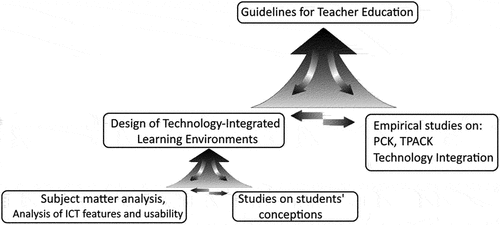
The ERTE model was developed based on the Model of Educational Reconstruction (MER, Duit et al. Citation2012) and it aims to bridge the gap between research and development. Namely, the ERTE model follows the same structure for teacher education as the MER (component (a) in ) does for educating students. Hence, the ERTE model brings empirical studies on teacher training and student learning into play when reconstructing the science content and the development of teacher training environments. Therefore, the findings of the study aim to provide guidelines and inform the design of professional development programmes for training teachers in designing science laboratory teaching material.
Participants
The participants of the study were 12 preservice elementary teachers in the fourth year of their studies. Prior to the study, they all had attended an undergraduate introductory science lesson and a science teaching methodology lesson. However, they had no previous training in teaching at the school laboratory or using dataloggers for science teaching. The learning community which was formed consisted of the 12 preservice teachers and a science education researcher (first author) as the administrator of the discussion by posing on-task questions and motivating preservice teachers’ engagement. The first author served also as the expert in the domains of science, school laboratory and technology.
Implementation of the study
The study took place during an elective undergraduate primary teacher MBL training course in which the 12 preservice teachers had enrolled and lasted one academic semester. The course consisted of an introductory phase, three design phases, and an implementation phase, as shown in .
Table 1. Implementation of the study.
More specifically: the introductory phase lasted two weeks and comprised both a theoretical lecture and a lab session about using datalogging systems in science education. Thereafter, each one of the three design phases comprised two weekly lab sessions, where preservice teachers in groups of two had to design and develop laboratory teaching material with the use of digital technology. In between the design phases, one Learning Community meeting (LC) was held for the purpose of engaging participants in reflecting on their lab practice and sharing knowledge with peers for the subsequent design phases (LC1 and LC2). Each team was assigned to design teaching material for one of the total six branches of science, divided into two sets as shown in , whilst at the end of each design phase, there was a cyclical swift of the assigned branches of science between the preservice teacher teams on the same set.
Figure 2. Branches of science assigned.
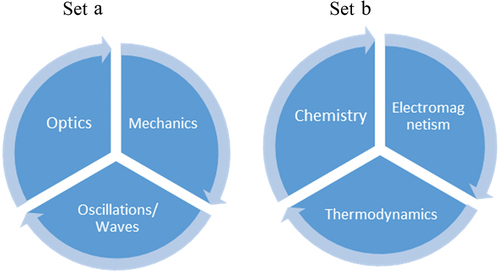
At the end of the course, the participants used the developed teaching material to teach school students during educational visits to the university science teaching lab. Finally, an overall reflection Learning Community meeting (Reflection LC) was held at the end of the semester, regarding their views and reflections about the use of digital technology in the school laboratory, collaborative learning, and the design, development and implementation of the teaching material.
Learning community meetings
Concerning the organisation of the learning community meetings, each group was asked to present their work and then discuss and reflect on it. The discussion was semi-scaffolded by the administrator, whilst the scaffolding questions posed to each experiment presentation were: a) what do you say/comment about the teaching goals in this experiment? Are they been fulfilled? b) what do you say/comment about the use of digital technologies in this experiment? The duration of each learning community meeting was approximately 3 hours.
Data collection & analysis
The data collected included the following: a) the developed teaching material, which included the science experiments that preservice teachers designed, developed and implemented along with exemplar worksheets, b) audio transcripts from the learning community meetings, and c) field notes from the researcher during the lab sessions about the development of the teaching material for triangulation. The notes were related to the implementation and use of dataloggers in the experiments, preservice teachers’ difficulties on the design, feedback from the researcher, and collaborative interactions between them.
Analysis of the nature of the discussions in the learning community
In order to analyse the discussion in the learning community meetings, categories from Kittleson and Southerland’s (Citation2004) research were derived and further modified for the needs of the present study, as shown in .
Figure 3. Coding scheme for the discussion in the learning community, as adapted from Kittleson and Southerland (Citation2004) and modified to the present study.
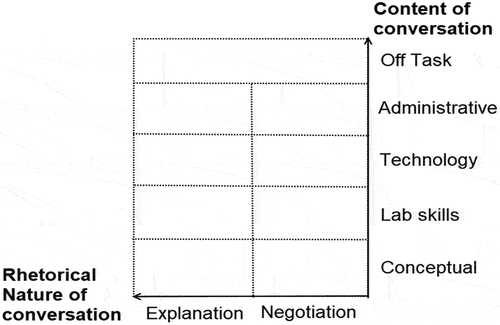
Hence, considering the content of the conversation, the nature of the discussion was coded as follows:
Conceptual (Con): discussion about science content.
