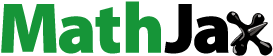
Abstract
Human activities have substantially disrupted phosphorus (P) cycles in the ecosystem, affecting producers and consumers along the food chain. To assess the ecological effects of imbalanced P on producers as well as consumer reactions to changes in food quality, the effects of P concentrations (HP, MP, LP) on Scenedesmus obliquus, were investigated. To investigate the indirect effects of P levels on consumer feeding behavior and life history strategies, the algae were fed to Rotaria rotatoria. The results showed that P concentrations increased population density, environment capacity, cell size, specific growth rate, chlorophyll a, and chlorophyll b substantially. As a result of the P intracellular concentration of algae produced in HP and MP media, the algae were classified as two categories of food quality (P-rich, P-poor). Rotifers' grazing and filtration rates were significantly increased when they were fed P-poor algae. In reaction to a lack of P in their diet, rotifers extended their juvenile and reproductive periods, resulting in a longer life span, generation time, and life expectancy at rotifer hatching. Despite living longer than P-rich groups, rotifers fed P-poor algae produced much fewer offspring and had a significantly lower net reproductive rate, which was linked to the rotifers' longer generation period. According to the findings, phosphorus has a major impact on algal food quality, and herbivorous consumers are subjected to significant food quality variation of algae, which they respond to by modifying their life history strategies and feeding behavior.
1. Introduction
Phosphorus (P), a fundamental element of all life, is essential to aquatic ecosystems (Aversa et al. Citation2016; Roy Citation2017). In organisms, P is not only the backbone component of nucleic acids but also the transmission center of chemical energy through ATP (Brembu et al. Citation2017). Particularly, P is an essential component of ribosomal RNA, which drives the synthesis of protein, and further influences the growth and reproduction of organisms (Zhou Citation2019). Studies also have demonstrated that the expression of many genes may be regulated indirectly by phosphate content (excess or limited) at the post-transcriptional or post-translational levels (Anne-Marie et al. Citation2020; Guo et al. Citation2016). In ecosystems, P, both inorganic and organic, is requisite nutrients for nearly all photoautotrophs, such as microbes, algae and plants (Elser et al. Citation2007; Grossman and Aksoy Citation2015). Therefore, the phosphorus in waters plays a vital role in maintaining the growth and metabolism of organisms and stability of ecosystems.
Phytoplankton biomass and production can be controlled by phosphorus availability, which is one of the classical paradigms in modern limnology (Lewis and Wurtsbaugh Citation2008). P input excessively in water results in eutrophication, exhibiting by harmful algal blooms, changes in phytoplankton and zooplankton community structure, as well as food chain chaos (Grossman and Aksoy Citation2015; Schindler et al. Citation2016; Schmoker et al. Citation2016; Xu et al. Citation2010). On the contrary, many freshwater ecosystems are oligotrophic caused by nutrient deficiency (Elser et al. Citation2009; Stockner et al. Citation2000). A low level of accessible P often impacts the community composition and limits the growth, biomass (Mousing et al. Citation2018; Reed et al. Citation2016; Teufel et al. Citation2017), the amount of highly unsaturated fatty acids (Anne-Marie et al. Citation2020; Challagulla et al. Citation2015) and metabolic activities (Karl Citation2014) of phytoplankton, resulting in different food quality for the subsequent zooplankton consumers. As a consequence of P limitation, photoautotrophs evolve a series of adaptive strategies to cope with phosphorus deficiency better, and nutrient-sparing reactions of algae were motivated in the environment of insufficient phosphorus (Grossman and Aksoy Citation2015). For example, the transcription factor inorganic phosphate starvation response1 (PSR1) and lipid remodeling regulator 1 (LRL1) were the global regulators of phosphorus deficiency responses in green algae Chlamydomonas reinhardtii (Bajhaiya et al. Citation2016; Hidayati et al. Citation2019). Studies showed that truncated hemoglobins (trHbs) may be implicated in functions other than oxygen delivery, for example, the depletion of some trHb genes (THB1 and THB2) may lead to a reduction in cell size and chlorophyll levels under conditions of P deprivation (Filina et al. Citation2019). However, little is known if the nutrient level in algae is affected positively by the phosphorus concentration in the culture medium.
