ABSTRACT
Addressing the current challenge of high melting points and long π-conjugated skeletons in luminescent nematic liquid crystals (LCs), this study explores the incorporation of push-pull dyes with biphenyl and tolane skeletons to extend fluorescence emission wavelengths into the visible light region. We synthesised push-pull-type biphenyl and tolane derivatives incorporating alkylamino groups as donors and cyano groups as acceptors. The thermal behaviour was investigated using optical polarisation microscopy, differential scanning calorimetry and X-ray diffraction. Notably, some molecules exhibited a nematic phase. The photophysical properties of the solution and solid states were also investigated. Furthermore, we formulated a room-temperature luminescent LC by blending several LCs. Importantly, the fluorescence in the nematic phase exhibited a red-shift compared to that observed in the solution and solid states. This study demonstrates the efficacy of the push-pull structure in the design of luminescent LCs. The findings of this study hold considerable implications for the development of advanced optoelectronic devices, particularly those requiring luminescent materials with tailored properties.
1. Introduction
Luminescent liquid crystals (LCs) using organic π-electron molecules as mesogens have undergone extensive exploration in recent years, holding promise for various optoelectronic applications [Citation1–27]. One valuable future application involves the creation of solid-state light-emitting materials by controlling the orientation of dyes using an LC field followed by crystallisation or by developing light-emitting switch materials leveraging the electric field response of nematic LCs [Citation28,Citation29]. Nematic LCs are particularly fascinating because of their response to external stimuli [Citation30,Citation31] and chiral higher-order structures, such as cholesteric [Citation32–36] and blue phases [Citation37,Citation38]. However, a considerable challenge lies in the high melting point [Citation39] and high viscosity of most luminescent nematic LCs [Citation40–43] attributed to their utilisation of a long π-electron skeleton in the mesogens, limiting stimulus responsiveness. Addressing these challenges necessitates the utilisation of short π-electron skeletons such as biphenyls and tolanes. However, employing such π-electron skeletons alone restricts fluorescence emission in the UV region. Therefore, incorporating push-pull dye, featuring a donor and an acceptor introduced at the opposite ends of the long-axis direction of the π-electron skeleton, proves effective. This push-pull structure is widely used in dye design [Citation44–48] as this leads to an increase in the absorption wavelength owing to a narrower band gap, coupled with a fluorescence wavelength shift to a longer wavelength owing to intramolecular charge transfer in the excited state [Citation49].
In this study, we synthesised biphenyl and tolane derivatives with mono- or di-alkylamino groups as donors and cyano groups as acceptors on sides opposite to each other and investigated their phase transition behaviours and photophysical properties. While the alkyl and alkoxy groups in the tail of LC molecules act as weak donors, incorporating strong donors such as alkylamino groups proves effective in obtaining visible light emission with biphenyl and tolane skeletons. In 1981, 4-alkylamino-4’-cyanobiphenyls were reported to exhibit nematic liquid crystallinity [Citation50]. However, detailed structural analysis of the liquid crystalline phases, the effect of introducing substituents at lateral positions, extension to tolanes and luminescence properties remain unexplored. This study aims to clarify these unexplored interests and develop room-temperature nematic LCs derived from mixtures of several LC molecules. In addition, this study includes measurement of the photophysical properties of room temperature nematic LCs.
2. Experimental section
2.1. Measurements
1H–NMR and 13C–NMR spectra were recorded on BRUKER Ascend 500 (125 MHz) spectrometers for CDCl3 solutions using tetramethylsilane (TMS) as an internal standard. High-resolution (HR) EI mass spectra were recorded on a double-focusing mass spectrometer JEOL JMS-70. FT–IR spectra were recorded on a JASCO FT-IR 469 plus spectrometer. The phase transition behaviour was investigated by polarising optical microscopy (POM) (Leica DM2500P microscopy equipped with a Mettler FP90 hot stage), differential scanning calorimetry (DSC) (Perkin-Elmer DSC 8500) at a scanning rate of 10°C min−1 and wide-angle-X-ray diffraction (WAXD) using a Cu Kα radiation (Bruker D8 DISCOVER equipped with a Vantec-500 detector). UV–Vis spectra were recorded on a JASCO V-670 UV–Vis spectrophotometer, and fluorescence spectra were recorded on a JASCO FP-6500 spectrofluorometer. Absolute quantum yields were measured by a Hamamatsu Photonics Quantaurus QY apparatus. All photophysical measurements were performed using dilute solutions with optical densities (ODs) around 0.1 at the maximum absorption wavelength in 1 cm path length quartz cells at room temperature (298 K). In addition, all sample solutions were deaerated by bubbling with argon gas for 15 min prior to the quantum yield.
2.2. Materials
All solvents and chemicals were commercially available and used without further purification unless otherwise noted. The purchased materials are shown in SI (see Supporting Information).
2.3. Synthesis
2.3.1. Synthesis of 4-bromo-N-octylaniline (2) [Citation51]
4-Bromoaniline (1) (1.72 g, 10.0 mmol), sodium hydrogen carbonate (1.01 g, 12.0 mmol) and sodium dodecyl sulphate (0.04 g, 0.1 mmol) were dissolved in water (40.0 ml). The solution was stirred at 100°C for 5 min, then 1-bromooctane (2.1 ml. 10 mmol) was added to the solution and the resulting mixture was stirred at 100°C for more than 21 h. After cooling to room temperature, the obtained mixture was extracted with ethyl acetate, and the organic layer was washed with water three times, dried over MgSO4, filtrated and evaporated under reduced pressure. The residue was purified by column chromatography on silica gel, eluting with hexane/dichloromethane (v/v = 8/1) to give crude 2 (0.95 g, 3.3 mmol, 33%) as yellow liquid. 1H-NMR (500 MHz, CDCl3) δ 7.23 (d, J = 8.2 Hz, 2 H), 6.47 (d, J = 8.5 Hz, 2 H), 3.62 (s, 1 H), 3.06 (t, J = 7.0 Hz, 2 H), 1.60 (quin, J = 7.0 Hz, 2 H), 1.45–1.17 (m, 10 H), 0.88 (t, J = 6.6 Hz, 3 H) ppm (Figure S65).
2.3.2. Synthesis of N-octyl-4-(4,4,5,5-tetramethyl-1,3,2-dioxaborolan-2-yl)aniline (3) [Citation52]
2 (2.06 g, 7.25 mmol), potassium acetate (2.14 g, 21.8 mmol), bis(pinacolato)diboron (2.76 g, 10.9 mmol), PdCl2(dppf)·CH2Cl2 (0.18 g, 0.22 mmol) were dissolved in argon-purged 1,4-dioxane (45.0 ml). The resulting mixture was stirred at 120°C for more than 18 h under argon atmosphere. The mixture was extracted with ethyl acetate, and the organic layer was washed with water three times, dried over MgSO4, filtrated and evaporated under reduced pressure. The residue was purified by column chromatography on silica gel, eluting with hexane/ethyl acetate (v/v = 12/1) to give crude 3 (0.75 g, 2.3 mmol, 31%) as yellow liquid. 1H-NMR (500 MHz, CDCl3) δ 7.62 (d, J = 8.2 Hz, 2 H), 6.56 (d, J = 8.2 Hz, 2 H), 3.84 (s, 1 H), 3.12 (t, J = 7.2 Hz, 2 H), 1.61 (quin, J = 7.5 Hz, 2 H), 1.42–1.20 (m, 22 H), 0.88 (t, J = 6.6 Hz, 3 H) ppm (Figure S69).