Lab skills (Lab): procedural talk referring to the laboratory procedure & the inquiry method used
Technology (Tech): discussion referring to digital technologies e.g. their features, affordances and use
Administrative (Adm): non-scientific talk that had to do with the completion of tasks and organisational issues.
Off task (Off): conversation that does not address the task at hand, for example, extracurricular activities of the students or humour.
Considering the rhetorical nature of the conversation, the discussion was categorised as follows:
Explanation (Exp): when one person or one preservice teacher group (2 persons) was presenting their teaching material simulating a teacher-centred lecture.
Negotiation (Neg): when more than one person was substantially contributing to the conversation, either agreeing with, commenting or criticising their peers.
The Thematic Quotation (TQ) was used as the main unit of analysis. TQ was defined as any excerpt of the conversation that includes adequately codable information, according to the categories mentioned above. Several criteria were set by following an inductive coding approach concerning an indicative part of the transcribed text of the learning community discussions and a coding guideline was formulated for the specific demarcation of the TQ. The text was then revised and recoded when needed.
Subsequently, the defined TQs were analysed considering the nature and the content of the discussion deductively, following a structuring analysis technique under a nominal category assignment (Mayring Citation2014). Coding rules for the nominal category system were also formulated and the material was revised and recoded when needed.
We assumed that active participation in the learning community discussion and particularly in the discussion concerning their peers’ teaching material is an indicator of high collaboration levels. In specific, the participation rate was defined by:
Noteworthily, in this part, only the discussion referring to the three on-task fields (Con-Lab-Tech) was analysed. Therefore, in what follows, collaboration is been examined in terms of the extent to which teachers engage in on-task discussions about the teaching material of colleagues.
Analysis of TQs was carried out with the use of Atlas.ti software. Subsequently, quantified data were exported for statistical analysis with the use of IBM SPSS Statistics. In specific, TQs are exported as cases whereas codes assigned in each TQ are exported as variables. Given that the data were presented in a binary scale, non-parametric tests were applied. In specific, Mann-Whitney U tests were used for tracing differences between LC1 and LC2, as well as chi-squared tests for differences per learning community member. Non-parametric Friedman tests were used in order to trace differences between the three on-task fields (Con-Lab-Tech). In parallel, Cochran Q tests were also used due to the binary scale of the data, which yield same results.
In order to gain more in-depth insights about teachers’ views and difficulties considering the design of teaching material integrating digital technologies, learning community discussions were also analysed qualitatively by using qualitative content analysis. Hence, recurring patterns were identified and coded, along with theoretically interesting typologies (Mayring Citation2014). Consequently, triangulation of results from the statistical analysis with the qualitative data was applied in several cases by following a multiple methods analysis method in order to increase validity (Morse Citation2010).
Analysis of the design influences
To examine the ways in which the design of the learning community supported the participants in developing digital technology-integrated science experiments, the analysis unit used is the Design Influence (DI). DI was defined as any idea or comment expressed verbally by a learning community member during the LC meetings regarding the design of an experiment and which was implemented by a peer teacher group in subsequent design phases.
Similarly to TQs, DIs were also defined in terms of inductively made criteria and a coding guideline was formulated. Since DIs concern relative data, network analysis (Borgatti, Everett, and Johnson Citation2018) was used for this part of the analysis. In particular, each DI is represented by an arrow starting from the sender – the learning community member that expressed the idea/comment and ending to the receiver – the teacher group members that implemented the idea in the design process of experiments.
For the purpose of the analysis, the following network metrics (Borgatti, Everett, and Johnson Citation2018) were used to probe the general interaction in the group:
Degree is simply the number of ties that a node has in a non-directed network. Given that the data are directed, we calculate Out Degree (OutDeg) and In Degree (InDeg) which were used to refer to the number of outgoing and ingoing ties/arrows respectively, in this case, the number of transmitted DIs.
Density is calculated by the number of ties in the network, expressed as a proportion of the number possible. Noteworthily, density is a metric that is used in a comparative way, as for example, to compare interaction levels of similar groups and its interpretation is highly related to the context.
Average degree is the average number of ties that a node has and is computed by dividing the number of ties that each node has with the total number of nodes.
Centralisation is a metric that refers to the extent to which a network is dominated by a single node. Since our data are directed, centralisation is also calculated separately as Centralisation(Out) for outgoing ties and Centralisation(In) for incoming ties.
Homophily represents the tendency of nodes to interact with other nodes of the same subgroup.
A network analysis was carried out with the use of UCINET software, whilst the NetDraw tool was used to create visualisations of the networks. The comparison between the densities of networks in LC1 and LC2 was made with the use of bootstrap methods.
Findings
Discussions in the LC
In this section, we discuss the content and the nature of the interactions and conversations that took place during the LC meetings (LC1 & LC2) in the context of a preservice teacher training course focusing on technology integration.
Therefore, the nature of the whole-group discussion regarding the content and the rhetorical nature of the discussion during both design LC meetings (LC1 & LC2) is presented in , whilst discussion in LC1 and discussion in LC2 are separately represented in respectively.
Figure 4. Discussion in both LCs.
