Abstract
Objective: To evaluate the efficiency and safety of percutaneous ablation of the tumor feeding artery (PAA) before radiofrequency ablation (RFA) for hypervascular hepatocellular carcinoma (HCC) under ultrasound guidance.
Patients and methods: In total, 94 patients with hypervascular HCC and tumor feeding artery visible by contrast-enhanced ultrasound were prospectively randomized to receive either PAA combined with RFA (RFA + PAA) or RFA alone. This study was registered at the clinical trials registry website (No. NCT03143140). The mean tumor size was 3.2 ± 0.9 cm (2.0–5.0 cm). The mean follow-up was 23.7 ± 9.7 months (4–44 months). The technical success, local tumor progression and intrahepatic distant recurrence rates were compared. Survival analysis was performed using the Kaplan–Meier method and compared with the log-rank test.
Results: The local tumor progression rate was lower for the PAA + RFA group than for the RFA group (8.5% vs 21.3%, p = .082). No significant differences in the technical success and intrahepatic distant recurrence rates were observed between the two groups (97.9% vs 91.5%, p = .203 and 40.4% vs 42.6%, p = .834). The 1- and 3-year local tumor progression-free survival rates were 91.5% and 69.9% vs 68.1% and 52.1% for the PAA + RFA vs RFA groups, respectively (p = .052). The 1- and 3-year overall survival rates were 95.7% and 69.1% vs 89.4% and 66.6% in the PAA + RFA vs RFA groups, respectively (p = .744). The major complication rate was 4.3% in both groups.
Conclusions: PAA appears to be an effective and safe technique for the treatment of hypervascular HCC, with a lower local tumor progression rate than that of conventional RFA.
Introduction
Hepatocellular carcinoma (HCC) is one of the most commonly diagnosed cancers. An estimated 745,500 liver cancer-related deaths occurred worldwide in 2012 [Citation1]. Recently, local ablation techniques, especially radiofrequency ablation (RFA), have been considered as curative treatment options for early-stage HCC [Citation2]. However, RFA appears to be less effective for hypervascular HCC due to its blood flow cooling effect [Citation3–5], which may lead to a residual tumor or recurrence. Various modalities have been introduced to improve clinical outcomes, such as RFA combined with transcatheter arterial chemoembolisation (TACE) [Citation6,Citation7] and RFA combined with percutaneous arterial embolization [Citation8]. In 2009, our center reported a novel technique called percutaneous ablation of the tumor feeding artery (PAA) before tumor ablation for the treatment of hypervascular HCC [Citation9]. With this method, the tumor is ablated under ischemic conditions after PAA, which results in a larger ablated necrotic area. The results show that PAA significantly decreases local tumor recurrence. With the emergence of new ablation equipment and ultrasonic imaging techniques, we have improved the operating strategy to make the treatment more precise and simple. The purpose of this study was to evaluate the efficiency and safety of PAA before RFA for the treatment of hypervascular HCC compared with conventional tumor ablation in a randomized controlled trial.
Patients and methods
Patients
From May 2014 to February 2017, 122 HCC patients who planned to receive RFA were prospectively recruited for this study. Hypervascular HCC was defined as a tumor artery that was detectable by three-dimensional contrast-enhanced ultrasonography (3D-CEUS). The patient inclusion criteria were as follows: (1) the tumor feeding artery can be detected by 3D-CEUS, and the tumor can be ablated percutaneously under ultrasound guidance; (2) non-surgical candidate; (3) non-TACE candidate due to previous TACE failure; (4) tumor size ≤5 cm and tumor number ≤3; and (5) platelet count ≥50,000/ml and international normalized ratio (INR) < 1.6. Of the 122 patients, 7 were unsuitable to receive percutaneous ablation (5 due to lack of a safety puncture path, and 2 withdrew consent), and 21 did not have a visible feeding artery by 3D-CEUS. Finally, 94 patients were enrolled in this study (). The patients were randomized into two groups using a simple randomization strategy as follows: (1) PAA + RFA group: percutaneous ablation of the feeding artery combined with tumor ablation and (2) RFA group: conventional tumor ablation.
