Abstract
Purpose: Hepatocellular carcinoma (HCC) after insufficient radiofrequency ablation (RFA) could induce epithelial–mesenchymal transition (EMT) in residual tumours, resulting in rapid and aggressive recurrence. However, the role of EMT-related Long noncoding RNAs (lncRNAs) in residual tumour progression remains unclear.
Methods: Insufficient RFA was simulated in vitro by heating Huh7 cells in water bath at 47 °C, named as Huh7-H. Cell invasion, migration assays and wound healing assay were conducted for functional analysis. Cell proliferation was determined by CCK8 assay. Differential expression profile of EMT-related lncRNAs between Huh7-H and Huh7 was analysed by LncPath human EMT array, and validated by qRT-PCR. Gain/loss-of-function assays of selected lncRNA were conducted by over-expressing or silencing its expression.
Results: Huh7-H presented characteristic EMT morphological changes. WB analysis showed significantly decreased E-cadherin in Huh7-H cells. Transwell assays indicated the abilities of Huh7-H cells in migration and invasion were evidently strengthened. A new lncRNA, FUNDC2P4, was identified by LncPath human EMT array to be significantly down-regulated in Huh7-H cells. In vitro studies showed overexpression of FUNDC2P4 inhibited proliferation, invasion and migration potential and up-regulated E-cadherin expression in SMMC-7721 cells, whereas silencing FUNDC2P4 promoted these potentials and down-regulated E-cadherin expression in Huh7 cells.
Conclusions: We explored that lncRNA FUNDC2P4 down-regulation promoted EMT leading to tumour proliferation, invasion and migration by reducing E-cadherin expression in residual HCC after insufficient RFA in vitro. These results suggest that FUNDC2P4 may have potentially therapeutic value for prevention and treatment of HCC recurrence after RFA in the future.
Introduction
Hepatocellular carcinoma (HCC) is one of the most common malignancies in the worldwide that leads to high morbidity and mortality [Citation1]. In China, HCC is the third leading cause of cancer death among both men and women in 2015 [Citation2]. Even though liver transplantation is the best option for HCC patients, limited supply of good donor organs restricted its application, thus other treatments including radiofrequency ablation (RFA) and resection were used to delay tumour recurrence. However, clinical studies have shown that due to anatomical location restrictions (such as adjacent to the important organs, blood vessels), insufficient ablative margin, heat loss via tumour blood vessels, the presence of a low-temperature area in ablation for a large tumour, HCC was difficult to achieve sufficient ablation [Citation3–6]. Currently RFA is most widely used to treat HCC patients who are not eligible for resection or orthotopic liver transplantation [Citation7]. However, the major problem with RFA is its difficulties to achieve complete damage to HCC lesions due to several reasons [Citation4–6]. More seriously, HCCs after insufficient RFA are prone to be recurrence rapidly and aggressively [Citation8–10].
Most cases of cancer mortalities results from metastatic tumours [Citation11]. Epithelial–mesenchymal transition (EMT) has been deemed to the crucial process of malignant tumour by detaching cancer cells from the primary tumour, invading neighbouring tissue and migrating to distant organs [Citation12]. One of the landmark changes in EMT process is the loss of epithelial cell features, indicated by the reduction or deletion of most notable surface marker, E-cadherin [Citation13,Citation14], and gain of mesenchymal features [Citation15]. Residual tumour progression after insufficient RFA was reported through promoting EMT to increase the potential of HCC cells invasiveness and metastasis [Citation16]. Residual HCC after insufficient RFA was found to express lower level of epithelial phenotype markers, including E-cadherin, cytokeratin-8, cytokeratin-19 and claudin1, whereas the higher level of mesenchymal phenotype markers, including N-cadherin, Snail1, Vimentin and Twist1 [Citation3,Citation16,Citation17]. Our previous study measured serum CECs and VEGF levels, found their closely relationship with the rate of recurrence or metastasis in HCC after RFA [Citation18]. All findings elucidated the pivotal role of EMT in studying the underlined mechanisms of residual tumour progression after insufficient RFA [Citation12,Citation16,Citation17].
Long non-coding RNAs (lncRNAs) are a class of transcripts with a length between 200 and 100 000 nt that have no ability to encode proteins. LncRNAs were reported to be widely involved in tumour growth, invasion and metastasis through their interactions with DNA, protein and RNA [Citation19]. Recently, many studies have shown that lncRNAs played a key role in the process of EMT in HCC [Citation20]. LncRNA ATB could up-regulate ZEB1/ZEB2 by competitively binding miR-200 s and promote EMT in HCC [Citation21]. LncRNA ZEB1-AS1, a biomarker of HCC poor prognosis, could regulate EMT by direct targeting ZEB1 expression [Citation22]. Another lncRNA AOC4P, which is reported to be a tumour suppressor gene of HCC, could be combined with Vimentin to promote its degradation, thus inhibit EMT to reduce HCC invasive and metastatic ability [Citation23]. LncRNA HULC induced EMT through the miR-200a-3p/ZEB1 signalling pathway in the form of ceRNA [Citation24]. However, the role of lncRNAs in residual tumour progression after incomplete RFA has remained widely elusive.
