ABSTRACT
This study aimed to compare impact loading between two age groups of preschool children (3–4 and 5–6 years old) and one group of young adults representing mature level of running skill (n = 15 per group). Three-dimensional biomechanical data were collected during running barefoot, in minimalist and running shoes. A two-way mixed ANOVA was performed to assess age and footwear differences in vertical instantaneous loading rate (VILR). An interaction was found in VILR. Older (5–6) preschoolers had 30–31% lower VILR than younger (3–4) (p = 0.012, d = 1.02; p = 0.001, d = 1.18) and adults had 51–77% lower VILR than younger preschoolers (p = 0.001, d = 1.85; p = 0.001, d = 2.82) in minimalist and running shoes, respectively. Additionally, adults had lower VILR than older preschoolers in running shoes (p = 0.001, d = 2.68). No differences were found between older children and adults in barefoot and minimalist shoes. Loading decreased with increasing age, particularly in minimalist and running shoes. Unchanged cadence and running speed did not explain the decrease of VILR during preschool age. The explanation likely underlies in lower limb alignment during footstrike and developmental ontogenetic changes.
Introduction
Children spend most of their awake time playing games which very often include running (Wood et al., Citation2020; Borghese & Janssen, Citation2019). While there are a number of different types of footwear for children, in recent years, minimalist shoes have gradually become popular with parents. This has often led parents to wonder about what to put on their children’s feet. Minimalistic footwear has been suggested to imitate barefoot locomotion (Davis et al., Citation2021), which has often been discussed with certain benefits such as prevention against flat feet (Rao & Joseph, Citation1992), improved sprinting performance (Mizushima et al., Citation2021), balance, and jumping skills in children (Zech et al., Citation2018). Wearing minimalist shoes (46% minimalist index) also showed improved balance skills in preadolescent and adolescent children (aged 9–12 years; Quinlan et al., Citation2022). Moreover, running kinematic similarities between barefoot and very minimalist shoes (96% minimalist index) have been observed in preadolescent (Hollander et al., Citation2014) and preschool children (Plesek et al., Citation2021). However, a recent study showed higher impact loading in very minimalist shoes compared to barefoot running and contrasted these promising characteristics of minimalist shoes in imitating barefoot running in the early years of running development (Plesek et al., Citation2022). Nevertheless, there is little research investigating possible differences in running kinetics between different age groups.
A considerable amount of literature has been published on the biomechanical development comparing early and advanced stages of walking skill acquisition (Zeininger et al., Citation2018; Fukuchi et al., Citation2019). Surprisingly, very few studies investigated differences in running footstrike biomechanics (including impact loading) between different ages of preschoolers, older children, adolescent or adult populations. Although running has been reported as the most common physical activity in preschool children (Wood et al., Citation2020), there is only one recent study that compared biomechanics including impact loading during shod running between preschool children (3–6 years) and adults (Wei et al., Citation2021). In the aforementioned study there were no significant differences in running impact loading. However, this study was limited to a small sample resulting in a very heterogeneous group of preschoolers (from a developmental perspective), and with the absence of non-standardised footwear conditions and joint kinematics at the footstrike.
Previous review papers suggested that minimalist shoes may be optimal for foot development in very young children (Davis et al., Citation2021),(Davis et al., Citation2022), including even toddlers (Krabak et al., Citation2019). These suggestions were made based on transferring research evidence from adult populations. Believing that starting toddlers, children, and young runners in minimalist shoes would likely use a forefoot strike pattern which would be helpful for greater foot and ankle strength along with a reduction of impact loading that could reduce the risk of future running-related injuries (Davis et al., Citation2021),(Davis et al., Citation2022),(Krabak et al., Citation2019). However, the authors also acknowledged that there is a research gap on minimalist footwear in this population (Davis et al., Citation2021),(Krabak et al., Citation2021)
Previous randomised crossover studies (Plesek et al., Citation2021),(Plesek et al., Citation2022) showed that preschool children adapted their footstrike pattern differently according to changed footwear conditions such as barefoot, minimalist, and standard running shoes (Plesek et al., Citation2021) and also that they have different impact loading depending on the footwear in specific preschool years (Plesek et al., Citation2022). Yet, there were some similarities observed between 3- and 4 year-olds (e.g., rearfoot-midfoot strike with less plantarflexed ankle in all footwear conditions; and high impact loading in minimalist shoes compared to barefoot), and 5- and 6 year-old children (e.g., midfoot-forefoot strike with more plantarflexed ankle in barefoot and minimalist shoes; and no differences in impact loading). However, to the best of our knowledge, there is no study in the literature comparing running impact loading between younger and older preschoolers and/or other age groups in different footwear conditions. Thus, it seems to be meaningful to look at and/or compare possible age-related differences or similarities in barefoot and shod conditions (e.g., minimalist and standard running shoes) between younger preschool children and their older counterparts and physically young adult people which represent a mature running skill technique. Understanding of biomechanical age-related differences during the stance phase could potentially establish age norms from which future interventional studies in populations of children can be based.
Therefore, the purpose of this study was to compare running impact loading in two groups of preschool children and one group of young adults in barefoot condition (B), minimalist (M) and standard running shoes (SRS). In our previous work, we observed that 5–6 year-old children were able to change their footstrike pattern according to footwear conditions and 3–4 year-olds were not (Plesek et al., Citation2021). This information led us to hypothesise that 3–4 year-old children would have higher impact loading than 5–6 year-old children and young adults. In addition, 5–6 year-olds would not differ from young adults because it has been shown that most children reach mastery in running skill before 6 years of life (Hardy et al., Citation2010)
Methods
Study design and study sample size
An a priori sample size estimation was calculated in G*Power (G*Power 3.1.9.7, Düsseldorf, Germany) for mixed two-way ANOVA (3 within – 3 between factor and interaction) with a minimal statistical power of 80% (alpha level 0.05). Based on key-dependent variables for this study, vertical instantaneous loading rate (VILR) (Plesek et al., Citation2022),(Rice et al., Citation2016),(Tam et al., Citation2016), and cadence (Wei et al., Citation2021),(McCarthy et al., Citation2015), footstrike index (Plesek et al., Citation2021), ankle angle at initial contact (IC) (Plesek et al., Citation2021),(Tam et al., Citation2016); knee angle at IC (McCarthy et al., Citation2015) were retrieved from previous studies and the calculation of the average effect size represented by η2 (η2 = 0.301 or Cohen´s f = 0.65) indicated that 21 participants (7 participant/group) would be sufficient to detect footwear-age differences and/or an interaction (3 x 3). However, we decided to use a more conservative approach to avoid an underpowered sample size for this study. Therefore, to detect more reliably statistical differences we used a boundary value of a large effect size (η2 = 0.140, f = 0.4). It was found that a total of 45 participants (15 participants/age group) would be sufficient.