Zooplankton dwells in rapidly changing environment, with the instability of abundance and quality of food resources. As unselective filter feeders, zooplankton is highly susceptible to algal food quality (Schälicke et al. Citation2019), so the food in nature for freshwater zooplankton (cladoceras, copepods and rotifers) are often restricted seriously (Kirk Citation1997). Previous studies have indicated that the dietary energy from algae is highly retained in the aquatic food webs (Guo et al. Citation2016). Insufficiency of nutrients such as nitrogen and phosphorus in the water body can cause the growth of algae to stagnate or decrease in reproduction, resulting in high mortality of zooplankton and a decline in the population of zooplankton. Thus, nutrient stoichiometry in the primary producers can affect subsequent consumers’ growth (Burian et al. Citation2020; Darchambeau Citation2005; Elser et al. Citation2001; Malzahn and Boersma Citation2012), egg size and number (Franco-Santos et al. Citation2018; Nobili Citation2013) and feeding behavior (Meunier et al. Citation2016; Vad et al. Citation2020). Therefore, the nutrient quality of algae is critical for the subsequent zooplankton consumers. Studying on the life history characteristics of rotifer is of great significance to explain the competition and utilization of food resources by grazing zooplankton.
Rotaria rotatoria is an asexual freshwater bdelloid rotifer, although which has been designated as indicator of heavy pollution, its response strategies to nutrient levels are still need to be further studied, and little evaluation has been explored to understand the relative importance of direct and indirect effects of nutrient element on producers and consumers. In this study, the main aims are: (1) to assess the relative effects of P limitation and rich on producers; (2) to explore the life history strategy of grazing consumers under different food quality; (3) to make clear how consumers regulate feeding behavior in response to changes in food quality.
2. Materials and methods
2.1. Experimental organisms
In this study, the algae S. obliquus was purchased from the Freshwater Algae Culture Collection at the Institute of Hydrobiology (FACHB-collection), then semi-continuously cultured in illumination incubator (28 ± 1 °C, 3000Lx, Light: Dark = 12h:12 h) with the BG-11 medium (Andersen Citation2005). The R. rotatoria was sampled from a pond near Jing village (31°73′67′′ N, 118°33′72′′ E) in Hexian County, Anhui Province, China. After collection, rotifer individuals were clonally cultured at 28 ± 1 °C, with the green algae S. obliquus as food at the density of 1.0 × 106 cells·mL−1. The rotifer culture was maintained using an EPA medium, which contained 96 mg NaHCO3, 60 mg CaSO4, 60 mg MgSO4·7H2O and 4 mg KCl per liter of distilled water (pH 7.4–7.8) (Peltier and Weber Citation1985).
2.2. Algal growth experiment
In this experiment, semicontinuous cultures of S. obliquus were established in BG-11 medium with high P (2 mg·L−1, HP), medium P (0.2 mg·L−1, MP) and low P (0.02 mg·L−1, LP) according to the P concentration classification in the environmental quality standards for surface water, and the medium without P was set as the control. The algae in the exponential growth phase were inoculated in four mediums to a final density of 1.0 × 105 cells·mL−1. During the experiments, density of algae in each group was determined for every day by counting using a hemocytometer. Seven days later, the experiment was terminated. According to the daily density of algae specific growth rate (μ) was calculated with EquationEquation (1)(1)
(1) .
(1)
(1)
where μi–j is the specific growth rate from time i to j; Xj is the density at time j; and Xi is the density at the time i.
At the end of the experiments, cell sizes of algae were determined by picture which photographed in a microscope (Leica DM2000), and cell volumes (V) were calculated with EquationEquation (2)(2)
(2) . Besides, algae were collected by centrifugation (12000 rpm, 5 min) to remove the excrescent medium. Centrifugal algae with 5 ml 80% acetone were dipped in a water bath (55 °C) for 60 min to extract chlorophyll. The absorptivity at 663 nm and 645 nm were determined by spectrophotometer, and the chlorophyll content was calculated with EquationEquations (3)
(3)
(3) and Equation(4)
(4)
(4) .
(2)
(2)
(3)
(3)
(4)
(4)
where a is the half of algae cell width, b is the half of algae cell length, A663 is the value of absorptivity at 663 nm, and A645 is the value of absorptivity at 645 nm.