LncPath™ Human EMT Array is designed to detect the expression level of 390 lncRNAs associated with EMT. Compared with conventional lncRNA chips, the number of differentially expressed lncRNAs in array is more accurate. In this study, we employed LncPath™ Human EMT Array to screen the differential expression profile of lncRNAs in Huh7-H cells, which was established by treating Huh7 cells under heat to mimic cancer cells after insufficient RFA in vitro. By compared with control cells, a new lncRNA FUNDC2P4, which was significantly down-regulated after heat treatment, was chosen as our target gene. By over expressing or silencing FUNDC2P4, we found it promoted EMT by reducing E-cadherin expression in residual HCC after insufficient RFA, leading to tumour progression.
Materials and methods
Cell lines and human specimen
Huh7, Hep 3B, SMMC 7721 and HCCLM3 cell lines (Type Culture Collection of the Chinese Academy of Sciences, Shanghai, China) were maintained in RPMI1640 medium (Gibco, USA) supplemented 10% foetal bovine serum (FBS), 100 U/ml penicillin and 100 mg/ml streptomycin in 5% CO2 chamber at 37 °C. The human HCC and corresponding adjacent normal tissues were obtained from 25 HCC patients who underwent surgical resection at the First Affiliated Hospital of Hainan Medical College between 2014 and 2016. The residual HCC tissues after RFA used in this study were obtained from another 15 HCC patients by puncture biopsy. Clinical and pathological characteristics were obtained from patient charts (). This study was approved by the Ethics Committee of the First Affiliated Hospital of Hainan Medical College, written consent was obtained from individual patients.
Table 1. Patient characteristics.
Establishment of Huh7-H cell line
Huh7-H cell line simulated by insufficient RFA in vitro was established as described in reference [Citation16,Citation25]. Briefly, Huh7 cells were seeded into 7 cm diameter petri dishes at the density of 1 × 105 cells/well, and incubated for 24 h. Then cells were subjected to repeated cycles as following: parafilm sealed plates were submerged in a 47 °C water bath for 5 min; then cells were recovered under normal culture condition; after grown to 80% confluence, cells were sequentially heated in 47 °C water bath for 10 min, 15 min, 20 min and 25 min in each cycle. Survived cells from last cycle were designated as Huh7-H cell line. The morphological changes of Huh7 and Huh7-H cells were determined under microscopy (Olympus, Tokyo, Japan).
Western blot analysis
Proteins were separated by 10% SDS-polyacrylamide gel electrophoresis (SDS-PAGE) and transferred to a polyvinylidene difluoride (PVDF) membrane. After blocking with 5% non-fat dried milk at room temperature for 1 h, the blots were hybridised with primary antibodies N-cadherin, E-cadherin, Vimentin (Cell Signaling Technology) and β-actin for 2 h at 24 °C. After four washes in TBST (Tris–HCl–buffered saline supplemented with 0.5% Tween 20) for 8 min each, the blots were probed with secondary antibody (Santa Cruz) at room temperature for 1.5 h. After another four washes, membranes were documented with an enhanced chemiluminescence (ECL) Western Blot detection system (Thermo Fisher Scientific Inc., Waltham, MA). The amount of proteins was quantified using Image J software (Bethesda, MD) and expressed as the ratio to β-actin.
Cell proliferation assay
Cell proliferation was detected using Cell Counting Kit-8 (CCK-8, Dojindo) per instruction. Briefly, after seeding 1 × 104 cells/well into 96-well plates, the proliferation of cells was measured at four time points (0, 24, 48 and 72 h). After an additional CCK-8 solution 10 μl for 4 h incubation at 37 °C in 5% CO2 chamber, the absorbance at 450 nm in each well was assessed with a Microplate Reader (Multiscan MK3, Thermo Fisher Scientific Inc.).
Cell migration and invasion assays
Cell migration and invasion were evaluated by a transwell chamber with and without Matrigel respectively using the Corning Kit (Corning) per instruction. In both assays, 2 × 104 cells were added into the upper well and 700 μl complete medium serum containing 10% FBS was added into the lower chamber. After incubation for 48 h, the wells were washed for three times with PBS, migrated cells were fixed with 4% paraformaldehyde for 20 min. After three washes, cells were incubated with crystal violet for 10 min, and then washed in distilled water. The number of cells was counted in 9 random fields under microscopy (CX41, Olympus, Tokyo, Japan). For the invasion assay, 100 μL gel solution containing Matrigel solution and serum-free high glucose DMEM medium at 1:15 (0.8 μg/μL) ratio was added to each upper chamber at the beginning of the experiment.