In total, 64 men and 30 women were enrolled in this study. The clinical characteristics of the patients are shown in . The mean age was 65.1 ± 11.0 years (range 34–84 years). Among the 94 patients, 68 had liver cirrhosis mostly related to hepatitis virus B infection. A total of 89 patients had Child-Pugh class A cirrhosis, and 5 patients had Child-Pugh class B cirrhosis. In addition, 34 patients had previously received TACE (16 in the PAA + RFA group and 18 in the RFA group). For the 94 enrolled patients, 115 tumors were ablated, including 1 tumor for 78 patients, 2 tumors for 11 patients and 3 tumors for 5 patients. When the tumor number was >1, all the tumors were ablated simultaneously in one procedure. However, only the largest tumor was included in our analysis, and the other smaller tumors with diameters less than 2 cm were not included. The mean tumor size was 3.2 ± 0.9 cm (range 2.0–5.0 cm). The mean follow-up after RFA was 23.7 ± 9.7 months (range 4–44 months).
Table 1. Clinical characteristics of the HCC patients (n = 94).
Equipment
Philip IU22 (Philips Healthcare, Bothell, WA) and GE Logiq E9 (GE Healthcare, New York, CT) ultrasound devices were used in this study. Colour Doppler ultrasound and 3 D contrast-enhanced ultrasound with a 1–6 MHz transducer (X6-1 xMatrix array, Philip IU22) were regularly performed to confirm the tumor feeding artery using 3.5–5.0 MHz small-sector and board-view convex probes (C1-5, GE Logiq E9) equipped with attachments for guidance. SonoVue (BraccoSpA, Milan, Italy) was used as the contrast agent in this study. RFA treatment was performed using the Celon RFA system (Olympus, Teltow, Germany).
Feeding artery confirmation by 3 D contrast-enhanced ultrasound
One radiologist (L.J.C.) with more than 5 years of experience in liver CEUS performed each 3D-CEUS with a mechanical index of 0.08. SonoVue was injected intravenously as a 1.5–2.0-ml bolus via a 20-gauge cannula, followed by a 5-ml saline flush. The tumor feeding artery was observed in the arterial phase (from 10–20 to 30–45 s after SonoVue injection). The 3 D region of interest and the scanning angle were adjusted to include the lesions and the normal liver parenchyma around the tumors. During the arterial phase, the patients were required to hold their breath. The 3D-CEUS sonograms were collected and stored on a computer. Then, the feeding artery was confirmed by sonogram analysis. Feeding arteries were defined as the arteries that approached, partially surrounded, or penetrated into lesions on the sonograms.
PAA and RFA procedures
PAA group
After the tumor feeding artery was assessed by 3D-CEUS, two electrode needles were inserted parallel with a distance between needles of less than 1 cm into the area where the artery entered the tumor. First, the feeding artery and surrounding liver parenchyma were ablated to cut off the blood supply to the tumor. For tumors with more than one feeding artery, only the major feeding artery was ablated. Then, the tumor plus an ablative margin of 0.5–1.0 cm of normal tissue were ablated under ischemic conditions ( and ).
Figure 2. Schematic diagram of percutaneous ablation of the tumor feeding artery. (Left) Two electrode needles were inserted parallel to the area where the artery entered the tumor. (Right) After blocking the feeding artery, the whole tumor was ablated.
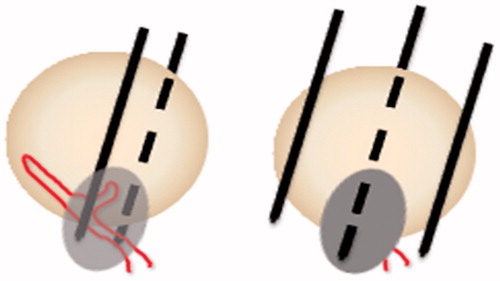
Figure 3. An 80-year-old woman with hepatocellular carcinoma at S4 was treated using PAA + RFA. (A) CT showed the tumor located at S4 with a 3.7-cm diameter. (B) Color Doppler ultrasound showed the feeding artery of the tumor. (C) CEUS showed that the tumor was hyper-enhanced in the arterial phase. (D) 3D-CEUS showed the feeding artery of the tumor (arrow). (E) The feeding artery and surrounding liver parenchyma were ablated by two electrode needles inserted parallel to each side of the artery. (F) Immediate CEUS showed that the tumor was under ischemic conditions. (G) Then, the whole tumor was coagulated under ultrasound guidance. (H) Enhanced CT 4 months after ablation showed that the tumor had completed necrosis.
