ABSTRACT
Liquid crystals with their unique ordered mesophases and anisotropic physical properties, such as optical, magnetic and dielectric anisotropy, have found wide opto-electronic applications, especially liquid crystal displays (LCDs) in digital electronic devices, such as smart phones, tablets, laptops, TVs, etc. Liquid crystals incorporating 2,5-disubstituted pyrimidine rings in their aromatic cores are often used in commercial LCDs. They are also critical building blocks of liquid crystalline organic semiconductors designed for use in novel organic electronic devices, such as organic light-emitting diodes (OLEDs), organic photovoltaics (OPVs), sensors, etc. This review will focus specifically on pyrimidine-based liquid crystals, such as calamitic, bent, discotic, taper-shaped, small molecules, oligomers and polymers. It will highlight the relationships between molecular structure and liquid crystalline behaviour, i.e. mesomorphism of relevance to organic and opto-electronic applications. It will highlight the development, optimisation and innovation of liquid crystalline materials containing pyrimidine rings, thereby stimulating the potential for new structural design and applications, such as sensors, flexible electronics, 4D printing and subsequently opening up completely new markets for these advanced functional materials.
GRAPHIC ABSTRACT
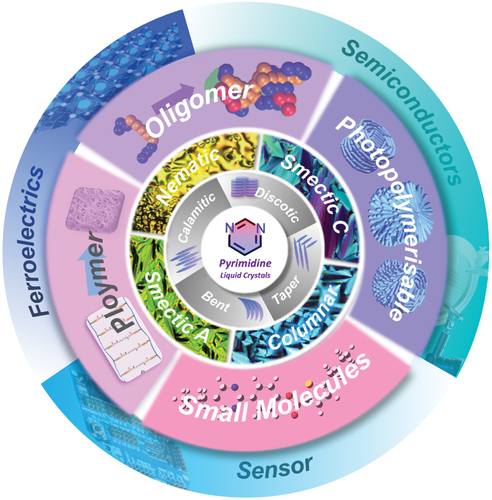
1. Introduction
Liquid crystals are currently an important phase of matter both scientifically and technologically. They are recognised as a stable phase exhibited by many compounds, thus putting them on equal footing to the solid, liquid, and gas phases. This situation is not a recent development and liquid crystals have been used in liquid crystalline displays (LCDs) for over 50 years [Citation1,Citation2]. Benefiting from the great commercial success of LCDs, liquid crystalline materials have attracted much attention from chemists and physicists in both academic and industrial fields. A variety of liquid crystal molecular structures have been designed and reported since the discovery of the first liquid crystal ‘cholesteryl benzoate’ by the Austrian botanist Friedrich Reinitzer in 1888 [Citation3,Citation4]. Undoubtedly, the major applications of liquid crystal materials are for displays. However, most of high-end active-matrix LCDs, such as TVs, computer screens, smartphones, pads, and so on, require nematic materials with low viscosity, medium birefringence (0.1), high resistivity, large dielectric anisotropy, and excellent stability [Citation5]. To fulfil above-mentioned properties, mixed liquid crystals such as fluorinated LC compounds, phenyl LC compounds, heterocyclic LC compounds, are commonly employed. But in this article, we stressed pyrimidine compounds as the reviewing perspective to give a summary of liquid crystal molecular structure, properties and application. gives the schematic exhibition of the review article structure.
Among these molecular structures, pyrimidine units containing two nitrogen atoms at C-1 and C-3 positions in a heterocyclic, six-membered aromatic ring, play a vital role in defining the mesophases and opto-electronic properties of liquid crystalline materials. The presence of heteroatoms, such as nitrogen (N), sulphur (S) and oxygen (O), for example, in aromatic rings and/or flexible chains, in the molecular structures of liquid crystals co-determines their mesogenic behaviour and other physical properties. These properties may differ to a significant degree from those of their corresponding non-heterocyclic analogues due, for example, to differences in polarisability and geometric shapes [Citation6–9]. Overall, the presence of mesophases is closely related to their intrinsic molecular structures, such as geometric shape, length-to-breadth ratio, nature and shape of their aromatic cores, nature, position and length of flexible chains, molecular polarity and polarisability, etc. The schematic exhibition is clearly shown in .
Figure 2. (Colour online) The schematic exhibition of structures and mesophases for pyrimidine liquid crystals based on geometric shape, flexible chains, molecular polarity and polarizability, etc.
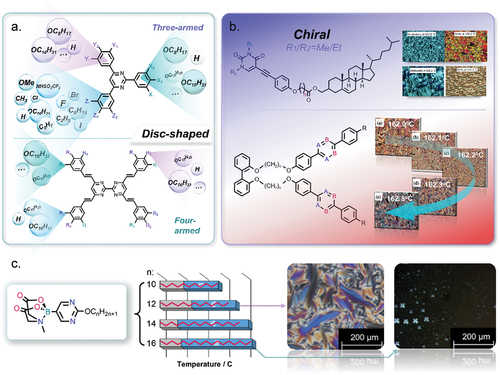
Upadhyay et al. [Citation10] compared the physical properties and mesomorphism of nitrogen-substituted liquid crystals and those of their non-heterocyclic analogues. They compared the values for the degree of polarisability, electronegativity, conjugation (delocalisation), HOMO-LUMO energy gaps and mesomorphic properties of 4-cyano-4’-pentylbiphenyl (5CB) with those of its nitrogen-containing analogues (see ). We can see clearly from that the pyrimidine compounds do significantly increase the melting temperature, but the effect of pyrimidine compounds on the melting temperature and solubility in eutectic mixtures can vary depending on specific conditions and the nature of the compounds involved. Pyrimidine compounds, which are nitrogen-containing heterocyclic aromatic molecules, may form stronger intermolecular interactions, such as hydrogen bonding or π-π stacking, with other components in a mixture. These interactions can lead to an increase in the melting temperature of the resulting eutectic mixture. The enhanced bonding between pyrimidine compounds and other components can raise the energy required to break these bonds during the melting process, effectively elevating the melting temperature. The relationship between melting temperature and solubility is intricate. In general, an increase in melting temperature may lead to a decrease in solubility, especially if the components of the eutectic mixture are not able to form strong interactions with the surrounding solvent or matrix. Higher melting temperatures often imply stronger cohesive forces between molecules, making it more difficult for the solute to dissolve. But if the pyrimidine compounds could form strong interactions with the solvent, such as hydrogen bonding, they would improve the solubility. Besides, when some branched alkyl chains are designed to construct the pyrimidine compounds, it will also decrease the melting points and solubility. Therefore, it is crucial to note that the behaviour of pyrimidine compounds can vary based on their specific chemical structure, as well as the other components present in the mixture. The solvent or matrix used for formulation also plays a significant role. The presence of functional groups and the overall molecular geometry of the pyrimidine compound can influence its interactions with other components, affecting both the melting temperature and solubility. In summary, while there is a tendency for pyrimidine compounds to increase melting temperatures and potentially decrease solubility in eutectic mixtures, the actual impact depends on the specific chemical context. Careful consideration of the molecular interactions and formulation conditions is necessary to predict and control these effects accurately. Schilling et al. [Citation11] reported a series of interesting boron liquid crystals containing heteroaryl rings, such as 2,5-disubstituted pyrimidine and pyridine rings (see ). Lai’s group [Citation12,Citation13] reported a new class of pyrimidine-based discotic liquid crystals exhibiting high clearing points and wide temperature ranges for their columnar mesophases (see ). The authors elucidated their structure–property relationships, such as the effects of the nature and position of polar groups in terms of electron-withdrawing effects, for example. The presence of the nitrogen atoms of the 2,5-disubstituted pyrimidine rings is considered to be a key factor that determines the enhanced mesomorphic properties with respect to their non-heterocyclic analogues. In addition, the presence of heterocyclic aromatic rings, such as the 2,5-disubstituted pyrimidine moiety, in disk-shaped molecules appears to favour the formation of columnar phases. Majumdar et al. [Citation14,Citation15] for example, studied the mesogenic behaviour of pyrimidine-based liquid crystals incorporating a cholesteryl ester with a number of chiral centres (see ). The presence of cholesterol-based molecular units in a liquid crystal can help stabilise frustrated fluid phases, such as blue phases and twist grain boundary phases.
Figure 3. (Colour online) The chemical structures, mesophase transition temperatures and POM textures of boron pyrimidine liquid crystals [Citation11].
![Figure 3. (Colour online) The chemical structures, mesophase transition temperatures and POM textures of boron pyrimidine liquid crystals [Citation11].](/cms/asset/9c223362-e3eb-46aa-a024-5ee9466f19d3/tlct_a_2361294_f0003_oc.jpg)
Figure 4. (Colour online) The chemical structures, mesophase transition temperatures and POM textures of cholesteric liquid crystals.
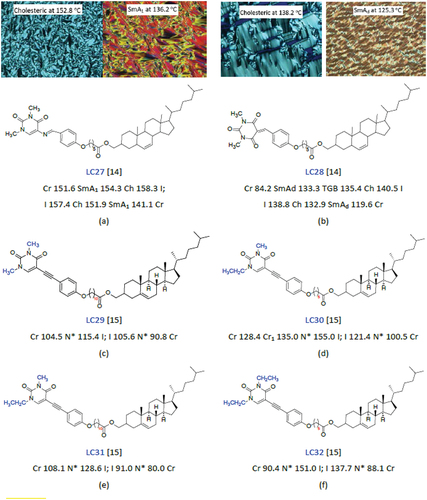
Table 1. The chemical structures and mesophase transition temperatures (oC) of nitrogen-substituted 5CB derivatives.
Table 2. The chemical structures and mesophase transition temperatures (oC) of discotic pyrimidine liquid crystals [Citation12,Citation13].
The chiral nematic, smectic A and smectic C phases are important mesophases for the realisation and operation of various electro-optical devices. A wide range of liquid crystals incorporating aromatic, six-membered heterocyclic rings, especially 2,5-disubstituted pyrimidine and pyridine rings, have found broad application in ferroelectric LCDs, organic semiconductors, sensors, etc. Nishiyama et al. [Citation16] reported a new class of laterally connected liquid crystals containing 2,5-disubstituted pyrimidine rings, which exhibit biaxiality in the nematic phase (see ). The pre-organisation effect on chirality, polarity and biaxiality in liquid crystals has been discussed in terms of their influence on the physical properties of mesogens incorporating these elements. Camerel’s group [Citation17], for example, reported a series of bipyrimidine-based discotic liquid crystals with an unusual combination of luminescent and nonlinear optical properties (see ). Detailed structure–property relationships were established using a combination of DFT calculations and solid-state temperature-dependent infrared spectroscopy [Citation18]. However, one of the most important applications for liquid crystals incorporating 2,5-disubstituted pyrimidine rings is in commercial ferroelectric liquid crystal displays (FLCDs). FLCDs possess an advantageous combination of electrooptic properties, such as a very fast opto-electronic response, hysteresis switching behaviour, in-plane switching of the optic axis, high optical contrast, wide viewing angles, low power consumption, sequential colours and inherent bistability [Citation19,Citation20]. Ferroelectric liquid crystals with a reduced molecular symmetry due to the presence of a chiral centre exhibit a spontaneous polarisation (Ps) in the absence of an external field. However, on application of an electric field, the direction of polarisation can be reoriented [Citation21]. Liquid crystals incorporating a 2,5-disubstituted pyrimidine unit have often been found to exhibit a smectic C mesophase. The presence of an optically active chiral centre in such molecular structures then gives rise to liquid crystals with chiral smectic C mesophases (SmC*) with macroscopic ferroelectric properties [Citation19,Citation22].
Figure 5. (Colour online) The chemical structures, mesophase transition temperatures and POM textures of laterally connected pyrimidine liquid crystals [Citation16].