Moreover, centrifuged algae were dried at bake oven (30 °C, 24 h) for C, N and P element detection by the Science Spectrum R&D Center (Qingdao, China). C and N were detected by an elemental analyzer (Elementar vario MICRO cubeMT) and P was detected by ICP-OES (iCAP7400).
2.3. Feeding experiments
The algae cultured in different (Control, HP, MP and LP) mediums were collected by centrifugation at 4000 rpm for 5 min on the seventh day. After collection, S. obliquus was washed three times with bicarbonate solution (15 mg·L−1) to remove trace of dissolved P in the algal medium. In the feeding experiment, ten neonates (less than 6 h old) were transferred to 8-mL glass beakers containing 5 mL EPA medium with the washed algae at the density of 1.0 × 106 cells·mL−1. Subsequently, a plastic board was used to cover on beaker for preventing the evaporation of water from EPA medium, and then the container was put in an incubator with natural illumination at 28 ± 1 °C. Each treatment was repeated three times. According to the previous results of life table experiment, the time at the first offspring of R. rotatoria appeared was about 60–80h. Therefore, all experiments were sustained for 60 hours for avoiding the disturbance of neonatal offsprings to the filtration and grazing rates, during the process no extra algae food was supplied to rotifers. In addition, for preventing the algal deposition in the experiment, we suspended the algae culture every 8 h. After that, the density of unconsumed algae cells was measured using a hemocytometer. The EPA medium, containing algae at 1.0 × 106 cells·mL−1 without rotifers, was set as reference.
The grazing rate refers to the amount of food that an individual ingests in a specific period of time, and the filtration rate is expressed as the volume of swept the filter-feeder to collect food. In other words, the filtration rate (F) is the average volume of liquid filtered by each rotifer per unit time (ml·ind.−1·h−1), and the grazing rate (G) is the average number of algae cells taken by each rotifer per unit time (cells·ind.−1·h−1), which can be calculated based on the EquationEquations (5)(5)
(5) and Equation(6)
(6)
(6) (Frost Citation1972):
(5)
(5)
(6)
(6)
where V is the medium volume (ml); t is the experimental time (h); n is the number of rotifers in each replicate; C0 and Ctf are the initial and final densities of a given algae; and Ct is the final algal density of the reference group in which no rotifer is present and the algae S. obliquus is cultured synchronously with the test groups.
2.4. Life history traits experiments
The life history experiment of R. rotatoria was also performed with four treated algae (Control, LP, MP and HP). For each treatment, 48 neonates, born within 6 h, were transferred into two 24-well tissue culture plates by introducing one neonate into each well which contained 0.5 mL EPA medium with 1.0 × 106 cells·mL−1 of S. obliquus. The eight plates (two plates for each group) were put in a nature illumination incubator at 28 °C. During the initial several days, all rotifers were inspected every 6 h until all neonates produced offspring. Here, we recorded the time of the first offspring produced. Afterwards, the number of original alive individuals and neonates were recorded every 12 h and the neonates were removed. Besides, the rotifer medium was renewed every 24 h, and simultaneously the fresh food was supplied before the whole cohort died.
Based on the data collected, the juvenile period (P1), reproductive period (P2), post-reproductive period (P3), the number of offspring (NO) and mean lifespan (ML) of rotifers fed with different treated algae were calculated. The age-specific survival (lx) and age-specific fecundity (mx) were constructed for each experiment group using conventional life-table techniques (Poole, 1974). Life expectancy at hatching (e0), generation time (T) and net reproductive rate (R0) were calculated with EquationEquations (7)–(9). The intrinsic rate of increase (rm) was first approximated using: r-rough = lnR0/T. For final calculation, we solved with EquationEquation (10)(10)
(10) .
(7)
(7)
(8)
(8)
(9)
(9)
(10)
(10)
2.5. Data analysis
All data were analyzed by using SPSS 25.0 and expressed as Mean ± SE (standard error). The one-sample Kolmogorov-Smirnov procedure and Levene’s test were used to test the data for normality and homogeneity of variances, respectively. Kaplan-Meier analyses were performed to test for the differences in the age-specific survivorships of rotifers fed with different quality algae. One-way analysis of variance (ANOVA) was conducted to identify the significant effect of phosphorus on each of the population variables of algae. The life-history traits of rotifers also performed by one-way ANOVA. Multiple comparisons were conducted using Student-Newman-Keulsa (SNK) to identify which groups were significantly different among treatments. The statistically significant level (p) was set as less than 0.05.