2.3.3. Synthesis of 4’-(octylamino)-[1,1’-biphenyl]-4-carbonitrile (2B8) [Citation53,Citation54]
3 (0.71 g, 2.1 mmol), 4-bromobenzonitrile (4a) (0.46 g, 2.5 mmol), potassium phosphate (1.34 g, 6.34 mmol), Pd(PPh3)4 (0.07 g, 0.06 mmol) were dissolved in argon-purged toluene/water/methanol (10.0 ml/4.0 ml/2.0 ml). The resulting mixture was stirred at 100°C for more than 4 h under argon atmosphere. The mixture was extracted with dichloromethane, and the organic layer was washed with water three times, dried over MgSO4, filtrated and evaporated under reduced pressure. The residue was purified by column chromatography on silica gel, eluting with hexane/dichloromethane (v/v = 3/1) and recrystallised with the mixed solvent of hexane and dichloromethane to give refined 2B8 (0.31 g, 1.0 mmol, 47%) as a colourless solid. 1H-NMR (500 MHz, CDCl3) δ 7.65 (d, J = 8.2 Hz, 2 H), 7.61 (d, J = 8.2 Hz, 2 H), 7.45 (d, J = 8.5 Hz, 2 H), 6.70 (d, J = 7.9 Hz, 2 H), 3.16 (t, J = 7.2 Hz, 2 H), 1.65 (quin, J = 7.0 Hz, 2 H), 1.44–1.28 (m, 10 H), 0.89 (t, J = 6.7 Hz, 3 H) ppm (Figure S72); 13C-NMR (126 MHz, CDCl3) δ 149.1, 145.7, 132.5, 128.1, 127.2, 126.3, 119.4, 112.9, 109.0, 43.8, 31.8, 29.5, 29.4, 29.3, 27.2, 22.7, 14.1 ppm (Figure S73); FT-IR (KBr) 3385 cm−1, 2219 cm−1 (Figure S127); HRMS(EI) Calcd for C21H26N2: 306.4508, Found 306.2096 (Figure S131).
2.3.4. Synthesis of 4-bromo-N-methyl-N-octylaniline (6) [Citation55]
4-Bromo-N-methylaniline (5) (3.3 ml, 27 mmol), potassium carbonate (5.65 mg, 40.9 mmol), 1-iodooctane (12.0 ml, 66.5 mmol) were dissolved in anhydrous N,N-dimethylformamide (45.0 ml). The resulting mixture was stirred at 80°C for 21 h under argon atmosphere. The mixture was extracted with hexane/ethyl acetate (v/v = 5/1), and the organic layer was washed with water three times, dried over MgSO4, filtrated and evaporated under reduced pressure. The residue was purified by column chromatography on silica gel, eluting with hexane/dichloromethane (v/v = 4/1) to give crude 6 (6.53 g, 21.9 mmol, 81.0%) as yellow liquid. 1H-NMR (500 MHz, CDCl3) δ 7.27 (d, J = 8.9 Hz, 2 H), 6.54 (d, J = 8.9 Hz, 2 H), 3.26 (t, J = 7.5 Hz, 2 H), 2.89 (s, 3 H), 1.55–1.52 (m, 2 H), 1.31–1.25 (m, 10 H), 0.88 (t, J = 6.6 Hz, 3 H) ppm (Figure S88).
2.3.5. Synthesis of N-methyl-N-octyl-4-(4,4,5,5-tetramethyl-1,3,2-dioxaborolan-2-yl)aniline (7)
This synthesis was used in the same way as synthesis of 3. The residue was purified by column chromatography on silica gel, eluting with hexane/ethyl acetate (v/v = 12/1) on silica gel chromatography to give crude 7 (1.29 g, 3.73 mmol, 47%) as yellow liquid. 1H-NMR (500 MHz, CDCl3) δ 7.66 (d, J = 8.5 Hz, 2 H), 6.64 (d, J = 8.5 Hz, 2 H), 3.33 (t, J = 7.5 Hz, 2 H), 2.95 (s, 3 H), 1.62–1.55 (m, 2 H), 1.35–1.25 (m, 22 H), 0.88 (t, J = 6.6 Hz, 3 H) (Figure S89).
2.3.6. Synthesis of 4’-(methyl(octyl)amino)-[1,1’-biphenyl]-4-carbonitrile (3B8)
3B8 was synthesised from 7 and 4a by using the same method as the synthesis of 2B8. The residue was purified by column chromatography on silica gel, eluting with hexane/ethyl acetate (v/v = 12/1) on silica gel chromatography and recrystallised with hexane to give refined 3B8 (0.08 g, 0.25 mmol, 21%) as colourless solid. 1H-NMR (500 MHz, CDCl3) δ 7.60–7.69 (m, 4 H), 7.50 (d, J = 8.9 Hz, 2 H), 6.75 (d, J = 8.9 Hz, 2 H), 3.36 (t, J = 7.5 Hz, 2 H), 3.00 (s, 3 H), 1.67–1.56 (m, 2 H), 1.43–1.13 (m, 10 H), 0.89 (t, J = 6.9 Hz, 3 H) ppm (Figure S90); 13C-NMR (126 MHz, CDCl3) δ 149.6, 145.6, 132.5, 128.0, 126.2, 125.6, 119.5, 112.1, 108.8, 52.6, 38.4, 31.8, 29.5, 29.3, 27.2, 26.8, 22.7, 14.1 ppm (Figure S91).
3. Results and discussion
3.1. Synthesis and characterisation
We synthesised D-π-A type biphenyl derivatives and tolane derivatives with alkylamino groups as donor moieties and cyano groups as acceptor moieties. For convenience, we designate the biphenyl derivatives with alkylamino groups as ‘secondary amine-type biphenyl derivatives (2Bn)’ and those with dialkylamino groups as ‘tertiary amine-type biphenyl derivatives (3Bn)’. Similarly, for the tolane derivatives, compounds with an alkylamino group are termed ‘secondary amine-type tolane derivatives (2Tn)’ and those with a dialkylamino group as ‘tertiary amine-type tolane derivatives (3Tn)’. The synthetic procedures for 2Bn, 2Bn[2F], 2Bn[3F], 2BEH[3F], 2Bn[3, 5F], 3Bn, 3Bn[3F], 2Tn, 2Tn[2F], 2Tn[3F], 3Tn, 3Tn[2F] and 3Tn[3F] (n = 6, 8 and 10) are shown in . (See Supporting Information for further details).
Scheme 1. Synthesis of the biphenyl derivatives. (i) 1. C12H25SO4Na, NaHCO3, 2. RBr, solvent: H2O; (ii) Bis(pinacolato)diboron, Pd(dppf)Cl2·CH2Cl2, AcOK, solvent: 1,4-Dioxane; (iii) Pd(PPh3)4, K3PO4, solvent: Toluene/H2O/MeOH; (iv) C8H17I, K2CO3, solvent: DMF.