![Figure 5. (Colour online) The chemical structures, mesophase transition temperatures and POM textures of laterally connected pyrimidine liquid crystals [Citation16].](/cms/asset/66860b88-df3c-45c0-b0fe-92b0e9fc7bde/tlct_a_2361294_f0005_oc.jpg)
Table 3. The chemical structures and mesophase transition temperatures (oC) of bipyrimidine discotic liquid crystals.
One 2,5-disubstituted pyrimidine ring and usually several such heterocyclic rings are often present in highly conjugated organic semiconductors for application in plastic electronic devices, such as organic light-emitting diodes (OLEDs) [Citation23–28], organic photovoltaics (OPVs) [Citation29–31], organic field-effect transistors (OFETs), etc [Citation32–34]. Such heterocyclic mesogens, with an extended conjugated backbone exhibit strong intermolecular π-π stacking with overlapping overlapping molecular orbitals, which affords the high charge-carrier mobility required for the operation of such plastic electronic devices. The polarised nature of 2,5-disubstituted pyrimidine rings, as a so-called electron-withdrawing unit, facilitates the design of highly conjugated ‘push-pull’ molecules with efficient intra-molecular charge transfer from the electron-donating molecular units with high-lying HOMO energy levels to electron-withdrawing units with low-lying LUMO energy levels. This donor-acceptor (D-A) molecular design promotes effective charge-transport mobility in oriented thin layers in a planar device configuration [Citation35–38]. Such heterocyclic liquid crystalline semiconductors have been synthesised with a combination of physical properties tailored for various plastic electronic devices. These properties are a complex combination of mesophase types, transition temperatures, charge carrier mobility, electroluminescent properties, etc.
Pyrimidine, also known as 1,3-diazine, can be found in many naturally occurring products, such as thymine, cytosine and uracil. Such heterocyclic compounds often exhibit a high biological activity of relevance for their use as pharmaceuticals, herbicides, antimicrobial, antibiotics, anticancer drugs, etc [Citation39–41]. The presence of two nitrogen atoms in the aromatic cores of such molecules affords the possibility of protonation, metal ion chelation and hydrogen bonding, etc. Heterocyclic liquid crystals are of significant potential importance due to the formation of thin, uniform layers of highly conjugated supramolecular assemblies. Such mesogens, especially incorporating at least one 2,5-disubstituted pyrimidine unit, have facilitated the development of a new generation of chemical and biological sensors in recent years [Citation42–44].
LCDs have become a part of our daily life, from wristwatches and pocket calculators to displays in all kinds of instruments, including portable computers, tablets, mobile phones and flat-panel televisions. The advantages of LCDs comprise low power consumption, low-voltage operation, full-colour, images at video rate with wide viewing angles, high contrast, thinness, and low weight. LCDs have established themselves as the dominant flat panel display over the last five decades, primarily due to this advantageous combination of attractive properties, but combined with scalability to very large screens and being cost competitive with competing technologies. However, research and development into liquid crystal technologies have taken them way beyond just the field of flat-panel displays. Scientifically, liquid crystals play an essential role in interdisciplinary material science research, involving chemistry, physics, mathematics, engineering, biology, etc., for a wide range of applications, such as photochromics [Citation45–47], organic semiconductors for use in opto-electronic devices [Citation48–50], 3D/4D printing materials [Citation51–54], etc. The aromatic 2,5-disubstituted pyrimidine ring also plays an essential role in the molecular structure of novel liquid crystalline materials for this wide range of applications. For example, Ryu et al. [Citation55,Citation56] used phenyl pyrimidines to study the relationship between thermoelastic anisotropy and liquid crystalline mesophases in terms of achieving structural stability and efficient heat dissipation, especially for novel high-performance LCDs incorporating 4D-printed liquid crystalline elastomers for high-frequency applications.
However, there are only a few recent review articles are available summarising the nature and properties of pyrimidine-based liquid crystals from the perspective of molecular structures, mesophases transition and their applications. Petrov [Citation57] has published a review article on pyrimidine as a structural fragment in calamitic, i.e. rod-like, liquid crystals. The author studied the relationship between the presence of the 2,5-disubstituted pyrimidine ring in the molecular structure of a wide range of calamitic liquid crystals on the nature and temperature ranges of their mesophases and their physicochemical and electro-optical properties. The article mainly focused on calamitic liquid crystals with linear bi-aryl and tri-aryl structures. However, since the publication of this review in 2006, the family of pyrimidine-based liquid crystals with a wide range of molecular structures, such as bent, discotic, taper-shaped, polymers, highly conjugated poly-aryl calamitics, and metal coordinated mesogens, has been very significantly extended and expanded for a wide range of applications in the intervening years. Achelle and Plé [Citation58] also briefly reviewed pyrimidine-based conjugated materials for opto-electronic applications in 2012 as part of a broad, general review of the synthesis of a wide range of 2,5-disubstituted pyrimidine derivatives. It proves the importance of pyrimidine rings in constructing organic semiconductors with highly conjugated aromatic cores for novel application in plastic electronics, such as OFET, OPV and OLED. Ghosh et al. [Citation59] also published a review on heterocycle-based calamitics in 2017. The authors briefly introduced many kinds of heterocyclic calamitic liquid crystals including five- and six-membered rings as well as fused aromatic rings. Therefore, we consider that a comprehensive review article, focused specifically on heterocyclic pyrimidine-based liquid crystals, would address a very significant and important knowledge gap. It should summarise the complex relationships between the molecular structures and the nature of their mesophases and their transition temperatures of relevance to specific opto-electronic applications. We make the case in this review article that innovation in terms of the synthesis of novel liquid crystalline materials containing pyrimidine rings will promote the rapid development of developing applications, such as 3D/4D printing, and flexible electronics facilitated by these advanced functional materials.
2. Pyrimidine-based liquid crystals for ferroelectric liquid crystal displays (FLCDs)
shows the schematic exhibition of pyrimidine-based liquid crystalline structures and mesophases for ferroelectric application in this section where it gives an overview of the structural development of pyrimidine-based liquid crystals specifically designed to exhibit chiral smectic C phases (SmC*) with ferroelectric properties for application in commercial FLCDs. This summary should help readers better understand the structure–property relationships of pyrimidine-based liquid crystals and stimulate the design and synthesis of novel liquid crystals for FLCDs with improved performance and also for new applications of ferroelectric mesogens.
Figure 6. (Colour online) The schematic exhibition of pyrimidine-based liquid crystalline structures and mesophases for ferroelectric application.
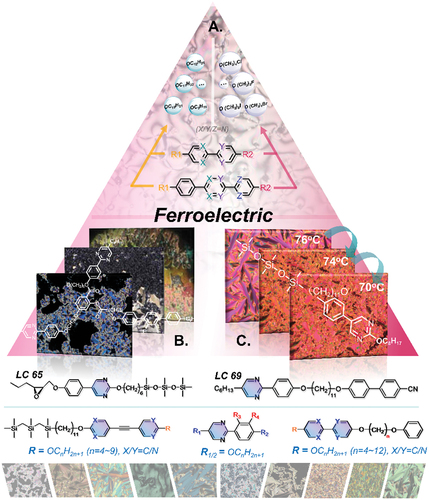
Liquid crystal displays (LCDs) have dominated the market of flat panel display (FDP) for many years due to their low cost, high resolution, long life, sophisticated processing technology and material system. However, current commercial LCDs using nematic liquid crystal mixtures still exist with potential issues of latency, image sticking and energy efficiency [Citation60]. Although organic light emitting diode (OLEDs) displays exhibit many advantages due to the absence of backlight and are taking market share from LCDs slowly, the limitations of lifetime, large size, relatively high power consumption, flaw and process yields, etc., have presented some challenges in terms of market acceptability and market share in the flat panel display industry. FLCDs represent a promising alternative to nematic LCDs in meeting current challenges in flat panel display market due to their unique characteristics, such as high optical contrast ratio, high response, memory effect, large birefringence, wide viewing angle, low threshold voltage, low power consumption, and inherent bistability [Citation20,Citation60–62].
Meyer et al. [Citation63] synthesised the liquid crystal 4-decyloxybenzylidene-4’-amino-2-methylbutylcinnamate in 1975 and reported the ferroelectric properties of a SmC* phase for the first time. Clark and Largerwall [Citation64] reported the first FLCD using a liquid crystal mixture in the SmC* phase in 1980. These developments stimulated significant research interest and activities in the design and synthesis of SmC liquid crystals (hosts), optically active dopants (guests) and (guest/host) SmC*mixtures for the development of prototype FLCDs. The presence of an heteroatom, such as nitrogen, sulphur and oxygen, in one or more of the aromatic rings in the molecular core of these mesogens was found to promote the formation of a SmC phase often with a low viscosity [Citation21]. Such guest/host mixtures were designed to manifest a mesophase sequence of Iso−N*−SmA*−SmC* on cooling features required for good defect-free alignment in FLCDs [Citation21]. One of the most commonly encountered heterocyclic rings in the aromatic core of such mesogens are 2,5-disubstituted pyrimidine and pyridine rings. Suitably substituted pyrimidine-based liquid crystals often have fairly low melting points and reasonably high SmC transition temperatures, i.e. a reasonably broad SmC mesophase. The design of novel organic materials with both SmA and SmC phases required for application in FLCDs mainly focussed on the modifications of the rigid aromatic cores and flexible alkyl chains because they can affect lamellar (smectic) ordering, van der Waals interactions and thus mesogenic properties [Citation65,Citation66]. Two- and three-ring phenyl-pyrimidine structures were mostly studied for use as ferroelectric liquid crystals due to the presence of appropriate mesophases and transition temperatures. Tykarska et al. [Citation67] reported a series of six ferroelectric liquid crystals containing phenyl pyrimidine cores and alkyl/alkoxy chains with different lengths as constituent components of liquid crystalline mixtures with the desired mesophases and temperature ranges (see ). These smectic liquid crystals were also utilised to prepare different ferroelectric mixtures with dispersed nano-sized copper oxide for improved dielectric and electro-optical parameters [Citation73]. Tykarska et al. reported that a longer alkoxy terminal chain attached to the pyrimidine ring or the presence of two alkoxy chains increases the tilt angle in smectic layers and produces high transition temperatures, e.g. SmC-SmA and SmA-I, for their requisite smectic mesophases. A value of 22.5° for title angle of the director of a smectic C phase is required for FLCDs and other applications in this article. Debnath et al. made use of related optically active, but non-mesogenic, dopants for preparing room-temperature ferroelectric liquid crystal mixtures suitable for FLCD applications [Citation74]. Chakraborty et al. [Citation75] used one of these materials to investigate the critical behaviour at mesophase transitions by exploiting high-resolution optical birefringence (Δn) measurements with a high degree of accuracy. LCDs based on nematic LCs require a birefringence value of between 0.05 and 0.10 in order to minimise variations in the chromatic retardance [Citation76]. The birefringence of liquid crystals is determined by their anisotropy of polarisability, which depends on a high degree of aromatic conjugation within the aromatic core. Pozhidaev et al. [Citation76] introduced trans-1,4-disubstituted cyclohexane rings into achiral liquid crystals designed for application in a range of different types of ferroelectric liquid crystal devices to study this effect. These authors used fluorinated phenylpyrimidine derivatives as chiral dopants in these studies in order to adjust the electro-optical parameters, such as the spontaneous polarisation (Ps) and the pitch of the smectic C* helix [Citation76,Citation77]. Such liquid crystal mixtures with a low value of the birefringence are suitable for most ferroelectric applications, such as surface-stabilised ferroelectric liquid crystal displays (SSFLCDs), deformed helix ferroelectric displays (DHFDs) and electrically suppressed helix displays (ESHDs).
Table 4. The chemical structures and mesophase transition temperatures (oC) of phenyl pyrimidine liquid crystals.