3. Results
3.1. Algal growth
In different experimental groups, the density of S. obliquus increased daily, and each group achieved maximum population density at day 7 (). The population density of treatment groups was higher and increased faster than that in control group. The relevant parameters were analyzed among different groups and the results showed in . The P concentration in the medium significantly increased the specific growth rate (μ) (p < 0.05). The cell volumes of algae were significantly bigger in the MP and HP treatments than the control group (p < 0.05). Moreover, the chlorophyll a (Ca) in groups of MP and HP were significantly much higher compared with the control and LP (p < 0.05). However, no significant difference was found in chlorophyll b (Cb) among all groups (p > 0.05).
Table 1. The growth parameters of S. obliquus in different P concentration.
In this experiment, the result showed that the C, N and P content in algae were 343.4–388.7 mg·g−1, 82.2–97.2 mg·g−1 and 0.6–11.5 mg·g−1, respectively. The nitrogen content in different experimental groups was nonsignificant (p > 0.05). However, the content of P in groups of MP and HP increased significantly compared with the control and LP, and the contents of C in three treatments were higher significantly than the control. We also analyzed the ratio of different elements (), and the results showed that the values of C:P ratio and N:P ratio in control and LP were significantly higher than MP and HP (p < 0.05), corresponding to two different food quality (P-poor at LP and control, P-rich at MP and HP). Additionally, significant differences were not found in C:N ratio (p > 0.05).
3.2. The feeding experiment of R. rotatoria
Alterations in feeding behaviors of zooplankton can be an indicator of food quality. The results of one-way ANOVA with SNK-q method indicated that food quality had a significant effect on feeding behaviors of rotifers, both the grazing and the filtration rates (p < 0.05, ). Compared with P-rich food (MP and HP), rotifers had higher grazing and filtration rates by feeding the P-poor algae (Control and LP). In other words, the P concentration in environment indirectly affected the feeding behavior of rotifers by adjusting the essential element components and ratio in algae (food quality).
3.3. Life history strategy
The results of the one-way ANOVA investigating the life history strategy on the development duration, offspring production and population growth are presented in . It was indicated that the life history demographic parameters were strongly correlated with food quality. The age at which the first offspring appeared (P1) was younger significantly with the enriching phosphorus in the algae (p < 0.05). In addition to earlier maturation, rotifers fed with the P-insufficient food (Control, LP and MP) reproduced for a longer duration (P2) than those fed with P-sufficient food (HP) (p < 0.05). There was no significant effect of food quality on the duration of post-reproductive period (P3) except for the significantly longer P3 in the HP group than that in MP (p > 0.05) (). Correspondingly, the mean lifespan (ML), the life expectancy at hatching (e0) and the generation time (T) of rotifers fed with P-poor food were significantly longer than those fed with P-rich food (p < 0.05) (). On the other hand, rotifers in the P-rich groups had high fecundity, as evidenced by a significant increase in the number of offspring (NO), the net reproductive rate (R0) and the intrinsic rate of increase (rm) with raising of P content in food (p < 0.05) ().
Figure 4. Life history parameters of rotifers in response to different algae quality (Mean ± SE). (I) Duration of productive period; (II) Duration of average lifespan, life expectancy at hatching and generation time; (III) offspring productions; (IV) Intrinsic rate of population increase. Different capitals indicated significant difference among treated food groups (p < 0.05).
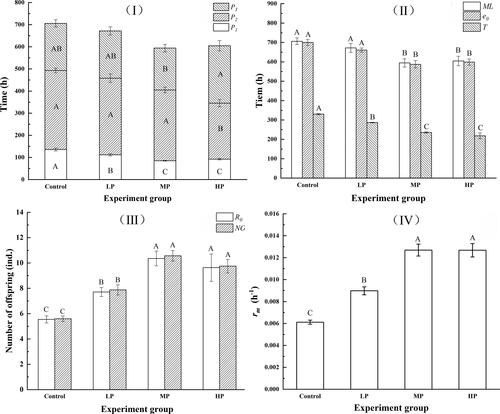
We compared the survivorship and fecundity of rotifers fed with different food quality of algae using standard life table methods (). The Kaplan-Meier survival analysis showed that no matter what the food quality was, food quality had no significant influence on the survivorship of R. rotatoria (p > 0.05). However, the age-specific survivorship (lx) of treatment groups tended to decrease earlier compared with the control. As for the age-specific fecundity (mx), the maximum values in control, LP, MP and HP groups were 0.50, 0.67, 1.13 and 1.14 ind. respectively, and their reproduction periods lasted 25, 25, 21 and 20 days, moreover, the peaks of all treatments appeared earlier compared with control.