Cell migration was also detected by wound healing assay. Briefly, 5 × 104 cells per well were seeded into 96-well plates. After growing more than 90% confluence, a sterile 200-ml pipette tip was used to create artificial wounds. Then the well was washed gently 2–3 times with serum-free RPMI 1640 and cultured with 5% FBS medium at 37 °C. Migrated cells into the wound were observed and photographed under fluorescent microscopy (IX71, Olympus, Tokyo, Japan) at 0, 8 h. Relative migration rate was determined by the wound distance averaged in three-independent wound sites.
Microarray and computational analysis
Total RNA from Huh7 and Huh7-H cells were isolated using TRIzol reagent (Shanghai Pufei Biotech Co., Ltd, Shanghai, China), and quantified by the NanoDrop ND-1000 (Thermo Fisher Scientific Inc.). Microarray analysis was conducted according to the manufacturer’s instruction. Briefly, fluorescent cRNA was transcribed from total RNA using Arraystar Flash RNA Labeling protocol (Arraystar), then hybridised onto the LncPath™ Human EMT Array (8 × 15K, Arraystar, KangChen Inc, Shanghai, China). After washing, the array was scanned by Agilent Scanner G2505C. Acquired images were analysed using Agilent Feature Extraction software (version 11.0.1.1), followed by quantile normalisation and data processing using R software package. After filtering, differentially expression pattern of EMT-related lncRNAs among samples were hierarchically clustered. LncRNAs with fold changes ≥1.5 and p values ≤0.05 were selected as candidates.
RNA preparation and qRT-PCR
Total RNA was isolated from cells or tissues in the same way as above; cDNA library was synthesised using Moloney Murine Leukemia Virus Reverse Transcriptase (M-MLV RT) kit (Promega, Madison, WI) according to the manufacturer's protocols. Quantitative real-time PCR (qRT-PCR) was carried out using Mx3000P qPCR System (Agilent, Santa Clara, CA). Relative expression levels of genes were calculated by the 2−ΔΔct method (16). Primers used were as follows: sense, 5′-CGCAAGACAAGCTCGATGAAAT -3′, antisense, 5′- AAACAAAACACCTCCCACAACT-3′ for FUNDC2P4; sense, 5′- AGAACATTGATGATGGCACCTC-3′, antisense, 5′-GAGTACCTTGTGGGCAGTAGAT-3′ for RPL27P7; sense, 5′-CGATCCCACCTAATATCATCCC -3′, antisense, 5′-CGGCTTCACAGGCAGCAAATAC-3′ for MTND4LP14; and sense, 5′- TGACTTCAACAGCGACACCCA -3′, antisense, 5′-CACCCTGTTGCTGTAGCCAAA-3′ for GAPDH (internal control).
Plasmid, lentiviral construction, cell transfection and stable cell lines construction
To determine whether lncRNA FUNDC2P4 affects HCC cell invasion and metastasis, we designed various plasmids to conduct gain-of-function and loss-of-function assays. In view of expressing profile in different HCC cell lines, lncRNA FUNDC2P4 was over-expressed in SMMC-7721 and knock downed in Huh7 cells. For overexpression, the full-length cDNA of human lncRNA FUNDC2P4 was synthesised and cloned into GV367-LV vectors with AgeI/NheI restriction enzyme sites (Genechem Company, Shanghai, China). To produce lentivirus, 293 T cells were co-transfected with the constructed FUNDC2P4 vector, pHelper 1.0 and pHelper 2.0 (Genechem Company, Shanghai, China), and Lipofectamine 2000 per instruction. After 48 h transfection, lentiviruses were harvested and named as LV-FUNDC2P4. For negative control, empty vectors were transfected and lentivirus were named as LV-Control. SMMC-7721 cells were used to be infected with either LV-FUNDC2P4 or LV-Control to generate stably over-expressing cell lines. For knocking down assays, three different FUNDC2P4-specific siRNAs and one negative control siRNA were designed and synthesised (Shanghai Sangon Biotech). The target sequences of siRNAs were as follows: FUNDC2P4-siRNA-121, 5′- GGCCGAGTCCAGTCAAGCAAA-3′; FUNDC2P4-siRNA-206, 5′- AAGCTGTTCAGGCAGGAATCT-3′; FUNDC2P4-siRNA-303, 5′- ATCCCAGAAGGTTGGAAAGTT-3′ and negative control, 5′- TTCTCCGAACGTGTCACGT-3′. Huh7 cells were transfected with either FUNDC2P4-siRNAs or control siRNA (si-control). The expression level of FUNDC2P4 was measured by qRT-PCR at 72 h after infection to determine the effects of siRNAs.
Statistical analyses
SPSS statistics 19.0 (SPSS Inc., Chicago, IL) and the GraphPad Prism 7.00 Software (GraphPad Software, La Jolla, CA) were employed to determine all statistical differences. Statistical analysis was calculated by one-way analysis of variance (ANOVA) followed by Tukey’s multiple comparison test, or a sample-independent t test. χ2 test or Fisher’s exact test were performed to analyse numeration data. Data were expressed as means ± standard deviations (SD). Differences were considered significant at a p value of <0.05.