Participants
This is a further analysis of the research project The Biomechanics of Running in Preschool Children which includes two previous papers (Plesek et al., Citation2021),(Plesek et al., Citation2022), where 48 preschool children in four age groups (12 children/age group) participated. We additionally recruited 15 young physically active adults aged 20–25 years and randomly selected 15 from 24 children aged 3–4 years and 15 from 24 children aged 5–6 years using a draw (lottery). The draw was counterbalanced to ensure the same number of males and females in each age group (8 males/7 females). The protocol of this study was approved by the Ethical Board of the University of Ostrava, Faculty of Education (protocol ID: OU-54483/45-2019) and was conducted in accordance with the principles of the Declaration of Helsinki. All participants (children´s parents or legal guardians) of the current study signed and granted informed consent.
The total sample of 45 participants included 30 typically developing habitually shod children and 15 habitually shod young adult non-runners (undergraduate students of physical education and sports sciences at the University of Ostrava). All adults were physically active and had successfully undergone a standardised testing battery of aerobic fitness and sport skill competencies required for the Physical Education teacher training study programme. All of the participants in this study were injury free and had no lower limb deformities (e.g., flatfoot, leg length discrepancy, genu varum, and genu valgum). They also had no previous experience of wearing minimalist shoes.
Additionally, parents or legal guardians returned a Barefoot Questionnaire (BFQ) assessing how often their children used the footwear in two weather conditions (cold, warm) and three environments (educational institution, sports, in and around the house). This BFQ was adapted for preschool children and previously described more in detail by Plesek and his colleagues (Plesek et al., Citation2021). Furthermore, Early Years Physical Activity Questionnaire (EY-PAQ) was also completed by the children´s parents. The above-mentioned questionnaire evaluated the amount of the average daily sedentary and physically moderate or vigorous active time during a typical week in the last month before biomechanical data collection. Finally, children completed the Movement Assessment Battery for Children Second Edition (MABC-2), which was carried out to reveal possible developmental coordination delay or disorders in children. As an inclusion criterion, the limit for this study was set over the 15th percentile for a certain age according to Czech norms. From the motor developmental perspective, the 15th percentile is considered as a limit for typically developing children for a given age.
Experimental set up
The biomechanics laboratory was equipped with a motion capture system. A 17-metre-long runway with a flat, hard, and non-slippery surface was contained within the laboratory. The motion capture system consisted of three embedded force platforms (Kistler 9286AA, 9281CA, and 9287CCAQ02, Kistler Instruments AG, Winterthur, Switzerland) which were surrounded by ten high-speed optoelectronic cameras (Oqus, Qualisys, Inc., Gothenburg, Sweden). All cameras were focused on the force platforms which were located approximately in the middle of the runway (as in ). Kinematic and kinetic data were synchronously collected by the three-dimensional motion capture system with the sampling frequency of 240 Hz (cameras) and 1200 Hz (force platforms).
Figure 1. Laboratory and protocol setting. Random counter balanced order of footwear conditions (six possible combination). Footwear conditions (barefoot, minimalist and standard running shoes) and foot marker placement. Abbreviation: B – barefoot (green), M – minimalist shoes (blue), SRS – standard running shoes (red).
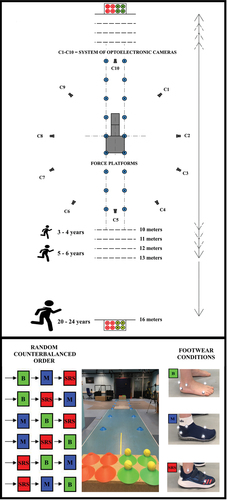
Anthropometric data (body height and body mass) of all participants were measured by a stadiometer (In Body 370, Biospace, Seoul, South Korea) and body composition analyser (Inbody 770, Biospace, Seoul, South Korea), respectively. Body mass index (BMI) for children and adults was calculated.
Protocol and Setting
All participants visited the Human Motion Diagnostic Centre at University of Ostrava. Parents or legal guardians chose shoe size fit for the children before the start of data collection (sizes ranged from 24 to 35 EU, from 8 K to 3.5 US). Adult participants also chose their laboratory shoes for data collection (sizes ranged from 38 to 44 EU, from 6 to 10.5 US). The shoes were assessed according to the minimalist index (MI;Esculier et al., Citation2015) which ranges from 0% (least minimalist) to 100% (most minimalist). The laboratory intervention shoes consisted of true minimalist shoes (M; Leguanito, Leguano, Buchholz, Germany; with MI 96%) and standard running shoes (SRS; FortaRun CF I and K, Adidas, Herzogenaurach, Germany; with MI 30%;). Shoes SRS for adults (DB1090, Alphabounce em m, Adidas, Herzogenaurach, Germany, with MI 26%). Although we could not use the same SRS for adults and children, we chose the most similar SRS to children´s SRS available from the same brand, and MI was very similar (26%) compared to children´s SRS (30%).
Marker placement was carried out by an experienced researcher in 3D movement analysis. Twenty-four retroreflective markers (6.4-mm diameter) were positioned on each participant´s specific anatomical points (18 right limb, 4 on the pelvis, 1 on the left greater trochanter and 1 left heel). Marker placement was carried out in line with the recommendations of Visual 3D software (C-motion, Germantown, MD, USA). Two calibration markers on the right foot/shoe were placed over the first and fifth metatarsal heads and three tracking markers were attached in a triangle on the heel over the bare calcaneus or intact shoe ().
Participants performed a simple running game (back and forth at a submaximal self-selected speed running between two posts) in barefoot, minimalist and standard running shoe conditions. The order of footwear conditions was randomly counterbalanced in each age group (see, ). The running game consisted of relocating balls at each end of the runway and a shuttle run between the ends. The game was invented with the purpose to maintain the external focus attention and motivation of the children. This was described more in detail previously (Plesek et al., Citation2021),(Plesek et al., Citation2022), including information about instructions for children such as “Run comfortably straight in the middle of the blue cones back and forth” corresponding to submaximal running pace. The protocol included periods of rest between running games (at least 3 minutes) and footwear conditions (approximately 10–15 minutes). Between each running game (at the end of a 3-minute-long break), an investigator asked the children: “Do you feel tired?” If the answer was “No, I do not”, then the next stage was started. Each stage was started with the instruction “Ready, set, go!”
