Abstract
Nutrient availability in prey items can have important consequences for the growth, reproduction, survival, and recruitment into adulthood of juvenile fish. For young of the year sturgeon, which are highly dependent on macroinvertebrates as prey, knowing the nutritional content across various prey items within their habitats can help managers during habitat restoration. The objective of this study was to test for differences in the macronutrient composition of major invertebrate groups commonly consumed by young of the year sturgeon in the lower Missouri River in the summer, when sturgeon habitat assessments occur. Potential prey vary considerably in size. In addition, there were significant differences in the concentrations of nutrients. The lowest concentration of lipid was found in Odonata (2.36 ± 1.83 mg 100 mg−1; mean ± pooled variance standard error) and the highest was in Diptera (14.49 ± 3.30 mg 100 mg−1). The lowest concentration of protein was found in Ephemeroptera (58.98 ± 1.90 mg 100 mg−1) and the highest concentration was in Trichoptera (70.07 ± 3.26 mg 100 mg−1). Some spatial differences were found in energy derived from protein in Ephemeroptera in the lower Missouri River, but not in energy derived from lipid. Our findings provide useful information that can contribute to adaptive management efforts for sturgeons in the lower Missouri River.
Introduction
Aquatic macroinvertebrates are essential components in aquatic food webs because they transfer nutrients from detritus and algae into forms that are available to higher trophic levels (Wallace and Webster Citation1996; Covich et al. Citation1999). Nutrients in prey, such as macroinvertebrates, can vary 5–30% in lipid and 20–80% in protein, both within and among species (Fagan et al. Citation2002; Raubenheimer et al. Citation2007; Lease and Wolf Citation2010; Wilder et al. Citation2013). The nutrient and energy content of food can have important consequences for the growth and survival of consumers such as predatory young-of-the-year (YOY) fish that rely heavily on lipids and proteins as macronutrients (Millikin Citation1983; Pangle et al. Citation2004; Ruohonen et al. Citation2007).
Both lipids and proteins serve specific physiological roles that are important in YOY fish (Lee et al. Citation2016). Lipid is often metabolized for energy and is the primary energy storage biomolecule in many organisms. Additionally, lipid accumulation in body tissue plays an important role in overwinter survival of YOY fishes when prey are not as readily available (Post and Evans Citation1989). While protein is primarily utilized for biomass production, it can also be catabolized for energy but with a higher cost to the consumer (Jobling Citation1983).
Energetic and elemental analysis alone can be a poor predictor of energy available in prey (Wilder et al. Citation2019). Elemental analyses of prey items can identify coarse measures of quality but are unable to distinguish between compounds that have similar elemental content but different digestibility (Anderson and Pond Citation2000; Anderson et al. Citation2004; Wilder and Eubanks Citation2010). For example, in arthropod prey, significant amounts of nitrogen can be found in both the indigestible exoskeleton and the digestible soft tissues (Klowden Citation2013). Hence, while crude protein (i.e. nitrogen × 6.25; Jones Citation1931) and total elemental content have been common measures of prey nutrient content, more direct measures of macronutrients may be important for assessing prey quality. Prey quality is defined hereafter as digestible lipid and protein content within the prey organism. Moreover, total caloric energy (e.g. bomb calorimetry) can overestimate the amount of energy available to consumers because it combusts materials that consumers cannot digest (Wilder et al. Citation2019). This is particularly relevant for consumers of arthropods, which contain a largely indigestible, chitinous exoskeleton. Total energy density alone, however, may not always be a strong measure of prey quality because of the potential differences in energetic yield between lipids and proteins, and in their primary functions for physiology, especially when predators are feeding on diverse prey.