Results
Establishment and characterisation of Huh7-H cell line
In this study, we sequentially treated Huh7 cells under 47 °C for 5 min, 10 min, 15 min, 20 min and 25 min in a water bath, successfully established a cell line in vitro to mimic residual tumour cells after insufficient RFA, named as Huh7-H, to evaluate the effect of insufficient RFA on HCC cells. We found that Huh7-H cells showed spindle cell-like morphology with less cell–cell adhesion compared with Huh7 cells and loss of polarity (). To evaluate EMT changes in Huh7-H cells, we tested several EMT markers. By western blot, we found a significant down-regulation of E-cadherin expression, and notably up-regulation of N-cadherin and vimentin (). Huh7-H displayed stronger proliferation ability compared with Huh7 at 24 h, 48 h and 72 h (). Using transwell assay, more cells were observed under microscopy (), indicating Huh7-H cells possessed greater abilities in cell migration and invasion compared with Huh7 cells.
Figure 1. Establishment and characterisation of Huh7-H cell line. Huh7 cells were heated in 47 °C water bath for 5 min, 5 min, 15 min, 20 min and 25 min gradually. Residual Huh7 was named as Huh7-H. (A) Insufficient RFA induced EMT-like morphological changes in Huh7-H cells. (B) Western blot analysis of EMT markers including E-cadherin, N-cadherin and Vimentin and (C) quantifications. (D) The cell growth rates were determined by CCK8 proliferation assays. (E, F) Invasion and migration assays in Huh7 and Huh7-H cells. Data were shown as mean ± SD from three independent experiments. *p < 0.05.
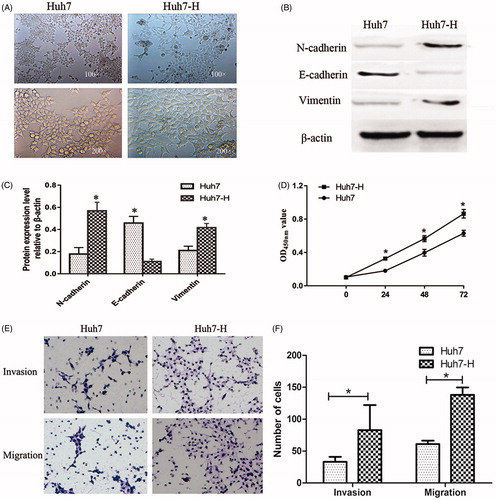
LncRNA FUNDC2P4 is down-regulated in Huh7-H cells and residual HCC tissues after RFA
To identify lncRNAs involved in EMT induced by insufficient RFA, LncPath™ Human EMT Array was employed to screen the differential lncRNAs between Huh7 and Huh7-H cells. Hierarchical clustering showed that the significantly differentially expressed lncRNAs were as follows: down-regulated lncRNAs including FUNDC2P4, RPL27P7 and up-regulated LncRNA including MTND4LP14 (fold changes ≥1.5 and p values ≤0.05) (). To validate findings from microarray analysis, we analysed the expression levels of these three lncRNAs using qRT-PCR between Huh7 and Huh7-H cells. We found that the expression of FUNDC2P4, RPL27P7, MTND4LP14 in Huh7-H cell was 0.137, 0.869, 1.037 times of that in Huh7 cells, respectively (), only lncRNA FUNDC2P4 was observed in significant change, then we chose it for further studies in HCC cell lines. qRT-PCR showed that FUNDC2P4 was expressed significantly lower in HCC cell lines with high invasive potential including SMMC-7721 and HCCLM3, than that in HCC cell lines with low invasive potential including Huh7 and Hep 3B (). Furthermore, FUNDC2P4 was highly expressed in normal liver tissues, however, significantly decreased in paired HCC tumours (). After treatment with insufficient RFA, FUNDC2P4 dramatically down-regulated in residual HCC tissues even compared with HCC tumours (), indicating FUNDC2P4 was negatively correlated with HCC progression.
Figure 2. LncRNA FUNDC2P4 was down-regulated in HCC. (A) Hierarchical Clustering of all lncRNAs using LncPathTM Human EMT Array. (B) The selected lncRNAs which were significantly differentially expressed were analysed by qRT-PCR, Huh7 cells as a control. (C) FUNDC2P4 expression level in HCC cell lines (SMMC-7721, HCCLM3, Huh7 and Hep3B) was analysed by qRT-PCR, a normal liver cell (L02) as a control. (D) FUNDC2P4 expression level was analysed by qRT-PCR in HCC tissues, paired normal tissues and residual HCC tissues after RFA. Data were shown as mean ± SD from three independent experiments. *p < 0.05.