The distances on the runway for each age group were set up based on the leg length with distances increasing by 6–8% for each age group (Fortney, Citation1983). Therefore, children completed shuttle run with a distance of approximately 10, 11, 12, and 13 m from stage to stage according to their age. Six-year-old children ran in total 780 metres including familiarisation (1st footwear condition: 52 metres during familiarisation, 104 metres during 1st stage – 3 minutes break – 104 metres during 2nd stage – 15 minutes break; 2nd footwear condition: familiarisation, 1st stage – 3 minutes break – 2nd stage – 15 minutes break; 3rd footwear condition: familiarisation, 1st stage – 3 minutes break – 2nd stage – end). Five-year-old children ran 720 metres, four-year-old 660 metres, and three-year-old 600 metres. Adjustments of the running distance per age and breaks were involved with the purpose to avoid possible fatigue which has been shown to influence running patterns (Latorre-Román et al., Citation2016),(Jewell et al., Citation2017). Adult participants also carried out the same procedure as children did. However, limited space in the laboratory allowed run back and forth at a distance of 16–17 metres as a maximum. Therefore, the distance of 16 metres was determined for young adults (total distance = 960 metres). Adults were asked also to run at their self-selected comfortable speed.
Data processing
Raw biomechanical data were processed by using QTM (Qualisys). Further data processing was carried out in Visual 3D software (C-motion). The stance phase was determined when the resultant ground reaction force was above a 15 N threshold. Six successful trials were selected and analysed based on pelvis velocity (closest trials to the median pelvic velocity). A successful running trial was identified when the entire right foot contacted the force platform. A low–pass Butterworth filter using a cut-off frequency of 10 Hz was applied for motion and 50 Hz for force data.
The main dependent variable in this study was the vertical instantaneous loading rate (VILR). As secondary outcome variables, we assessed cadence, footstrike index, ankle angle, knee angle, and hip angle at footstrike. In addition, we also analysed pelvis velocity and normalised speed (Froude number) as control variables. The VILR was determined by calculating the first derivative of the corresponding vertical ground reaction force (VGRF) with respect to time. The maximum (peak) VILR value was obtained from the first 27% of stance as a local maximum using the same approach as in Rice et al. (Rice et al., Citation2016). Footstrike index was analysed based on the location of the centre of pressure (COP) at the initial foot/shoe-ground contact, where the location of the COP at initial contact along the longitudinal axis of the foot/shoe coordinate system, is normalised by the foot/shoe length measured from the heel and multiplied by 100 (Altman & Davis, Citation2012). For footstrike index, three regions were displayed: rearfoot strike (RFS) 0–33%; midfoot strike (MFS) 33–67% and forefoot strike (FFS) 67–100%.
Joint angles were modelled in Visual 3D and calculated using an x-y-z Cardan rotation sequence. Ankle angles were determined as the relative position of the foot to the shank, knee angles as the relative position of the shank to the thigh, and hip angles as the relative position of the thigh to the pelvis during the first initial foot contact with a force plate. VILR was normalised to the body mass of each participant. To avoid the effects of running speed on the aforementioned dependent variables, we calculated a dimensionless speed known as Froude number (see following equation) based on pelvic horizontal velocity (v) and lower extremity length (lo) and we use these variables as control variables for this study (Alexander, Citation1989)
dimensionless speed (Froude Number) = v2/glo (1)
Statistical analysis
An independent sample t-test was used to determine differences in age, height, mass, BMI, MABC-2, BFQ, EY-PAQ sedentary time, moderate to vigorous physical activity time, and vigorous physical activity time between two age groups of preschool children. A two-way mixed ANOVA (3 x 3) was performed on the VILR; cadence; pelvis velocity; Froude number; and lower limb kinematics at IC (ankle, knee, and hip angle). If Mauchly’s sphericity tests were found to be significant and ε ≥ 0.75, then Huynt–Feldt correction was applied. If a significant interaction was found, then one-way repeated measures ANOVA was used to compare mean values among three footwear conditions (i.e., within factor: B – M – SRS) followed by post hoc with LSD adjustment for pairwise comparisons of three treatments (three footwear conditions) as recommended by Meier (Meier, Citation2006), in each age group separately. In addition, a one-way omnibus ANOVA was used to test differences among three age groups (i.e., between factor: young preschoolers – older preschoolers – adults) in all three footwear conditions separately. The post hoc test for multiple pairwise comparisons between age groups used a Bonferroni correction. All analyses were completed in SPSS 24 (IBM, Armonk, NY, USA). An alpha level of significance was set at 0.05 for all statistical tests.
Effect size was calculated for the main effect in the one-way repeated measures ANOVA and one-way omnibus ANOVA was weighed using η2 (small (0.01–0.06), medium (0.07–0.14), and large (>0.14)) and Cohen´s d (small (0.2–0.5), medium (0.5–0.8), and large (>0.8)) in pairwise comparisons of footwear and age group differences. We implemented a Cohen´s d as proposed by Sawilowski (Sawilowsky, Citation2009) to a very small 0.01–0.20 and very large >1.2 effect.
Results
There were differences between two groups of preschool children in age, height, body mass and lower leg length (p = 0.001; p = 0.001; p = 0.001; p = 0.001), respectively. No differences were found in BMI (d = 0.09), BFQ (d = 0.41), MABC-2 (d = 0.42), moderate to vigorous physical activity (d = 0.45), sedentary time (d = 0.59), between 3–4 and 5–6 year-old children. However, there was a higher level of vigorous physical activity with a moderate-large effect for older children (p = 0.015, d = 0.77; ).
Table 1. Participants Characteristics.
Vertical instantaneous loading rate and spatiotemporal variables
The interaction between footwear and age groups in VILR can be observed in and .
Table 2. Two-way mixed ANOVA (3x3). Tests of within – subjects and between-subjects effect. N = 45 participants (15 participants in each group (8 Males/ 7 Females)).