Although general nutritional and energetic values are available for aquatic macroinvertebrates in the literature (Cumminns and Wuycheck Citation1971), nutritional content in macroinvertebrates can vary spatially since they can be dependent on local streamside vegetation (Sweeney Citation1993). Consequently, differences in the prey quality of foraging habitats can result in spatial differences in predator recruitment (Litzow et al. Citation2002). In the lower Missouri River (LMOR), whether major orders of aquatic macroinvertebrates have similar nutritional content or if spatial differences exist within orders is unknown. Additionally, little information is available regarding wild prey quality, especially regarding digestible macronutrient content (i.e. lipid and protein) in the LMOR. For fisheries species management, knowing prey quality among foraging sites of species of interest may also have some implication for assessing habitat quality and recruitment of managed areas. Currently, it is not clear if any of the documented foraging locations of shovelnose sturgeon (Scaphirhynchus platorynchus), the pallid sturgeon (Scaphirhynchus albus), and their hybrids produce greater chances of survival compared to others (USACE 2017). Pallid sturgeon are federally listed as endangered and are the main focus of habitat restoration efforts in the LMOR (Civiello et al. Citation2018; USACE 2018; Gemeinhardt et al. Citation2019). Due to similarities in early life history, shovelnose sturgeon are also protected and managed as a surrogate species for pallid sturgeon (Gosch et al. Citation2018). Both species feed primarily on Diptera at the YOY life stage, but will also consume Ephemeroptera and Trichoptera (Civiello et al. Citation2018; Gosch et al. Citation2019). Scaphirhynchus sturgeons have low abundance and poor recruitment (Jacobson et al. Citation2016). A major hypothesis for explaining low abundance of Scaphirhynchus sturgeons in the LMOR is that prey quality or availability is limiting juvenile growth and recruitment (Jacobson et al. Citation2016). However, recent studies found the low percentage of empty stomachs and number of food items consumed by Scaphirhynchus sturgeons suggest that food resources are not liming in the LMOR (Gosch et al. Citation2016; Civiello et al. Citation2018). Civiello et al. (Citation2018) mentions if food availability during early life history is not a limiting factor, then focusing on other hypothesized factors that influence YOY Scaphirhynchus sturgeons condition (e.g. fat reserves) could provide valuable insight for future management efforts.
In this study, we tested for differences in digestible lipid and protein content of common potential prey encountered in known foraging habitats of YOY Scaphirhynchus sturgeons in the LMOR and whether the nutrient content varied among reaches of the LMOR. Documenting differences (or lack thereof) of digestible macronutrients of prey in LMOR could complement bioenergetics models and improve ability to draw informative conclusions and/or rationale for pallid sturgeon conservation and management in LMOR.
Materials and methods
Study reaches
The Missouri River stretches 4957 kilometers from the headwaters to the Mississippi River confluence. The LMOR is 1305 km from Gavins Point Dam (Yankton, SD) to the Mississippi River confluence (St. Louis, MO). This segment of the river has been modified via channelization, riprap installment, and levee construction for navigation, bank erosion prevention, and flood prevention, which, in turn has led to loss of shallow water and riparian habitat. We collected macroinvertebrates at four reaches: Copeland, Langdon, Wolf Creek, and Lexington, where YOY Scaphirhynchus sturgeons are known to occur (). Copeland, Langdon, and Wolf Creek are each about 0.24 km wide, and range in length from 24 km long (Copeland and Langdon) to 19 km at Wolf Creek. Lexington is slightly larger, being approximately 0.4 km wide and 32 km long. All reaches have little to no canopy cover along the edges as most areas adjacent to the reaches have been cleared for agriculture.
Collection and identification
Macroinvertebrate were opportunistically removed from trawl debris in July 2017 while accompanying Nebraska Game and Parks Commission (NGPC; http://outdoornebraska.gov/) during routine trawling surveys at Copeland, Langdon, and Wolf Creek using a OT04 with a 4 mm mesh trawl (4.9 m wide with 0.91 × 0.38 m otter doors). Macroinvertebrate sampling was not intended to be comprehensive in this study. Trawl surveys by NGPC were in support for the Habitat Assessment and Monitoring Project (HAMP; Bryan et al. Citation2010) under the Missouri River Recovery Program (http://www.nwo.usace.army.mil/mrrp/). Trawl debris was stored a 500 mL Nalgene™ bottle and preserved in 75% ethanol for later sorting in the lab. When the debris from a single trawl was over 500 mL in volume, a subsample was collected until the sample bottle was full. In addition, a pipe dredge (0.15 m circumference × 0.15 depth) was used to collect benthic samples. Sediment was sifted through a larger sieve (NO. 10, 2.0 mm mesh) and rinsed into a bucket to remove larger sand particles in the field. Floating macroinvertebrates were collected and the remaining sediment was then rinsed through a smaller sieve (NO. 70, 0.210 mm mesh) and stored in 250 mL Nalgene™ bottles with 75% ethanol.