Pramanik et al. [Citation68] reported three binary types of liquid crystal mixtures created by mixing an achiral phenyl pyrimidine smectic compound with three different orthoconic anti-ferroelectric (SmCA*) analogues exhibiting a mesophase sequence of SmA*-SmC*-SmCA* (see ). FLCDs based on orthoconic anti-ferroelectric liquid crystals have attracted much attention due to their fast response times, wide viewing angles, ideal dark state, broad grey scale and absence of substrate surface treatment. However, these mixtures exhibit high viscosity values for the SmC* and SmCA* mesophases, which leads to unacceptably long response times for commercially viable SSFLCDs. Therefore, pyrimidine-based achiral liquid crystals with a low viscosity are often used to dope chiral orthoconic anti-ferroelectric liquid crystals, which possess high Ps values, in order to produce the desired electro-optical properties of the resulting mixtures. For example, this doping procedure often produces mixtures with a low spontaneous polarisation, long pitch and very short response times for use in high-speed devices. However, low Ps values and poor mechanical stability result in a quite challenging task in terms of optimisation of these liquid crystal mixtures for commercial FLCDs. Generally, the presence of a higher number of aromatic rings in the aromatic cores of liquid crystalline compounds results in higher viscosity values as well as higher liquid crystal transition temperatures. Lapanik et al. [Citation20] recently reported new achiral smectic compounds with four or five aromatic rings in the aromatic core to induce shock-stable FLCDs with improved electro-optical properties (see ). However, in order to reduce the viscosity of such host SmC mixtures for fast switching FLCDs, the authors utilised additional low-viscosity achiral phenylpyrimidine derivatives and doped them with chiral dopants with a high Ps value.
Czerwiński et al. [Citation19] reported new ferroelectric liquid crystal mixtures of achiral two-ring pyrimidine compounds as parent mixture and chiral dopants. These mixtures show a stable enantiotropic ferroelectric phase with very good alignment in surface-stabilised FLCDs. The authors discussed the dependence of the ferroelectric properties, such as dipolar and multipolar interactions, of these materials on their molecular parameters. The study results show that the limited dipolar interactions in favour of quadrupolar ones are a feature observed previously for materials comprising chiral dopant with two chiral centres what is in contrast to mixtures with one chiral centre, where a linear relationship is observed. The structure–property relationship is still as complex as the influence of molecular parameters on the dipolar and multipolar interactions within FLC. Authors proved that an extended analysis of structure–property relation is an excellent tool to improve the knowledge of molecular interactions in LC media and is an advanced tool for LC media materials engineering. This group also used chiral dopants with pyrimidine rings in order to investigate the influence of the dopant structures on ferroelectric properties, such as title angle, spontaneous polarisation and layer spacing [Citation78]. However, some challenges still remain in terms of commercial SSFLCDs in terms of defect-free alignment, zero or a minimal layer shrinkage, wide mesophase temperature ranges, etc [Citation79]. An important development is the potential use of ‘de Vries’ smectic liquid crystals, which exhibit a maximum layer contraction of ≤ 1% upon transition from the SmA* phase to the titled SmC* phase and within the whole temperature range of the SmC* phase [Citation79]. Pyrimidine-based liquid crystals possess intrinsic structural advantages for such ‘de Vries’ smectic liquid crystals with a small layer shrinkage and wide mesophase temperatures [Citation21]. The Vij group reported such a series of ‘de Vries’ liquid crystals containing pyrimidine rings for ferroelectric applications with a small layer shrinkage and a fast electro-optical switching time [Citation60,Citation79–81]. They designed and synthesised two 5-phenyl pyrimidine epoxy derivatives with different either trisiloxane (LC65) or perfluorinated (LC66) terminal chains [Citation79], see . The trisiloxane (LC65) was found to be a good ‘de Vries’ smectic liquid crystal as it exhibited both SmA* and SmC* phases with broad mesophase temperature ranges, a small layer shrinkage with 1.7% at 13°C below the SmA*-SmC* transition and a reduction factor with 0.37 [Citation79]. The group further synthesised two new pyrimidine-based de Vries smectic liquid crystals with siloxane-terminal chains, LC74 and LC75 (see ), exhibiting wide temperature ranges for the SmA* and SmC* phases and fast electro-optical switching [Citation60]. These two compounds exhibit a low layer shrinkage of ca. 1.5% in the SmC* phase, extremely fast electronic response and a large, field-induced birefringence in the SmA* phase. Compound LC75 possesses a wide mesophase temperature range, thus making it highly suitable for FLCDs [Citation60,Citation80]. Furthermore, the group also synthesised LCs with carbosilane sidechains instead of siloxane terminal groups to study their de Vries properties [Citation81]. The phenylpyrimidine LCs, LC76 and LC77, with tri- and tetra-carbosilane terminal groups, respectively, exhibit good ‘de Vries’ characteristics with a set of phase-transitions of Iso-SmA*-SmC*-SmX-Cr (see ). Compound LC77, with a tetra-carbosilane chain, exhibits a smaller layer shrinkage (0.9%) compared with that (1.9%) of LC76 with a shorter tri-carbosilane chain. Therefore, the authors postulate that an even longer carbosilane chain could lead to a further reduction or even to zero-layer shrinkage. In addition, the authors replaced the methyl group in the terminal chains of compounds LC76 and LC77 with a perfluoromethyl group to give two new phenylpyrimidine compounds (NLC9 and NLC10). Unfortunately, these new materials do not exhibit any mesophases (see ). These results demonstrate once more that heteroatoms present in molecular structures of LCs can significantly influence the molecular polarity and thus the exhibition of liquid crystal mesophases.
Table 5. The chemical structures and mesophase transition temperatures (oC) of pyrimidine-based liquid crystals with epoxy [Citation79], branched tricarbosilane [Citation82], bent-core dimers [Citation83], taper-shaped trimers [Citation84].
Table 6. The chemical structures and mesophase transition temperatures (oC) of biphenyl pyrimidine compounds with siloxane/carbosilane terminal chains.
The Lemieux group has reported a wide variety of de Vries smectic liquid crystals with phenylpyrimidine cores and siloxane/carbosilane terminal chains for many years [Citation69,Citation85–88]. The design of these new materials is based on de Vries characteristics requiring the presence of both SmA* and SmC* phases, a small layer shrinkage at their transition, and a broad mesophase temperature range. An organosiloxane- or carbosilane-terminated chain promotes the exhibition of a SmC phase and a phenylpyrimidine core often induces the formation of a SmA phase [Citation69,Citation85,Citation86]. Lemieux’s group used the presence of organosiloxane terminal chains in compounds designed to exhibit de Vries smectic mesophases (see ). The compounds 3(n) (see ) exhibit a maximum layer contractions ranging from 0.5% to 1.4% [Citation85]. These values are comparable to those of the classic de Vries LCs 3 M 8422 and TSiKN65 with layer contractions of 0.4% and 0.65%, respectively. In addition, the authors also studied the effect of halogen end-groups on promoting the formation of a SmA phase in phenylpyrimidine LCs [Citation87] (see ). Fluorinated phenylpyrimidine derivatives were widely studied in the 1990s in terms of smectic layer contraction and related ferroelectric properties [Citation70,Citation71,Citation77,Citation91,Citation92] with some ongoing research interest [Citation93,Citation94]. Lemieux’s group mainly focuses on LCs with chloro-groups in terminal positions in the expectation of promoting the presence of an SmA phase due to the electron-withdrawing nature of chloro end-groups reducing electrostatic repulsion between the alkoxyl chains and increasing van der Waals interactions between the aromatic cores [Citation87]. Therefore, the authors designed and synthesised a series of phenylpyrimidine de Vries LCs containing organosiloxane-terminated chains and chloro-substituted alkoxyl chains (see ). These novel compounds exhibit the desired SmA* and SmC* phases with excellent alignment and electro-optical properties as ferroelectric liquid crystals [Citation88]. However, in order to avoid the hydrolytic instability of siloxane structures [Citation95], chemically inert carbosilane-terminated groups were introduced into similar molecular structures to provide a novel series of de Vries liquid crystals [Citation82,Citation89,Citation90,Citation95–99] (see ). The compounds LC81 and LC91 (see ) exhibit broad SmC mesophases and reduction factors R of 0.17 and 0.18, respectively, for potential use as chevron-free ferroelectric liquid crystals [Citation89]. These results illustrate the fact that the presence of carbosilane-terminal chains do not dramatically change the mesomorphism and de Vries properties compared to those of analogous compounds with siloxane-terminated chains [Citation89]. The title angle of these materials could be controlled by modifying the length of carbosilane chains without compromising ‘de Vries’ properties. The analogous compounds LC85-LC90 (see ) with an inverted orientation of the nitrogen atoms in the pyrimidine rings cause a suppression of de Vries properties possibly due to non-covalent, core–core interactions in the intercalated smectic bilayers [Citation89]. The group also synthesised de Vries liquid crystals, such as LC67 and LC68 (see ), with branched carbosilane end groups [Citation82]. These compounds exhibit a comparable mesomorphic behaviour and de Vries properties to those of the analogous compounds with linear tricarbosilane chains [Citation82]. However, the presence of a mono-carbosilane chain, rather than a tri-carbosilane chain, results in significantly lower de Vries properties with an increase in the reduction factor R possibly due to a reduction in the electroclinic effect (ECE), nano-segregation and the quality of the lamellar ordering [Citation90,Citation97]. The chiral compound LC94 (see ) with a long tricarbosilane-terminated chain exhibits a maximum layer contraction of only 0.2% and shows no appreciable optical stripe defects due to horizontal chevron formation [Citation90,Citation99]. In addition to flexible siloxane/carbosilane-terminated chains, the group also investigated the influence of rigid phenoxy end-groups in a terminal position of LCs on their mesomorphic behaviour [Citation65]. These studies show that the presence of the phenoxy end group (LC148-LC160, NLC13-NLC17) causes a significant increase in melting point and inhibits, at least partially, the mesomorphism of these materials (see ). In most cases, the broad enantiotropic SmC phase exhibited by the parent isomers is suppressed in the analogous compounds with a phenoxy end group (LC148-LC160, NLC13-NLC17) [Citation65]. In addition, the group also studied the influence of the ethynyl spacer between aromatic rings on de Vries properties [Citation96] (see ). These studies confirm the assumption that an ethynyl spacer would create more free volume in the core sublayer and this would consequently reduce the orientational order parameter S2 in the SmA mesophase of such compounds [Citation96]. On the other hand, the ethynyl spacer could be unstable under UV exposure and the process is known as UV-induced or photochemical polymerisation [Citation100]. The carbon–carbon triple bond is composed of one σ bond and two π bonds. The presence of multiple π bonds makes alkynes reactive and susceptible to undergoing polymerisation reactions. Ultraviolet light provides the energy needed to break the π bonds in the alkyne. The energy from UV light is absorbed by the π electrons, promoting them to higher energy levels and leading to the weakening or breaking of the π bond. It is important to note that the specific conditions, such as initiators, temperatures and UV wavelength (energy), are required to initiate or prevent the polymerisation of the ethynyl spacers.
Figure 7. (Colour online) The chemical structures, mesophase transition temperatures (oC) and POM textures of 2(n) and 3(n) series of liquid crystals [Citation85].
![Figure 7. (Colour online) The chemical structures, mesophase transition temperatures (oC) and POM textures of 2(n) and 3(n) series of liquid crystals [Citation85].](/cms/asset/791d06bd-99f8-4504-83b5-9750798dc551/tlct_a_2361294_f0007_oc.jpg)
Table 7. The chemical structures and mesophase transition temperatures (oC) of phenyl-pyrimidine liquid crystals with siloxane/carbosilane terminal chains.
Table 8. Effect of halogen end-groups with fluoro-, bromo-, iodo-substitution on mesophases and their transition temperatures for phenyl-pyrimidine liquid crystals.