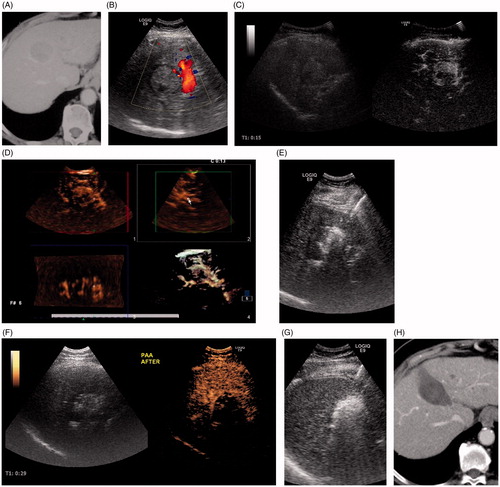
RFA group
The tumor was coagulated using the conventional tumor ablation technique. The ablation was performed with the intention of coagulating the tumor itself plus an ablative margin of 0.5–1.0 cm. Two to three bipolar electrodes were inserted directly into the edges of the tumor at its maximum dimension. The distance between the needles was equal to or less than 2.5 cm. The tip of the needle was placed approximately 0.5 cm deeper than the tumor under ultrasound guidance. RF energy was simultaneously applied at 30–40 W. For tumors larger than 3 cm, a strategy of multiple overlapping ablations was used [Citation10]. After treatment, track ablations were performed routinely.
All the treatments were performed by two radiologists with more than ten years of experience with ultrasound-guided RFA (C.M.H., Y. K., W.W. and Y.W.).
Assessment of efficacy and follow-up
The treatment response was evaluated using contrast-enhanced computed tomography (CECT) or magnetic resonance imaging (MRI) 1 month after the RFA procedure. CECT using the triple-phase technique includes arterial, portal and delay phases. The MRI protocol included at least T1-weighted imaging, T2-weighted imaging, diffusion-weighted imaging and dynamic contrast-enhanced T1-weighted imaging. Then, the serum alpha-fetal protein (AFP) test, an abdominal ultrasound and a CECT or MRI were performed every 3 months for 2 years and every 6 months thereafter. Technique success was defined as no abnormal enhancement or washout within or around the ablation zone one month after the RFA. The ablation was considered a technique failure if abnormal enhancement was observed within or around the ablation zone one month after the RFA. A case with no abnormal enhancement found one month after the RFA but the development of abnormal enhancement washout around the ablation zone at follow-up was defined as local tumor progression. Identification of a new tumor in another region of the liver was defined as intrahepatic distant recurrence.
Safety assessment
Complications were recorded for both groups during follow-up. Major complications were referred to as events that threatened the patients’ lives, caused disability or prolonged hospitalization [Citation11]. All other complications were regarded as minor complications.
Study registration and ethical arrangements
This study was registered at the clinical trials registry website (https://clinicaltrials.gov/) (No. NCT03143140). This clinical study was approved by the Institutional Review Board of Peking University Cancer Hospital. Written informed consent was obtained from all the patients before the RFA procedure.
Statistical analyses
Continuous variables were expressed as the mean ± SD. Continuous variables were compared using Student’s t test. Categorical variables were compared using the Chi-square test or Fisher’s exact test. Survival analysis was performed with the Kaplan–Meier method, and comparisons were performed with the log-rank test. The level of significance was set at 0.05 for all tests. SPSS 19.0 software (SPSS Inc., Chicago, IL) was used for all the statistical analyses.