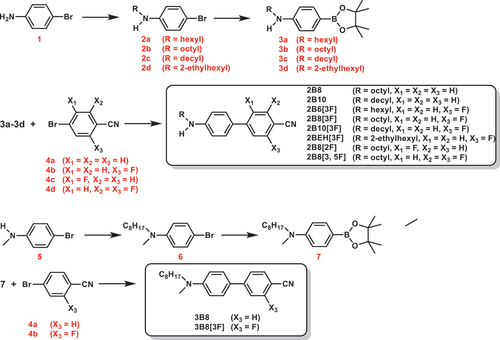
Biphenyl derivatives were synthesised via Suzuki-Miyaura cross-coupling reactions [Citation53,Citation54] involving the corresponding arylboronic acids (3 or 7) and 4-bromobenzonitrile derivatives (4a-4d) (). Secondary amine-type tolane derivatives (2T8, 2T10, 2T8[2F], 2T8[3F], 2T10[3F]) were synthesised from the corresponding Boc-protected aniline derivatives (12a, 12b) and (fluorinated) 4-ethynylbenzonitrile (9a-9c) by Sonogashira cross-coupling reaction [Citation56,Citation57]. Deprotection of the trimethylsilyl group of 4-((trimethylsilyl)ethynyl)benzonitrile (8a) was carried out using a methanol suspension of potassium carbonate (procedure a). However, the reaction with fluorinated substrates (8b and 8c) did not proceed using potassium carbonate. In this case, tetrabutylammonium fluoride was effective (procedures b). When hydrochloric acid was used to deprotect the tert-butoxycarbonyl group (procedures c), by-products were formed, but this problem was solved by using trifluoroacetic acid and strictly controlling the concentration and stirring time of the reaction system (procedures d). Tertiary amine-type tolane derivatives (3T8, 3T8[2F], 3T8[3F]) were synthesised from the corresponding alkynylated aryl (15) and (fluorinated) 4-bromobenzonitrile (4a-4c) via Sonogashira cross-coupling reaction [Citation56,Citation57] ().
Scheme 2. Synthesis of the tolan derivatives. (i) Pd(PPh3)4, CuI, PPh3, solvent: Et3N/THF; (ii) condition a or b: a = K2CO3, THF/MeOH, b = [N(C4H9)4]+F−; (iii) 1. Et3N, solvent: H2O/THF, 2. Boc2O; (iv) RX = 1-iodooctane, 1-bromodecane, NaH, solvent: THF; (v) Pd(PPh3)2Cl2, CuI, PPh3, solvent: i-Pr2NEt; (vi) condition c or d: c = 1. CF3COOH, CH2Cl2, 2. NaHCO3aq, d = 1. HClaq, EtOAc, 2. NaHCO3aq. (vii) Pd2(PPh3)2Cl2, CuI, PPh3, solvent: piperidine; (viii) [N(C4H9)4]+F−, solvent: THF; (ix) Pd(PPh3)2Cl2, CuI, PPh3, solvent: i-Pr2NEt.
![Scheme 2. Synthesis of the tolan derivatives. (i) Pd(PPh3)4, CuI, PPh3, solvent: Et3N/THF; (ii) condition a or b: a = K2CO3, THF/MeOH, b = [N(C4H9)4]+F−; (iii) 1. Et3N, solvent: H2O/THF, 2. Boc2O; (iv) RX = 1-iodooctane, 1-bromodecane, NaH, solvent: THF; (v) Pd(PPh3)2Cl2, CuI, PPh3, solvent: i-Pr2NEt; (vi) condition c or d: c = 1. CF3COOH, CH2Cl2, 2. NaHCO3aq, d = 1. HClaq, EtOAc, 2. NaHCO3aq. (vii) Pd2(PPh3)2Cl2, CuI, PPh3, solvent: piperidine; (viii) [N(C4H9)4]+F−, solvent: THF; (ix) Pd(PPh3)2Cl2, CuI, PPh3, solvent: i-Pr2NEt.](/cms/asset/9d26087c-dde8-4520-b73e-c3c09bbbf6e6/tlct_a_2381547_sch0002_oc.jpg)
The synthesised compounds were identified using 1H-NMR and 13C-NMR spectroscopy. HR–EI mass spectroscopy and FT-IR spectroscopy were employed to characterise 2B8, 2B8[3F], 2T8 and 2T8[3F].
3.2. Phase transition behaviours
The phase transition behaviour of the synthesised compounds was evaluated using DSC and POM. In POM observations, we observed the phase transition behaviours upon cooling and heating at a rate of 10°C min−1 for a molten sample sandwiched between untreated glass plates. However, it is noteworthy that 2B8 exhibited a distinctive preference, orienting the nematic director perpendicular to untreated glass substrates. Consequently, POM of 2B8 was conducted for a molten sample sandwiched between a polyimide-coated substrate with planar alignment treatment. The temperatures and enthalpies determined by DSC analysis and DSC thermograms are the result of the third heating/cooling cycle.
3.2.1. Phase transition behaviours of biphenyl derivatives
The phase transition temperatures and enthalpies of the biphenyl derivatives (2B8–3B8[3F]) determined by DSC analysis are summarised in . The DSC thermograms of these compounds are shown in and Supporting Information.
Figure 1. (Colour online) (a) A POM image at 86°C during cooling (left) and DSC thermograms (right) of 2B8. (b) A POM image at 40°C during cooling (left) and DSC thermograms (right) of 2B8[3F].
![Figure 1. (Colour online) (a) A POM image at 86°C during cooling (left) and DSC thermograms (right) of 2B8. (b) A POM image at 40°C during cooling (left) and DSC thermograms (right) of 2B8[3F].](/cms/asset/0eab598c-a24e-42c8-9b9d-0342217a0a75/tlct_a_2381547_f0001_oc.jpg)
Table 1. Phase transition behaviours of the compounds.
2B8 and 2B10.
2B8 formed an enantiotropic nematic phase, exhibiting two distinct endothermic peaks at 84.0°C and 92.7°C, along with corresponding exothermic peaks at 90.7°C and 64.8°C, respectively, as evidenced by the heating and cooling DSC thermograms ( (a)). Under a POM, 2B8 displayed a schlieren texture characteristic of the nematic phase within the temperature range between these two peaks ( and Figure S2). Notably, the high-temperature transition exhibited an enthalpy change as small as 2% of that measured for the low-temperature transition. The value of TNI of 2B8 upon cooling is higher than those of 9CB (4′-(alkyl)[1,1′-biphenyl]-4-carbonitriles) [Citation30], 8OCB (4′-(octyloxy)[1,1′-biphenyl]-4-carbonitriles) [Citation30] and 8SCB (4′-(octylthio)[1,1′-biphenyl]-4-carbonitriles) [Citation58].
Similarly, 2B10 also formed an enantiotropic nematic phase, exhibiting two endothermic and exothermic peaks in the heating and cooling DSC thermograms (Figure S6) and displaying a schlieren texture under a POM (Figures S4 and S5). Compared to 2B8, 2B10 demonstrated higher transition temperatures and a narrower nematic LC temperature range. While our findings align with previously reported nematic phase formation and phase transition temperatures [Citation50], there is a discrepancy with the literature regarding the formation of smectic A phases by 2B8 and 2B10 during the heating process. The literature presents ambiguities in the phase transition observations, failing to specify whether the LCs were monotropic or enantiotropic.
2B8[3F] and 2B10[3F]
2B8[3F] exhibited a monotropic nematic phase. The DSC thermograms showed endothermic peaks at 42.1°C and 52.6°C, an exothermic peak at 43.2°C during heating and exothermic peaks at 52.7°C and 21.2°C during cooling. POM observations revealed a schlieren texture () at 40°C during cooling, whereas only the melting of the crystals was observed during heating. WAXD measurements of 2B8[3F] were performed at 42°C, 45°C, 49°C and 53°C to investigate, in detail, the phase transition behaviour during heating (Figures S9–S16). No discernible disparity in the WAXD patterns between 42°C and 45°C was observed; however, as the temperature increased, the peaks broadened. Consequently, 2B8[3F] displayed a monotropic nematic phase. The endothermic peak at 42.1°C and the exothermic peak at 43.2°C are presumed to stem from crystal–crystal phase transition. The small transition enthalpies of these peaks suggest small changes in packing between this transition, which explains the absence of this transition in both POM and WAXD measurements.