Table 9. The chemical structures and mesophase transition temperatures (oC) of tricarbosilane-terminated phenylpyrimidine with ethynyl spacer.
Table 10. The chemical structures and mesophase transition temperatures (oC) of pyrimidine-based liquid crystals with phenoxy end groups.
Nanocomposites consisting of liquid crystals and nanoparticles have attracted a great deal of attention in recent years due to the combination of liquid crystal properties, such as excellent electro-optical behaviour, and quantum-confined properties of nanoparticles [Citation101–104]. Romero-Hasler et al. [Citation72] reported that a nanocomposite consisting of a ferroelectric liquid crystal and magnetic FeCo nanoparticles exhibits a high magnetic response to a very small applied electric field. The authors chose a phenylpyrimidine-based fluorinated compound with a chiral smectic phase (SmC*) (see ) as the ferroelectric material. More detailed studies are still needed to systematically investigate the relationship between the physical properties of the liquid crystalline (host) compounds and the optical, electrical, magnetic properties of the (guest) nanoparticles on the resultant (guest/host) nanocomposite.
The presence of heteroatoms in the aromatic cores of LCs exerts a strong influence on their mesogenic properties and other physical properties, such as birefringence, dielectric anisotropy, etc [Citation105–108]. Wilson’s group [Citation105,Citation109–111] synthesised heterocyclic ferroelectric LCs with a 2,5-disubstituted thiophene and a 2,5-disubstituted pyrimidine ring in the aromatic molecular core (see ). The 2,5-disubstituted thiophene ring produces a non-linear, i.e. bent aromatic core of LCs with, for example, an anti-ferroelectric SmC phase, low melting points and broad mesophase transition temperatures. It should be noted that the compounds with benzyloxy end-groups were first designed as intermediate materials for the synthesis of a variety of dialkyl thiophene-pyrimidine liquid crystalline materials by removing the protecting group, but the intermediates also exhibit mesophases [Citation109] (see ). Yoshizawa’s group [Citation83] also reported bent liquid crystal dimers consisting of two mesogenic moieties joined by an aliphatic linkage (see ). These compounds also exhibit antiferroelectric SmC* mesophases as well as a nematic phase. This combination is favourable for fabricating antiferroelectric LCDs without defects, which are often produced on the direct-phase transition from an isotropic liquid to the SmC* phase [Citation83]. In order to identify the relationship of molecular structures and antiferroelectric mesophases, Yoshizawa’s group [Citation84] synthesised a series of taper-shaped LC trimers containing 2,5-disubstituted pyrimidine rings, which exhibit antiferroelectric SmC phases (see ). The taper shape is similar to a combination of linear and bent molecular structures. These taper-shaped molecules can form an intercalated structure due to the intermolecular core–core interactions. The intercalated head-to-tail dipole–dipole interactions between phenyl pyrimidine cores help stablise the SmA mesophases. However, the competition between intercalated dipole–dipole interactions and mono-layer packing entropy effects appears to promote the presence of a SmC phase. The transition of the antiferroelectric SmC* phase to the chiral SmC* phase might be driven by conformational change and the molecular reorganisation caused by packing entropy factors due to the bent molecular shape of these compounds [Citation84]. In addition, the group also studied the effect of the presence of two chiral centres in phenylpyrimidine LCs on their mesomorphic behaviour and electro-optical parameters from the perspective of structure–property relationships [Citation112–114] (see ).
Table 11. The chemical structures and mesophase transition temperatures (oC) of thienyl-phenyl-pyrimidine compounds.
Table 12. The chemical structures and mesophase transition temperatures (oC) of thienyl-pyrimidine liquid crystals.
Table 13. The chemical structures and mesophase transition temperatures (oC) of phenyl pyrimidine liquid crystals with chiral centres.
Although recent decades have witnessed significant progress in the synthesis and study of novel ferroelectric liquid crystals with optimised mesomorphic and other physical properties, FLCDs have not been mass-produced on a very large scale [Citation21]. However, the ever-increasing requirement for future display technologies with very much greater information density combined with very fast response rates renders FLCDs of ongoing commercial interest in terms of R&D. This is especially the case for related fast switching electro-optical devices, such as spatial light modulators (SLMs), telecommunications switching, optical recognition, optical computing, etc [Citation21]. Therefore, one of the main goals of this review article is to promote the rapid development of novel ferroelectric LCs and, especially, those based on phenylpyrimidine-based derivatives.
3. Pyrimidine-based liquid crystals for organic semiconductors
gives the schematic exhibition of pyrimidine-based polycatenar oligomers, photopolymerisable liquid crystals and copolymers for application in organic semiconductors. Organic molecules are usually regarded as insulators, but compounds with highly conjugated aromatic molecular cores possess a conjugated electron cloud with a high degree of delocalisation, and may exhibit semiconductor properties. However, for most of the organic semiconductors used for OLEDs, OPVs and OFETs, the hole mobility exhibits several orders of higher than electron mobility, so that the unbalanced electron-hole recombination in the active layer often results in poor quantum efficiency [Citation115]. So in order to create more efficient devices, the organic materials with high electron mobility are demanded. Therefore, many highly conjugated heteroaromatic compounds have been studied as organic semiconductors due to a combination of relatively high electron mobility and appropriate morphology, such as the glassy state at and above room temperature [Citation22,Citation115–117].
Figure 8. (Colour online) The schematic exhibition of pyrimidine-based polycatenar oligomers, photopolymerisable liquid crystals and copolymers for application in organic semiconductors.
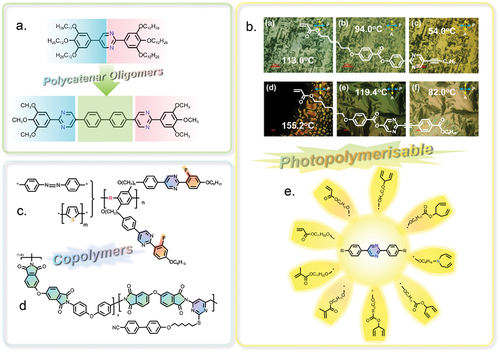
Liquid crystalline semiconductors have attracted much attention due to a favourable combination of mesophases, such as nematic, smectic and columnar (discotic), spontaneous thin-film formation with homogeneous alignment on planar surfaces, high charge transport, efficient photoluminescence and electroluminescence, etc. Columnar liquid crystals first attracted interest as ordered organic semiconductors with high charge carrier mobility attributable to their highly conjugated discotic aromatic cores being arranged above each other, with overlapping π-electron orbitals, in fluid columns of ordered discs that facilitate charge mobility [Citation118–120]. Smectic liquid crystals also have an ordered structure comprised of layers of molecules with a rod-like, calamitic structure, so they have also often been studied as potential organic semiconductors for use in plastic electronics [Citation121–123]. Nematic liquid crystals possess orientational order and no positional order, so they exhibit the least ordered structure and low viscosity among these mesophases [Citation124–127]. However, their relatively low viscosity facilitates their macroscopical alignment as thin films at room temperature for application in OLEDs, OPVs and OFETs. Hence, a wide range of different liquid crystalline semiconductors exhibiting nematic, smectic or columnar phases have been widely reported for use as charge-transporting and/or luminescent materials in recent years [Citation128–131]. However, liquid crystalline semiconductors containing 2,5-disubstituted pyrimidine rings have rarely been reported for application in OLEDs, OPVs or OFETs. Liquid crystals for FLCDs usually consist of two or three aromatic rings in their molecular core. In contrast organic semiconductors require larger aromatic cores to facilitate a high degree of electron delocalisation. Therefore, aromatic oligomers and polymers with large aromatic cores have been designed for use as organic semiconductors. Tong et al. [Citation132] reported copolymers containing pyrimidine-based aromatic cores (see ). Such compounds can exhibit good optical transparency, thermal stability and mechanical properties, e.g. for use as alignment layers with enhanced anchoring energy in LCDs. The Goto group at the University of Tsukuba reported a series of highly conjugated liquid crystalline oligomers and polymers containing pyrimidine rings. The group reported a low-bandgap liquid crystalline conjugated polymer (see ) for potential use as a polarising emission material in the near-infrared spectroscopy (NIR) range [Citation133]. The liquid crystal polymer incorporated phenyl pyrimidine-based moieties as side chains to generate mesophases and heterocyclic thiophene derivatives in the main chain to afford high conjugation and low bandgap. However, although the characteristic mesophase textures from string-like to large fan-shaped domain upon cooling have been observed by polarised optical microscopy (POM), DSC analysis did not give a clear transition signal [Citation133]. Other liquid crystalline polymers consisting of a highly conjugated phenylene-thiophene main chain and pyrimidine-based side chains have also been reported by the group [Citation134,Citation135] (see ). Interestingly, the group also studied the influence of a chiral nematic liquid crystal as a reaction medium in terms of induction of chiral properties in the polymers [Citation136,Citation138] (see ). In addition, the azobenzene unit was also introduced to the main chain of the liquid crystalline polymers to replace the thiophene core, giving rise to photoisomerisation of the polymers [Citation137] (see ).
Table 14. The chemical structures of pyrimidine-based copolymers.
Polymerisable liquid crystalline monomers have attracted much attention for application in some opto-electronic devices, such as augmented reality and virtual reality including AR/VR displays [Citation139], due to the combined advantages of polymers and low-molecular-weight materials [Citation140–144]. Lee’s group designed two calamitic liquid crystal monomers containing photopolymerisable acrylate groups and pyrimidine rings (see ) for application as host materials in guest-host polarisers [Citation145]. Rahman et al. reported a series of pyrimidine-based bent-core liquid crystal monomers (see ) for use as photo-switchable devices [Citation45]. The Kelly and O’Neill group at the University of Hull reported many photopolymerisable liquid crystals for application in OLEDs, OPVs and OFETs [Citation130]. They studied a series of pyrimidine-based reactive mesogens exhibiting a SmC phase as electron transporting materials. Different photopolymerisable end groups were also investigated in terms of their effect on the mesomorphic behaviour, transition temperatures and cross-linking efficiency [Citation146–148] (see ). Kelly and co-workers also studied a wide series of the phenylpyrimidine-based compounds with polymerisable vinyl groups at Roche in the 1990s [Citation146]. They mainly investigated the influence of the positions and length of C-C double bonds on their mesomorphic behaviour [Citation149]. They also reported polycatenar oligomers containing pyrimidine rings (see ) with columnar phases for use as high charge carriers [Citation150].
Figure 9. (Colour online) The chemical structures, mesophase transition temperatures (oC) and POM textures of photopolymerisable pyrimidine liquid crystals [Citation145].
![Figure 9. (Colour online) The chemical structures, mesophase transition temperatures (oC) and POM textures of photopolymerisable pyrimidine liquid crystals [Citation145].](/cms/asset/7eafe79e-17d4-4ffa-b5b8-2694ece4e322/tlct_a_2361294_f0009_oc.jpg)
Table 15. The chemical structures and mesophase transition temperatures (oC) of azobenzene pyrimidine compounds.
Table 16. The chemical structures and mesophase transition temperatures (oC) of pyrimidine-based photopolymerisable liquid crystals.
Table 17. The chemical structures and mesophase transition temperatures (oC) of pyrimidine-based polycatenary oligomers.
Organic semiconductors have experienced rapid development in terms of their opto-electronic properties in recent years. For instance, OLEDs are taking market share from LCDs due to a favourable combination of low power consumption without requiring backlight, high brightness, low switch-on and operating voltages, wide viewing angles, etc [Citation129,Citation151]. However, commercial OLEDs often use a vacuum-evaporated film-forming process and solution-processed OLEDs suitable for roll-to-roll printing and large-scale displays offer significant potential for future progress.