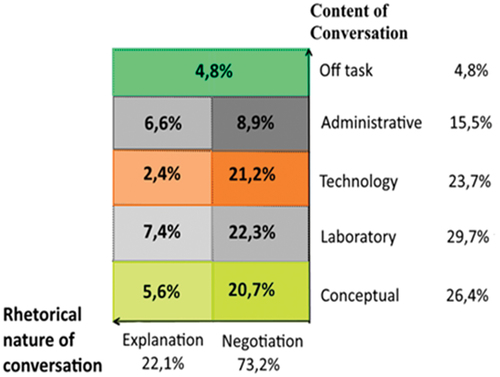
Figure 5. (a) Discussion in LC1. (b) Discussion in LC2.
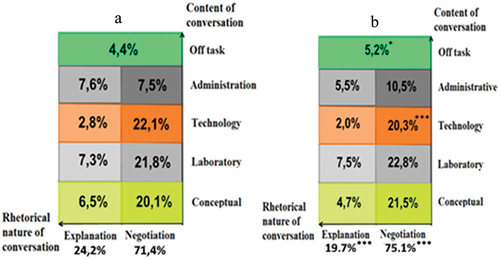
At a first glance, it becomes obvious that the discussion remained mostly on-task regarding the three on-task fields; however, discussion for lab skills prevailed in both LC meetings (p < 0,001).
Concerning the nature of the discussion, preservice teachers significantly preferred to discuss in a more negotiating manner. Moreover, this tendency became more obvious in LC2, where negotiating dialogue significantly increased over explanatory lecturing. Consequently, the participants made extended use of the student-centred discussion setting of the learning community framework. We can infer that both the semi-scaffolding structure of the discussion as well as teachers’ orientation towards more negotiating talk – since they suggested as co-administrators a shift on the discussion agenda – affected this result.
As regards the content of the discussion, the fact that discussion about lab skills significantly prevails could initially be interpreted by the need that teachers had on learning about the experimental process, practical issues as well as the inquiry method applied in the experiments. Analysis of their lesson plans as well as qualitative analysis of the discussions confirm their difficulties in this section, regarding the formulation of the teaching goals and implementing them in the specific experimental procedure. Specifically, in a percentage of 11% of total descriptions of experiments (n = 11 out of 90), serious incompatibilities of the teaching goals with the experimental procedure were coded, whereas in a percentage of 11% (n = 11 out of 90), partial incompatibilities were coded. That may sound contradictory in a sense, since teachers are having adequate academic training in science teaching and inquiry. However, it is the lack of interconnected knowledge of PCK when using MBL i.e. TPACK knowledge that also levelled up the rate of difficulty in designing relative lab procedures and drove teachers to discuss extensively in the lab skills section. The excerpts below provide some reflections on this issue.
4a: and the last and bigger problem that I think all of us had in the course – imagine how much more for a kid – was how to combine the experiment with ICT. What experiment should I find so that (ICT) measurements could be implemented.
1b: difficulty in interconnecting the experiments with microcomputer systems, I mean many we had found some experiments, but we couldn’t (Transcript from a group conversation).
A significant decrease occurred regarding the discussion about Technology from LC1 to LC2. Reflections from preservice teachers reveal that teachers addressed several difficulties in getting to know and implement digital technology tools in the design, especially in earlier stages. Moreover, the constructivistic setting of learning about datalogging features by exploring or testing by themselves was deemed demanding, since teachers felt they needed additional training about using dataloggers and in a rather more traditional manner.
1b: difficulty in understanding the operation of (ICT) systems. I mean, in the beginning, because we hadn’t analysed how it works and hadn’t discussed about it, I didn’t know how to use that. I mean it took me some time to learn how to do measurements. Not from the first time we did, I couldn’t do that, but then I got familiarised and learned how to do the measurement, because we didn’t explain much of it. (Transcript from a group conversation)
Exploration of the potentialities of digital technology tools from the teachers following a rather pragmatic approach initially left teachers with a lack of knowledge needed, given the short period of the training phase. Consequently, the learning community setting was called upon to complete this lack of expertise needed, especially in LC1. Noteworthily, negotiating discussion about digital technology in LC1 was comparatively higher from Lab skills or Conceptual, whilst the opposite occurs in LC2.
Learning community members’ participation in the discussion
In this section, we further examine the participation of the LC members in discussions concerning the teaching material of their peers. Specifically, we examine the participation rate of preservice teachers, which was deemed to be an indicator of collaboration among peers.
Hence, in , we present the extent of participation and participation rate of the learning community members. Obviously, the expert was excluded from the participation rate analysis, since he was not designing his own teaching material.
Table 2. Participation & participation rate in the discussion.