Figure 2. Impact loading, spatiotemporal and kinematics variables. A) Vertical instantaneous loading rate (VILR); B) cadence; C) speed; D) dimensionless speed; E) footstrike index; F) ankle angle; G) knee angle; H) Hip angle. Data are presented as mean and standard deviation. Statistically significant differences between age groups are indicated as *B (for barefoot), *M (for minimalist shoes), *SRS (standard running shoes), and *all (for all footwear conditions). The lines with arrows connect relevant age groups where significant differences appeared. Statistically significant differences between footwear conditions are reported only in the text. Abbreviation: B – barefoot (green), M – minimalist shoes (blue), SRS – standard running shoes (red).
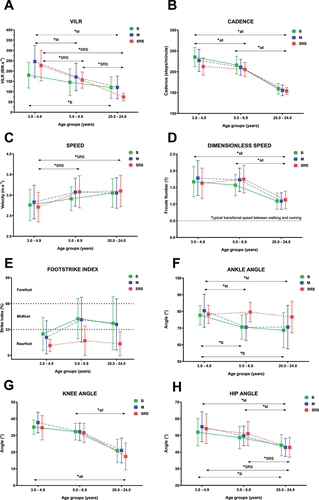
Further analysis showed that significantly lower VILR emerged in younger children when they ran barefoot in comparison with both shod conditions (M: p = 0.011, d = 0.92; SRS: p = 0.032, d = 0.67). Older children also ran with lower VILR in the barefoot condition but none of these differences were statistically significant. On the contrary, the lowest VILR in adults was interestingly observed in SRS compared to barefoot (p = 0.006, d = 1.18) and M (p = 0.006, d = 1.13).
A one-way ANOVA was performed to determine age-related differences. A decreasing trend was apparent in VILR in all three footwear conditions (B: p = 0.031; M: p = 0.001; and SRS: p = 0.001). In the B, there were significant differences only between younger preschoolers and adults (p = 0.027, d = 1.04). In the M, there were significances between younger and older preschoolers (p = 0.012, d = 1.02) and also younger and adults (p = 0.001, d = 1.85). No differences were found between the older children (5–6 year-olds) and adults in both the B and M. The greatest reduction of VILR was confirmed in the SRS among all age groups with linearly decreasing average impact loading (younger-older: p = 0.001, d = 1.18; younger-adults: p = 0.001, d = 2.82; and older-adults: p = 0.001, d = 2.68).
For cadence, there was no significant footwear-age interaction. Although significant differences for the main effect of footwear condition were found across age, the post hoc analysis showed differences with small or very small effects between certain footwear conditions (B-M, (p = 0.029, d = 0.13), B-SRS (p = 0.001, d = 0.37), M-SRS (p = 0.001, d = 0.21)). However, a large main effect of age-related differences was confirmed for cadence. As in , children had significantly higher cadence than adults. Both children´s groups displayed differences compared to adults, when younger-adults (p = 0.001, d = 3.80) and older-adults (p = 0.001, d = 3.54). In addition, there were moderate differences between younger and older children (p = 0.019, d = 0.678).
There were significant differences in speed and normalised speed. An interaction was found in pelvis velocity and Froude number. As expected due to differences in lower limb length between age groups, children had a lower speed than adults. However, statistical differences were confirmed only in the SRS (younger-older: p = 0.035; younger-adults: p = 0.024; older-adults: p = 1.000). On the other hand, children had higher normalised speed than adults in all three footwear conditions (p < 0.05), but normalised speed did not differ between younger and older children (p = 1.000). In terms of footwear-related differences in the specific age groups, the group of older children (5–6 year-olds) ran significantly slower barefoot than in shod conditions. No effect of footwear was observed in young children (p = 0.173) or adults (p = 0.771).
Footstrike index and joint angles at footstrike
Footstrike index and sagittal plane joint kinematics at footstrike (ankle, knee, and hip angles at IC) are presented in . No interaction was found for the footstrike index () nor the main effect of age. However, there was a significant effect among footwear conditions (p = 0.001, η2 = 0.392). A post hoc pairwise comparison showed significant differences between the B and SRS with large ES (p = 0.001, d = 1.02), and also between the M and SRS (p = 0.001, d = 0.89), where the footstrike index was lower in the SRS (rearfoot strike). No significant differences were found between B and M resulting in a very small effect size (p = 0.307, d = 0.09).
There was a significant interaction for the ankle angles at initial contact (footwear condition and age group) (p = 0.005, η2 = 0.159). No footwear differences were found in the younger preschoolers (3- and 4-year-olds) (P > 0.05). Large effects of footwear were observed in older children (p = 0.001, η2 = 0.473) and adults (p = 0.001, η2 = 0.425). Further pairwise comparisons of footwear showed statistical differences in ankle angle with very large ES for older preschool children and a large effect for adults between B and SRS (p = 0.002, d = 1.37; p = 0.001, d = 0.80). Five-six year-old children and adults had a more plantarflexed ankle when running barefoot compared to the SRS. In addition, very similar trend was also observed between the M and SRS in older children (p = 0.001, d = 1.29) and medium effect in adults (p = 0.007, d = 0.54). However, no differences were found between the B and M (). Moreover, the pairwise comparison with Bonferroni correction for multiple comparisons between age groups showed that older preschoolers and adults had more plantarflexed ankle than younger preschool children when they ran barefoot (p = 0.061, d = 1.08; p = 0.013, d = 1.06 respectively). The same trend was confirmed for the M, ankle angles differed with large effect between younger preschoolers in comparison with their older counterparts and adults (p = 0.039, d = 1.10; p = 0.042, d = 0.84). No age differences were found in SRS.
For knee angles, no interaction was found (). There was a main effect for footwear () and also a main effect for the age groups. All participants had less flexed knee at footstrike when they run in the SRS compared to the M (p = 0.001, d = 0.25) or B (p = 0.042, d = 0.16). However, the effect sizes of the differences were small or very small. In addition, the post hoc analysis for the age groups indicated that all children had greater knee flexion angles compared to adults (p = 0.001, d = 2.40; p = 0.001, d = 1.91) with very large effect sizes. No significant differences in knee angles were found between younger and older preschoolers (p = 0.084) but with a moderate effect of lower knee flexion angle in older children (d = 0.68).
A significant interaction between footwear and age was also found for the hip angle (see, ). However, a one-way repeated measures ANOVA did not show any differences for footwear in any age group. A one-way ANOVA showed significant age differences among age groups in all footwear conditions (B: p = 0.016; M: p = 0.001; and SRS: p = 0.001) where adults generally displayed a less flexed hip joint at footstrike than the children. When participants ran barefoot, there was a significant difference only between the younger children and adults (p = 0.013, d = 1.07). In addition, there was a significant difference between younger or older children compared adults in both shod condition (M: younger-adults (p = 0.001, d = 1.67), older-adults (p = 0.029, d = 1.22); SRS: younger-adults (p = 0.001, d = 1.48), older-adults (p = 0.013, d = 1.25)). No differences were observed for hip between younger and older preschoolers regardless of footwear used (see, ).