Lexington, was sampled differently than the other three reaches since it is not surveyed by NGPC. However, since Lexington has been surveyed in previous YOY Scaphirhynchus sturgeons gut content studies and is a reach of interest within HAMP, we decided to include macroinvertebrates from this reach in this study (Civiello et al. Citation2018; USACE 2018). At Lexington, macroinvertebrate sampling was done near the shore where conditions permitted for samples to be collected safely using a 0.3 × 0.25 m D-frame dip net (1 mm mesh size) and 0.3 × 0.3 m surber sampler (1 mm mesh size). For depths up to 0.4 m, the surber sampler was placed perpendicular to the current and sediment was disturbed to collect macroinvertebrates in the net (Murphy and Willis Citation1996). For depths 0.4–1.2 m, water flow and substrate conditions did not safely permit sampling protocol with surber net, thus a D-frame dip net was used to scour the benthos against the current instead (Murphy and Willis Citation1996). Pipe dredge samples at Lexington were handled and preserved the same way as the other three reaches.
For all sampling methods, larger macroinvertebrates were segregated and stored individually in 75% ethanol in 1.5 mL tubes in the field. Diptera larvae, regardless of size, were grouped in a single 1.5 mL tube per sampling event due to their smaller size and stored in 75% ethanol in the field as well. All samples (macroinvertebrates, trawl, and benthic samples) were stored in a refrigerator or cooler for 1–5 days until they were transported to Oklahoma State University (Stillwater, OK).
Following field collection, samples were stored in a 6 °C freezer until processing within five months. During processing, macroinvertebrates were quantified and identified to family using a taxonomic key by Merrit et al. (Citation1996) as reference. Macroinvertebrates segregated in the field were stored separate from those extracted from trawl debris or benthic samples in the lab. Additionally, macroinvertebrates segregated in the field remained in their original ethanol preservative for later nutrient analysis.
Nutrient analyses
Only macroinvertebrates segregated in the field were used for nutrient analysis. Macroinvertebrates and all storage ethanol that could contain soluble lipids from the samples were transferred to glass tubes and dried in an oven at 60 °C for 24 h prior to measuring lipid and protein content. After drying, we measured lipid content as the difference in mass before and after soaking and extraction in chloroform, thrice, over three consecutive days and dried a final time (Wilder et al. Citation2013). Protein content was evaluated in triplicate using the Bradford Assay on the lipid-extracted samples (Wilder et al. Citation2013). Macroinvertebrates with a dry mass less than 1.5 mg (post lipid extraction) were pooled with same family taxa until they reached a dry mass ≥1.5 mg prior to protein extraction. Dry masses of nutrients were converted into energy using standard conversion factors (protein = 17 kJ/g, and lipid = 37 kJ/g; Raubenheimer and Rothman Citation2013). Carbohydrates were not measured because they are typically present at very low concentrations in arthropods (Raubenheimer and Rothman Citation2013). Carbohydrates ingested by insects are generally either metabolized or converted into storage lipids; not typically stored within the insect body (Klowden Citation2013). Additionally, we did not analyze nutrient ratios in this study (Raubenheimer Citation1995).
Statistics
Mass variables were not normally distributed, therefore we applied a cube-root transformation to the individual macroinvertebrate wet and dry masses and a square-root transformation to normalize the macronutrient masses after confirming a log transformation was not sufficient. We tested for differences among prey types in wet and dry masses, macronutrient content (lipid and protein), and energy using a one-way ANOVA. When sample mass were sufficient within a prey order, we also tested for differences among order. Only Ephemeroptera had sufficient mass within a prey order to for differences among reaches. We detected differences at the 0.05 statistical significance level, and used Tukey post hoc tests to identify which treatments were different from each other. Statistics were assessed using JMP software package (version 12, SAS Institute, Cary, NC, USA).
Results
We identified and analyzed 1,410 macroinvertebrates (). Diptera was the most frequently encountered order (n = 995), followed by Ephemeroptera (n = 308), Trichoptera (n = 67), and Odonata (n = 40). Within the order Diptera, Chironomidae larvae were the most common (n = 957), followed by Ceratopogonidae (n = 38). Ephemeroptera was the most diverse order with nine families collected. Within Ephemeroptera, Caenidae were the most frequently sampled family (n = 142). When separated by reach, Wolf Creek had the highest number of total prey items for all orders combined (n = 691), followed by Lexington (n = 249), Langdon (n = 239), and Copeland (n = 233). Due to low sample size within reaches and family, taxa were grouped together by order for statistical analysis: Odonata, Ephemeroptera, Diptera, and Trichoptera. Few Plecopera were collected and were therefore excluded from further analysis. Only Ephemeroptera had sufficient sample mass per reach to test for spatial differences among reaches.
Table 1. List of macroinvertebrates collected in four reaches of the lower Missouri River.