As shown in the Table, 4 learning community members had relatively low participation in the discussion (TQs<500), whilst, on the contrary, 3 learning community members were actively participating (TQs>800). Concerning their participation rate in discussions of their peers, we can see that 4 learning community members had low participation in peers’ teaching material (<30%), whilst 3 of them are also members with low participation in general. However, important is the fact that 5 learning community members showed a significant increase in their participation rate from LC1 to LC2, while 2 showed a decrease. Important to note is that 3a had a voice problem in LC2 that indisputably affected her participation in the group discussion of other groups. Therefore, we can infer that most preservice teachers (n = 8) showed adequate participation in the group discussion and in the discussion concerning peers, whereas their participation rate showed a general tendency to increase from LC1 to LC2. In an effort to justify this increase, we could pose two arguments. First, we could argue that the participants were more actively engaged in the discussion since they were more familiar with the learning community context, as it is also apparent in the negotiating nature of the discussion presented above in .
2b: For example, if you assign me Optics to do something, I couldn’t do that […] I don’t know how to handle that. But on those that I was assigned and I have accomplished I know them very well I believe. (Transcript from a group conversation)
Second, as becomes apparent in the excerpt above, we could argue that the groups that were assigned to design experiments in a branch of science, could participate more in the discussion especially regarding this part, since their knowledge and skills were probably enhanced.
Development of technology-enhanced science experiments
In this section, we discuss the effect of the LC framework on the design of teaching material by preservice teachers. Specifically, we examine the DIs that the preservice teachers shared and received from fellow LC members, as well as the general network patterns that appear. Particularly, as shown below, Sociogram 1 represents DIs in both LCs whilst 2a and 2b represent DIs in LC1 and LC2, respectively.
Sociogram 1: DIs in both LCs.
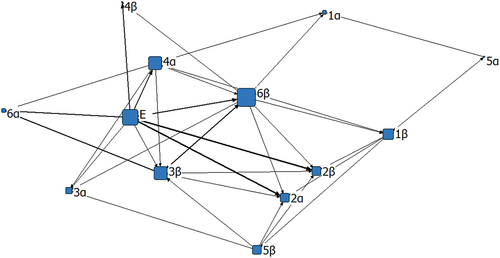
Sociogram 2b: DIs in LC2.
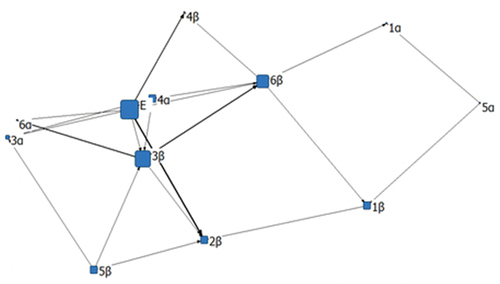
Hence, regarding the whole network, as shown in , total DIs were 28 and density had a value of 0,359.
Table 3. Network metrics for DIs.
Considering that the total experiments in design phases B and C were 60 –since in phase A there was no previous LC meeting to affect the design of experiments–, we can infer that preservice teachers got influenced by peers to an adequate number of experiments (46,7% in phases B & C). Additionally, DIs from design phase B to design phase C followed a rather increasing pattern (p = 0,114), since preservice teachers got influenced more by peers in the latter design phase.
Regarding the content of DIs, as presented in , most DIs relate to Con & Lab field, also contributing to the rather increased difficulty in the Tech field, as mentioned previously in the analysis.
Table 4. Content of DIs.
shows that the network has a rather moderate centralisation value of 0,472 for outdegrees and 0,201 for indegrees. It is important to note, however, that the difference between outcentralization and incentralisation relates on the one hand to the fact that DIs were coded to both persons in the teacher group who implemented the DI and on the other hand because the expert was not participating as a receiver of DIs. Moreover, comparing networks in design phases B and C, we can also see that the network in design phase C is more centralised, which means that preservice teachers got influenced by the expert – which was the more influencing node – more in the third design phase. This finding is in accordance with the difficulty that participants expressed in learning community discussions about designing non-trivial experiments in the latter phase, as mentioned below.
4b:Yes, that is a problem that all groups had, and also, the science domains were limited. Thus, suppose I had been assigned to Optics and the girls get assigned to it once more, the third group would then face severe restrictions, and would say: those (experiments) have already been developed, what, how could we modify an experiment since we already had faced difficulty to search and implement ICT. (Transcript from a group conversation)
Consequently, we can infer that assistance from the expert was also deemed valuable for preservice teachers in the design process, in a similar way to the previous part of the analysis.
shows that all groups took into consideration ideas from peers to some extent.
Table 5. Dis per learning community member.
In specific, 1 group can be rather characterised as low influenced (DIs≤2), 3 groups as moderately influenced (3 ≤ DIs≤5) and two groups as highly influenced (DIs≥6). On the other hand, diversity was noted regarding the transmission of DIs (DIs out), since a non-negligible number (n = 6) of learning community members were not taken into consideration, whilst 5 learning community members – the expert included – transmitted DIs to more than 4 peers i.e. 2 teacher groups. Notably, comparing design phase B with C, 4 preservice teachers increased their participation as transmitters of DIs, whilst only one showed a decrease, contributing to the general tendency of the learning community group to collaborate more in the design process. Therefore, we can infer that the tendency for increased collaboration in the design process is a tension rather related to the whole group level and not to an individual member or the expert.