Results
Tumor response
According to the enhanced CT or MRI one month post-treatment, the ablation zone diameter was larger in the PAA + RFA group than in the RFA group (4.5 ± 0.9 cm vs 4.1 ± 0.8 cm, p = .027). The comparison of treatment effects between the two groups is shown in . No significant difference in the technical success rate was observed between the two groups (97.9% vs 91.5%, p = .203). Technical failure was found in one case in the PAA + RFA group (a tumor >3 cm) and five cases in the RFA group (three cases of tumors >3 cm, one case of a tumor adjacent to the colon and one case of a tumor adjacent to a large vessel). In addition, four patients received repeated RFAs, and two other patients received radiotherapy. Local tumor progression was found in four cases in the PAA + RFA group at 4–17 months (treated with a repeat RFA in three cases and radiotherapy in one case) and in 10 cases in the RFA group (treated with a repeat RFA in eight cases and radiotherapy in two cases) at 4–11 months. The local tumor progression rate was lower for the PAA + RFA group than for the RFA group (8.5% vs 21.3%, p = .082). The 1- and 3-year local tumor progression rates were 7.4% and 9.1% vs 22.1% and 22.1% in the PAA + RFA vs RFA groups (p = .066) (). No significant difference was found in intrahepatic distant recurrence (40.4% vs 42.6%, p = .834). Intrahepatic distant recurrence was found in 19 cases in the PAA + RFA group (treated with a repeat RFA in 14 cases and TACE in 5 cases) and in 20 cases in the RFA group (treated with a repeat RFA in 14 cases and TACE in 6 cases).
Figure 4. Local tumor progression rates in the PAA + RFA and RFA groups. The 1- and 3-year local tumor progression rates were 7.4% and 9.1% vs 22.1% and 22.1% in the PAA + RFA vs RFA groups (p = .066).
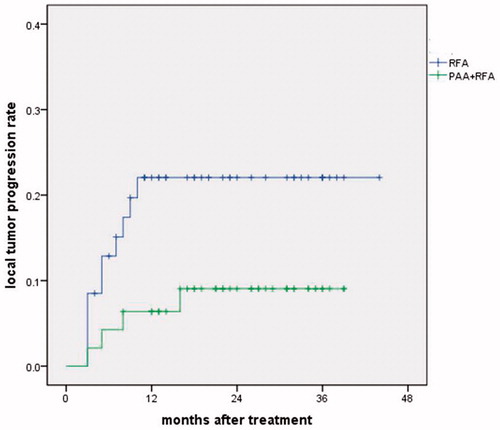
Table 2. Comparison of clinical outcomes after treatment.
Survival
All follow-ups ended in January 2018. The mean follow-up after RFA was 23.7 ± 9.7 months (range 4–44 months). At the time of the last follow-up, 73 patients were alive, and 21 patients had died of HCC progression. The 1-, 2- and 3-year local tumor progression-free survival rates were 91.5%, 77.7% and 69.9% vs 68.1%, 55.9% and 52.1% in the PAA + RFA vs RFA groups, respectively (p = .052) (). The 1-, 2- and 3-year overall survival rates were 95.7%, 84.5% and 69.1% vs 89.4%, 77.1% and 66.6% in the PAA + RFA vs RFA groups, respectively. No significant difference in the overall survival rate was found between the two groups (p = .744) ().
Complications
In the PAA + RFA group, one case of post-treatment hemorrhage around the liver and one case of portal vein thrombosis were reported. The hemorrhage was controlled by conservative treatment without further interventional therapy. The vein thrombosis was cured by anticoagulant therapy. In the RFA group, two cases of liver abscess occurred and were resolved after antibiotic therapy and drainage. No significant difference in the major complication rate was found between the two groups (p = .692).
Discussion
RFA has been widely utilized for the treatment of HCC. The effect of ablation is partially limited by blood flow – induced thermal loss in the tumor and liver tissue, which is called the heat-sink effect. The reduction of blood flow during ablation can increase the coagulation zone in both in vitro and in vivo models [Citation12]. To improve the RFA outcomes, various modalities have been introduced to reduce the heat-sink effect. Hepatic vascular inflow occlusion by the Pringle maneuver during open laparotomy or a laparoscopic operation can effectively expand the coagulation zone [Citation13,Citation14]. However, the Pringle maneuver cannot be performed during percutaneous RFA.