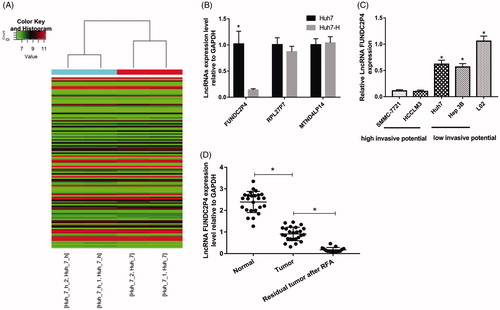
LncRNA FUNDC2P4 inhibits cell proliferation, migration and invasion
Due to low expression level in SMMC-7721 (), we developed stably over-expressed cell line of FUNDC2P4 by infecting SMMC-7721 cells with LV-FUNDC2P4 (). To investigate the functions of FUNDC2P4, we evaluated its abilities in cell proliferation, migration and invasion. We found that FUNDC2P4 overexpression significantly inhibited cell proliferation ability compared with control cell line (). By transwell assay, lower cells were observed under microscopy (), indicating that cells were losing the ability of invasion and migration after over expression of FUNDC2P4. By wound healing assay, we observed the same outcomes, SMMC-7721 cells with over-expressed FUNDC2P4 possessed lower potential in wound healing (). In contrast, after knocking down endogenous FUNDC2P4 in Huh7 cells using siRNAs (), FUNDC2P4-siRNA-121 displayed significant reduction potential in its expression than any other siRNAs, then we developed Huh7 cells with stably silencing FUNDC2P4 by infecting with FUNDC2P4-siRNA-121. By depletion of FUNDC2P4, we found that cells gained significantly higher abilities in cell proliferation (), invasion () and migration () compared with control cells. Taken together, these results suggested that FUNDC2P4 suppressed HCC cell progression.
Figure 3. Overexpression of LncRNA FUNDC2P4 inhibited proliferation, invasion and migration potential of SMMC-7721 cells and silencing LncRNA FUNDC2P4 promoted proliferation, invasion and migration potential of Huh7 cells. (A, C) SMMC-7721 cells were infected with LV-FUNDC2P4 and stably over-expressed LncRNA FUNDC2P4. (B, D) Huh7 cells were infected with siRNAs, and LncRNA FUNDC2P4 expression was test by qRT-PCR. Magnification: 100×. (E, F) Cell migration of SMMC-7721 cells overexpressing LncRNA FUNDC2P4 and Huh7 cells silencing LncRNA FUNDC2P4 were determined by CCK8 proliferation assays. (G, H) Transwell invasion and migration assays were performed in SMMC-7721 cells overexpressing LncRNA FUNDC2P4 and Huh7 cells silencing LncRNA FUNDC2P4. Magnification: 200×. Data shown are mean ± SD from three independent experiments. *p < 0.05.
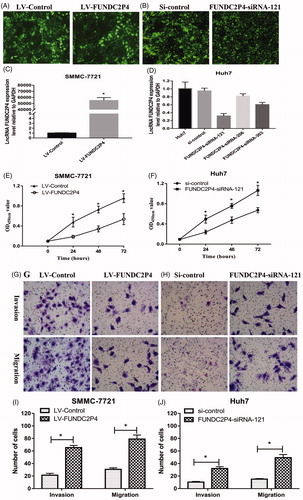
Figure 4. LncRNA FUNDC2P4 inhibits EMT by regulating E-cadherin expression. (A, B, C, D) Wound healing assays were performed in SMMC-7721 cells overexpressing LncRNA FUNDC2P4 and Huh7 cells silencing LncRNA FUNDC2P4. (E, F, G) EMT markers including E-cadherin, N-cadherin and vimentin were examined by Western blot and semi quantitative assays in SMMC-7721 cells overexpressing LncRNA FUNDC2P4 and Huh7 cells silencing LncRNA FUNDC2P4. Data shown are mean ± SD from three independent experiments. *p < 0.05.
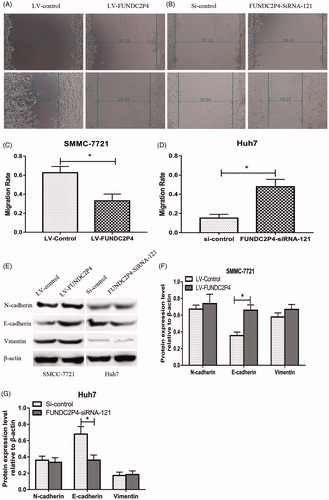
LncRNA FUNDC2P4 inhibits EMT by regulating E-cadherin expression
In view of the findings that FUNDC2P4 inhibited HCC cell abilities in cell proliferation, migration and invasion in vitro, we further investigated the potential mechanism by detecting the expression levels of E-cadherin, N-cadherin and Vimentin. Western blots results showed that the expression of E-cadherin was significantly up-regulated in SMMC-7721 cells with over-expressed FUNDC2P4 compared with that in control cells (), whereas neither N-cadherin nor Vimentin expressions were changed. In contrast, the depletion of FUNDC2P4 in Huh7 cells displayed lower expression level of E-cadherin, but no change was found in N-cadherin and Vimentin expressions (). These results suggested that FUNDC2P4 inhibited EMT through E-cadherin, not N-cadherin or Vimentin.