4. Discussion
Compared with terrestrial organic matter, algae are generally recognized as high-quality food for the growth and reproduction of subsequent consumers (Guo et al. Citation2016). However, environmental change-induced fluctuation of P element could cause an alternation of algal food quality directly, which may further lead to significant influence on the subsequent consumers. Previously it was reported that P is the limiting factor for the phytoplankton growth, and its excessive content in the environment leads to the massive growth of algae (Song et al. Citation2018; Young and Beardall Citation2003). While severe P limitation caused algae to develop high C:P and N:P ratio in biomass and that imbalanced stoichiometric components provide poor-quality food for consumers (Souza et al. Citation2012; Sterner and Elser Citation2002). What’s more, the minimum law of Liebig also states that under any circumstances, there is only one factor that can restrict the growth and reproduction of organisms, which shows that the increase in growth rate should stabilize with the increase of P concentration if P is a restrictive element (Becker and Boersma Citation2003). In the present study, population density, environmental capacity, specific growth rate, chlorophyll a, cell sizes and P content in algal cells increased with the gradual enhancement of P in the medium. No matter what the population parameter is, significantly difference was not found between group MP and HP. The experimental results are consistent with the previous theories and supplied more ecological characteristics for algal culture under the fluctuant environment.
The feeding behaviors of zooplankton can be changed over time, depending on endogenous rhythms and exogenous variables (Gustav-Adolf Citation1988). Previous studies have shown that zooplankton can amplify their feeding ability in response to low stoichiometric food to make up for deficiencies in essential elements (Hillebrand et al. Citation2009). Mandal et al. (Citation2018) also found that phytoplankton with high cellular C:N and C:P ratio was grazed more intensely by Daphnia, and fatty acid unsaturation and short average chain length were more important than any aspect of algal stoichiometry in feeding conversion efficiencies of Daphnia. Because of the interdependency among the grazing rate, growth and reproduction, high grazing rates of zooplankton will benefit the growth and reproduction of filter feeders (without limitation of food quantity) and result in rapid growth of zooplankton (Ashforth and Yan Citation2008; Rinke and Vijverberg Citation2005). In this study, the results showed that S. obliquus cultured in BG-11 medium with low P concentration (0–0.02 mg/L) had higher C:P ratios, which resulted in the grazing and filtration rates of R. rotatoria fed with P-poor food were significantly higher than those fed with P-rich food. Our results indicate that rotifers increase their food consumption rate in order to compensate for low stoichiometric food quality. However, the offspring productions of rotifers with high feeding rates (Control and LP) were significantly lower than those with low feeding rates (MP and HP), which may attribute to the interpretation that the increasing food quantity does not offset the restriction of low food quality on the reproduction of rotifers. The effect of S. obliquus on R. rotatoria reproduction is likely a combined influence of grazing resistance and poor food quality (element composition).
Rotifera is one of the oldest model organisms in ecology, evolution and environmental science because of its sensitivity to environmental changes. Many studies have shown that the difference in life-history strategies of zooplankton induced by varied food quality (Lürling et al. Citation1997; Vanni and Lampert Citation1992). Jensen and Verschoor (Citation2004) found that the duration of juvenile period, reproductive period and post-reproductive period of Brachionus calyciflorus differed significantly among food types. Moreover, Vanni (Citation1987) suggested that the age at first reproduction of Daphnia was earlier in eutrophic lake water. In this study, the duration of juvenile period and reproductive period were significantly shortened with the increase of P content in food. Our results are consistent with this conclusion. Due to the difference in age structure, the mean lifespan, generation time and the life expectancy at hatching of rotifers fed with P-poor algae are significantly longer than those with P-rich food, which could be attributed to the faster metabolic rate under sufficient phosphorus supply.