Similarly, 2B10[3F] exhibited a monotropic nematic phase, displaying a schlieren texture in the range of 58.2–52.7°C during cooling (Figure S18). 2B10[3F] had higher melting and clearing points and a narrower nematic phase temperature range compared to 2B8[3F]. Although the DSC thermograms of 2B10[3F] did not exhibit any peaks indicative of the mesophase (Figure S19), it is hypothesised that the isotropic–mesophase transition peak and mesophase–crystal transition peak overlapped in the DSC measurement owing to the narrow temperature range exhibiting the mesophase relative to the cooling rate. The two peaks at 39.7°C and 45.7°C during heating, along with the peak at 38.8°C during cooling, could be attributed to crystal–crystal phase transition, akin to that in 2B8[3F], as no anomalies were observed in POM observations. Compared to 2B8, 2B8[3F] features a 38.0°C lower clearing point and a 43.6°C lower melting point during cooling. The incorporation of one fluorine group ortho to the cyano group of the biphenyl derivative significantly lowered the phase transition temperature, resulting in monotropism. This trend was also evident in the phase transition behaviours of 2B10 and 2B10[3F]. The lateral introduction of fluorine groups disrupts the molecular packing and reduces the intermolecular forces, consequently lowering the phase transition temperature [Citation59,Citation60]. The lower phase transition temperatures of 2B8[3F] and 2B10[3F] may be attributed to these factors.
2B6[3F], 2BEH[3F] and 2B8[2F]
In contrast, neither 2B6[3F] nor 2BEH[3F] exhibited mesophases (Figures S7 and S20). Similarly, 2B8[2F] exhibited no mesophase (Figure S21). The introduction of one fluorine group in the meta position to the cyano group resulted in the loss of mesophase in biphenyl. Generally, the phase transition temperature of 2B8[2F] was higher than those of 2B8 and 2B8[3F]. The introduction of a fluorine group at the meta position may lead to H–F interactions, thereby enhancing the interaction aspects of 2B8[2F]. Dihedral angle scan calculations using density functional theory (DFT) showed that the dihedral angles of 2B1 and 2B1[2F] exhibited minima at approximately 30º and 35º, respectively (see Figure S135). This suggests that the increase in the biphenyl dihedral angle owing to fluorine steric repulsion is small. Consequently, 2B8[2F] primarily cannot form mesophases via H–F interactions.
2B8[3, 5F]
2B8[3, 5F] exhibits a monotropic nematic phase, indicating exothermic peaks at 3.7°C in the cooling DSC thermograms (Figure S24) and a schlieren texture during POM observations under ice-cold conditions (Figure S23), confirming its monotropic nematic phase. Notably, the clearing point of 2B8[3, 5F] during cooling was 87.0°C lower than that of 2B8 and a further 49.0°C lower than that of 2B8[3F]. This significant reduction in the phase transition temperature suggests that the introduction of two fluorine groups at the ortho position of the cyano group in biphenyl derivatives more effectively suppresses molecular packing and reduces intermolecular forces.
3B8 and 3B8[3F]
Neither 3B8 nor 3B8[3F] exhibited any mesophases (Figures S25 and S26). A previous report [Citation50] indicated an isotropic–nematic phase transition of 3B8 at 74.0°C during cooling, even though this phenomenon was not observed in our study. In contrast to 2B8 and 2B8[3F], which showed mesophase, 3B8 and 3B8[3F] did not show any mesophase. These results indicate that tertiary amine-type biphenyl derivatives are disadvantageous for the appearance of mesophases. The introduction of a methyl group may hinder the alkyl chain aggregation and inhibit packing. A further possibility is that the mesophase may be stabilised by hydrogen bonding in the case of 2B8[3F]. Such stabilisation by hydrogen bonding has been reported [Citation61–63].
3.2.2. Phase transition behaviours of tolane derivatives
The phase transition behaviours of the tolane derivatives (2T8–3T8[2F]) are summarised in . The DSC thermograms of these compounds are shown in and Supporting Information.
Figure 2. (Colour online) (a) A POM image at 86°C during cooling (left) and DSC thermograms (right) of 2T8. (b) A POM image at 45°C during cooling (left) and DSC thermograms (right) of 2T8[3F].
![Figure 2. (Colour online) (a) A POM image at 86°C during cooling (left) and DSC thermograms (right) of 2T8. (b) A POM image at 45°C during cooling (left) and DSC thermograms (right) of 2T8[3F].](/cms/asset/75b4f787-45d0-497f-b228-883a24074bf4/tlct_a_2381547_f0002_oc.jpg)
2T8 and 2T10
Both 2T8 and 2T10 displayed a monotropic nematic phase. The two peaks were observed during both heating and cooling in the DSC measurements ( and Figure S32). Additionally, in the POM observations during cooling, schlieren textures were evident for temperatures between these two peaks ( and Figure S31). Conversely, only crystal melting was observed during heating. The presence of two peaks in the DSC measurements during heating suggests a crystal–crystal phase transition with a small packing change.
2T8[3F] and 2T10[3F]
2T8[3F] showed a monotropic nematic phase. shows the DSC thermogram of 2T8[3F]. During the heating process, endothermic peaks were observed at 72.7°C and 75.8°C, while the exothermic peaks were observed at 60.6°C and 31.5°C during the cooling process. The POM observations revealed only crystal melting during heating, whereas the schlieren texture () was observed at 59.0°C during cooling. The endothermic peak at 72.7°C is considered a crystal–crystal phase transition as no change was observed in the POM findings.
Similar to 2T8[3F], 2T10[3F] also exhibited a monotropic nematic phase. The POM observation of 2T10[3F] outcomes during cooling showed crystallisation at 49.5°C alongside the schlieren structure (Figure S37). The DSC thermograms of 2T10[3F] exhibited endothermic and exothermic peaks at 70.1°C and 57.9°C, respectively, during heating and cooling (Figure S38), indicating a slow crystallisation rate. Notably, its clearing point was lower compared to that of 2T8[3F]. Similar to the biphenyl derivatives, the introduction of one fluorine group ortho to the cyano group of the tolane derivatives significantly lowered the phase transition temperature.
2T10[2F]
In contrast, 2T10[2F] indicated an enantiotropic nematic phase, showing two endothermic peaks at 80.6°C and 85.0°C during heating and two exothermic peaks at 82.7°C and 72.7°C during cooling in the DSC thermograms (Figure S42). Schlieren texture was observed at temperatures between these two peaks during both heating and cooling in the POM observations (Figures S40 and S41). The introduction of one fluorine group at the meta position of the cyano group of the tolane derivatives altered the monotropic to enantiotropic properties and reduced the phase transition temperature. This trend was different from that of the biphenyl derivatives. It was speculated that the difference in the aspect ratios of the mesogens caused this result. In DSC measurement during cooling, a more exothermic peak was observed at 61.9°C, likely indicating the crystal–crystal phase transition, as no observation was made by POM.
3T8, 3T8[3F] and 3T8[2F]
3T8, 3T8[3F] and 3T8[2F] did not exhibit mesophases. Similar to the biphenyl derivatives, tertiary amine-type tolane derivatives are disadvantageous for the appearance of mesophases.
3.3. Photophysical properties
3.3.1. Photophysical properties of biphenyl derivatives
The absorption and fluorescence spectra and quantum yields (Φ) of the biphenyl derivatives (2B8, 2B8[2F], 2B8[3F], 2B8[3, 5F], 3B8, 3B8[3F]) were measured in the THF solution and the solid-state (polycrystalline solid). The photophysical properties of the biphenyl derivatives are summarised in and .