Macroinvertebrate taxa varied widely in size from Diptera that had a wet mass less than 1 mg to Odonata that weighed approximately 48 mg (). Given the large size variation within and among prey taxa, we analyzed nutrient concentrations in prey rather than by nutrient content mass. The concentration of lipid in Odonata was significantly lower than that of the three other taxa: Diptera, Ephemeroptera, and Trichoptera (p = <0.0001). For protein, the lowest concentration was found in Ephemeroptera and the highest in Trichoptera. Concentrations of protein in Diptera and Odonata were intermediate and not significantly different from Ephemeroptera or Trichoptera. Despite their larger size, Odonata had significantly lower total energy density compared to the three other taxa (p = 0.0003). The lower total energy concentration in Odonata appeared largely driven by the low amounts of lipid-derived energy in this order relative to the other three taxa ().
Figure 2. Partitioning of energy available by macronutrient (protein and lipid) per mass (g) of macroinvertebrate taxon (mean ± pooled variance standard error). Different letters indicate significant variation between taxa. DIP: Diptera; EPH: Ephemeroptera; ODO: Odonata; TRI: Trichoptera.
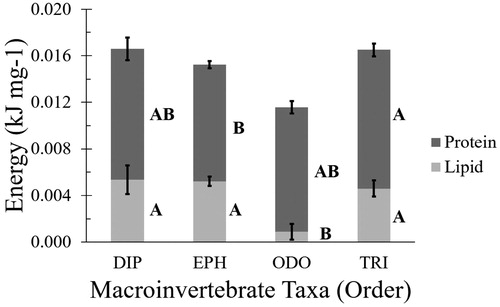
Table 2. Summary nutrient contents (mean ± pooled variance standard error) of whole macroinvertebrates collected from the lower Missouri River.
When comparing Ephemeroptera among sample reaches, total energy was greater at Copeland than Langdon, while Lexington and Wolf Creek were intermediate (F3,68=2.96, p = 0.04; ). Lipid-derived energy per unit mass did not differ by reach (F3,70= 0.65, p = 0.58). However, Ephemeroptera from Lexington had significantly lower protein-derived energy than those from Copeland and Wolf Creek (F3,70=3.08, p = 0.03).
Figure 3. Partitioning of energy available by macronutrient (protein and lipid) per mass (g) of Ephemeroptera among reaches (mean ± pooled variance standard error) of the LMOR. Different letters indicate significant differences in protein energy between reaches (lipid energy did not significantly vary among reaches).
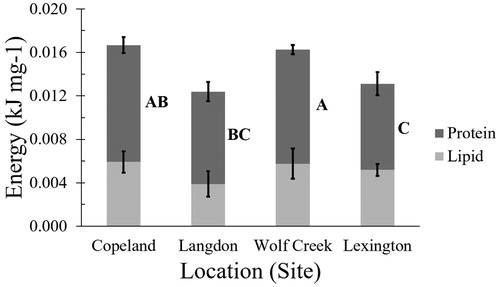
Discussion
Our results show that there are differences in the nutritional concentrations of different macroinvertebrate taxa. For example, Trichoptera had relatively high lipid and high protein concentration, whereas Odonata had high protein concentration and low lipid concentration. Some spatial differences were found in energy derived from protein in Ephemeroptera in the LMOR, but not in energy derived from lipid. Further work is needed to better understand spatial variation in prey nutrient concentration and the factors responsible. Given that different prey taxa appear to vary in nutrient concentration, changes in the community composition of macroinvertebrates in streams and changes in nutrient concentration of individual taxa among locations in a stream could have consequences for the growth and survival of fish.
In addition to variations in the concentrations of macronutrients, potential prey taxa also differ in body mass. Thus, a fish predator could obtain similar nutritional content from a single larger ephemeropteran prey than by consuming multiple smaller dipteran prey. For example, an individual fish that consumed a single Ephemeropera would need to ingest approximately 62 dipterans to obtain similar lipid mass (g); approximately 57 dipterans to obtain a similar mass of protein, and approximately 58 dipterans to obtain similar total energy intake. A better understanding of the nutritional ecology of YOY Scaphirhychus sturgeon will require integrating information on the nutrient concentration of prey, prey size, prey abundance in nature, and prey or habitat preferences of the fish.