Running a Pearson correlation test between DI networks of the second and the third design phase, we found a significant and rather strong correlation (0,466, p < 0.001), which means that the specific learning community members who highly interacted, the ones who showed low interaction and the ones who didn’t interact follow, to a great extent, a similar pattern. Regardless of the increase in in/out DIs, however, most participants kept their network attitudes at similar levels.
Moreover, examining homophily between the set of teacher groups A and the set of teacher groups B – the ones that shared the same branches of science–, we can see in that the network was, to a considerable extent, homophilous.
Table 6. DI Homophily between set of teacher groups.
That result derives from the fact that teacher groups who had already been assigned a specific branch of science had cultivated considerably developed topic-specific content knowledge, lab skills and TCK and were more likely to participate and influence peers within the same branch of science. As stated below:
‘2b: actually that assisted the teams that were in the same part. For example us who we were assigned Thermodynamics, Chemistry, Electromagnetism, the three (groups) of us that had these
Expert: you mean of the same set of groups, right?
2a: yes. That substantially helped. Because I was listening to experiments in Mechanics and even though I was getting into the process of thinking if it was like that or otherwise, however
Expert: you could help though. Maybe you didn’t get any help yourself but you could help others
2a: yes but it was tiring
1b: she would be more concentrated on the other ones (experiments) though’ (Transcript from a group conversation)
‘2b: the only positive that I benefitted from here is that we were watching the branches of science that we were about to have in the future, we knew what we would have on the one hand, we will listen to what the girls from Chemistry will say, yes, in case we say some idea on how to evolve it ok -but on branches of science that were not related to me, for example Optics that I wouldn’t be assigned, and I couldn’t understand because I hadn’t read about its theory and I haven’t experienced it, I couldn’t express an opinion. (Transcript from a group conversation)
Noteworthily, from the above reflections of 2b,1b and 2a, we can infer another parameter for the increased DIs between the same set of groups, that of task relevance. The groups within the same set who shared the same content knowledge had a reason to collaborate more and give extra attention to peers in relation to the teacher groups who shared different content knowledge. We can hypothesise that the same parameter also applies to the shared lab instruments and datalogging tools regarding those branches of science. The above reflections implicitly refer to increased PCK and TPACK from the teachers regarding these branches of science.
Overall, the findings show that the opportunity to engage within learning communities provided participants with support to overcome content and pedagogical difficulties. As illustrated in the findings, the participants acted as active listeners to peers’ ideas, and furthermore used a considerable amount of them in order to design their own teaching material. Therefore, they made use of collaborative learning and multiple perspectives dimensions which are key characteristics of an LC framework (Slutsky and Allen Citation2005). Furthermore, assistance from the expert was made available and facilitated the design of the teaching material when needed, following recommendations that appear in the literature about LCs (Couso Citation2016).
Conclusions and implications
The purpose of this study was to examine the effect that the collaborative learning community framework had in supporting preservice elementary teachers’ knowledge and skill development in the context of a technology integration laboratory training programme. More specifically, the study aimed at examining: a) the nature of the discussions during the learning community meetings, and b) the ways in which designing science experiments in a datalogging systems environment support participants’ engagement. Empirical data from the analysis support that collaboration did have a mediating effect on preservice teachers’ training, since: a) preservice teachers participated substantially more in negotiating discussions concerning science concepts, laboratory skills and technology, b) most preservice teachers had adequate participation in the discussion, as well as in discussions about peers’ teaching material, acting as active listeners (Slutsky and Allen Citation2005), c) preservice teachers influenced and got influenced from peers concerning the design of technology-enhanced experiments.
Hence, the findings indicate that a dialogical and inquiry-oriented environment was fostered, which is an important prerequisite for an effective learning community (Couso Citation2016). Furthermore, the findings of the study revealed another parameter about the implementation of learning communities: that support from the learning community was also time-dependent, or more accurately process-dependent. Although the number of iterations and the LC meetings was small in the current programme, yet significant shifts were observed between the iterations of the design phases. In specific, most preservice teachers increased the influences concerning the design process, increased their participation in discussions concerning peers, as well as they increased the negotiating dialogue between them. Consequently, the effect of collaboration was becoming more apparent as the iterations proceeded.
Importantly, preservice teachers also claimed increased difficulties in the latter design phases regarding the design of non-trivial experiments, i.e. experiments that their peers haven’t already accomplished. However, the collaboration levels, in terms of negotiative discussions, peers’ participation and design influences increased. Therefore, collaboration facilitated preservice teachers in demanding conditions where complexity increased.
We offer two possible explanations for the increasing trend in collaboration levels. First, driven by the qualitative analysis of the participants’ reflections, the groups that were assigned a branch of science, with specific digital technology tools and under specific lab settings had cultivated increased knowledge, skills and experience in that area. This finding also derives from the homophily of DIs observed between the teacher groups who shared the same branches of science. Hence, these peers could increase their assistance to peers who would be assigned the same science areas. Second, the participants who shared the same branches of science had increased levels of joint enterprise (Wenger Citation1998), since they perceived higher task relevance with these peers; therefore, they were not only more skilled but also more motivated to interact.