Discussion
It has been suggested that there is a lack of scientific information about the possible differences in running kinetics at footstrike between different age groups (children, adolescents, adults). Therefore, the main research question of this study was: does running impact loading change according to age? The aim of this study was to compare lower limb kinematics at foot strike and running impact loading for two age children’s age groups and young adults in barefoot, minimalist and standard running shoe conditions. We hypothesised that 3–4 year-olds would have greater impact loading than 5–6 year-olds and young adults. In addition, 5–6 year-old children would not differ from young adults. Our hypothesis was partially confirmed, when younger children (3–4 year-olds) displayed higher impact forces than older children (5–6 year-olds) in the shod conditions (M and SRS). However, the results of the current study showed that running impact loading significantly decreased with age in all footwear conditions until young adulthood (B, M, SRS).
Relationships and comparisons of spatiotemporal parameters (e.g., step length, velocity, and cadence), lower limb kinematics at footstrike (knee angle, ankle angle, foot angle), and also various types of footwear and their effects on running impact loading (ground reaction forces) have been studied in various populations (Hollander et al., Citation2014),(Plesek et al., Citation2021),(Plesek et al., Citation2022),(Tam et al., Citation2016),(Cheung et al., Citation2017),(Hollander et al., Citation2018),(Wille et al., Citation2014),(Hobara et al., Citation2012),(Johnson & Davis, Citation2022). Some studies showed that increased cadence (Hobara et al., Citation2012) and lower velocity could decrease running impact loading in adults (Keller et al., Citation1996). However, the velocity and cadence would not adequately explain this phenomenon of decreasing impact loading trend in the current study. As would be expected, decreasing cadence while increasing absolute velocity should lead to a reverse result to that we observed in the current study. Moreover, there were also no differences between the younger and older children´s groups in relative running speed, although the impact loading decreased significantly. Therefore, we had to take a closer look at footstrike kinematics, and body alignment or discuss the influence of ontogenetic differences (possible growth-related differences) to explain the study outcomes.
From a running footstrike biomechanical point of view, we can explain lower impact forces in older children or adults compared to younger children during barefoot running or in minimalist shoes by the type of footstrike itself and the ankle angle’s relative position at the initial foot-ground contact. Five to six year-old children and adults generally display a midfoot strike with a more plantar flexed ankle as have been shown in the current and also previous study (Rice et al., Citation2016). However, data from this study showed a significant decline in loading in the SRS condition with increasing age although the footstrike or ankle angle at initial contact did not change (i.e., remain rearfoot). A recent study (Johnson & Davis, Citation2022) indicated that rearfoot strikes in adult runners with a very low loading rate had a significantly less flexed knee and hip angle at initial contact compared to rearfoot strikes with very high loading rate. This alignment of the lower limb at footstrike allowed runners from the abovementioned study to have a greater knee angle excursion during the absorption phase resulting in lower knee stiffness. According to our study, it appears that our 3–4 year-old preschoolers may not have fully used knee excursion during impact attenuation due to more flexed knee angle at footstrike (impact).
Due to a lack of studies investigating impact loading in children during running, it is difficult to compare loading rate values with previous research. However, Wei et al. (Citation2021) compared shod running biomechanics (including VILR) between preschool children (3–6 years, mean age = 4.2 years, SD = 1.6) and young adults (mean age = 35.1, SD = 9.5). Their preschoolers displayed VILR about 63.1 BW/s (SD = 11.8) and adults 59.4 BW/s (SD = 12.9). These authors did not find any differences in vertical instantaneous loading rate (VILR) during submaximal speed running between preschoolers and adults in their usual shod condition. However, they used a different method of evaluation of impact loading originally developed for adults. Values of VILR in SRS of the current study were about 190 BW/s which is approximately three-fold greater compared to the preschoolers of Wei et al. (Wei et al., Citation2021). Some authors suggested that 70–75% of children between 4.0–4.9 years of age reached the mastery level in running skill (a qualitative assessment) (Hardy et al., Citation2010); however, our data showed that, from the impact loading perspective, they did not reach a mastery level of running. Although this study used only cross-sectional data for age-related changes and not longitudinal study design data, there is an obvious trend of decreasing loading, particularly in shod conditions (3–4 years old: 226.5 ± 74.0 BW/s; 5–6 years old: 156.4 ± 39.4 BW/s, and adults: 74.3 ± 17.8 BW/s).
From an evolutionary body and running skill development perspective, the Achilles tendon is a very young structure that probably developed for endurance running. Running efficiency has significantly improved with Achilles tendon elongation and the appearance of the longitudinal arch of the foot because these elastic structures play an energy-saving role during running (Alexander, Citation1989),(Bramble & Lieberman, Citation2004),(Malvankar & Khan, Citation2011). If we assume that ontogeny copies phylogeny then the development of running, including changes of footstrike patterns during preschool age, may relate to significant growth of the Achilles tendon and building of the medial longitudinal foot arch in that age (Plesek et al., Citation2021),(Bérnard et al., Citation2011),(Forriol & Pascual, Citation1990). The medial longitudinal arch starts to develop between the second and third years of age and systematically increases in height until school age (Uden et al., Citation2017). It seems that the growth of the medial longitudinal arch and Achilles tendon may develop in parallel to running skill development. Likewise, several authors attribute running development to those structures also from a phylogeny perspective (Bramble & Lieberman, Citation2004),(Malvankar & Khan, Citation2011). Echarri & Forriol (Echarri & Forriol, Citation2003) showed that age is a primary predictive factor of a flat foot rather than wearing footwear. They found that the prevalence of flat foot in habitually barefoot and shod children decreases with age, e.g., in 3–4 year-old children prevalence of flat foot was around 70–80% but in 5–8 years-old the prevalence of flat foot was almost half than in younger children (about 35–50%). In addition, it is well known that longer muscle fascicle of the gastrocnemius and higher Achilles tendon stiffness are required for efficient running than during walking (Lichtwark & Wilson, Citation2008). It has been shown that the length of the gastrocnemius fascicle and Achilles tendon increases by 5% and 6% per year from 5 to 12 years, respectively (Bérnard et al., Citation2011). Additionally, muscle-tendon units during growth and maturation become stiffer if the appropriate levels of mechanical loading are applied (Bérnard et al., Citation2011),(Buchanan & Marsh, Citation2001). This study confirmed that 5–6 year-old children engaged more in physical activity/active play, which makes them sweat or breathe harder during vigorous physical activity including running and jumping activities than 3–4 year-old preschoolers. This seems to be in line with a previous study which showed increasing vigorous activity with age during the preschool period (Downing et al., Citation2021) even though differences in the total amount of active time (moderate to vigorous physical activity) between younger and older children in the current study showed differences with only small-moderate effect. Thus, the different types of physical activity and not sufficiently developed musculoskeletal system of 3–4 year-old children may limit their ability to the diminution of impact loading.