Combining traditional elemental and macronutrient approaches to understand predator physiology can increase the ability to predict the flow of nutrients though ecosystems (Wilder et al. Citation2019). Bioenergetics models, which commonly use total energy density of prey, are often applied in developing pragmatic solutions in fisheries species management as they can be linked to abiotic and biotic properties in physical habitats among species of interest and their prey (Hansen et al. Citation1993; Deslauriers et al. Citation2016). That is to say, bioenergetics models can be informative when evaluating habitat quality for YOY fishes (Nislow et al. Citation2000; Jenkins and Keeley Citation2010; Urabe et al. Citation2010) or in identifying subtle physiological differences in sympatric congeners such as shovelnose sturgeon and pallid sturgeon (Porreca et al. Citation2017). Although elemental and calorimetric analysis remain as common techniques for measuring prey quality (Cumminns and Wuycheck Citation1971; Fagan et al. Citation2002; Deslauriers et al. Citation2016), applying macronutrient analysis to quantify the nutrient concentration may also provide a more accurate measure of nutrient availability. Many predators are unable to digest significant amounts of chitin, which is particularly relevant for consumers of arthropods, which contain a largely indigestible, chitinous exoskeleton (Barnes et al. Citation2019). Therefore, a measurement of metabolizable nutrients available to predators, such as from macronutrient analysis, may be more suitable. For example, the protein analyses used in the present study measures protein concentration in the soft tissues of prey whereas elemental analysis would measure nitrogen in both the metabolizable soft tissues and the indigestible exoskeleton.
In addition to improving the understanding of digestible macronutrient concentration of common prey items, there are several other implications for the conservation and management of Scaphirhynchus sturgeons in the LMOR from our results. For instance, optimal foraging theory suggests that diet selection is strongly dictated by the net energy intake per unit time, which is a function of prey density within an area and their profitability (Charnov Citation1976). The abundance of Diptera taxa in our sampling effort reinforces the assertion that dipterans are likely the most prevalent prey available to YOY Scaphirhynchus sturgeons compared to other groups that appear to be less common in the field and consumed less frequently (Civiello et al. Citation2018). In the LMOR floodplains, which are also used for foraging by YOY sturgeon, Diptera was also the predominant prey item available (Gosch et al. Citation2014). Thus, knowledge of how macronutrient concentration of Diptera larvae compares to other less frequently consumed prey of YOY Scaphirhynchus sturgeons allows us to make interpretations of continuing diet and habitat studies relating to YOY Scaphirhynchus sturgeons in LMOR. For example, although Diptera are consumed at higher numbers and are the dominant prey of YOY Scaphirhynchus sturgeons (Civiello et al. Citation2018), when Ephemeroptera are consumed, the consumption of Diptera decreases and the overall number of total prey items in the gut is reduced (González and Long Citation2019). Since Ephemeroptera are much larger than dipterans, they are potentially making up energy allocation in size and mass rather than by quantity. However, gross preliminary data suggesting whether consuming a few large prey is commensurate with consuming many small prey is lacking and remains to be verified. For example, in 2016, juvenile sturgeon in the lower Missouri River consumed Diptera larvae at a 5:1 ratio compared to Ephemeroptera (González and Long Citation2019). This is in contrast to a 58:1 ratio that would result in equivalent energy intake based on our study. Although there should be an increase energetic expenditure for digesting protein and chitin (McCue Citation2006; Secor Citation2009; Barnes et al. Citation2019), we don’t know the digestive passage rate of YOY sturgeon or the physiological expense when digesting Ephemeroptera or Diptera. However, many previous studies measured the consumption of chironomids and other macroinvertebrates by presence of their head capsules in the digestive tract (Gosch et al. Citation2014; Civiello et al. Citation2018).
In addition to effects of energetic limitation on physiology, consumers may also encounter behavioral consequences while pursuing macronutrient demands. For example, Yilmaz et al. (Citation2005) found that common carp, Cyprinus carpio, fed a higher protein diet increased foraging behavior and attention to the servicer (observer during the experiment). This shift in behavior has also been found in other predator–prey systems. For example, spiders consuming higher protein prey foraged for longer duration of time and lowered their attack latency (Koemel et al. Citation2019). For juvenile fish, increased movement could increase vulnerability to predators, potentially decreasing survival probability. Under our model of differences in macronutrients, this could suggest differences in behavior in relation to prey where movement is increased when consuming many Odonata because of short-term energy use of protein-based nutrients leading to lower reserves in body weight. Currently, however, YOY Scaphirhynchus sturgeons appear to consume Odonata rarely, although other fish species make use of this prey type more regularly (e.g. Flathead Catfish Pylodictis olivaris, Hogberg and Pegg Citation2016).