Results regarding the facilitating effect of LC mentioned above were also triangulated by most preservice teachers’ reflections during the Reflection LC discussion and ppt presentations, where they were explicitly asked to comment about the influence of the LC in designing teaching material. Nevertheless, we acknowledge that the findings of the study are limited due to the short-term nature of this intervention. Future steps of our work will examine whether this impact was sustained through a follow-up study with the same participants in the future.
The findings also advocate that assistance from the expert was quite important, both in the design of datalogging experiments, in participating actively in discussions, as well as in cognitive interaction by sharing content and skill knowledge, which is in agreement with existing literature (Couso Citation2016; Waight and Abd-El-Khalick Citation2012; Yeh, Chan, and Hsu Citation2021). As the findings from the analysis of the role of the expert reveal, the expert’s support was also content- and time-dependent, adjusting to preservice teachers’ needs in each phase or field, contributing to calls for flexible and adaptive design of guidance in the literature (van Riesen et al. Citation2018). Noteworthily, the expert’s support was more dominant in the field of technology, revealing that integrating digital technology and digital technology-related issues were also considered of high complexity (Nipyrakis and Stavrou Citation2022; Waight and Abd-El-Khalick Citation2012) in the present study as well.
Moreover, the fact that the discussion remained to a great extent on-task could be also considered an aiding parameter for effective collaboration. We could infer that scaffolding of the discussion, as well as having an administrator of the discussion affected this result, albeit a more careful examination of the administrator’s interventions and type of orchestration of the discussion is needed (Lehesvuori et al. Citation2019). However, the findings showed that a small number of participants had limited contribution to the cognitive interaction and concurrently low participation in the discussion and in influencing peers in the design process, whereas peers who were quite active in the discussion, were also active in the design process. There seems to be a correlation between active participation in the discussion and active participation in the design process, a statement which requires further examination.
Overall, the findings of the study speak to the importance of learning communities and collaborative tasks in technology integration efforts. Guidelines for learning community contexts are provided in which critical reflection, sharing ideas and iterative engagement to common goals can progressively assist teachers to integrate digital technology and overcome difficulties. An in-depth examination of the nature of teachers’ collaboration as members of the learning community during the implementation of the teaching material in school settings could provide evidence of the sustainability of the learning community following teacher preparation.
Disclosure statement
No potential conflict of interest was reported by the authors.
References
- Bell, R. L., J. L. Maeng, and I. C. Binns. 2013. “Learning in Context: Technology Integration in a Teacher Preparation Program Informed by Situated Learning Theory.” Journal of Research in Science Teaching 50 (3): 348–379. doi:10.1002/tea.21075.
- Bennett, J., S. Hogarth, F. Lubben, B. Campbell, and A. Robinson. 2010. “Talking Science: The Research Evidence on the Use of Small Group Discussions in Science Teaching.” International Journal of Science Education 32 (1): 69–95. doi:10.1080/09500690802713507.
- Borgatti, S. P., M. G. Everett, and J. C. Johnson. 2018. Analyzing Social Networks. London: Sage.
- Chen, S., W. H. Chang, C. H. Lai, and C. Y. Tsai. 2014. “A Comparison of students’ Approaches to Inquiry, Conceptual Learning, and Attitudes in Simulation‐based and Microcomputer‐based Laboratories.” Science Education 98 (5): 905–935. doi:10.1002/sce.21126.
- Couso, D. 2016. “Participatory Approaches to Curriculum Design from a Design Research Perspective.” In Iterative Design of Teaching-Learning Sequences, edited by D. Psillos and P. Kariotoglou, 47–71. Dordrecht: Springer.
- Donnelly-Hermosillo, D. F., L. F. Gerard, and M. C. Linn. 2020. “Impact of Graph Technologies in K-12 Science and Mathematics Education.” Computers & Education 146: 103748. doi:10.1016/j.compedu.2019.103748.
- Duit, R., Gropengießer, H, Kattmann, U., Komorek, M., Parchmann, I. 2012. The Model of Educational Reconstruction – a Framework for Improving Teaching and Learning Science. In Science Education Research and Practice in Europe. Cultural Perpectives in Science Education, edited by D. Jorde, J. Dillon. 5th ed. Rotterdam: SensePublishers.
- Duit, R., and M. Tesch. 2010. On the Role of the Experiment in Science Teaching and Learning–Visions and the Reality of Instructional Practice. M. Kalogiannakis, D. Stavrou, and P. Michaelidis, edited by Proceedings of the 7th International Conference on Hands-on Science. 25-31 July 2010, Rethymno-Crete, 17–30.
- Elster, D. 2010. “Learning Communities in Teacher Education: The Impact of E‐competence.” International Journal of Science Education 32 (16): 2185–2216. doi:10.1080/09500690903418550.
- European Commission. 2021. Proposal for a Council Recommendation on Blended Learning for High Quality and Inclusive Primary and Secondary Education. https://eur-lex.europa.eu/legal-content/EN/TXT/PDF/?uri=CELEX:52021DC0455&from=EN
- Gee, J. 2015. Social Linguistics and Literacies: Ideology in Discourses. Abingdon: Routledge.