Perspective for using minimalist shoes during running in preschool age
Recently, publications have emerged that recommended minimalist footwear when children start to run, with the hope of reducing future running-related injuries in these individuals (Davis et al., Citation2021),(Davis et al., Citation2022),(Krabak et al., Citation2019). However, this and our previous research (Plesek et al., Citation2022) showed that the acute loading at 3–4 years of age is relatively very high in minimalist shoes, and combined with heel striking, could overload the calcaneus (and possibly the knee joint in the frontal plane), at least during the adaptation period. On the contrary, higher loading could potentially contribute to the strengthening of the foot bones (Walther et al., Citation2008). However, given that VILR values in 3-4-year-old children were 2 times higher than in adults and 5 times higher compared to the study by Rice et al. (Rice et al., Citation2016) we should be more cautious when considering minimalist footwear instead of barefoot condition (as an alternative to barefoot). Furthermore, some authors discussed the higher impact forces during locomotion (walking and running) on hard surfaces (Plesek et al., Citation2022),(Zeininger et al., Citation2018) regarding possible occult calcaneal injuries previously observed in toddlers (Laliotis et al., Citation1993),(Starshak et al., Citation1984). The results of the current study suggest that probably a more appropriate period for the use of minimalist footwear may be at approximately 5–6 years of age at the earliest when the impact load decreases by more than 30% (compared to younger preschoolers) and children are usually at a more advanced stage of running skill (already able to run with a forefoot strike and less flexed knee). In addition, at the age of 5–6 years, children have more developed foot, Achilles tendon, and calf structures due to ontogeny or already physiologically corrected genu valgum (Rao & Joseph, Citation1992),(Bérnard et al., Citation2011),(Forriol & Pascual, Citation1990),(Echarri & Forriol, Citation2003). However, we suppose that ontogenetic musculoskeletal constraints for loading attenuation in 3–4 years old children could be alleviated only via barefoot running due to direct foot contact with the ground leading to better proprioception.
Strength and Limitations
This is the first study comparing age-related differences in impact loading in three different footwear conditions (particularly recently very often discussed minimalist shoes) during a crucial running developmental period. We used a sufficient sample size (15 per age group) and we included into the analysis a random sample of participants (randomised counterbalanced order for age and sex). We assessed the motor developmental level of the participants via motor assessment battery. Lastly, we designed a movement task for this study that ensured the motivation of children was high during testing. Concurrently, running distance covered by participants respected body composition differences in specific age (distance adjusted by average lower limb length). However, the size of the laboratory set the maximum runway length at 16 m for the adult runners and this may have affected their relative speed (Froude number), which appeared to be lower than that of the preschoolers.
This study was however limited by the nature of the cross-sectional design. Additionally, we were not able to measure the structural properties of muscle-tendon units (calf and foot structural properties) using ultrasonography or magnetic resonance due to a time-consuming procedure that required attention and calm during measurement which seems to be quite problematic at that very young age group. In terms of footwear used in this study, we did not have the same type of SRS for adults and children. However, we chose the most similar SRS to children´s SRS available from the same brand in the market, and the minimalist index was very similar (26%) compared to children´s SRS (30%). Lastly, we did not include younger children than 3 year-old children (when first running attempts usually appear), because such young children were not able to accomplish the movement task during piloting stage of this research project. Therefore, we cannot generalise the results of this study to the running toddlers (1.5–3.0 aged) and either school-aged children or adolescents.
Future investigations should focus on the long-term effects of footwear on running mechanics in preschool children. An evaluation of the possible relationship between impact loading variables and foot development (e.g., arch index, bone mineral density of the foot, cross-sectional area of the intrinsic muscles of the foot) seems worthwhile. The next step would be to recruit and longitudinally measure the children of parents who themselves have chosen to wear minimalist footwear for their children and compare these children with children who have been conventionally shod (or with habitually barefoot children from less developed countries).
Conclusion
This study found that running impact loading generally decreased during the preschool age period. This trend of decreased loading probably continues until young adulthood regardless of footwear conditions. However, a lesser decline of loading according to age was observed in the barefoot condition. When considering footwear for preschool children and its acute effects, it seems that only barefoot running is the most beneficial in terms of loading for 3–4 year-olds. The time of full and load-safe use of any footwear (including minimalist shoes with high minimalist index) for the introduction of running activities on hard surfaces probably begins at age 5–6 years and continues into adulthood.
Author contributions
JP, JH, JFS and DJ created design of the study; JP carried out the measurements and data collection; JP, JS, DJ performed data processing and data analysis; JP and DJ participated in the biomechanical analysis; JP and JS performed the statistical analysis, JP wrote first draft of the manuscript; JH, JFS and DJ reviewed the manuscript and wrote some specific parts of the manuscript. All authors have read and approved the final version of the manuscript, and agree with the order of presentation of the authors.
Acknowledgments
We appreciate all of the participants in this study. We would also like to thank Marek Pavelka for his help with creating of figure design (laboratory diagram). This work was supported by Czech Ministry of Education, Youth and Sport and European Union structural funds under the grant “Healthy Aging in Industrial Environment” (program 4 HAIE CZ.02.1.01/0.0/0.0/16_019/0000798) and student’s grant (SGS14/PdF/2017-18), internal funding of the Faculty of Education at University of Ostrava.
Disclosure statement
The authors report there are no competing interests to declare.
Correction Statement
This article has been republished with minor changes. These changes do not impact the academic content of the article.