4. Pyrimidine-based liquid crystals for sensors
gives the schematic exhibition of pyrimidine-based liquid crystals coordinated with palladium or platinum as metallomesogens and non-conventional functional mesomorphic materials for application in sensors. Stimuli-responsive materials possess wide applications in sensors, 4D printing, soft robotics, artificial muscles, drug delivery, etc [Citation152–154]. Liquid crystals exhibiting unique ordered mesophases and optical anisotropy provide a highly promising class of responsive materials [Citation51,Citation155–157]. Phenyl pyrimidine-based liquid crystals particularly have attracted a lot of attention for use as chem/bio-sensors [Citation158–160]. Compounds containing N-heterocyclic rings, such as pyrimidine, are often used as sensing materials due to their electrophilic nature and tendency to form coordination complexes with metal ions and form hydrogen bonds [Citation161–164]. Tschierske’s group [Citation163] reported six series of pyrimidine-based calamitic/discotic liquid crystals coordinated with palladium and platinum as metallomesogens and non-conventional functional mesomorphic materials (see ). Pyrimidine-based sensors have been widely studied for selective and sensitive detection in solution as well as in the vapour phase for many applications, such as in water treatment and in other chemical, medical and biological fields, for example [Citation161].
Figure 10. (Colour online) The schematic exhibition of pyrimidine-based liquid crystals coordinated with palladium and platinum as metallomesogens and non-conventional functional mesomorphic materials for application in sensors.
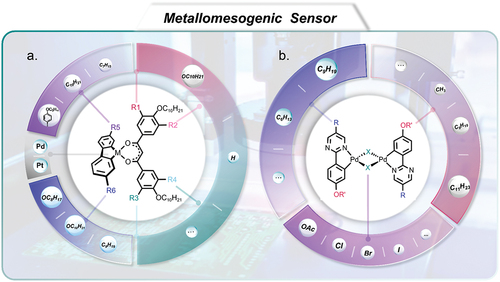
Table 18. The chemical structures and mesophase transition temperatures (oC) of pyrimidine-based organometallic calamitic/discotic liquid crystals.
Abbott’s group makes use of computational chemistry models to design a series of novel chemo-responsive LCs with improved chemical selectivity [Citation158–160]. In particular, the authors focused on selectivity against water, as water is one of the most common substances in chemical sensing. Interestingly, LCs containing pyrimidine cores in particular were found to exhibit a good tolerance to humidity and selectivity when responding to a target analyte [Citation158]. This behaviour could be attributable to the fact that binding of pyrimidine is stronger than that of a cyano-biphenyl mesogen, for example, but weaker than that of pyridine because modest binding forces can be achieved due to dynamic sensing by an anchoring and de-anchoring process. The result has been confirmed by computational chemistry and experiments [Citation158]. However, cyanobiphenyl LCs exhibit highly sensitive orientational response to target oxidising gases such as O3 and Cl2 [Citation160]. These examples suggest that the choice of mesogens coordinated with metal ions is particularly important for selective and sensitive detection, but they have not yet been deeply explored. Although many pyrimidine-based compounds have been studied for coordination with metal ions, such as palladium (see ) and alkali metal ions, for example, they were not used in sensing fields [Citation165,Citation166] or did not exhibit liquid crystalline mesophases [Citation161]. Most LC sensors are still based on a limited number commercial LCs, commonly used in opto-electronic displays, but which have not been optimised for use in sensors, for example. Therefore, it is intended that this review could inspire more researchers to design and synthesise new liquid crystals for application in highly sensitive and selective sensors.
Table 19. The chemical structures and mesophase transition temperatures (oC) of binuclear cyclopalladated phenylpyrimidine compounds.
5. Conclusion and perspective
In this review, we summarise the use of pyrimidine-based liquid crystals in ferroelectric display devices, organic semiconductors, and highly selective and sensitive detection, for example. A wide variety of electron-deficient pyrimidine-based LCs with different types of structures including calamitic, bent, discotic, taper-shaped, photopolymerizable monomers, as well as oligomers and polymers, for example, have been discussed for use as ferroelectric LCs, organic semiconductors and sensors. Although many generations of novel LCs have been developed for flat panel displays (LCDs) of ever-increasing size, complexity and image quality, the design of novel LCs for some fields of technological applications, such as LC sensors, flexible electronics, 3D/4D printing, etc., have received less attention. Many studies have used a small number of standard, commercial LCs, such as RM82, RM257, 5CB, etc., which have excellent properties in LCDs but not optimised properties for LC sensors or 3D/4D printing, for example. Therefore, we also point out the current challenge for the design of novel pyrimidine-based liquid crystalline structures and mesophases, and the study of the complex relationship between molecular structures, mesophases and application modes and mechanisms of operation. We hope that this review will promote the rapid invention and development of novel heterocyclic LCs in general and phenylpyrimidies in particular, for many high-tech applications requiring new advanced functional materials to realise their potential.
Abbreviation
Cr, Cr’, Cr1, Cr2: | = | crystalline phase |
G: | = | glass state |
N: | = | nematic phase |
Sm: | = | smectic phase |
SmA1: | = | a monolayer smectic A phase |
SmAd: | = | a partial bilayer smectic A phase |
Col: | = | columnar phase |
ColL: | = | lamellar columnar phase |
Colh, Colhex: | = | hexagonal columnar phase |
Colhd: | = | disordered hexagonal columnar phase |
NCol: | = | columnar nematic phase |
LamColrec = | = | lamellas-columnar phase with rectangular symmetry |
Ch: | = | cholesteric phase |
I: | = | isotropic liquid state |
IsoX: | = | isotropic X phase |
TGB: | = | a filament texture similar to the so-called fingerprint texture |
SmCanti: | = | antiferroelectric smectic C phase |
SmCalt: | = | alternating smectic C phase |
*: | = | chiral mesophase |
LC: | = | liquid crystal |
NLC: | = | non-liquid crystal |
Disclosure statement
No potential conflict of interest was reported by the author(s).
Additional information
Funding
References
- Geelhaar T, Griesar K, Reckmann B. 125 Years of liquid crystals—A scientific revolution in the home. Angew Chem Int Ed. 2013;52(34):8798–8809. doi: 10.1002/anie.201301457
- Schadt M. Nematic liquid crystals and twisted-nematic LCDs. Liq Cryst. 2015;42:646–652. doi: 10.1080/02678292.2015.1021597
- Reinitzer F. Beiträge zur Kenntniss des Cholesterins. Monatshefte für Chemie und verwandte Teile anderer Wissenschaften. Monatshefte für Chemie - Chemical Monthly. 1888;9(1):421–441. doi: 10.1007/BF01516710
- Reinitzer F. Contributions to the knowledge of cholesterol. Liq Cryst. 1989;5(1):7–18. doi: 10.1080/02678298908026349
- Yang DK, Wu ST. Fundamentals of liquid crystal devices. 2nd ed. West Sussex (UK): John Wiley & Sons; 2014.
- Hu G, Kitney SP, Bao W, et al. Novel heterocyclic liquid crystalline semiconductors with polymorphism. Phase Transit. 2020;93(12):1143–1156. doi: 10.1080/01411594.2020.1845677
- Hu G, Kitney SP, Harrison W, et al. Synthesis and mesomorphic behaviour of highly ordered liquid crystalline D-A-D-type carbazole-TPD-Carbazole. Phase Transit. 2020;93(8):759–772. doi: 10.1080/01411594.2020.1775830
- Vardar D, Ocak H, Akdaş Kılıç H, et al. Synthesis and characterization of new pyridine-based chiral calamitic liquid crystals. Liq Cryst. 2021;48(6):850–861. doi: 10.1080/02678292.2020.1825841
- Bremer M. Liquid crystals based on 2- fluoropyrimidine and -pyridine: synthesis, dielectric anisotropy and phase behavior*. Adv Mater. 1995;7(9):803–807. doi: 10.1002/adma.19950070910
- Upadhyay P, Rastogi MK, Kumar D. Polarizability study of nematic liquid crystal 4-cyano-4′-pentylbiphenyl (5CB) and its nitrogen derivatives. Chem Phys. 2015;456:41–46. doi: 10.1016/j.chemphys.2015.03.011
- Schilling C, Bauer A, Knöller JA, et al. Tailoring boron liquid crystals: mesomorphic properties of iminodiacetic acid boronates. J Mol Liq. 2022;367:120519. doi: 10.1016/j.molliq.2022.120519
- Chien C-W, Liu K-T, Lai CK. Heterocyclic columnar pyrimidines: synthesis, characterization and mesomorphic properties. Liq Cryst. 2004;31(7):1007–1017. doi: 10.1080/02678290410001713342
- Lin Y-C, Lai CK, Chang Y-C, et al. Formation of hexagonal columnar phases by heterocyclic pyrimidine derivatives. Liq Cryst. 2002;29(2):237–242. doi: 10.1080/02678290110097800
- Majumdar KC, Mondal S, Pal N, et al. Synthesis and mesomorphic behaviour of new mesogenic compounds possessing a cholesteryl ester moiety connected to a pyrimidine core. Tetrahedron Lett. 2009;50(17):1992–1995. doi: 10.1016/j.tetlet.2009.02.065
- Majumdar KC, Ghosh T. Synthesis and mesomorphic behavior of novel calamitic liquid crystalline dimesogens possessing a cholesteryl moiety connected to a pyrimidine core. Mol Cryst Liq Cryst. 2013;577(1):15–24. doi: 10.1080/15421406.2013.779180
- Nishiyama I, Tabe Y, Yamamoto J, et al. Pre-organization effects on chirality, polarity and biaxiality in liquid crystals: novel laterally connected mesogens showing anomalous properties. Mol Cryst Liq Cryst. 2011;549(1):174–183. doi: 10.1080/15421406.2011.581528
- Akdas-Kilig H, Godfroy M, Fillaut J-L, et al. Mesogenic, luminescence, and nonlinear optical properties of New Bipyrimidine-based multifunctional octupoles. J Phys Chem C. 2015;119(7):3697–3710. doi: 10.1021/jp511486y
- Barszcz B, Bogucki A, Świetlik R, et al. Conformational studies of bipyrimidine-based mesogens by combination of DFT calculations and temperature-dependent infrared studies. Liq Cryst. 2019;46(9):1403–1414. doi: 10.1080/02678292.2019.1575990
- Czerwiński M, Gaładyk K, Morawiak P, et al. Pyrimidine-based ferroelectric mixtures – the influence of oligophenyl based chiral doping system. J Mol Liq. 2020;303:112693. doi: 10.1016/j.molliq.2020.112693
- Lapanik V, Lugovski A, Timofeev S. A new generation of ferroelectric liquid crystals with homogeneous and shock-stable orientation. Liq Cryst. 2023;50(2):203–209. doi: 10.1080/02678292.2022.2110956
- Hird M. Ferroelectricity in liquid crystals—materials, properties and applications. Liq Cryst. 2011;38(11–12):1467–1493. doi: 10.1080/02678292.2011.625126
- Yuan H, Li M-Y, Chen C-N, et al. Substituent effects on the UV absorption energy of 2,5-disubstituted pyrimidines. J Phys Org Chem. 2018;31(10):e3860. doi: 10.1002/poc.3860
- Komatsu R, Sasabe H, Kido J. Recent progress of pyrimidine derivatives for high-performance organic light-emitting devices. J Photonics Energy. 2018;8(3):032108–032108. doi: 10.1117/1.JPE.8.032108
- Yoon J, Lee C, Park SH, et al. Pyrimidine-based bipolar host materials for high efficiency solution processed green thermally activated delayed fluorescence OLEDs. J Mater Chem C. 2020;8(6):2196–2204. doi: 10.1039/C9TC05727G