TACE, which is widely used for unresectable HCC, can cause embolization of the feeding artery, resulting in tumor ischemic necrosis. Previous studies showed that TACE combined with RFA improved clinical outcomes compared with RFA alone. Peng et al. [Citation7] compared the effects of RFA after TACE with RFA alone in 139 patents with an HCC smaller than 5 cm. The 1-, 3- and 5-year recurrence-free survival rates were significantly higher in the combined TACE + RFA group than in the RFA group (80%, 45% and 40% vs 64%, 18% and 18%, respectively, p = .005). Meta-analysis showed that the combination of TACE and RFA was significantly associated with higher 5-year survival rates (OR = 1.87, 95%CI 1.23–2.83, p = .003) [Citation15]. However, some patients are not candidates for TACE or have poor responses to TACE. Therefore, other methods to reduce the heat-sink effect are needed for those patients.
Recently, our center developed two novel techniques to block the tumor feeding artery in patients with hypervascular HCC before RFA. One technique was named ultrasound-guided percutaneous arterial embolization (PAE) [Citation8]. PAE was performed using 20-gauge catheter needles in 23 HCC patients with hypervascular tumors; the mean tumor size was 4.6 ± 1.2 cm. Colour Doppler ultrasonography showed effective embolization in 29 arteries. The complete necrosis rate after 1 month was 96.0%, and the 5-year survival rate was 25.0%. These findings indicated that PAE was a promising option for non-TACE candidate patients with a hypervascular HCC larger than 3 cm.
The second technique was named PAA and was the topic of the present study. In 2009, our center first reported that PAA effectively blocked the feeding artery of HCC and decreased tumor recurrence [Citation9]. The average tumor sizes in the PAA and non-PAA groups were 4.3 ± 1.1 cm and 4.1 ± 1.0 cm, respectively (p = .21). HCC recurrence rate at 6 months post-treatment was lower in the PAA group than in the non-PAA group (17.3% vs 31.37%, p = .04). After PAA, the feeding artery of the tumor was blocked to reduce blood flow to the tumor. Then, RFA was performed with less of a heat-sink effect, which was similar to the result obtained with TACE before RFA. Compared with other modalities, PAA can be performed immediately before tumor ablation without the need for drug injection or other instruments. In addition, Cheng et al. [Citation16] reported that PAA decreased local tumor progression in HCC patients with a <3-cm tumor (PAA vs non-PAA: 17.6% vs 48.6%, p = .038).
Following advancements in equipment, we aimed to improve the PAA method. First, 3D-CEUS was used to confirm the feeding artery in the present study. 3D-CEUS provides more details of the feeding artery than 2D-CEUS or color Doppler ultrasonography. Lee et al. [Citation8] compared the efficacy of 3D- and 2D-CEUS in detecting the feeding artery; the feeding artery could be demonstrated in 78.4% (29/37) of hepatic malignancy lesions by 3D-CEUS compared to 13.5% (5/37) of the lesions by 2D-CEUS (p < .001). Second, the PAA strategy has been modified with a new RFA technique. In the past, the artery was ablated with 2–3 overlapping ablation foci (2–3 cm in diameter each) in different directions or depths using expandable electrodes [Citation9]. Instead, two bipolar electrodes were inserted parallel to each side of the artery with a distance between the two needles of less than 1 cm, which made the operation simpler.
To the best of our knowledge, this study is the first clinical trial to compare the outcome between PAA + RFA and RFA alone. In the present study, local tumor progression was lower in the PAA + RFA group than in the RFA group (8.5% vs 21.3%, p = .082), although the technical success rates were similar (97.9% vs 91.5%, p = .203). This result indicated that the improved PAA method was also effective for hypervascular HCC, which was similar to the results of our previous studies.