Additional information
Funding
References
- Alexander, R. (1989). Optimization and Gaits in the Locomotion of Vertebrates. Physiol Rev, 69(4), 1199–1227. https://doi.org/10.1152/physrev.1989.69.4.1199
- Altman, A. R., & Davis, I. S. (2012). A kinematic method for footstrike pattern detection in barefoot and shod runners. Gait Posture, 35(2), 298–300. https://doi.org/10.1016/j.gaitpost.2011.09.104
- Bérnard, M. R., Harlaar, J., Becher, J. G., Huijing, P. A., & Jaspers, R. T. (2011). Effects of growth on geometry of gastrocnemius muscle in children: A three-dimensional ultrasound analysis. J Anat, 219(3), 388–402. https://doi.org/10.1111/j.1469-7580.2011.01402.x
- Borghese, M. M., & Janssen, I. (2019). Duration and intensity of different types of physical activity among children aged 10–13 years. Can J Public Heal, 110(2), 178–186. https://doi.org/10.17269/s41997-018-0157-z
- Bramble, D. M., & Lieberman, D. E. (2004). Endurance running and the evolution of Homo. Nature, 432(7015), 345–352. https://doi.org/10.1038/nature03052
- Buchanan, C. I., & Marsh, R. L. (2001). Effects of long-term exercise on the biomechanical properties of the Achilles tendon of Guinea fowl. J Appl Physiol, 90(1), 164–171. https://doi.org/10.1152/jappl.2001.90.1.164
- Cheung, R. T. H., Wong, R. Y. L., Chung, T. K. W., Choi, R. T., Leung, W. W. Y., & Shek, D. H. Y. (2017). Relationship between foot strike pattern, running speed, and footwear condition in recreational distance runners. Sport Biomech, 16(2), 238–247. https://doi.org/10.1080/14763141.2016.1226381
- Davis, I. S., Hollander, K., Lieberman, D. E., Ridge, S. T., Sacco, I. C. N., & Wearing, S. C. (2021). Stepping Back to Minimal Footwear: Applications Across the Lifespan. Exerc Sport Sci Rev, 49(4), 228–243. https://doi.org/10.1249/JES.0000000000000263
- Davis, I. S., wei, C. T. L., & Wearing, S. C. (2022). Reversing the Mismatch With Forefoot Striking to Reduce Running Injuries. Front Sport Act Living, 4, 1–9. https://doi.org/10.3389/fspor.2022.794005
- Downing, K. L., Hinkley, T., Timperio, A., Salmon, J., Carver, A., Cliff, D. P., Okely, A. D., & Hesketh, K. D. (2021). Volume and accumulation patterns of physical activity and sedentary time: Longitudinal changes and tracking from early to late childhood. Int J Behav Nutr Phys Act, 18(1), 1–11. https://doi.org/10.1186/s12966-021-01105-y
- Echarri, J. J., & Forriol, F. (2003). The development in footprint morphology in 1851 Congolese children from urban and rural areas, and the relationship between this and wearing shoes. J Pediatr Orthop Part B, 12, 141–146. doi:10.1097/01.bpb.0000049569.52224.57. https://journals.lww.com/jpo-b/Abstract/2003/03000/The_development_in_footprint_morphology_in_1851.12.aspx
- Esculier, J. F., Dubois, B., Dionne, C. E., Leblond, J., Roy, . J. S. . (2015). A consensus definition and rating scale for minimalist shoes. J Foot Ankle Res, 8(1), 1–9. https://doi.org/10.1186/s13047-015-0094-5
- Forriol, F., & Pascual, J. (1990). Footprint Analysis Between Three and Seventeen Years of Age. Foot Ankle Int, 11(2), 101–104. https://doi.org/10.1177/107110079001100208
- Fortney, V. L. (1983). Kinematics and kinetics of the running pattern of two-,four-, and six-year-old children. Res Q Exerc Sport, 54(2), 126–135. https://doi.org/10.1080/02701367.1983.10605284
- Fukuchi, C. A., Fukuchi, R. K., & Duarte, M. (2019). Effects of walking speed on gait biomechanics in healthy participants: A systematic review and meta-analysis. Syst Rev, 8(1), 1–11. https://doi.org/10.1186/s13643-019-1063-z
- Hardy, L. L., King, L., Farrell, L., Macniven, R., & Howlett, S. (2010). Fundamental movement skills among Australian preschool children. J Sci Med Sport, 13(5), 503–508. https://doi.org/10.1016/j.jsams.2009.05.010
- Hobara, H., Sato, T., Sakaguchi, M., Sato, T., Nakazawa, K., & Functions, M. (2012). Step Frequency and Lower Extremity Loading During Running. Int J Sport Med, 33(4), 310–313. https://doi.org/10.1055/s-0031-1291232
- Hollander, K., De, V. J. E., Venter, R., Sehner, S., Wegscheider, K., Zech, A., Medicine, E., Africa, S., Physiology, E., & Medicine, E. (2018). Foot Strike Patterns Differ Between Children and Adolescents Growing up Barefoot vs. Shod. Int J Sports Med, 39(2), 97–103. https://doi.org/10.1055/s-0043-120344
- Hollander, K., Riebe, D., Campe, S., Braumann, K. M., & Zech, A. (2014). Effects of footwear on treadmill running biomechanics in preadolescent children. Gait Posture, 40(3), 381–385. https://doi.org/10.1016/j.gaitpost.2014.05.006
- Jewell, C., Boyer, K. A., & Hamill, J. (2017). Do footfall patterns in forefoot runners change over an exhaustive run? J Sports Sci, 35(1), 74–80. https://doi.org/10.1080/02640414.2016.1156726
- Johnson, C. D., & Davis, I. S. (2022). What differentiates rearfoot strike runners with low and high vertical load rates? Gait Posture, 96, 149–153. https://doi.org/10.1016/j.gaitpost.2022.05.019
- Keller, T. S., Weisberger, A. M., Ray, J. L., Hasan, S. S., Shiavi, R. G., & Spenger, D. M. (1996). Relationship between vertical ground reaction force and speed during walking, slow jogging, and running. Clin Biomech, 11(5), 253–259. https://doi.org/10.1016/0268-0033(95)00068-2