Figure 3. (Colour online) Absorption (dashed line) and fluorescence (solid line) spectra of the biphenyl derivatives (a) in THF solution and (b) in the solid state.
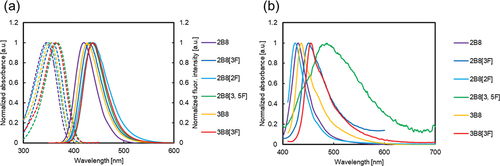
Table 2. The photophysical properties of the compounds.
The absorption and fluorescence spectra of all biphenyl derivatives exhibited similarities. Time-dependent density functional theory (TD-DFT) calculations at the B3LYP/6–31 g (d) level suggested that the maximum absorption wavelengths (λabs) of all biphenyl derivatives were attributed to the HOMO-LUMO transition, with calculated λabs values showing good agreement with the experimental data (Figures S136, S137, Table S3). Although the molar absorption coefficients (ε) varied in the range 18,000–34000 M−1cm−1, DFT calculations showed no significant differences in the oscillator strengths (see Supporting Information).
In the THF solution, the λabs, maximum fluorescence wavelength (λfl) and Φ of 2B8 were 346 nm, 420 nm and 0.95, respectively, with 2B8 exhibiting fluorescence in the visible region. The λabs and λfl of 2B8[3F] were 358 nm and 434 nm, respectively, and approximately 13 nm red-shifted from those of 2B8 owing to the electron-withdrawing fluorine group. The Φ of 2B8[3F] was 0.96. Similar red-shifts in λabs and λfl, attributed to the introduction of fluorine groups, were observed in 2B8[2F] and 2B8[3, 5F], with Φ values remaining consistent. The λabs and λfl of 3B8 were 353 nm and 428 nm, respectively, and both red-shifted by approximately 7 nm compared to that of 2B8. This was attributed to the increased electron-donating of the alkyl amino group owing to the introduction of a methyl group. Furthermore, the λabs and λfl of 3B8[3F] were red-shifted by approximately 20 nm compared to that of 2B8 owing to the introduction of fluorine and methyl groups. All compounds have Φ values above 0.93, suggesting that the presence of fluorine groups or variations between secondary and tertiary amine-type biphenyl derivatives have a negligible effect on Φ.
The solid-state fluorescence spectra are shown in (b). The λfl values of 2B8, 2B8[3F], 2B8[2F] and 2B8[3, 5F] were 430, 451, 424 and 487 nm, respectively, all falling within the visible range. The correlation between λfl of 2B8 and 2B8[3F] differed between the THF solution and the solid state, indicating distinct behaviours influenced by intermolecular interactions in aggregated systems such as the solid state. The λfl of 3B8 and 3B8[3F] in the solid state were 437 nm and 455 nm, respectively. The Φ values of the biphenyl derivatives in the solid state ranged from 0.07 to 0.40, typical of most dyes, with lower values compared to those in solution. Φ in the solid state depended on many factors such as the formation of the aggregates [Citation64–66], overlap of aromatic rings in the packing structures [Citation67] and intermolecular distance [Citation68,Citation69], making it challenging to establish a direct correlation between the molecular structure and Φ.
3.3.2. Photophysical properties of tolane derivatives
The photophysical properties of the tolane derivatives (2T8, 2T8[3F], 2T10[2F], 3T8, 3T8[3F], 3T8[2F]) are summarised in and .
Figure 4. (Colour online) Absorption (dashed line) and fluorescence (solid line) spectra of the tolane derivatives (a) in THF solution and (b) in the solid state.
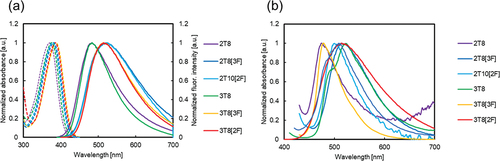
The absorption and fluorescence spectra of all the tolane derivatives exhibited similarities. DFT calculations suggest that λabs of all tolane derivatives correspond to the HOMO-LUMO transition, with calculated λabs values closely aligning with the experimental value (Figures S136 and S137, Table S3).
The λabs and λfl of 2T8 in THF were 369 nm and 484 nm, respectively. The λabs and λfl of 2T8, compared with those of 2B8, exhibited a red-shift of 23 nm and 64 nm, respectively, owing to the conjugation length of 2T8. Notably, the Stokes shift of 2T8 was 1347 cm−1 larger than that of 2B8, a trend consistent across various tolane derivatives. Similar observations were reported for tolane derivatives with dimethylamino and formyl groups at both ends [Citation70,Citation71]. The substitution of mesogens with a fluorine group, as in 2T8[3F] and 2T10[2F], led to red-shifted λabs and λfl, mirroring the behaviour observed in biphenyl derivatives. The λabs and λfl of 3T8 in THF were 376 nm and 483 nm, respectively. The λabs of 3T8, which is the tertiary amine type, was 7 nm red-shifted, compared with that of 2T8. Conversely, the introduction of a methyl group resulted in blue-shifts of λfl for 3T8[3F] and 3T8[2F]. Notably, the Φ of the secondary amine-type tolane derivatives (2T8, 2T8[3F], 2T10[2F]) ranged from 0.09 to 0.13, while those of the tertiary amine-type tolane derivatives (3T8, 3T8[3F], 3T8[2F]) ranged from 0.21 to 0.30. The TD-DFT calculations and the transient absorption spectra analysis of p-N,N-dimethylamino-p′-cyanodiphenylacetylne (3T1) suggested the formation of an intramolecular charge transfer (TICT) state, wherein aromatic rings are twisted perpendicularly to each other [Citation72]. In addition, a previous study reported Φ and fluorescence lifetime of 3T1 [Citation71], supporting its quenching due to the formation of the TICT state [Citation73,Citation74]. Based on these results, the tolane derivatives synthesised in this study were also quenched owing to the formation of a TICT state.
The photophysical properties of the solid state are shown in (b). For instance, the λfl of 2T8, at 474 nm, exhibited red-shift compared to that of biphenyl derivatives in THF and solid-state, consistent with the extended conjugation. The λfl of 2T8[3F] and 2T10[2F] were 515 nm and 500 nm, respectively, exhibiting red-shift of 41 nm and 26 nm compared to that of 2T8. For 3T8, 3T8[2F] and 3T8[3F], the λfl values were 524 nm, 508 nm and 478 nm, respectively. Among the secondary amine-types (2T8, 2T8[3F], 2T10[2F]), the Φ of 2T8 and 2T10[2F] were less than 0.10. Conversely, 2T8[3F] exhibited a high Φ (0.76), with its luminescence significantly enhanced in the solid state. Furthermore, Φ of the tertiary amine types (3T8, 3T8[3F], 3T8[2F]) were greater than 0.59, surpassing those in the THF solution.
3.3.3. Room-temperature nematic LC mixture
To achieve room-temperature nematic liquid crystal by blending, we decided to mix other compounds based on 2B8[3F], which has a particularly low phase transition temperature. Molecules with a similar structure to 2B8[3F] were preferred for blending because of their photophysical properties. Furthermore, from the point of view of mesophase stability, molecules with mesophase were preferred to those without. Therefore, 2B8, 2B10, 2B10[3F] and 2BEH[3F] were mixed in different mixing ratios with respect to 2B8[3F]. The compounds were dissolved in THF for spectroscopic measurements and stirred for a sufficient amount of time using an ultrasound. The blended samples were obtained by evaporating the solvent. The weight fraction ratios of the blended compounds and their phase transition behaviours are listed in Table S1. As a result of the trial, sample 22 (the weight fractions of 2B8, 2B10, 2B8[3F], 2B10[3F] and 2BEH[3F] in this sample are 7.3, 6.9, 69.6, 7.3 and 8.9 wt%, respectively) retained the nematic phase around 25°C.