- Gess-Newsome, J. 2015. “A Model of Teacher Professional Knowledge and Skill Including PCK: Results of the Thinking from the PCK Summit.” In Re-Examining Pedagogical Content Knowledge in Science Education, edited by A. Berry, P. Friedrichsen, and J. Loughran, 28–42. Abingdon: Routledge.
- Iliaki, G., A. Velentzas, E. Michailidi, and D. Stavrou. 2019. “Exploring the Music: A Teaching-Learning Sequence About Sound in Authentic Settings.” Research in Science & Technological Education 37 (2): 218–238. doi:10.1080/02635143.2018.1526170.
- Jones, M. G., G. E. Gardner, L. Robertson, and S. Robert. 2013. “Science Professional Learning Communities: Beyond a Singular View of Teacher Professional Development.” International Journal of Science Education 35 (10): 1756–1774. doi:10.1080/09500693.2013.791957.
- Kafyulilo, A. C., P. Fisser, and J. Voogt. 2015. “Supporting Teachers Learning Through the Collaborative Design of Technology-Enhanced Science Lessons.” Journal of Science Teacher Education 26 (8): 673–694. doi:10.1007/s10972-015-9444-1.
- Kittleson, J. M., and S. A. Southerland. 2004. “The Role of Discourse in Group Knowledge Construction: A Case Study of Engineering Students.” Journal of Research in Science Teaching 41 (3): 267–293. doi:10.1002/tea.20003.
- Lämsä, J., R. Hämäläinen, P. Koskinen, and J. Viiri. 2018. “Visualising the Temporal Aspects of Collaborative Inquiry-Based Learning Processes in Technology-Enhanced Physics Learning.” International Journal of Science Education 40 (14): 1697–1717. doi:10.1080/09500693.2018.1506594.
- Lavonen, J., K. Juuti, and V. Meisalo. 2003. “Designing a User‐friendly Microcomputer‐based Laboratory Package Through the Factor Analysis of Teacher Evaluations.” International Journal of Science Education 25 (12): 1471–1487. doi:10.1080/0950069032000072755.
- Lehesvuori, S., M. Hähkiöniemi, J. Viiri, P. Nieminen, K. Jokiranta, and J. Hiltunen. 2019. “Teacher Orchestration of Classroom Interaction in Science: Exploring Dialogic and Authoritative Passages in Whole-Class Discussions.” International Journal of Science Education 41 (17): 2557–2578. doi:10.1080/09500693.2019.1689586.
- Mathabathe, K. C., and M. Potgieter. 2017. “Manifestations of Metacognitive Activity During the Collaborative Planning of Chemistry Practical Investigations.” International Journal of Science Education 39 (11): 1465–1484. doi:10.1080/09500693.2017.1336808.
- Mayring, P. 2014. Qualitative Content Analysis: Theoretical Foundation, Basic Procedures and Software Solution. Klagenfurt.
- Mishra, P. 2019. Considering Contextual Knowledge: The TPACK Diagram Gets an Upgrade.
- Mishra, P., and M. J. Koehler. 2006. “Technological Pedagogical Content Knowledge: A Framework for Teacher Knowledge.” Teachers College Record 108 (6): 1017. doi:10.1111/j.1467-9620.2006.00684.x.
- Morse, J. M. 2010. “Procedures and Practice of Mixed Method Design: Maintaining Control, Rigor, and Complexity.“ In Sage Handbook of Mixed Methods in Social & Behavioural Research, edited by A. Tashakkori, C. Teddlie, 339–353. 2nd ed. Thousand Oaks: Sage.
- Nicolaou, C. T., I. Nicolaidou, Z. Zacharia, and C. P. Constantinou. 2007. “Enhancing Fourth graders’ Ability to Interpret Graphical Representations Through the Use of Microcomputer-Based Labs Implemented Within an Inquiry-Based Activity Sequence.” Journal of Computers in Mathematics and Science Teaching 26 (1): 75–99.
- Nipyrakis, A., and D. Stavrou. 2022. “Integration of ICT in Science Education Laboratories by Primary Student Teachers.” In STEM, Robotics, Mobile Apps in Early Childhood and Primary Education, edited by S. Papadakis and M. Kalogiannakis, 55–78. Singapore: Springer.
- Odom, A. L., J. M. Marszalek, E. R. Stoddard, and J. M. Wrobel. 2011. “Computers and Traditional Teaching Practices: Factors Influencing Middle Level students’ Science Achievement and Attitudes About Science.” International Journal of Science Education 33 (17): 2351–2374. doi:10.1080/09500693.2010.543437.
- Oshima, J., R. Oshima, I. Murayama, S. Inagaki, M. Takenaka, H. Nakayama, and E. Yamaguchi. 2004. “Design Experiments in Japanese Elementary Science Education with Computer Support for Collaborative Learning: Hypothesis Testing and Collaborative Construction.” International Journal of Science Education 26 (10): 1199–1221. doi:10.1080/0950069032000138824.