- Krabak, B. J., Roberts, W. O., Tenforde, A. S., Ackerman, K. E., Adami, P. E., Baggish, A. L., Barrack, M., Cianca, J., Davis, I., D’Hemecourt, P., Fredericson, M., Goldman, J. T., Harrast, M. A., Heiderscheit, B. C., Hollander, K., Kraus, E., Luke, A., Miller, E., Moyer, M., … Wasfy, M. M. (2021). Youth running consensus statement: Minimising risk of injury and illness in youth runners. Br J Sports Med, 55(6), 305–318. https://doi.org/10.1136/bjsports-2020-102518
- Krabak, B. J., Tenforde, A. S., Davis, I. S., Fredericson, M., Harrast, M. A., D’Hemecourt, P., Luke, A. C., & Roberts, W. O. (2019). Youth Distance Running: Strategies for Training and Injury Reduction. Curr Sports Med Rep, 18(2), 53–59. https://doi.org/10.1249/JSR.0000000000000564
- Laliotis, N., Pennie, B. H., Carty, H., & Klenerman, L. (1993). Toddler’s fracture of the calcaneum. Injury, 24(3), 169–170. https://doi.org/10.1016/0020-1383(93)90284-D
- Latorre-Román, P. Á., Fernández-Sánchez, M., Moriana-Coronas, F. J., & García-Pinillos, F. (2016). Design and validation of a cardiorespiratory capacity test for preschool children. South African J Res Sport Phys Educ Recreat, 38, 129–137. https://hdl.handle.net/10520/EJC192943
- Lichtwark, G. A., & Wilson, A. M. (2008). Optimal muscle fascicle length and tendon stiffness for maximising gastrocnemius efficiency during human walking and running. J Theor Biol, 252(4), 662–673. https://doi.org/10.1016/j.jtbi.2008.01.018
- Malvankar, S., & Khan, W. S. (2011). Evolution of the Achilles tendon: The athlete’s Achilles heel? Foot, 21(4), 193–197. https://doi.org/10.1016/j.foot.2011.08.004
- McCarthy, C., Fleming, N., Donne, B., & Blanksby, B. (2015). Barefoot running and Hip kinematics: Good news for the knee? Med Sci Sports Exerc, 47(5), 1009–1016. https://doi.org/10.1249/MSS.0000000000000505
- Meier, U. (2006). A note on the power of Fisher’s least significant difference procedure. Pharm Stat, 5(4), 253–263. https://doi.org/10.1002/pst.210
- Mizushima, J., Keogh, J. W. L., Maeda, K., Shibata, A., Kaneko, J., Ohyama-Byun, K., & Ogata, M. (2021). Long-term effects of school barefoot running program on sprinting biomechanics in children: A case-control study. Gait Posture, 83, 9–14. https://doi.org/10.1016/j.gaitpost.2020.09.026
- Plesek, J., Freedman Silvernail, J., Hamill, J., & Jandacka, D. (2021). Running Footstrike Patterns and Footwear in Habitually Shod Preschool Children. Med Sci Sport Exerc, 53(8), 1630–1637. https://doi.org/10.1249/MSS.0000000000002629
- Plesek, J., Hamill, J., Blaschova, D., Freedman-Silvernail, J., & Jandacka, D. (2022). Acute effects of footwear on running impact loading in the preschool years. Sport Biomech, 00, 1–17. https://doi.org/10.1080/14763141.2022.2058599
- Quinlan, S., Sinclair, P., Hunt, A., & Yan, A. F. (2022). The long-term effects of wearing moderate minimalist shoes on a child’s foot strength, muscle structure and balance: A randomised controlled trial. Gait Posture, 92, 371–377. https://doi.org/10.1016/j.gaitpost.2021.12.009
- Rao, U. B., & Joseph, B. (1992). The Influence of Footwear on the Prevalence of Flat Foot: A Survey of 2300 Children. J Bone Jt Surg, 74(4), 525–527. https://doi.org/10.1302/0301-620X.74B4.1624509
- Rice, H. M., Jamison, S. T., & Davis, I. S. (2016). Footwear Matters: Influence of Footwear and Foot Strike on Load Rates during Running. Med Sci Sports Exerc, 48(12), 2462–2468. https://doi.org/10.1249/MSS.0000000000001030
- Sawilowsky, S. S. (2009). New Effect Size Rules of Thumb. J Mod Appl Stat Methods, 8(2), 597–599. https://doi.org/10.22237/jmasm/1257035100
- Starshak, R. J., Simons, G. W., & Sty, J. R. (1984). Occult fracture of the calcaneus - another toddler´s fracture. Pediatr Radiol, 14(1), 37–40. https://doi.org/10.1007/BF02386729
- Tam, N., Tucker, R., & Astephen Wilson, J. L. (2016). Individual Responses to a Barefoot Running Program. Am J Sports Med, 44(3), 777–784. https://doi.org/10.1177/0363546515620584
- Uden, H., Scharfbillig, R., & Causby, R. (2017). The typically developing paediatric foot: How flat should it be? A systematic review. J Foot Ankle Res, 10(1), 37. https://doi.org/10.1186/s13047-017-0218-1
- Walther, M., Herold, D., Sinderhauf, A., & Morrison, R. (2008). Children sport shoes-A systematic review of current literature. Foot Ankle Surg, 14(4), 180–189. https://doi.org/10.1016/j.fas.2008.04.001
- Wei, R. X. Y., Chan, Z. Y. S., Zhang, J. H. W., Shum, G. L., Chen, C. Y., & Cheung, R. T. H. (2021). Difference in the running biomechanics between preschoolers and adults. Brazilian J Phys Ther, 25, 162–167. https://doi.org/10.1016/j.bjpt.2020.05.003
- Wille, C. M., Lenhart, R. L., Wang, S., Thelen, D. G., & Heiderscheit, B. C. (2014). Ability of sagittal kinematic variables to estimate ground reaction forces and joint kinetics in running. J Orthop Sports Phys Ther, 44(10), 825–830. https://doi.org/10.2519/jospt.2014.5367
- Wood, A. P., Imai, S., McMillan, A. G., Swift, D., & DuBose, K. D. (2020). Physical activity types and motor skills in 3-5-year old children: National Youth Fitness Survey. J Sci Med Sport, 23(4), 390–395. https://doi.org/10.1016/j.jsams.2019.11.005
- Zech, A., Venter, R., de Villiers, J. E., Sehner, S., Wegscheider, K., & Hollander, K. (2018). Motor skills of children and adolescents are influenced by growing up barefoot or shod. Front Pediatr, 6. https://doi.org/10.3389/fped.2018.00115
- Zeininger, A., Schmitt, D., Jensen, J. L., & Shapiro, L. J. (2018). Ontogenetic changes in foot strike pattern and calcaneal loading during walking in young children. Gait Posture, 59, 18–22. https://doi.org/10.1016/j.gaitpost.2017.09.027