The fluorescence spectra and Φ of sample 22 were measured at 25°C. The excitation wavelength for these measurements was 390 nm, consistent with the values used for the solid-state measurements of 2B8, 2B10 and 2BEH[3F].
λfl was 469 nm, exhibiting a red-shift of more than 35 nm compared to that of the THF solution states of any of the compounds (2B8, 2B10, 2B8[3F], 2B10[3F] and 2BEH[3F]) in sample 22, and more than 17 nm compared to that in their solid state (). The Φ of the blend sample was 0.18, a value lower than that observed in the THF solution and solid states of these compounds. The dimer of fluorinated tolane forms J-aggregates, and the fluorescence is red-shifted in the LC phase [Citation75]. Conversely, n-alkyl cyano biphenyl forms excimers, leading to red-shifted fluorescence in the LC phase compared to the solution and the solid state [Citation76,Citation77]. We also measured λfl, of sample 22 in THF solution (concentrations were 10−5, 10−4, 10−3 and 10−2 mol/L) to investigate the effect of such intermolecular interactions on λfl. The λfl values of sample 22 in the THF solution are summarised in Figure S63. The λfl values range from 428 to 434 nm, representing a blue-shift of 35 nm compared to that in the LC phase. The consistent λfl values across different concentrations suggest that the red-shift observed in the LC phase is not solely attributed to intermolecular interactions, as the λfl values remain unchanged even in high concentration solution. The blend stabilises the nematic phase at 25°C, enabling fluorescence spectra and quantum yield measurements at this temperature.
4. Conclusion
We synthesised push-pull-type biphenyl derivatives and tolane derivatives with alkylamino groups as donor moieties and cyano groups as acceptor moieties and investigated their phase transition behaviours and photophysical properties. Among the biphenyl derivatives, 2B8, 2B10, 2B8[3F], 2B10[3F] and 2B8[3, 5F] exhibited a nematic phase, notably with 2B8[3F] showing a particularly low-temperature range of 52.7–21.2°C during cooling. In contrast, the tertiary amine type did not exhibit a mesophase. Notably, these biphenyl derivatives exhibited blue fluorescence in the THF solution and solid state. Among the tolane derivatives, 2T8, 2T10, 2T8[3F], 2T8[3F] and 2T10[2F] demonstrated a nematic phase with 2T8[3F] exhibiting a temperature range of 60.5–31.5°C during cooling. Conversely, the tertiary amine-type tolane derivatives, similar to the tertiary amine-type biphenyl derivatives, did not exhibit a mesophase. These tolane derivatives showed green fluorescence both in the THF solution and in the solid state. Additionally, we successfully developed a room-temperature luminescent LC by blending these compounds. Fluorescence spectroscopic and quantum yield analysis of the blended samples at 25°C revealed a red-shift in its maximum fluorescence wavelength. This study proves the utilisation of the push-pull structure in designing LC molecules with both low-phase transition temperatures and luminescent properties. The successful synthesis and characterisation of push-pull-type luminescent LCs broaden the scope of molecular design strategies for achieving desired fluorescence emission wavelengths and phase transition behaviours. Future research could focus on optimising the molecular structures to further enhance the luminescence efficiency and broaden the applications of these materials in areas such as displays, sensors and light-emitting devices.
Supplemental Material
Download PDF (5 MB)Acknowledgments
The authors thank Mr Masato Koizumi (Materials Analysis Division, Tokyo Institute of Technology) for the HRMS measurements. The division is independent of our laboratory to ensure fairness. This project was supported in part by MEXT/JSPS KAKENHI grants 23H02036, 23K02036 and17H05145 (G.K.). We also thank the Iketani Science and Technology Foundation for finacial support.
Disclosure statement
No potential conflict of interest was reported by the author(s).
Supplementary material
Supplemental data for this article can be accessed online at https://doi.org/10.1080/02678292.2024.2381547
References
- Ranjithaa BS, Alassar M, Shanker G. Luminous and fluorescent cyanobiphenyls exhibiting super-cooled nematic phases. Liq Cryst Early Access. 2024:1–12. doi: 10.1080/02678292.2023.2294959
- Sawatari Y, Shimomura Y, Takeuchi M, et al. Supramolecular liquid crystals from the dimer of L-shaped molecules with tertiary amide end groups. Aggregate. 2024;5(507e). doi: 10.1002/agt2.507
- Yang ZW, Huang XY, Cheng JC, et al. Novel fluorescence liquid crystals based on thiophene-vinylnitrile Schiff-base derivatives. Liq Cryst. 2023;50(15):2521–2528. doi: 10.1080/02678292.2022.2028316
- Wu XJ, Niu XL, Zhu SB, et al. A novel multi-stimuli responsive fluorescence liquid crystal material with aggregation-induced emission effect. Liq Cryst. 2023;50(6):1035–1045. doi: 10.1080/02678292.2023.2200263
- da Silva Fn, Silva EO, dos Santos G, et al. Unlocking the potential of 2,1,3-benzoxadiazole-based luminescent liquid crystals. Liq Cryst. 2023;50(11–12):1688–1698. doi: 10.1080/02678292.2023.2202160
- Chang XY, Zhong WY, Han J. Synthesis, mesomorphic and fluorescent properties of stilbene-containing 1,3,4-oxadiazole compounds. Liq Cryst. 2023;50(11–12):1709–1714. doi: 10.1080/02678292.2023.2203104
- Weber CSB, Tavares A, Araújo B, et al. Rod-shaped cyanoacrylic derivatives with D-π-A architecture: synthesis, thermal, photophysical and theoretical studies. Liq Cryst. 2023;50(2):278–288. doi: 10.1080/02678292.2022.2122088
- Zharnikova NV, Akopova OB, Kazak AV, et al. Effect of alkyloxy substituents on mesomorphic and photophysical properties of star-shaped tristriazolotriazines. Liq Cryst. 2023;50(7–10):1296–1309. doi: 10.1080/02678292.2023.2196523
- Luo S, Yang G, Zhang M, et al. Difluoromethoxyl bridged substituted 2-cyano-pyrrole based pure organic luminescent liquid crystals towards white light emitting single-molecule. Liq Cryst. 2023;50(11–12):1699–1708. doi: 10.1080/02678292.2023.2203123
- Yamada S, Konno T. Development of donor-π-acceptor-type fluorinated tolanes as compact condensed phase luminophores and applications in photoluminescent liquid-crystalline molecules. Chem Rec. 2023;23(9):e202300094. doi: 10.1002/tcr.202300094
- Yamada S, Yoshida K, Uto E, et al. Development of photoluminescent liquid-crystalline dimers bearing two fluorinated tolane-based luminous mesogens. J Mol Liq. 2023;363:119884. doi: 10.1016/j.molliq.2022.119884
- Voskuhl J, Giese M. Mesogens with aggregation-induced emission properties: materials with a bright future. Aggregate. 2022;3(1):e124. doi: 10.1002/agt2.124