Factors that affect the growth and recruitment of YOY pallid sturgeon remain poorly understood. Our data suggest that the two most common potential prey items (Diptera and Ephemeroptera) in the LMOR are relatively similar in nutrient content. Yet, shifts in the availability or consumption of other prey that differ in nutrient content could affect nutrient ingestion by sturgeon or other fish that feed on benthic macroinvertebrates. Recent and ongoing studies have focused on examining abiotic factors that influence habitat use, prey consumption, and condition of YOY Scaphirhynchus sturgeons in the LMOR (Civiello et al. Citation2018; Deslauriers et al. Citation2018; Gemeinhardt et al. Citation2019). However, understanding how other biotic factors may also be influencing recruitment and survival at age-0 still needs to be further understood. Our findings may help elucidate ongoing studies regarding spatial differences in body condition, habitat, and growth rates of YOY Scaphirhynchus sturgeons in the LMOR (Civiello et al. Citation2018).
Acknowledgments
We thank Jerrod Hall and the Nebraska Game and Parks Commission staff for field assistance and Taylor Brooks for laboratory assistance. We also thank Andy Dzialowski and Wyatt Hoback for providing valuable feedback. Any use of trade, firm, or product names is for descriptive purposes only and does not imply endorsement by the U.S. Government.
Disclosure statement
The authors declare they have no conflict of interest.
Data availability statement
The data that support the findings of this study are available from the corresponding author upon reasonable request.
Additional information
Funding
Notes on contributors
Alin González
Alin González is a Senior Research Specialist at the Oklahoma Cooperative Fish and Wildlife Research Unit within the Natural Resources and Ecology Management Department at Oklahoma State University.
Cody L. Barnes
Cody L. Barnes Postdoctoral Fellow II at Utah State University within the Biology Department.
Shawn M. Wilder
Shawn M. Wilder is an Associate Professor in the Department of Integrative Biology at Oklahoma State University.
James M. Long
James M. Long is a Research Fish Biologist for U.S. Geological Survey, Oklahoma Cooperative Fish and Wildlife and an Adjunct Associate Professor within the Natural Resource Ecology and Management Department at Oklahoma State University.
References
- Anderson TR, Boersma M, Raubenheimer D. 2004. Stoichiometry: linking elements to biochemicals. Ecology. 85(5):1193–1202.
- Anderson TR, Pond DW. 2000. Stoichiometric theory extended to micronutrients: comparison of the roles of essential fatty acids, carbon, and nitrogen in the nutrition of marine copepods. Limnol Oceanogr. 45(5):1162–1167.
- Barnes CL, Hawlena D, McCue MD, Wilder SM. 2019. Consequences of prey exoskeleton content for predator feeding and digestion: black widow predation on larval versus adult mealworm beetles. Oecologia. 190(1):1–9.
- Bryan JL, Wildhaber ML, Gladish DW. 2010. Power to detect trends in Missouri River fish populations within the Habitat Assessment Monitoring Program: U.S. Geological Survey Open-File Report 2010–1011.
- Charnov EL. 1976. Optimal foraging, the marginal value theorem. Theor Popul Biol. 9(2):129–136.
- Civiello AP, Gosch NJC, Gemeinhardt TR, Miller ML, Bonneau JL, Chojnacki KA, DeLonay AJ, Long JM. 2018. Diet and condition of age-0 Schaphirhynchus sturgeon: implications for shallow-water habitat restoration. North Am J Fish Manage. 38(6):1324–1338.
- Covich AP, Palmer MA, Crowl TA. 1999. The role of benthic invertebrate species in freshwater ecosystems: zoobenthic species influence energy flows and nutrient cycling. BioScience. 49(2):119–127.
- Cumminns KW, Wuycheck JC. 1971. Caloric equivalents for investigations in ecological energetics: with 2 figures and 3 tables in the text. Int Ver Theor Angew Limnol. Mitt. 18:1–158.
- Deslauriers D, Heironimus LB, Chipps SR. 2016. Test of a foraging-bioenergetics model to evaluate growth dynamics of endangered pallid sturgeon (Scaphirhynchus albus). Ecol Model. 336:1–12.
- Deslauriers D, Heironimus LB, Rapp T, Graeb BDS, Klumb RA, Chipps SR. 2018. Growth potential and habitat requirements of endangered age‐0 pallid sturgeon (Scaphirhynchus albus) in the Missouri River, USA, determined using a individual‐based model framework. Ecol Freshw Fish. 27(1):198–208.