The intrinsic rate of population growth, a good indicator of a specific population response to environment changes, can sensitively reflect subtle changes in the environment. In the study on the effect of different nutritional limitations on the growth rate, Kilham et al. (Citation1997) found that population growth rates of Daphnia pulicaria reduced significantly under conditions of P-poor algae. In addition, the P-poor diet may bring the population density and biomass down compared with the P-rich food (Pulkkinen et al. Citation2014). In our study, the highest intrinsic rates of population growth occurred when R. rotatoria fed with P-rich algae. At the same time, the intrinsic rates of population growth increased significantly with the raise of P content in food in a certain range.
Conclusion
This study indicated that the chlorophyta S. obliquus, which is one of the most widespread and dominant mixotrophic flagellate genus in freshwater, generated the P-poor and P-rich food quality and altered the life history strategy in rotifers. Briefly, the P concentration in environment directly affected the growth of algae, including the population density, specific growth rate, photosynthetic pigment and food quality (element ratio and cells sizes). Furthermore, P-rich algae significantly shortened the duration of development and reproductive period by accelerating the metabolic rate, but with the compensation of increasing offspring reproduction and net reproductive rate. We compared the feeding behaviors of R. rotatoria by providing four types of algae cultured under different P concentrations, not only grazing rate but also filtration rate enhanced significantly as rotifers fed with P-poor food. Consequently, it was concluded that the P content not only affected the growth of primary producer directly, but also affected the subsequent consumers indirectly via alteration in the stoichiometric food quality. This study may improve our understanding of the underlying role of phosphorus in affecting aquatic food chain in complex natural environment.
Disclosure statement
The authors declare that they have no conflict interest.
Additional information
Funding
References
- Andersen RA. 2005. Algal culturing techniques. Amsterdam, Netherlands: Elsevier Academic Press; p. 435–436.
- Anne-Marie K, Yee W, Loh SH, Aziz A, Cha TS. 2020. Effects of excess and limited phosphate on biomass, lipid and fatty acid contents and the expression of four fatty acid desaturase genes in the tropical Selenastraceaen Messastrum gracile SE-MC4. Appl Biochem Biotechnol. 190(4):1438–1145.
- Ashforth D, Yan ND. 2008. The interactive effects of calcium concentration and temperature on the survival and reproduction of Daphnia pulex at high and low food concentrations. Limnol Oceanogr. 53(2):420–432.
- Aversa R, Petrescu RV, Apicella A, Petrescu FI. 2016. The basic elements of life's. Am J Eng Appl Sci. 9(4):1189–1197.
- Bajhaiya AK, Dean AP, Zeef LAH, Webster RE, Pittman JK. 2016. PSR1 is a global transcriptional regulator of phosphorus deficiency responses and carbon storage metabolism in Chlamydomonas reinhardtii. Plant Physiol. 170(3):1216–1234.
- Becker C, Boersma M. 2003. Resource quality effects on life histories of Daphnia. Limnol Oceanogr. 48(2):700–706.
- Brembu T, Mühlroth A, Alipanah L, Bones AM. 2017. The effects of phosphorus limitation on carbon metabolism in diatoms. Phil Trans R Soc B. 372(1728):20160406.
- Burian A, Nielsen JM, Winder M. 2020. Food quantity–quality interactions and their impact on consumer behavior and trophic transfer. Ecol Monogr. 90(1):e01395.
- Challagulla V, Fabbro L, Nayar S. 2015. Biomass, lipid productivity and fatty acid composition of fresh water microalga Rhopalosolen saccatus cultivated under phosphorous limited conditions. Algal Res. 8:69–75.
- Darchambeau F. 2005. Filtration and digestion responses of an elementally homeostatic consumer to changes in food quality: a predictive model. Oikos. 111(2):322–336.
- Elser JJ, Andersen T, Baron JS, Bergström AK, Jansson M, Kyle M, Nydick KR, Steger L, Hessen DO. 2009. Shifts in lake N:P stoichiometry and nutrient limitation driven by atmospheric nitrogen deposition. Science. 326(5954):835–837.