- Srinatha MK, Zeba A, Ganjiwale A, et al. The influences of lateral groups on 4-cyanobiphenyl-benzonitrile-based dimers. Liq Cryst. 2022;49(2):217–229. doi: 10.1080/02678292.2021.1956610
- Matraszek J, Grzeskiewicz K, Górecka E, et al. Fluorescent bent-core mesogens with thiophene-based central unit. Liq Cryst. 2020;47(12):1803–1810. doi: 10.1080/02678292.2020.1729427
- Yang X, Zhou M, Wang Y, et al. Electric-field-regulated energy transfer in chiral liquid crystals for enhancing upconverted circularly polarized luminescence through steering the photonic bandgap. Adv Mater. 2020;32(24):2000820. doi: 10.1002/adma.202000820
- Arakawa Y, Sasaki S, Igawa K. Birefringence and photoluminescence properties of diphenylacetylene-based liquid crystal dimers. New J Chem. 2020;44(40):17531–17541. doi: 10.1039/D0NJ04426A
- Pratap G, Malkar D, Varathan E, et al. 3-cyano thiophene-based π-conjugated mesogens: XRD and 13C NMR investigations. Liq Cryst. 2019;46(5):680–693. doi: 10.1080/02678292.2018.1518548
- Buchs J, Gessner A, Heyne B, et al. Fluorescent liquid crystals with rod-shaped π-conjugated hydrocarbon core. Liq Cryst. 2019;46(2):281–298. doi: 10.1080/02678292.2018.1499150
- Zhang W, Sakurai T, Aotani M, et al. Highly fluorescent liquid crystals from excited-state intramolecular proton transfer molecules. Adv Opti Mater. 2019;7(2):1801349. doi: 10.1002/adom.201801349
- Padalkar VS, Tsutsui Y, Sakurai T, et al. Optical and structural properties of ESIPT inspired HBT–fluorene molecular aggregates and liquid crystals. J Phys Chem B. 2017;121(45):10407–10416. doi: 10.1021/acs.jpcb.7b08073
- Ghosh T, Lehmann M. Recent advances in heterocycle-based metal-free calamitics. J Mater Chem C. 2017;5(47):12308–12337. doi: 10.1039/C7TC03502K
- Wöhrle T, Wurzbach I, Kirres J, et al. Discotic liquid crystals. Chem Rev. 2016;116(3):1139–1241. doi: 10.1021/acs.chemrev.5b00190
- Fleischmann EK, Zentel R. Liquid‐crystalline ordering as a concept in materials science: from semiconductors to stimuli‐responsive devices. Angew Chem Int Ed. 2013;52(34):8810. doi: 10.1002/anie.201300371
- Marcelo NF, Vieira AA, Cristiano R, et al. Polarized light emission from aligned luminescent liquid crystal films based on 4,7-disubstituted-2,1,3-benzothiadiazoles. Synth Met. 2009;159(7–8):675–680. doi: 10.1016/j.synthmet.2008.12.014
- Sagara Y, Kato T. Stimuli-responsive luminescent liquid crystals: change of photoluminescent colours triggered by a shear-induced phase transition. Angew Chem Int Ed. 2004;47(28):5175–5178. doi: 10.1002/anie.200800164
- Giménez R, Piñol M, Serrano JL. Luminescent liquid crystals derived from 9,10-bis(phenylethynyl)anthracene. Chem Mater. 2004;16(7):1377–1383. doi: 10.1021/cm030582u
- O’Neill M, Kelly SM. Liquid crystals for charge transport, luminescence, and photonics. Adv Mater. 2003;15(14):1135–1146. doi: 10.1002/adma.200300009
- Salamonczyk M, Kovarova A, Svoboda J, et al. Switchable fluorescent liquid crystals. Appl Phys Lett. 2009;95(17):171901. doi: 10.1063/1.3250163
- Tsutsui Y, Zhang W, Ghosh S, et al. Electrically switchable amplified spontaneous emission from liquid crystalline phase of an AIEE-Active ESIPT molecule. Adv Optical Mater. 2020;8(14):1902158. doi: 10.1002/adom.201902158
- Gray GW, Harrison KJ, Nash JA. New family of nematic liquid crystals for displays. Electron Lett. 1973;6:130–131. doi: 10.1049/el:19730096
- Goodby JW. The nanoscale engineering of nematic liquid crystals for displays. Liq Cryst. 2011;38(11–12):1363–1387. doi: 10.1080/02678292.2011.614700
- Ozaki M, Matsuhisa Y, Yoshida H, et al. Photonic crystals based on chiral liquid crystal. Phys Status Solid A. 2007;204(11):3777–3789. doi: 10.1002/pssa.200776422
- Uchimura M, Watanabe Y, Araoka F, et al. Development of laser dyes to realize low threshold in dye-doped cholesteric liquid crystal lasers. Adv Mater. 2010;22:4473–4478. doi: 10.1002/adma.201001046
- Gevorgyan AH, Simoni F. Optical properties of heliconical cholesterics: effects of losses and photonic density of states. Liq Cryst. 2024:1–10. doi: 10.1080/02678292.2024.2346919
- Gong ZL, Li ZQ, Zhong YW. Circularly polarized luminescence of coordination aggregates. Aggregate. 2022;3(5):e177. doi: 10.1002/agt2.177
- He YR, Lin SY, Guo JB, et al. Circularly polarized luminescent self-organized helical superstructures: from materials and stimulus-responsiveness to applications. Aggregate. 2022;3(5):e141. doi: 10.1002/agt2.141
- Yang J, Zaho W, He W, et al. Liquid crystalline blue phase materials with three-dimensional nanostructures. J Mater Chem C. 2019;7(43):13352–13366. doi: 10.1039/C9TC04380B
- Cao W, Muñoz A, Palffy-Muhoray P, et al. Lasing in a three-dimensional photonic crystal of the liquid crystal blue phase II. Nat Mater. 2002;1(2):111–113. doi: 10.1038/nmat727
- Demus D. One century liquid crystal chemistry: from vorländer’s rods to disks, stars and dendrites. Mol Cryst Liq Cryst Technol Sec A. 2001;364(1):25–91. doi: 10.1080/10587250108024978
- Dąbrowski R, Kula P, Herman J. High birefringence liquid crystals. Crystals. 2013;3(3):443–482. doi: 10.3390/cryst3030443
- Arakawa Y, Kang S, Tsuji H, et al. The design of liquid crystalline bistolane-based materials with extremely high birefringence. RSC Adv. 2016;6(95):92845–92851. doi: 10.1039/C6RA14093A
- Arakawa Y, Kang S, Nakajima S, et al. Diphenyltriacetylenes: novel nematic liquid crystal materials and analysis of their nematic phase-transition and birefringence behaviours. J Mater Chem C. 2013;1(48):8094–8102. doi: 10.1039/C3TC31658K
- Arakawa Y, Nakajima S, Ishige R, et al. Synthesis of diphenyl-diacetylene-based nematic liquid crystals and their high birefringence properties. J Mater Chem. 2012;22(17):8394–8398. doi: 10.1039/C2JM16002A
- Tanaka T, Matsumoto A, Klymchenko AS, et al. Fluorescent solvatochromic probes for long-term imaging of lipid order in living cells. Adv Sci. 2024;11(17):2309721. doi: 10.1002/advs.202309721
- Klymchenko AS. Solvatochromic and fluorogenic dyes as environment-sensitive probes: design and biological applications. Acc Chem Res. 2017;50(2):366–375. doi: 10.1021/acs.accounts.6b00517
- Sasaki S, Igawa K, Konishi G. The effect of regioisomerism on the solid-state fluorescence of bis(piperidyl)anthracenes: structurally simple but bright AIE luminogens. J Mater Chem C. 2015;3(23):5940–5950. doi: 10.1039/C5TC00946D
- Niko Y, Kawauchi S, Konishi G. Solvatochromic pyrene analogues of prodan exhibiting extremely high fluorescence quantum yields in apolar and polar solvents. Chem Eur J. 2013;19(30):9760–9765. doi: 10.1002/chem.201301020
- Lord SJ, Conley NR, Lee HL, et al. A photoactivatable push−pull fluorophore for single-molecule imaging in live cells. J Am Chem Soc. 2008;130(29):9204–9205. doi: 10.1021/ja802883k
- Valeur B, Berberan–Santos MN. Molecular fluorescence, principles and application. Weinheim: Wiley-VCH; 2012.