- Fagan WF, Siemann E, Mitter C, Denno RF, Huberty AF, Woods HA, Elser JJ. 2002. Nitrogen in insects: implications for trophic complexity and species diversification. Am Nat. 160(6):784–802.
- Gemeinhardt TR, Gosch NJC, Civiello AP, Chrisman NJ, Shaughnessy HH, Brown TL, Long JM, Bonneau JL. 2019. The influence of depth and velocity on age‐0 Scaphirhynchus sturgeon prey consumption: implications for aquatic habitat restoration. River Res Applic. 35(3):205–215.
- González A, Long JM. 2019. Assessment of prey consumption and body condition of Missouri River age-0 Scaphirhynchus sturgeon. Quarterly Report 12 to U.S. Army Corps of Engineers. Cooperative Agreement W912HZ-16-0026, Kansas City, Missouri.
- Gosch NJ, Gemeinhardt TR, Civiello AP, Harrison AB, Bonneau JL. 2019. Dietary assessment of age-0 pallid sturgeon and shovelnose sturgeon: implications for surrogacy. Endang Species Res. 40:321–327.
- Gosch NJC, Civiello AP, Gemeinhardt TR, Bonneau JL, Long JM. 2018. Are shovelnose sturgeon a valid diet surrogate for endangered pallid sturgeon during the first year of life? J Appl Ichthyol. 34(1):39–41.
- Gosch NJC, Miller ML, Dziawloski AR, Morris DM, Gemeinhardt TR, Bonneau JL. 2014. Assessment of Missouri River floodplain invertebrates during historic inundation: implications for river restoration. Knowl Manag Aquat Ec. 412(5):1–15.
- Gosch NJC, Miller ML, Gemeinhardt TR, Starks TA, Civiello AP, Long JM, Bonneau JL. 2016. Age‐0 shovelnose sturgeon prey consumption in the lower Missouri River. River Res Applic. 32(8):1819–1823.
- Hansen MJ, Boisclair D, Brandt SB, Hewett SW, Kitchell JF, Lucas MC, Ney JJ. 1993. Applications of bioenergetics models to fish ecology and management: where do we go from here? T Am Fish Soc. 122(5):1019–1030.
- Hogberg NP, Pegg MA. 2016. Flathead Catfish Pylodictis olivaris diet composition during extreme flow events in a large river. J Freshwater Ecol. 31(3):431–441.
- Jacobson RB. Annis ML, Colvin ME, James DA, Welker TL, Parsley MJ. 2016. Missouri River Scaphirhynchus albus (Pallid Sturgeon) effects analysis—integrative report 2016: U.S. Geological Survey Scientific Investigations Report 2016–5064.
- Jenkins AR, Keeley ER. 2010. Bioenergetic assessment of habitat quality for stream-dwelling cutthroat trout (Oncorhynchus clarkii bouvieri) with implications for climate change and nutrient supplementation. Can J Fish Aquat Sci. 67(2):371–385.
- JMP, version 12. 1989–2019. Cary (NC): SAS Institute Inc.
- Jobling M. 1983. Towards an explanation of specific dynamic action (SDA). J Fish Biol. 23(5):549–555.
- Jones DB. 1931. Factors for converting percentages of nitrogen in foods and feeds into percentages of proteins. Circular 183. U. S. Department of Agriculture (slightly revised 1941).
- Klowden MJ. 2013. Physiological systems in insects. San Diego, CA: Academic Press.
- Koemel NA, Barnes CL, Wilder SM. 2019. Metabolic and behavioral responses of predators to prey nutrient content. J Insect Physiol. 116:25–31.
- Lease HM, Wolf BO. 2010. Exoskeletal chitin scales isometrically with body size in terrestrial insects. J Morphol. 271(6):759–768.
- Lee S, Haller LY, Fangue NA, Fadel JG, Hung S. 2016. Effects of feeding rate on growth performance and nutrient partitioning of young‐of‐the‐year white sturgeon (Acipenser transmontanus). Aquacult Nutr. 22(2):400–409.
- Litzow MA, Piatt JF, Prichard AK, Roby DD. 2002. Response of pigeon guillemots to variable abundance of high-lipid and low-lipid prey. Oecologia. 132(2):286–295.
- McCue MD. 2006. Specific dynamic action: a century of investigation. Comp Biochem Physiol Part A Mol Integr Physiol. 144(4):381–394.
- Merrit RW. Cummins KW, Berg MB 1996. An introduction to the aquatic insects of North America. Dubuque (IA): Kendall. Hunt.