Discussion
At present, RFA has become the main local treatment of advanced HCC which was not able to be removed [Citation26]. However, insufficient RFA could promote HCC cell abilities of invasiveness and metastasis [Citation16], leading to residual tumour recurrence and progression rapidly. In this study, to mimic in vivo residual tumour cells after insufficient RFA, we simulated Huh7 by heat treatment using a water bath at 47 °C gradually, induced HCC cells to show EMT-like morphological changes. By western blots we found decreased E-cadherin but increased N-cadherin and Vimentin expression. Huh7-H cell abilities of proliferation, migration and invasion were enhanced, indicating in vitro cell model for mimicking residual tumour cells after insufficient RFA was established successfully.
In order to investigate the role of lncRNAs in EMT process, we hybridised RNAs isolated from Huh7-H and Huh7 cells with EMT array, found several up-regulated and down-regulated genes. Among them, we selected lncRNA FUNDC2P4, RPL27P7, MTND4LP14 for further studies. However by qRT-PCR, we only found significantly lower expression level of FUNDC2P4, no significant differences were observed in RPL27P7 and MTND4LP14, indicating false positive results were existed during data analysis. Increasing the limitations of filters or using qRT-PCR for validation were good strategies to reduce the rate of false positive.
FUNDC2P4 is a new lncRNA. In our study, we found that its expression level in HCC cell lines with high invasive potential was significantly lower than that in HCC cell lines with low invasive potential. By detecting human specimen, FUNDC2P4 in residual HCC tissues after RFA was significantly lower than HCC tissues, which was dramatically lower than that in corresponding adjacent normal tissues. For the first time we elucidated the expression profile of FUNDC2P4 in HCC progression, found its negative correlations with tumour development. Furthermore, overexpression of FUNDC2P4 inhibited SMMC-7721 cell potentials in proliferation, invasion and migration, whereas silencing FUNDC2P4 displayed opposite outcomes in Huh7 cells, showing consistent effects. Both gain or loss-of-function experimental approaches suggested that FUNDC2P4 could act as a tumour suppressor gene to inhibite tumour progression.
FUNDC2P4 localised in q13.1 in human chromosome 22 with length of 572 bp, since it was a new lncRNAs, there was no reference about its functions. Studies have reported lncRNAs could perform their functions by binding to adjacent genes [Citation27,Citation28], the target gene for same enhancer of FUNDC2P4 may be Chromobox protein homologue 7 (CBX7) which is close to FUNDC2P4 (GENECARDS). CBX7 is a member of the Polycomb group (PcG) family, a transcription factor that is in low expression level in most malignancies in diverse organs, including glioblastoma [Citation29], lung [Citation30], pancrease [Citation31], bladder [Citation32], thyroid [Citation33], breast [Citation34], colorectal [Citation35], acted as a key gene for tumour progression [Citation36,Citation37]. CBX7 can bind to the promoter region of multiple tumour-related genes (such as CDH1, SPP1 gene), up-regulate E-cadherin and osteopontin expression level, consequently inhibit tumour invasiveness and metastasis [Citation38,Citation39].
E-cadherin is a key cancer suppressor gene and its expression is often down-regulated in tumour [Citation40]. In various tumour cell lines, Snail, Slug, ZEB1, ZEB2 and Twist were reported to be induced through various signalling pathways, such as TGF-β/SMAD, Wnt/β-catenin, MAPK/ERK, PI3K/Akt, to bind to E-boxes within the promoter of CDH1 gene encoding E-cadherin and proceed to repress the expression of E-cadherin [Citation13,Citation40]. E-cadherin was reported to be negatively associated with tumour stages, tumour invasiveness and metastasis, decreased E-cadherin resulted in poorer clinical prognosis in cancers in diverse organs, including breast, lung, gastric, liver and colon [Citation41]. N-cadherin, like E-cadherin, is also a member in cadherin family, however it was not expressed in epithelial cells. During EMT, increased N-cadherin expression is always associated with decreased E-cadherin expression, which was called “cadherin switch” [Citation42]. Vimentin was also not expressed in epithelial cells, but identified as an EMT marker in epithelial malignant tumours [Citation43]. In this study, overexpression of FUNDC2P4 up-regulated the expression of E-cadherin in SMMC-7721 cells and silencing FUNDC2P4 down-regulated the expression of E-cadherin in Huh7 cells, without any changes in expression levels of N-cadherin and Vimentin. Together with all findings, we hypothesised that the underlined mechanism could be FUNDC2P4 interacted with CBX7, through activating/inactivating its target tumour related genes, consequently inhibited EMT via increasing E-cadherin expression level, FUNDC2P4 may only inhibit the process of losing E-cadherin, but had no effects on gain of mesenchymal features. Further investigations should be performed to explore the specific molecular mechanisms between FUNDC2P4 and CBX7 in residual tumour cells after insufficient RFA.