- Zhen Y, Zhang F, Liu H, et al. Impact of peripheral groups on pyrimidine acceptor-based HLCT materials for efficient deep blue OLED devices. J Mater Chem C. 2022;10(27):9953–9960. doi: 10.1039/D2TC01766K
- Li B, Li Z, Song X, et al. Pyrimidine-based thermally activated delayed fluorescent materials with unique asymmetry for highly-efficient organic light-emitting diodes. Dyes Pigm. 2022;203:110373. doi: 10.1016/j.dyepig.2022.110373
- Chen H-W, Lee J-H, Lin B-Y, et al. Liquid crystal display and organic light-emitting diode display: present status and future perspectives. Light: Sci Appl. 2018;7(3):17168–17168. doi: 10.1038/lsa.2017.168
- Huang Y, Hsiang E-L, Deng M-Y, et al. Mini-LED, micro-LED and OLED displays: present status and future perspectives. Light: Sci Appl. 2020;9(1):105. doi: 10.1038/s41377-020-0341-9
- Venkateswararao A, Wong K-T. Small molecules for vacuum-processed organic photovoltaics: past, Current status, and Prospect. Bull Chem Soc Jpn. 2021;94(3):812–838. doi: 10.1246/bcsj.20200330
- Kim J, Lee J, Chae S, et al. Conjugated polymers containing pyrimidine with electron withdrawing substituents for organic photovoltaics with high open-circuit voltage. Polymer. 2016;83:50–58. doi: 10.1016/j.polymer.2015.12.017
- El Aslaoui Z, Karzazi Y. Theoretical investigation of new pyrimidines π-conjugated based materials for photovoltaic applications. J Mater Environ Sci. 2017;8:1291–1300. https://www.jmaterenvironsci.com/Document/vol8/vol8_N4/137-JMES-El%20aslaoui.pdf
- Kojima T, J-I N, Tokito S, et al. New n-type field-effect transistors based on pyrimidine-containing compounds with (Trifluoromethyl)phenyl groups. Chem Lett. 2009;38(5):428–429. doi: 10.1246/cl.2009.428
- Shamoon Ahmad S, Abdullah MM. DFT modeling of 4,6-Di(2-furyl)pyrimidine derivatives as efficient charge transfer materials. Russ J Phys Chem. 2018;92(10):1996–2002. doi: 10.1134/S003602441810031X
- Karmegam V, Udamulle Gedara CM, Biewer MC, et al. Synthesis and opto-electronic properties of functionalized pyrimidine-based conjugated polymers. J Polym Sci Part A. 2018;56(22):2547–2553. doi: 10.1002/pola.29234
- Pandidurai J, Jayakumar J, Chen Y-K, et al. Constitutional isomers of carbazole–benzoyl-pyrimidine-based thermally activated delayed fluorescence emitters for efficient OLEDs. J Mater Chem C. 2021;9(44):15900–15909. doi: 10.1039/D1TC03998A
- Li Y, Yao J, Wang C, et al. Highly efficient deep-red/near-infrared D-A chromophores based on naphthothiadiazole for OLEDs applications. Dyes Pigm. 2020;173:107960. doi: 10.1016/j.dyepig.2019.107960
- Fu C, Sun W, Zhao Y, et al. Facile access to high-performance reverse intersystem crossing OLED materials through an unsymmetrical D-A-D’ molecular scaffold. Chem Eng J. 2022;450:137989. doi: 10.1016/j.cej.2022.137989
- Duan L, Qiao J, Sun Y, et al. Strategies to design bipolar small molecules for OLEDs: donor-acceptor structure and non-donor-acceptor structure. Adv Mater. 2011;23(9):1137–1144. doi: 10.1002/adma.201003816
- Trivedi HD, Joshi VB, Patel BY. Pyrazole bearing pyrimidine analogues as the privileged scaffolds in antimicrobial drug discovery: a Review. Anal Chem Lett. 2022;12(2):147–173. doi: 10.1080/22297928.2021.1910565
- Tiwari SV, Sarkate AP, Lokwani DK, et al. Explorations of novel pyridine-pyrimidine hybrid phosphonate derivatives as aurora kinase inhibitors. Bioorg Med Chem Lett. 2022;67:128747. doi: 10.1016/j.bmcl.2022.128747
- Oukoloff K, Lucero B, Francisco KR, et al. 1,2,4-Triazolo[1,5-a]pyrimidines in drug design. Eur J Med Chem. 2019;165:332–346. doi: 10.1016/j.ejmech.2019.01.027
- Zhan X, Liu Y, Yang K-L, et al. State-of-the-Art Development in Liquid Crystal Biochemical Sensors. Biosensors (Basel). 2022;12(8):577. doi: 10.3390/bios12080577
- Bisoyi HK, Li Q. Liquid crystals: versatile self-organized smart soft materials. Chem Rev. 2022;122(5):4887–4926. doi: 10.1021/acs.chemrev.1c00761
- Popov N, Honaker LW, Popova M, et al. Thermotropic Liquid Crystal-assisted chemical and biological sensors. Materials. 2018;11(1):20. doi: 10.3390/ma11010020
- Rahman ML, Hegde G, Yusoff MM, et al. New pyrimidine-based photo-switchable bent-core liquid crystals. New J Chem. 2013;37(8):2460–2467. doi: 10.1039/C3NJ00359K
- Huang Y, Bisoyi HK, Huang S, et al. Bioinspired synergistic photochromic luminescence and programmable liquid crystal actuators. Angew Chem Int Ed. 2021;60(20):11247–11251. doi: 10.1002/anie.202101881
- Luo M, Liu Y, Zhao J, et al. Magic tetraphenylethene Schiff base derivatives with AIE, liquid crystalline and photochromic properties. Dyes Pigm. 2022;202:110222. doi: 10.1016/j.dyepig.2022.110222
- Han MJ, Lee D-W, Lee EK, et al. Molecular orientation control of liquid crystal organic semiconductor for high-performance organic field-effect transistors. ACS Appl Mater Interfaces. 2021;13(9):11125–11133. doi: 10.1021/acsami.0c22393
- Suleymanova AF, Shafikov MZ, Chen X, et al. Construction and performance of OLED devices prepared from liquid-crystalline TADF materials. Phys Chem Chem Phys. 2022;24(36):22115–22121. doi: 10.1039/D2CP02684H
- Chen HY, Hu DQ, Yang QG, et al. All-small-molecule organic solar cells with an ordered liquid crystalline donor. Joule. 2019;3(12):3034–3047. doi: 10.1016/j.joule.2019.09.009
- Hu G, Zhang B, Kelly SM, et al. Photopolymerisable liquid crystals for additive manufacturing. Addit. Manuf. 2022;55:102861. doi: 10.1016/j.addma.2022.102861
- Liao W, Yang Z. 3D printing programmable liquid crystal elastomer soft pneumatic actuators. Mater Horiz. 2023;10(2):576–584. doi: 10.1039/D2MH01001A
- Del Pozo M, Jahp S, Aphj S, et al. 4D Printing of liquid crystals: what’s right for me? Advanced Materials. 2022;34(3):2104390. doi: 10.1002/adma.202104390
- Ula SW, Traugutt NA, Volpe RH, et al. Liquid crystal elastomers: an introduction and review of emerging technologies. Liq Cryst Rev. 2018;6(1):78–107. doi: 10.1080/21680396.2018.1530155
- Ryu M, Cang Y, Wang Z, et al. Temperature-dependent thermoelastic anisotropy of the phenyl pyrimidine liquid crystal. J Phys Chem C. 2019;123(28):17148–17154. doi: 10.1021/acs.jpcc.9b04270
- Cang Y, Liu J, Ryu M, et al. On the origin of elasticity and heat conduction anisotropy of liquid crystal elastomers at gigahertz frequencies. Nat Commun. 2022;13(1):5248. doi: 10.1038/s41467-022-32865-1
- Petrov VF. Pyrimidine as a structural fragment in calamitic liquid crystals. Mol Cryst Liq Cryst. 2006;457(1):121–149. doi: 10.1080/15421400600598545
- Achelle S, Plé N. Pyrimidine ring as building block for the synthesis of functionalized II-conjugated materials. Curr Org Synth. 2012;9(2):163–187. doi: 10.2174/157017912799829067
- Ghosh T, Lehmann M. Recent advances in heterocycle-based metal-free calamitics. J Mater Chem C. 2017;5(47):12308–12337. doi: 10.1039/C7TC03502K
- Swaminathan V, Panov VP, Panov A, et al. Design and electro-optic investigations of de vries chiral smectic liquid crystals for exhibiting broad temperature ranges of SmA* and SmC* phases and fast electro-optic switching. J Mater Chem C. 2020;8(14):4859–4868. doi: 10.1039/C9TC04405A
- Gupta SK, Budaszewski D, Singh DP. Ferroelectric liquid crystals: futuristic mesogens for photonic applications. Eur Phys J Spec Top. 2022;231(4):673–694. doi: 10.1140/epjs/s11734-021-00390-9
- Zhao Y, Pan S, Li Y, et al. High contrast ratio and fast response ferroelectric liquid crystal displays based on alignment optimization. Opt Mater. 2023;143:114257. doi: 10.1016/j.optmat.2023.114257
- Meyer RB, Liebert L, Strzelecki L, et al. FERROELECTRIC LIQUID-CRYSTALS. J Phys Lett. 1975;36(3):69–71. doi: 10.1051/iphyslet:0197500360306900
- Clark NA, Lagerwall ST. SUBMICROSECOND BISTABLE ELECTRO-OPTIC SWITCHING in LIQUID-CRYSTALS. Appl Phys Lett. 1980;36(11):899–901. doi: 10.1063/1.91359
- Thompson M, Carkner C, Bailey A, et al. Tuning the mesogenic properties of 5-alkoxy-2-(4-alkoxyphenyl)pyrimidine liquid crystals: the effect of a phenoxy end-group in two sterically equivalent series. Liq Cryst. 2014;41(9):1246–1260. doi: 10.1080/02678292.2014.913721
- Patari S, Chakraborty S, Nath A. The optical anisotropy and orientational order parameter of two mesogens having slightly different flexible side chain – a comparative study. Liq Cryst. 2016;43(8):1017–1027. doi: 10.1080/02678292.2016.1155767
- Tykarska M, Dąbrowski R, Czerwiński M, et al. The influence of the chiral terphenylate on the tilt angle in pyrimidine ferroelectric mixtures. Phase Transit. 2012;85(4):364–370. doi: 10.1080/01411594.2011.646274
- Pramanik A, Das MK, Das B, et al. Preparation and study of the electro-optical properties of binary mixtures of orthoconic anti-ferroelectric Esters and achiral phenyl pyrimidine liquid crystal. Soft Mater. 2015;13(4):201–209. doi: 10.1080/1539445X.2015.1063510
- Roberts JC, Kapernaum N, Giesselmann F, et al. Design of liquid crystals with “de vries-like” properties: organosiloxane Mesogen with a 5-Phenylpyrimidine Core. J Am Chem Soc. 2008;130(42):13842–13843. doi: 10.1021/ja805672q
- Radcliffe MD, Brostrom ML, Epstein KA, et al. Smectic a and C materials with novel director tilt and layer thickness behaviour. Liq Cryst. 1999;26(6):789–794. doi: 10.1080/026782999204471
- Rieker TP, Janulis EP. Enhanced thermal response of the SAd1 layer thickness in highly fluorinated thermotropic liquid crystals. Liq Cryst. 1994;17(5):681–687. doi: 10.1080/02678299408037339
- Romero-Hasler PN, Kurihara LK, Mair LO, et al. Nanocomposites of ferroelectric liquid crystals and FeCo nanoparticles: towards a magnetic response via the application of a small electric field. Liq Cryst. 2020;47(2):169–178. doi: 10.1080/02678292.2019.1633429
- Khan S, Chauhan S, Chandran A, et al. Enhancement of dielectric and electro-optical parameters of a newly prepared ferroelectric liquid crystal mixture by dispersing nano-sized copper oxide. Liq Cryst. 2020;47(2):263–272. doi: 10.1080/02678292.2019.1643506
- Debnath A, Mandal PK, Sarma A, et al. Effect of non-mesogenic chiral terphenylate on the formulation of room temperature ferroelectric liquid crystal mixtures suitable for display applications. J Mol Liq. 2019;292:111317. doi: 10.1016/j.molliq.2019.111317
- Chakraborty A, Chakraborty S, Kumar Das M. Critical behavior at the isotropic to nematic, nematic to smectic-A and smectic-A to smectic-C phase transitions in a pyrimidine liquid crystal compound. Physica B. 2015;479:90–95. doi: 10.1016/j.physb.2015.09.039
- Pozhidaev E, Torgova S, Barbashov V, et al. Development of ferroelectric liquid crystals with low birefringence. Liq Cryst. 2019;46(6):941–951. doi: 10.1080/02678292.2018.1542749
- Wand MD, Thurmes WN, Vohra RT, et al. New chiral dopants based on the 2-Fluoro-2-methylalkoxy tail for use in ferroelectric liquid crystal mixtures. Mol Cryst Liq Cryst. 1995;263(1):217–222. doi: 10.1080/10587259508033586