Although this study was a prospective randomized trial, it was limited by the short-term follow-up and small patient sample. Multicenter randomized trials with long-term follow-up are warranted to confirm the role of PAA in the treatment strategy for HCC. Second, other modalities to reduce the blood flow, such as TACE, were not compared in this study. Therefore, whether PAA is superior or inferior to other modalities is still unclear.
In conclusion, PAA before RFA appears to be an effective and safe modality for the treatment of hypervascular HCC. This treatment strategy can be an alternative option for hypervascular HCC, with a lower local tumor progression rate than that of conventional RFA.
Disclosure statement
No potential conflict of interest was reported by the authors.
Additional information
Funding
References
- Torre LA, Bray F, Siegel RL, et al. Global cancer statistics, 2012. CA Cancer J Clin. 2015;65:87–108.
- Fitzmorris P, Shoreibah M, Anand BS, et al. Management of hepatocellular carcinoma. J Cancer Res Clin Oncol. 2015;141:861–876.
- Lehmann KS, Ritz JP, Valdeig S, et al. Ex situ quantification of the cooling effect of liver vessels on radiofrequency ablation. Langenbecks Arch Surg. 2009;394:475–481.
- Bitsch RG, Düx M, Helmberger T, et al. Effects of vascular perfusion on coagulation size in radiofrequency ablation of ex vivo perfused bovine livers. Invest Radiol. 2006;41:422–427.
- Lehmann KS, Poch FG, Rieder C, et al. Minimal vascular flows cause strong heat sink effects in hepatic radiofrequency ablation ex vivo. J Hepatobiliary Pancreat Sci. 2016;23:508–516.
- Peng ZW, Zhang YJ, Chen MS, et al. Radiofrequency ablation with or without transcatheter arterial chemoembolization in the treatment of hepatocellular carcinoma: a prospective randomized trial. J Clin Oncol. 2013;31:426–432.
- Peng ZW, Zhang YJ, Liang HH, et al. Recurrent hepatocellular carcinoma treated with sequential transcatheter arterial chemoembolization and RF ablation versus RF ablation alone: a prospective randomized trial. Radiology. 2012;262:689–700.
- Yang W, Yan K, Chen MH, et al. Preliminary experience with direct percutaneous arterial embolisation combined with radiofrequency ablation for hypervascular HCC. Int J Hyperthermia. 2017;33:1–845.
- Hou YB, Chen MH, Yan K, et al. Adjuvant percutaneous radiofrequency ablation of feeding artery of hepatocellular carcinoma before treatment. WJG. 2009;15:2638–2643.
- Chen MH, Yang W, Yan K, et al. Large liver tumors: protocol for radiofrequency ablation and its clinical application in 110 patients-mathematic model, overlapping mode, and electrode placement process. Radiology. 2004;232:260–271.
- Ahmed M, Solbiati L, Brace CL, et al. Image-guided tumor ablation: standardization of terminology and reporting criteria-a 10-year update. Radiology. 2014;273:241–260.
- Goldberg SN, Hahn PF, Tanabe KK, et al. Percutaneous radiofrequency tissue ablation: does perfusion-mediated tissue cooling limit coagulation necrosis? J Vasc Interv Radiol. 1998;9:101–111.
- Scott DJ, Fleming JB, Watumull LM, et al. The effect of hepatic inflow occlusion on laparoscopic radiofrequency ablation using simulated tumors. Surg Endosc. 2002;16:1286–1291.
- Park MH, Cho JS, Shin BS, et al. Comparison of internally cooled wet electrode and hepatic vascular inflow occlusion method for hepatic radiofrequency ablation. Gut Liver. 2012;6:471–475.
- Yan S, Xu D, Sun B. Combination of radiofrequency ablation with transarterial chemoembolization for hepatocellular carcinoma:a meta-analysis. Dig Dis Sci. 2013;58:2107–2113.
- Cheng YT, Jeng WJ, Lin CC, et al. Percutaneous radiofrequency ablation of tumor feeding artery before target tumor ablation may reduce local tumor progression in hepatocellular carcinoma. Biomed J. 2016;39:400–406.