- Elser JJ, Bracken MES, Cleland EE, Gruner DS, Harpole WS, Hillebrand H, Ngai JT, Seabloom EW, Shurin JB, Smith JE. 2007. Global analysis of nitrogen and phosphorus limitation of primary producers in freshwater, marine and terrestrial ecosystems. Ecol Lett. 10(12):1135–1142.
- Elser JJ, Hayakawa K, Urabe J. 2001. Nutrient limitation reduces food quality for zooplankton: Daphnia response to seston phosphorus enrichment. Ecology. 82(3):898–903.
- Filina V, Grinko A, Ermilova E. 2019. Truncated hemoglobins 1 and 2 are implicated in the modulation of phosphorus deficiency-induced nitric oxide levels in Chlamydomonas. Cells. 8(9):947–959.
- Franco-Santos RM, Auel H, Boersma M, De Troch M, Meunier CL, Niehoff B. 2018. Bioenergetics of the copepod Temora longicornis under different nutrient regimes. J Plankton Res. 40(4):420–435.
- Frost BW. 1972. Effects of size and concentration of food particles on the feeding behavior of the marine planktonic copepod Calanus pacificus. Limnol Oceanogr. 17(6):805–815.
- Grossman AR, Aksoy M. 2015. Algae in a phosphorus‐limited landscape. Annu Plant Rev Online. 48:337–374.
- Guo F, Kainz MJ, Sheldon F, Bunn SE. 2016. The importance of high-quality algal food sources in stream food webs – current status and future perspectives. Freshw Biol. 61(6):815–831.
- Gustav-Adolf P. 1988. Feeding rates and behavior of zooplankton. Bull Mar Sci. 43:430–445.
- Hidayati NA, Yamada-Oshima Y, Iwai M, Yamano T, Kajikawa M, Sakurai N, Suda K, Sesoko K, Hori K, Obayashi T, et al. 2019. Lipid remodeling regulator 1 (LRL1) is differently involved in the phosphorus-depletion response from PSR1 in Chlamydomonas reinhardtii. Plant J. 100(3):610–626.
- Hillebrand H, Borer ET, Bracken MES, Cardinale BJ, Cebrian J, Cleland EE, Elser JJ, Gruner DS, Harpole WS, Ngai JT, Sandin S, et al. 2009. Herbivore metabolism and stoichiometry each constrain herbivory at different organizational scales across ecosystems. Ecol Lett. 12(6):516–527.
- Jensen TC, Verschoor AM. 2004. Effects of food quality on life history of the rotifer Brachionus calyciflorus Pallas. Freshwater Biol. 49(9):1138–1151.
- Karl DM. 2014. Microbially mediated transformations of phosphorus in the sea: new views of an old cycle. Ann Rev Mar Sci. 6:279–337.
- Kilham SS, Kreeger DA, Goulden C, Lynn S. 1997. Effects of algal food quality on fecundity and population growth rates of Daphnia. Freshw Biol. 38(3):639–647.
- Kirk KL. 1997. Life-history responses to variable environments: starvation and reproduction in planktonic rotifers. Ecology. 78(2):434–441.2.0.CO;2]
- Lewis JWM, Wurtsbaugh WA. 2008. Control of lacustrine phytoplankton by nutrients: erosion of the phosphorus paradigm. Int Rev Hydrobiol. 93(4-5):446–465.
- Lürling M, DE Lange HJ, VAN Donk E. 1997. Changes in food quality of the green alga: Scenedesmus induced by Daphnia infochemicals: biochemical composition and morphology. Freshw Biol. 38(3):619–628.
- Malzahn AM, Boersma M. 2012. Effects of poor food quality on copepod growth are dose dependent and non-reversible. Oikos. 121(9):1408–1416.
- Mandal S, Wilkins A, Shurin JB. 2018. Compensatory grazing by Daphnia generates a trade-off between top-down and bottom-up effects across phytoplankton taxa. Ecosphere. 9(12):e0253.
- Meunier CL, Boersma M, Wiltshire KH, Wiltshire KH, Malzahn AM. 2016. Zooplankton eat what they need: copepod selective feeding and potential consequences for marine systems. Oikos. 125(1):50–58.
- Mousing EA, Richardson K, Ellegaard M. 2018. Global patterns in phytoplankton biomass and community size structure in relation to macronutrients in the open ocean. Limnol Oceanogr. 63(3):1298–1312.