- Gaładyk K, Piecek W, Czerwiński M, et al. An effect of chiral dopants on mesomorphic and electro-optical properties of ferroelectric smectic mixture. Liq Cryst. 2019;46(15):2134–2148. doi: 10.1080/02678292.2019.1613689
- Sreenilayam SP, Rodriguez-Lojo D, Panov VP, et al. Design and investigation of de vries liquid crystals based on 5-phenyl-pyrimidine and (RR)-2,3-epoxyhexoxy backbone. Phys Rev E. 2017;96(4):042701. doi: 10.1103/PhysRevE.96.042701
- Swaminathan V, Panov VP, Kocot A, et al. Molecular orientational distribution function of a chiral de vries smectic liquid crystal from birefringence measurements. J Chem Phys. 2019;150(8):084901. doi: 10.1063/1.5080222
- Sreenilayam SP, Rodriguez-Lojo D, Agra-Kooijman DM, et al. de Vries liquid crystals based on a chiral 5-phenylpyrimidine benzoate core with a tri- and tetra-carbosilane backbone. Phys Rev Mater. 2018;2(2):025603. doi: 10.1103/PhysRevMaterials.2.025603
- Schubert CPJ, Müller C, Bogner A, et al. Design of liquid crystals with ‘de vries-like’ properties: structural variants of carbosilane-terminated 5-phenylpyrimidine mesogens. Soft Matter. 2017;13(18):3307–3313. doi: 10.1039/C7SM00355B
- Furue H, Furutani M, Ito A, et al. Alignment structure of dimeric liquid crystal molecules having bent molecular structure. Ferroelectrics. 2010;394(1):22–31. doi: 10.1080/00150191003677569
- Kashima S, Takanishi Y, Yamamoto J, et al. Flexible taper-shaped liquid crystal trimer exhibiting a modulated smectic phase. Liq Cryst. 2014;41(12):1752–1761. doi: 10.1080/02678292.2014.950353
- Roberts JC, Kapernaum N, Song Q, et al. Design of liquid crystals with “de vries-like” properties: frustration between SmA- and SmC-promoting elements. J Am Chem Soc. 2010;132(1):364–370. doi: 10.1021/ja9087727
- Song Q, Nonnenmacher D, Giesselmann F, et al. Tuning the frustration between SmA- and SmC-promoting elements in liquid crystals with ‘de vries-like’ properties. Chem Commun. 2011;47(16):4781–4783. doi: 10.1039/C1CC10344J
- Rupar I, Mulligan KM, Roberts JC, et al. Elucidating the smectic A-promoting effect of halogen end-groups in calamitic liquid crystals. J Mater Chem C. 2013;1(23):3729–3735. doi: 10.1039/C3TC30534A
- Roberts JC, Kapernaum N, Giesselmann F, et al. Fast switching organosiloxane ferroelectric liquid crystals. J Mater Chem. 2008;18(43):5301–5306. doi: 10.1039/B810209K
- Schubert CPJ, Bogner A, Porada JH, et al. Design of liquid crystals with ‘de vries-like’ properties: carbosilane-terminated 5-phenylpyrimidine mesogens suitable for chevron-free FLC formulations. J Mater Chem C. 2014;2(23):4581–4589. doi: 10.1039/C4TC00393D
- Schubert CPJ, Müller C, Giesselmann F, et al. Chiral 5-phenylpyrimidine liquid crystals with ‘de vries-like’ properties: dependence of electroclinic effect and ferroelectric properties on carbosilane nanosegregation. J Mater Chem C. 2016;4(36):8483–8489. doi: 10.1039/C6TC03120J
- Liu H, Nohira H. Influence of fluorination extent on liquid crystalline properties of semi-perfluorinated phenylpyrimidine ferroelectric liquid crystals. Liq Cryst. 1998;24(5):719–726. doi: 10.1080/026782998206830
- Rieker TP, Janulis EP. Dimerlike smectic- a and - C phases in highly fluorinated thermotropic liquid crystals. Phys Rev E. 1995;52(3):2688–2691. doi: 10.1103/PhysRevE.52.2688
- Poll K, Sims MT. Sub-layer rationale of anomalous layer-shrinkage from atomistic simulations of a fluorinated mesogen. Mater Adv. 2022;3(2):1212–1223. doi: 10.1039/D1MA00714A
- Abhilash TK, Varghese H, Czerwiński M, et al. Probing the effect of chiral dopant fluorination on dielectric and electro-optical properties of the ferroelectric liquid crystalline mixture. J Mol Liq. 2021;341:117392. doi: 10.1016/j.molliq.2021.117392
- Song Q, Nonnenmacher D, Giesselmann F, et al. Tuning ‘de vries-like’ properties in siloxane- and carbosilane-terminated smectic liquid crystals. J Mater Chem C. 2013;1(2):343–350. doi: 10.1039/C2TC00338D
- Ahmed Z, Müller C, Johnston JJ, et al. Design of liquid crystals with ‘de vries-like’ properties: the effect of an ethynyl spacer in the core structure. Liq Cryst. 2019;46(6):896–904. doi: 10.1080/02678292.2018.1536810
- Mulligan KM, Bogner A, Song Q, et al. Design of liquid crystals with ‘de vries-like’ properties: the effect of carbosilane nanosegregation in 5-phenyl-1,3,4-thiadiazole mesogens. J Mater Chem C. 2014;2(39):8270–8276. doi: 10.1039/C4TC01364F
- Müller C, Schubert CPJ, Lemieux RP, et al. The influence of carbosilane nanosegregation on the Dynamics in ‘de vries-type’ liquid crystals. Chemphyschem. 2018;19(20):2703–2708. doi: 10.1002/cphc.201800537
- Schubert CPJ, Müller C, Wand MD, et al. Electroclinic effect in a chiral carbosilane-terminated 5-phenylpyrimidine liquid crystal with ‘de vries-like’ properties. Chem Commun. 2015;51(63):12601–12604. doi: 10.1039/C5CC05212B
- Lin P-T, Wu S-T, Chang C-Y, et al. UV stability of high birefirngence liquid crystals. Mol Cryst Liq Cryst. 2004;411(1):243–253. doi: 10.1080/15421400490435233
- Yadav SP, Yadav K, Lahiri J, et al. Ferroelectric liquid crystal nanocomposites: recent development and future perspective. Liq Cryst Rev. 2018;6(2):143–169. doi: 10.1080/21680396.2019.1589400
- Poryvai A, Šmahel M, Švecová M, et al. Chiral, magnetic, and photosensitive liquid crystalline nanocomposites based on multifunctional nanoparticles and achiral liquid crystals. ACS Nano. 2022;16(8):11833–11841. doi: 10.1021/acsnano.1c10594
- Bukowczan A, Hebda E, Pielichowski K. The influence of nanoparticles on phase formation and stability of liquid crystals and liquid crystalline polymers. J Mol Liq. 2021;321:114849. doi: 10.1016/j.molliq.2020.114849
- Kumar A, Priyam, Meena H, et al. Recent advances on semiconducting nanomaterials–ferroelectric liquid crystals nanocomposites. J Phys Condens Mat. 2022;34(1):013004. doi: 10.1088/1361-648X/ac2ace
- McDonald R, Lacey D, Watson P, et al. Synthesis and evaluation of some novel chiral heterocyclic liquid crystalline materials exhibiting ferro‐ and antiferro‐electric phases. Liq Cryst. 2005;32(3):319–330. doi: 10.1080/02678290500033711
- Foo KL, Ha ST, Yeap GY. Synthesis and phase transition behavior of calamitic liquid crystals containing heterocyclic core and lateral ethoxy substituent. Phase Transit. 2022;95(2):178–192. doi: 10.1080/01411594.2021.2023745
- Jian J, Hong F, Xia Z, et al. New fluorescent N-heterocyclic liquid crystals with high birefringence. J Mol Liq. 2016;224:909–913. doi: 10.1016/j.molliq.2016.10.071
- Hu K, Weng Q, Chen R, et al. Benzoxazole-terminated liquid crystals with high birefringence and large dielectric anisotropy. Liq Cryst. 2020;47(9):1274–1280. doi: 10.1080/02678292.2019.1710777
- Sharma S, Lacey D, Wilson P. The SYNTHESIS and THERMAL PROPERTIES of NOVEL HETEROCYCLIC LIQUID CRYSTALLINE MATERIALS. Mol Cryst Liq Cryst. 2003;401(1):111–121. doi: 10.1080/744815207
- Sharma S, Lacey D, Wilson P. Synthesis and characterization of a range of heterocyclic liquid crystalline materials incorporating the novel thiophene-pyrimidine moiety. Liq Cryst. 2003;30(4):451–461. doi: 10.1080/0267829031000091138
- Wilson P, Lacey D, Sharma S, et al. The synthesis and characterisation of novel thienyl-pyrimidine liquid crystalline materials. Mol Cryst Liq Cryst. 2001;368(1):279–292. doi: 10.1080/10587250108029957
- Hori K, Matsunaga Y, Yoshizawa A, et al. Diversity in the packing modes of mesogenic diphenylpyrimidines with two chiral centres in their crystal structures: the role of interactions between the pyrimidine rings. Liq Cryst. 2004;31(6):759–766. doi: 10.1080/02678290410001697576
- Mukherjee A, Bhattacharyya SS, Chaudhuri BK, et al. Dielectric spectroscopy of a dichiral mesogen with a unique phase sequence. Mol Cryst Liq Cryst. 2010;518(1):60–69. doi: 10.1080/15421400903568039
- Maeda Y, Yokoyama H, Yoshizawa A, et al. Phase behaviour under pressure of a dichiral liquid crystal with an optically isotropic cubic phase: 2-{4-[(R)-2-fluorohexyloxy]phenyl}-5-{4-[(S)-2-fluoro-2-methyldecanoyloxy]phenyl}pyrimidine. Liq Cryst. 2007;34(1):9–18. doi: 10.1080/02678290600905412
- Wong K-T, Hung TS, Lin Y, et al. Suzuki coupling approach for the synthesis of Phenylene−Pyrimidine alternating oligomers for blue light-emitting material. Org Lett. 2002;4(4):513–516. doi: 10.1021/ol017066z
- Wong K-T, Lu Y-R, Liao Y-L. Synthesis and properties of pyrimidine-containing linear molecules. Tetrahedron Lett. 2001;42(36):6341–6344. doi: 10.1016/S0040-4039(01)01275-8
- Wong K-T, Fang F-C, Cheng Y-M, et al. A new series of Pyrimidine-containing linear molecules: their elegant crystal structures and intriguing photophysical properties. J Org Chem. 2004;69(23):8038–8044. doi: 10.1021/jo048914h
- Termine R, Golemme A. Charge mobility in discotic liquid crystals. Int J Mol Sci. 2021;22(2):877. doi: 10.3390/ims22020877
- Wöhrle T, Wurzbach I, Kirres J, et al. Discotic liquid crystals. Chem Rev. 2016;116(3):1139–1241. doi: 10.1021/acs.chemrev.5b00190
- Sergeyev S, Pisula W, Geerts YH. Discotic liquid crystals: a new generation of organic semiconductors. Chem Soc Rev. 2007;36(12):1902–1929. doi: 10.1039/B417320C
- Wu H, Iino H, J-I H. Bilayered Crystalline Organic Semiconductors for Solution-processed OFETs: Asymmetrically-substituted Smectic Liquid Crystal of Benzo[1,2-b: 4,5-b′]dithiophene Derivatives. Derivatives Chem Lett. 2018;47(4):510–513. doi: 10.1246/cl.180066
- Nikaido K, Inoue S, Kumai R, et al. Mixing-induced orientational ordering in liquid-crystalline organic semiconductors. Adv Mater Interfaces. 2022;9(35):2201789. doi: 10.1002/admi.202201789
- Aldred MP, Carrasco‐Orozco M, Contoret AEA, et al. Organic electroluminescence using polymer networks from smectic liquid crystals. Liq Cryst. 2006;33(4):459–467. doi: 10.1080/02678290500487073
- Hu G, Kelly SM, Kitney SP, et al. Novel nematic and glassy liquid crystalline oligomers as electroluminescent organic semiconductors. Liq Cryst. 2021;48(5):626–640. doi: 10.1080/02678292.2020.1800847
- Hu G, Kitney SP, Billa MR, et al. A novel nematic tri-carbazole as a hole-transport material for solution-processed OLEDs. Liq Cryst. 2021;48(1):63–74. doi: 10.1080/02678292.2020.1765262
- Hu G, Billa MR, Kitney SP, et al. Symmetrical carbazole–fluorene–carbazole nematic liquid crystals as electroluminescent organic semiconductors. Liq Cryst. 2018;45(7):965–979. doi: 10.1080/02678292.2017.1404156
- Hu G, Kitney SP, Kelly SM, et al. Novel liquid crystalline organic semiconducting oligomers incorporating N-heterocyclic carbazole moieties for fluorescent OLEDs. Liq Cryst. 2017;44(11):1632–1645. doi: 10.1080/02678292.2017.1306633
- Han MJ, Wei D, Kim YH, et al. Highly oriented liquid crystal semiconductor for organic field-effect transistors. ACS Cent Sci. 2018;4(11):1495–1502. doi: 10.1021/acscentsci.8b00465
- Bushby RJ, O’Neill M, Kelly SM. Liquid crystalline semiconductors: materials, properties and applications. Dordrecht (Netherlands): Springer; 2013.