In conclusion, for the first time we identified a new lncRNA, FUNDC2P4, found it was associated with HCC cells development. By overexpressing or silencing experiments, we explored its functions in the process of EMT that FUNDC2P4 led to tumour proliferation, invasion and migration by reducing E-cadherin expression in residual HCC after insufficient RFA in vitro. These results suggest that FUNDC2P4 may have potentially therapeutic value for prevention and treatment of HCC recurrence and metastasis after RFA in the future.
Disclosure statement
The authors declare no conflicts of interest.
Additional information
Funding
References
- Siegel RL, Miller KD, Jemal A. (2016). Cancer statistics, 2016. CA Cancer J Clin 66:7–30.
- Chen W, Zheng R, Baade PD, et al. (2016). Cancer statistics in China, 2015. CA Cancer J Clin 66:115–32.
- Zhang N, Wang L, Chai ZT, et al. (2014). Incomplete radiofrequency ablation enhances invasiveness and metastasis of residual cancer of hepatocellular carcinoma cell HCCLM3 via activating beta-catenin signaling. PLoS One 9:e115949.
- Teng W, Liu KW, Lin CC, et al. (2015). Insufficient ablative margin determined by early computed tomography may predict the recurrence of hepatocellular carcinoma after radiofrequency ablation. Liver Cancer 4:26–38.
- Poch FG, Rieder C, Ballhausen H, et al. (2016). The vascular cooling effect in hepatic multipolar radiofrequency ablation leads to incomplete ablation ex vivo. Int J Hypertherm 32:749–56.
- Zheng XW, Zhao YF, Tang Z, et al. (2016). [Efficiency and influencing factors of ultrasound-guided radiofrequency ablation as treatment in hepatic malignant tumors]. Zhonghua gan zang Chin J Hepatol 24:69–73.
- Donadon M, Solbiati L, Dawson L, et al. (2016). Hepatocellular carcinoma: the role of interventional oncology. Liver Cancer 6:34–43.
- Ruzzenente A, Manzoni GD, Molfetta M, et al. (2004). Rapid progression of hepatocellular carcinoma after radiofrequency ablation. World J Gastroenterol 10:1137–40.
- Ke S, Ding XM, Kong J, et al. (2010). Low temperature of radiofrequency ablation at the target sites can facilitate rapid progression of residual hepatic VX2 carcinoma. J Transl Med 8:73.
- Kang TW, Lim HK, Cha DI. (2017). Aggressive tumor recurrence after radiofrequency ablation for hepatocellular carcinoma. Clin Mol Hepatol 23:95–101.
- Chaffer CL, Weinberg RA. (2011). A perspective on cancer cell metastasis. Science 331:1559–64.
- Yang J, Weinberg RA. (2008). Epithelial-mesenchymal transition: at the crossroads of development and tumor metastasis. Dev Cell 14:818–29.
- Lamouille S, Xu J, Derynck R. (2014). Molecular mechanisms of epithelial-mesenchymal transition. Nat Rev Mol Cell Biol 15:178–96.
- Heery R, Finn SP, Cuffe S, et al. (2017). Long non-coding RNAs: key regulators of epithelial-mesenchymal transition, tumour drug resistance and cancer stem cells. Cancers (Basel) 9:38.
- Serrano-Gomez SJ, Maziveyi M, Alahari SK. (2016). Regulation of epithelial-mesenchymal transition through epigenetic and post-translational modifications. Mol Cancer 15:18.
- Dong S, Kong J, Kong F, et al. (2013). Insufficient radiofrequency ablation promotes epithelial-mesenchymal transition of hepatocellular carcinoma cells through Akt and ERK signaling pathways. J Transl Med 11:273.
- Yoshida S, Kornek M, Ikenaga N, et al. (2013). Sublethal heat treatment promotes epithelial-mesenchymal transition and enhances the malignant potential of hepatocellular carcinoma. Hepatology 58:1667–80.
- Wang-Yuan Z, Jiang-Zheng Z, Lu YD, et al. (2016). Clinical efficacy of metronomic chemotherapy after cool-tip radiofrequency ablation in the treatment of hepatocellular carcinoma. Int J Hypertherm 32:193–8.
- Schmitt AM, Chang HY. (2016). Long noncoding RNAs in cancer pathways. Cancer Cell 29:452–63.
- Yu FJ, Zheng JJ, Dong PH, et al. (2015). Long non-coding RNAs and hepatocellular carcinoma. Mol Clin Oncol 3:13–17.