- Nobili R. 2013. The effects of food quality and temperature on mesozooplankton physiology [PhD thesis]. Norwich, England: University of East Anglia.
- Peltier WH, Weber CI. 1985. Methods for measuring the acute toxicity of effluents to freshwater and marine organisms. United States environmental protect agency. Cincinnati: United States of America.
- Pulkkinen K, Wojewodzic MW, Hessen DO. 2014. Phosphorus limitation enhances parasite impact: feedback effects at the population level. BMC Ecol. 14:29–40.
- Reed ML, Pinckney JL, Keppler CJ, Brock LM, Hogan SB, Greenfield DI. 2016. The influence of nitrogen and phosphorus on phytoplankton growth and assemblage composition in four coastal, southeastern USA systems. Estuar Coast Shelf Sci. 177:71–82.
- Rinke K, Vijverberg J. 2005. A model approach to evaluate the effect of temperature and food concentration on individual life-history and population dynamics of Daphnia. Ecol Modell. 186(3):326–344.
- Roy ED. 2017. Phosphorus recovery and recycling with ecological engineering: a review. Ecol Eng. 98:213–227.
- Schälicke S, Sobisch LY, Martin-Creuzburg D, Wacker A. 2019. Food quantity-quality co-limitation: interactive effects of dietary carbon and essential lipid supply on population growth of a freshwater rotifer. Freshw Biol. 64(5):903–912.
- Schindler DW, Carpenter SR, Chapra SC, Hecky RE, Orihel DM. 2016. Reducing phosphorus to curb Lake Eutrophication is a success. Environ Sci Technol. 50(17):8923–8929.
- Schmoker C, Russo F, Drillet G, Trottet A, Mahjoub MS, Hsiao SH, Larsen O, Tun K, Calbet A. 2016. Effects of eutrophication on the planktonic food web dynamics of marine coastal ecosystems: the case study of two tropical inlets. Mar Environ Res. 119:176–188.
- Song WW, Fu XQ, Pang Y, Song DH, Xu Q, Zhang P. 2018. Research on water environment regulation of artificial playground lake interconnected Yangtze River. IJERPH. 15(10):2110–2139.
- Souza V, Siefert J, Escalante AE, Elser JJ, Eguiarte LE. 2012. The Cuatro Ciénegas Basin in Coahuila, Mexico: an astrobiological Precambrian Park. Astrobiology. 12(7):641–647.
- Sterner RW, Elser JJ. 2002. Ecological stoichiometry: the biology of element from molecules to biosphere. United States of America: Princeton University Press.
- Stockner JG, Rydin E, Hyenstrand P. 2000. Cultural oligotrophication: cause and consequences for fisheries resources. Fisheries. 25(5):7–14.
- Teufel AG, Li W, Kiss AJ, Morgan-Kiss RM. 2017. Impact of nitrogen and phosphorus on phytoplankton production and bacterial community structure in two stratified Antarctic lakes: a bioassay approach. Polar Biol. 40(5):1007–1022.
- Vad CF, Schneider C, Lukic D, Horváth Z, Kainz MJ, Stibor H, P Tacnik R. 2020. Grazing resistance and poor food quality of a widespread mixotroph impair zooplankton secondary production. Oecologia. 193(2):489–502.
- Vanni MJ. 1987. Colonization dynamics and life history traits of seven Daphnia pulex genotypes. Oecologia. 72(2):263–271.
- Vanni MJ, Lampert W. 1992. Food quality effects on life history traits and fitness in the generalist herbivore Daphnia. Oecologia. 92(1):48–57.
- Xu H, Paerl HW, Qin BQ, Zhu GW, Gaoa G. 2010. Nitrogen and phosphorus inputs control phytoplankton growth in eutrophic Lake Taihu, China. Limnol Oceanogr. 55(1):420–432.
- Young EB, Beardall J. 2003. Rapid ammonium- and nitrate-induced perturbations to Chl a fluorescence in nitrogen-stressed Dunaliella tertiolecta (Chlorophyta). J Phycol. 39(2):332–342.
- Zhou L. 2019. Stoichiometric mismatch between phytoplankton and zooplankton consumers: Effects at contemporary, transgenerational, and evolutionary timescales [PhD thesis]. The Netherlands: Utrecht University.