- Millikin MR. 1983. Interactive effects of dietary protein and lipid on growth and protein utilization of age‐0 striped bass. Trans Am Fish Soc. 112(2A):185–193.
- Murphy BR, Willis DW, editors. 1996. Fisheries techniques. 2nd ed. Bethesda (MD): American Fisheries Society; p. 335–350.
- Nislow KH, Folt CL, Parrish DL. 2000. Spatially explicit bioenergetic analysis of habitat quality for age-0 Atlantic salmon. Trans Am Fish Soc. 129(5):1067–1081.
- Pangle KL, Sutton TM, Kinnunen RE, Hoff MH. 2004. Overwinter survival of juvenile lake herring in relation to body size, physiological condition, energy stores, and food ration. T Am Fish Soc. 133(5):1235–1246.
- Porreca AP, Hintz W, Coulte DP, Garvey JE. 2017. Subtle physiological and morphological differences explain ecological success of sympatric congeners. Ecosphere. 8(10):e01988.
- Post JR, Evans DO. 1989. Size-dependent overwinter mortality of young-of-the-year perch (Perca flavescens): laboratory, in situ enclosure, and field experiments. Can J Fish Aquat Sci. 46(11):1958–1968.
- Poulton BC, Wildhaber ML, Charbonneau CS, Fairchild JF, Mueller BG, Schmitt CJ. 2003. A longitudinal assessment of the aquatic macroinvertebrate community in the channelized lower Missouri River. Environ Monit and Assess. 85(1):23–53.
- Raubenheimer D. 1995. Problems with ratio analysis in nutritional studies. Func Ecol. 9(1):21–29.
- Raubenheimer D, Mayntz D, Simpson SJ, Tøft S. 2007. Nutrient-specific compensation following diapause in a predator: implications for intraguild predation. Ecology. 88(10):2598–2608.
- Raubenheimer D, Rothman JM. 2013. Nutritional ecology of entomophagy in humans and other primates. Annu Rev Entomol. 58:141–160.
- Ruohonen K, Simpson SJ, Raubenheimer D. 2007. A new approach to diet optimization: a re-analysis using European whitefish (Coregonus lavaretus). Aquaculture. 267(1-4):147–156.
- Secor SM. 2009. Specific dynamic action: a review of the postprandial metabolic response. J Comp Physiol B, Biochem Syst Environ Physiol. 179(1):1–56.
- Sweeney BW. 1993. Effects of streamside vegetation on macroinvertebrate communities of White Clay Creek in eastern North America. Proc Acad Natl Sci Philadelphia. 144:291–340.
- Urabe H, Nakajima M, Torao M, Aoyama T. 2010. Evaluation of habitat quality for stream salmonids based on a bioenergetics model. T Am Fish Soc. 139(6):1665–1676.
- USACE [United States Army Corps of Engineers]. 2017. Biological assessment [BA] for the operation of the Missouri River Mainstem reservoir system, the operation and maintenance of the bank stabilization and navigation project, the operation of Kansas River reservoir system, and the implementation of the Missouri River recovery management plan; [accessed 2018 Jul]. https://usace.contentdm.oclc.org/digital/collection/p16021coll3/id/677.
- USACE [United States Fish and Wildlife Service]. 2018. Biological opinion: operations of the Missouri River mainstem reservoir system, the operation and maintenance of the bank stabilization and navigation project, operation of the Kansas River reservoir system, and the implementation of the Missouri River Recovery Management Plan, Denver, CO; [accessed 2018 Jul]. https://usace.contentdm.oclc.org/utils/getfile/collection/p16021coll3/id/670.
- Wallace JB, Webster JR. 1996. The role of macroinvertebrates in stream ecosystem function. Annu Rev Entomol. 41:115–139.
- Wilder SM, Barnes CL, Hawlena D. 2019. Predicting predator nutrient intake from prey body contents. Front Ecol E. 7(42):1–7.
- Wilder SM, Eubanks MD. 2010. Might nitrogen limitation promote omnivory among carnivorous arthropods? Comment. Ecology. 91(10):3114–3117.
- Wilder SM, Norris M, Lee RW, Raubenheimer D, Simpson SJ. 2013. Arthropod food webs become increasingly lipid-limited at higher trophic levels. Ecol Lett. 16(7):895–902.
- Yilmaz E, Şahin A, Duru M, Akyurt İ. 2005. The effect of varying dietary energy on growth and feeding behaviour of common carp, Cyprinus carpio, under experimental conditions. Appl Anim Behav Sci. 92(1–2):85–92.