- Yuan JH, Yang F, Wang F, et al. (2014). A long noncoding RNA activated by TGF-beta promotes the invasion-metastasis cascade in hepatocellular carcinoma. Cancer Cell 25:666–81.
- Li T, Xie J, Shen C, et al. (2016). Upregulation of long noncoding RNA ZEB1-AS1 promotes tumor metastasis and predicts poor prognosis in hepatocellular carcinoma. Oncogene 35:1575–84.
- Wang TH, Lin YS, Chen Y, et al. (2015). Long non-coding RNA AOC4P suppresses hepatocellular carcinoma metastasis by enhancing vimentin degradation and inhibiting epithelial-mesenchymal transition. Oncotarget 6:23342–57.
- Li SP, Xu HX, Yu Y, et al. (2016). LncRNA HULC enhances epithelial-mesenchymal transition to promote tumorigenesis and metastasis of hepatocellular carcinoma via the miR-200a-3p/ZEB1 signaling pathway. Oncotarget 7:42431–46.
- Obara K, Matsumoto N, Okamoto M, et al. (2008). Insufficient radiofrequency ablation therapy may induce further malignant transformation of hepatocellular carcinoma. Hepatol Int 2:116–23.
- Shiina S, Tateishi R, Arano T, et al. (2012). Radiofrequency ablation for hepatocellular carcinoma: 10-year outcome and prognostic factors. Am J Gastroenterol 107:569–77.
- Wang P, Xue Y, Han Y, et al. (2014). The STAT3-binding long noncoding RNA lnc-DC controls human dendritic cell differentiation. Science 344:310–13.
- Sun CC, Li SJ, Li G, et al. (2016). Long intergenic noncoding RNA 00511 acts as an oncogene in non-small-cell lung cancer by binding to EZH2 and suppressing p57. Mol Ther Nucleic Acids 5:e385.
- Li G, Warden C, Zou Z, et al. (2013). Altered expression of polycomb group genes in glioblastoma multiforme. PLoS One 8:e80970.
- Forzati F, Federico A, Pallante P, et al. (2012). CBX7 is a tumor suppressor in mice and humans. J Clin Investig 122:612–23.
- Karamitopoulou E, Pallante P, Zlobec I, et al. (2010). Loss of the CBX7 protein expression correlates with a more aggressive phenotype in pancreatic cancer. Eur J Cancer (Oxford, England: 1990) 46:1438–44.
- Hinz S, Kempkensteffen C, Christoph F, et al. (2008). Expression parameters of the polycomb group proteins BMI1, SUZ12, RING1 and CBX7 in urothelial carcinoma of the bladder and their prognostic relevance. Tumor Biol 29:323–9.
- Pallante P, Federico A, Berlingieri MT, et al. (2008). Loss of the CBX7 gene expression correlates with a highly malignant phenotype in thyroid cancer. Cancer Res 68:6770–8.
- Mansueto G, Forzati F, Ferraro A, et al. (2010). Identification of a new pathway for tumor progression: MicroRNA-181b up-regulation and CBX7 down-regulation by HMGA1 protein. Genes Cancer 1:210–24.
- Pallante P, Terracciano L, Carafa V, et al. (2010). The loss of the CBX7 gene expression represents an adverse prognostic marker for survival of colon carcinoma patients. Eur J Cancer (Oxford, England: 1990) 46:2304–13.
- Pallante P, Forzati F, Federico A, et al. (2015). Polycomb protein family member CBX7 plays a critical role in cancer progression. Am J Cancer Res 5:1594–601.
- Ni S, Wang H, Zhu X, et al. (2017). CBX7 suppresses cell proliferation, migration, and invasion through the inhibition of PTEN/Akt signaling in pancreatic cancer. Oncotarget 8:8010–21.
- Federico A, Pallante P, Bianco M, et al. (2009). Chromobox protein homologue 7 protein, with decreased expression in human carcinomas, positively regulates E-cadherin expression by interacting with the histone deacetylase 2 protein. Cancer Res 69:7079–87.
- Sepe R, Formisano U, Federico A, et al. (2015). CBX7 and HMGA1b proteins act in opposite way on the regulation of the SPP1 gene expression. Oncotarget 6:2680–92.
- Onder TT, Gupta PB, Mani SA, et al. (2008). Loss of E-cadherin promotes metastasis via multiple downstream transcriptional pathways. Cancer Res 68:3645–54.
- Chun HW, Hong R. (2016). Significance of the hedgehog pathway-associated proteins Gli-1 and Gli-2 and the epithelial-mesenchymal transition-associated proteins Twist and E-cadherin in hepatocellular carcinoma. Oncol Lett 12:1753–62.
- Hazan RB, Qiao R, Keren R, et al. (2004). Cadherin switch in tumor progression. Ann NY Acad Sci 1014:155–63.
- Zeisberg M, Neilson EG. (2009). Biomarkers for epithelial-mesenchymal transitions. J Clin Investig 119:1429–37.