- Ruoliene J, Adomeńs P, Tubelyt A, et al. Liquid crystalline 4-alkylamino-4’-cyanobiphenyls. Liq Cryst. 1981;78(1):211–216. doi: 10.1080/00268948108082157
- Chingakham BS, Veerababurao K, Akshaya KS, et al. Aqueous-mediated N-Alkylation of amines. Eur J Org Chem. 2007;2007(8):1369–1377. doi: 10.1002/ejoc.200600937
- Lee DR, Han SH, Lee CW, et al. Bis(diphenyltriazine) as a new acceptor of efficient thermally activated delayed fluorescent emitters. Dyes Pigm. 2018;151:75–80. doi: 10.1016/j.dyepig.2017.12.048
- Miyaura N, Suzuki A. Palladium-catalyzed Cross-coupling reactions of organoboron compounds. Chem Rev. 1995;95(7):2457–2483. doi: 10.1021/cr00039a007
- Lou S, Fu GC. Palladium/Tris(tert-butyl)phosphine-catalyzed Suzuki cross couplings in the presence of water. Adv Synth Catal. 2010;352(11–12):2081–2084. doi: 10.1002/adsc.201000267
- Wink C, Detert H. Donor-substituted distyrylpyrazines: influence of steric congestion on UV–vis absorption and fluorescence. J Phys Org Chem. 2013;26(2):137–143. doi: 10.1002/poc.2978
- Sonogashira K, Tohda Y, Hagihara N. A convenient synthesis of acetylenes: catalytic substitutions of acetylenic hydrogen with bromoalkenes, iodoarenes and bromopyridines. Tetrahedron Lett. 1975;16(50):4467–4470. doi: 10.1016/S0040-4039(00)91094-3
- Martin S, Manolis DT, Benjamin B, et al. Donor–acceptor (D–A)-Substituted polyyne chromophores: modulation of their optoelectronic properties by varying the length of the acetylene spacer. Chem Eur J. 2013;19(38):12693–12704. doi: 10.1002/chem.201301642
- Cruickshank E, Strachan GJ, Storey JMD, et al. Chalcogen bonding and liquid crystallinity: understanding the anomalous behaviour of the 4′-(alkylthio)[1,1′-biphenyl]-4-carbonitriles (nSCB). J Mol Liq. 2022;346(15):117094. doi: 10.1016/j.molliq.2021.117094
- Hird M. Fluorinated liquid crystals–properties and applications. Chem Soc Rev. 2007;36(12):2070–2095. doi: 10.1039/B610738A
- Shimomura Y, Tokita M, Kawamura A, et al. Fluorinated poly(pentylene 4,4′-bibenzoate)s with low isotropization temperatures and unique phase transition behaviour. Macromolecules. 2023;56(13):5152–5161. doi: 10.1021/acs.macromol.3c00773
- Kato T, Frechet JMJ. A new approach to mesophase stabilization through hydrogen-bonding molecular interactions in binary mixtures. J Am Chem Soc. 1989;111(22):8533–8534. doi: 10.1021/ja00204a044
- Arakawa Y, Sasaki Y, Tsuji H. Supramolecular hydrogen-bonded liquid crystals based on 4-n-alkylthiobenzoic acids and 4,4′-bipyridine: their mesomorphic behavior with comparative study including alkyl and alkoxy counterparts. J Mol Liq. 2019;280:153–159. doi: 10.1016/j.molliq.2019.01.119
- Walker R, Pociecha D, Martinez-Felipe A, et al. Hydrogen bonding and the design of twist-bend nematogens. J Mol Liq. 2020;303:112630. doi: 10.1016/j.molliq.2020.112630
- Shimomura Y, Konishi G. Push-pull bridged distyrylbenzene with highly bright solid-state red-orange aggregation-induced emission. Chem Eur J. 2023;29(42). doi: 10.1002/chem.202301191
- Iwai R, Suzuki S, Sasaki S, et al. Bridged stilbenes: AIEgens designed via a simple strategy to control the non-radiative decay pathway. Angew Chem Int Ed. 2020;59(26):10566–10573. doi: 10.1002/anie.202000943
- Deng Y, Yuan W, Jia Z, et al. H- and J-Aggregation of fluorene-based Chromophores. J Phys Chem B. 2014;118(49):14536–14545. doi: 10.1021/jp510520m
- Liao G, Zhang J, Zheng X, et al. Crystallization-induced emission enhancement of highly electron-deficient dicyanomethylenebridged triarylboranes. Chem Commun. 2021;57(64):7926–7929. doi: 10.1039/d1cc03311e
- Lakowicz JR. Principles of Fluorescence Spectroscopy. 3rd ed. (NY): Springer; 2006.
- Shimizu M, Hiyama T. Organic fluorophores exhibiting highly efficient photoluminescence in the solid state. Chem Asian J. 2010;5:1516–1531. doi: 10.1002/asia.200900727
- Sasaki S, Niko Y, Andrey SK, et al. Design of donor–acceptor geometry for tuning excited-state polarization: fluorescence solvatochromism of push–pull biphenyls with various torsional restrictions on their aryl–aryl bonds. Tetrahedron. 2014;70(41):7551–7559. doi: 10.1016/j.tet.2014.08.002
- Małgorzata S, Irena B, Wiesław W. Influence of an electron-acceptor substituent type on the photophysical properties of unsymmetrically substituted diphenylacetylene. J Photochem Photobiol A: Chem. 2016;2016(326):76–88. doi: 10.1016/j.jphotochem.2016.03.023
- Fujiwara T, Zgierski MZ, Lim EC. Combined experimental and computational study of intramolecular charge transfer in p-N,N-Dimethylamino-p′-cyano-diphenylacetylene. J Phys Chem A. 2011;115(5):586–592. doi: 10.1021/jp109674t
- Hong Y, Lam JWY, Tang BZ. Aggregation-induced emission: phenomenon, mechanism and applications. Chem Commun. 2009;(29):4332–4353. doi: 10.1039/B904665H
- Li Q, Li Z. The strong light‐emission materials in the aggregated state: what happens from a single molecule to the collective group. Adv Sci. 2017;4:1600484. doi: 10.1002/advs.201600484
- Yamada S, Uto E, Yoshida K Development of photoluminescent liquid-crystalline dimers bearing two fluorinated tolane-based luminous mesogens. J Mol Liq. 2022;363:119884. doi: 10.1016/j.molliq.2022.119884
- Ikeda T, Kurihara S, Tazuke S. Excimer formation kinetics in liquid–crystalline alkylcyanobiphenyls. J Phys Chem. 1990;94(17):6550–6555. doi: 10.1021/j100380a008
- Subramanian R, Patterson LK, Levanon H. Luminescence behaviour as a probe for phase transition s and excimer formation in liquid crystals: dodecylcyanobiphenyl. Chem Phys Lett. 1982;93(6):578–581. doi: 10.1016/0009-2614(82)83733-0