- O’Neill M, Kelly SM. Ordered materials for organic electronics and photonics. Adv Mater. 2011;23(5):566–584. doi: 10.1002/adma.201002884
- O’Neill M, Kelly SM. Liquid crystals for charge transport, luminescence, and photonics. Adv Mater. 2003;15(14):1135–1146. doi: 10.1002/adma.200300009
- Tong F, Chen S, Chen Z, et al. Mesogen-co-polymerized transparent polyimide as a liquid-crystal alignment layer with enhanced anchoring energy. RSC Adv. 2018;8(20):11119–11126. doi: 10.1039/C8RA00479J
- Wang A, Kawabata K, Goto H. Synthesis of isothianaphthene (ITN) and 3,4-Ethylenedioxy-thiophene (EDOT)-based low-bandgap liquid crystalline conjugated polymers. Materials. 2013;6(6):2218–2228. doi: 10.3390/ma6062218
- Ito R, Goto H. Synthesis of aromatic liquid crystal π-conjugated copolymers bearing three-ring pyrimidine-based mesogens, and optical texture observations. Mol Cryst Liq Cryst. 2021;723(1):33–44. doi: 10.1080/15421406.2021.1897943
- Kawabata K, Goto H. Liquid crystalline π-conjugated copolymers bearing a pyrimidine type mesogenic group. Materials. 2009;2(1):22–37. doi: 10.3390/ma2010022
- Ohkawa S, Ohta R, Kawabata K, et al. Polymerization in liquid crystal medium: preparation of polythiophene derivatives bearing a bulky pyrimidine substituent. Polymers. 2010;2(4):393–406. doi: 10.3390/polym2040393
- Otaki M, Kumai R, Sagayama H, et al. Synthesis of polyazobenzenes exhibiting photoisomerization and liquid crystallinity. Polymers. 2019;11(2):348. doi: 10.3390/polym11020348
- Wang A, Kawabata K, Kawashima H, et al. Synthesis of a pyrimidine-based new chiral inducer for construction of cholesteric liquid crystal electrolyte solution and its electrochemical polymerization, and stimulated emission like interference. Polymer. 2013;54(15):3821–3827. doi: 10.1016/j.polymer.2013.04.053
- Yin K, Hsiang E-L, Zou J, et al. Advanced liquid crystal devices for augmented reality and virtual reality displays: principles and applications. Light: Sci Appl. 2022;11(1):161. doi: 10.1038/s41377-022-00851-3
- Liedtke A, O’Neill M, Wertmöller A, et al. White-light OLEDs using liquid crystal polymer networks. Chem Mater. 2008;20(11):3579–3586. doi: 10.1021/cm702925f
- McCulloch I, Coelle M, Genevicius K, et al. Electrical properties of reactive liquid crystal semiconductors. Jpn J Appl Phys. 2008;47(1S):488. doi: 10.1143/JJAP.47.488
- Aldred MP, Eastwood AJ, Kelly SM, et al. Light-emitting fluorene photoreactive liquid crystals for organic electroluminescence. Chem Mater. 2004;16(24):4928–4936. doi: 10.1021/cm0351893
- Contoret AEA, Farrar SR, Jackson PO, et al. Polarized electroluminescence from an anisotropic nematic network on a non-contact photoalignment layer. Adv Mater. 2000;12(13):971–974. doi: 10.1002/1521-4095(200006)12:13<971:AID-ADMA971>3.0.CO;2-J
- Rastogi P, Njuguna J, Kandasubramanian B. Exploration of elastomeric and polymeric liquid crystals with photothermal actuation: a review. Eur Polym J. 2019;121:109287. doi: 10.1016/j.eurpolymj.2019.109287
- Ye Y, He R, Oh E, et al. Syntheses and properties of new smectic reactive mesogens and their application in guest-host polarizer. Macromol Res. 2020;28(10):910–918. doi: 10.1007/s13233-020-8121-1
- Aldred MP, Vlachos P, Dong D, et al. Heterocyclic reactive mesogens: synthesis, characterisation and mesomorphic behaviour. Liq Cryst. 2005;32(8):951–965. doi: 10.1080/02678290500248400
- Vlachos P, Kelly SM, Mansoor B, et al. Electron-transporting and photopolymerisable liquid crystals. Chem Commun. 2002;8(8):874–875. doi: 10.1039/B200526C
- Hu G, Kitney SP, Kelly SM, et al. Polymer network hole transport layers based on photochemically cross-linkable N′N′-diallyl amide tri-N-substituted triazatruxene monomers. RSC Adv. 2018;8(16):8580–8585. doi: 10.1039/C8RA00830B
- Kelly SM, Fünfschilling J, Villiger A. 2-(4-alkylphenyl)-5-(alkenyloxy)pyrimidines: synthesis, liquid crystal transition temperatures and some physical properties. Liq Cryst. 1994;16(5):813–829. doi: 10.1080/02678299408027852
- Sultana NH, Kelly SM, Mansoor B, et al. Polycatenar oligophenylene liquid crystals. Liq Cryst. 2007;34(11):1307–1316. doi: 10.1080/02678290701682357
- Kelly SM. Flat panel displays: advanced organic materials. Cambridge (UK): The Royal Society of Chemistry; 2000.
- Patdiya J, Kandasubramanian B. Progress in 4D printing of stimuli responsive materials. Polym-Plast Tech Mat. 2021;60(17):1845–1883. doi: 10.1080/25740881.2021.1934016
- Hu L, Zhang Q, Li X, et al. Stimuli-responsive polymers for sensing and actuation. Mater Horiz. 2019;6(9):1774–1793. doi: 10.1039/C9MH00490D
- Xia X, Spadaccini CM, Greer JR. Responsive materials architected in space and time. Nat Rev Mater. 2022;7(9):683–701. doi: 10.1038/s41578-022-00450-z
- Guan Z, Wang L, Bae J. Advances in 4D printing of liquid crystalline elastomers: materials, techniques, and applications. Mater Horiz. 2022;9(7):1825–1849. doi: 10.1039/D2MH00232A
- Oladepo SA. Development and application of liquid crystals as stimuli-responsive sensors. Molecules. 2022;27(4):1453. doi: 10.3390/molecules27041453
- Chen Y, Lu P, Li Z, et al. Dual stimuli-responsive high-efficiency circularly polarized luminescence from light-emitting chiral nematic liquid crystals. ACS Appl Mater Interfaces. 2020;12(50):56604–56614. doi: 10.1021/acsami.0c17241
- Yu H, Szilvási T, Rai P, et al. Computational chemistry-guided design of selective chemoresponsive liquid crystals using pyridine and pyrimidine functional groups. Adv Funct Mater. 2018;28(13):1703581. doi: 10.1002/adfm.201703581
- Nayani K, Yang Y, Yu H, et al. Areas of opportunity related to design of chemical and biological sensors based on liquid crystals. Liq Cryst Today. 2020;29(2):24–35. doi: 10.1080/1358314X.2020.1819624
- Bao N, Gold JI, Szilvási T, et al. Designing chemically selective liquid crystalline materials that respond to oxidizing gases. J Mater Chem C. 2021;9(20):6507–6517. doi: 10.1039/D1TC00544H
- Jindal G, Kaur N. Biologically significant pyrimidine appended optical sensors: An inclusive anthology of literature from 2005 to 2020. Coord Chem Rev. 2021;435:213798. doi: 10.1016/j.ccr.2021.213798
- Datta S, Bhattacharya S. Differential response of cholesterol based pyrimidine systems with oxyethylene type spacers to gelation and mesogen formation in the presence of alkali metal ions. Soft Matter. 2015;11(10):1945–1953. doi: 10.1039/C4SM02792B
- Hegmann T, Kain J, Diele S, et al. Molecular design at the calamitic/discotic cross-over point. Mononuclear ortho-metallated mesogens based on the combination of rod-like phenylpyrimidines and -pyridines with bent or half-disc-shaped diketonates. J Mater Chem. 2003;13(5):991–1003. doi: 10.1039/B210250A
- Guang W, Han J, Wan W, et al. Synthesis and liquid crystal properties of dinuclear cyclopalladated 5-alkyl-2-(4′-alkoxyphenyl)pyrimidine and 3-(4′-alkoxyphenyl)-6-alkoxypyridazine complexes. Liq Cryst. 2003;30(11):1259–1264. doi: 10.1080/0267829031000154372
- Ghedini M, Pucci D, De Munno G, et al. Transition metals complexed to ordered mesophases. Part 7. Synthesis, characterization and mesomorphic properties of binuclear cyclopalladated phenylpyrimidine species. Crystal structure of bis{(5-(1-hexyl)-2-[(4’-methoxy)phenyl-2’-ato]pyrimidine-N’,C2’)-.Mu.-acetato}dipalladium(II). Chem Mater. 1991;3:65–72. doi: 10.1021/cm00013a018
- Ghedini M, Pucci D, Bartolino R, et al. X-ray investigations on the cyclopalladated mesogen bis-{5-(1-nonyl)-2{[4′1-nonyloxy)phenyl-2′-ato]}pyrimidine-N′,C2′]-μ-iodo}dipalladium(II). Liq Cryst. 1993;13(2):255–263. doi: 10.1080/02678299308026299