- Prestridge, S. 2017. “Examining the Shaping of teachers’ Pedagogical Orientation for the Use of Technology.” Technology, Pedagogy and Education 26 (4): 1–15. doi:10.1080/1475939X.2016.1258369.
- Psillos, D., and P. Kariotoglou. 2016. “Theoretical Issues Related to Designing and Developing Teaching-Learning Sequences.” In Iterative Design of Teaching-Learning Sequences, edited by D. Psillos and P. Kariotoglou, 11–34. Dordrecht: Springer.
- Rane, L. V. 2018. “Microcomputer Based Laboratory–An Effective Instructional Tool: A Review.” IJRAR-International Journal of Research and Analytical Reviews (IJRAR) 5 (1): 530–538.
- Sgouros, G., and D. Stavrou. 2019. “Teachers’ Professional Development in Nanoscience and Nanotechnology in the Context of a Community of Learners.” International Journal of Science Education 41 (15): 2070–2093. doi:10.1080/09500693.2019.1659521.
- Shulman, L. S. 1986. “Those Who Understand: Knowledge Growth in Teaching.” Educational Researcher 15 (2): 4–14. doi:10.3102/0013189X015002004.
- Slutsky, R., and A. Allen. 2005. “A Time for Change: Implementation of a Community of Learners Model.” The Teacher Educator 40 (4): 278–298. doi:10.1080/08878730509555366.
- Sokoloff, D. R., P. W. Laws, and R. K. Thornton. 2007. RealTime Physics: Active Learning Labs Transforming the Introductory Laboratory. European Journal of Physics 28 (3): S83. 10.1088/0143-0807/28/3/S08
- Stammes, H., I. Henze, E. Barendsen, and M. de Vries. 2020. “Bringing Design Practices to Chemistry Classrooms: Studying teachers’ Pedagogical Ideas in the Context of a Professional Learning Community.” International Journal of Science Education 42 (4): 526–546. doi:10.1080/09500693.2020.1717015.
- Strømme, T. A., and A. Furberg. 2015. “Exploring Teacher Intervention in the Intersection of Digital Resources, Peer Collaboration, and Instructional Design.” Science Education 99 (5): 837–862. doi:10.1002/sce.21181.
- Thompson, J. J., S. Hagenah, S. McDonald, and C. Barchenger. 2019. “Toward a Practice‐based Theory for How Professional Learning Communities Engage in the Improvement of Tools and Practices for Scientific Modeling.” Science Education 103 (6): 1423–1455. doi:10.1002/sce.21547.
- Van Dijk, E. M., and U. Kattmann. 2007. “A Research Model for the Study of Science teachers’ PCK and Improving Teacher Education.” Teaching and Teacher Education 23 (6): 885–897. doi:10.1016/j.tate.2006.05.002.
- van Riesen, S. A., H. Gijlers, A. Anjewierden, and T. de Jong. 2018. “The Influence of Prior Knowledge on Experiment Design Guidance in a Science Inquiry Context.” International Journal of Science Education 40 (11): 1327–1344. doi:10.1080/09500693.2018.1477263.
- Vossen, T. E., I. Henze, R. C. A. Rippe, J. H. Van Driel, and M. J. De Vries. 2018. “Attitudes of Secondary School Students Towards Doing Research and Design Activities.” International Journal of Science Education 40 (13): 1629–1652. doi:10.1080/09500693.2018.1494395.
- Waight, N., and F. Abd‐el‐khalick. 2007. “The Impact of Technology on the Enactment of “Inquiry” in a Technology Enthusiast’s Sixth Grade Science Classroom.” Journal of Research in Science Teaching 44 (1): 154–182. doi:10.1002/tea.20158.
- Waight, N., and F. Abd-El-Khalick. 2012. “Nature of Technology: Implications for Design, Development, and Enactment of Technological Tools in School Science Classrooms.” International Journal of Science Education 34 (18): 2875–2905. doi:10.1080/09500693.2012.698763.
- Wang, C., T. Fang, and Y. Gu. 2020. “Learning Performance and Behavioral Patterns of Online Collaborative Learning: Impact of Cognitive Load and Affordances of Different Multimedia.” Computers & Education 143: 103683. doi:10.1016/j.compedu.2019.103683.
- Warr, M., P. Mishra, and B. Scragg. 2019, March. Beyond TPACK: Expanding Technology and Teacher Education to Systems and Culture. In Society for Information Technology & Teacher Education International Conference, 2558–2562. Las Vegas: Association for the Advancement of Computing in Education (AACE).
- Wenger, E. 1998. Communities of Practice: Learning, Meaning, and Identity. Cambridge: Cambridge University Press.
- Yeh, Y. F., K. K. H. Chan, and Y. S. Hsu. 2021. “Toward a Framework That Connects Individual TPACK and Collective TPACK: A Systematic Review of TPACK Studies Investigating Teacher Collaborative Discourse in the Learning by Design Process.” Computers & Education 171: 104238. doi:10.1016/j.compedu.2021.104238.