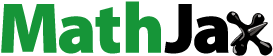
Abstract
The Bigeye Chub (Hybopsis amblops) once inhabited streams and rivers in eastern and southeastern Illinois and was thought to be extirpated in the state by the 1970s. However, it was rediscovered in the 1990s from the Little Vermilion River. Today, H. amblops has become widespread and locally abundant in streams of the Wabash River drainage. Currently, little is known about the spawning and reproductive ecology of H. amblops. We conducted a study in the Salt Fork Vermilion River to determine the spawning schedule and microhabitats of H. amblops. Based upon near-weekly samples in May and June in 2019 and 2021, we determined H. amblops spawns in moderate water velocity over sandy gravel substrates when water temperatures are between 18–22 °C. Understanding such ecological traits is necessary for the continued success of H. amblops throughout its range.
Introduction
With the passage of the Clean Water Act in 1972, rehabilitating much of the United States’ polluted river and stream ecosystems has been focal. Calls have been made for restoration efforts focused at the watershed level by implementing a series of hierarchical strategies focusing on water quality, connectivity, restoration of natural ecological processes, and habitat enhancement (Roni et al. Citation2002). Nation-wide, stream restoration projects have been numerous and focused on all levels; however, follow-up monitoring of their success has been sorely lacking (Bernhardt et al. Citation2005; Alexander and Allan Citation2006). Limited monitoring of restoration and rehabilitation efforts shows recovery in numerous temperate-stream fish communities affected by chronic and transient perturbations (Detenbeck et al. Citation1992). Although metrics such as species richness can illustrate recovery within a year to pulse disturbances, population densities of resilient species took longer to recover, and recovery of less resilient species is difficult to quantify (Detenbeck et al. Citation1992). Often colonization is directly linked to how fast a species can recover from environmental perturbations and habitat degradation, although with significant inter-specific variation. Removal studies within a stream fish community found inter-specific variation in recovery rates related to upstream movement (Albanese et al. Citation2009). However, there must be sources from where colonizers can expand and suitable habitats for biological processes such as spawning for population recovery.
An imperiled species showing evidence of recovery in Illinois is the state-threatened Bigeye Chub (Hybopsis amblops) (Family Leuciscidae). It was formerly widespread across southern and eastern Illinois and could be found in rocky, free-flowing areas, often near vegetation in small to medium rivers (Smith Citation1979; Page and Retzer Citation2002; Sherwood and Wylie Citation2015). However, H. amblops declined in abundance and range due to water quality degradation and habitat alterations in Illinois, and thus likely became extirpated by the 1970s (Smith Citation1971; Smith Citation1979; Warren and Burr Citation1988). In 1992, H. amblops was rediscovered from the Little Vermilion River (Burr et al. Citation1996), and populations have since rebounded in Illinois, likely because of improved physicochemical conditions of streams. The minnow now occurs throughout the Wabash River drainage and a small portion of the Kankakee River drainage (Burr et al. Citation1996; Page and Retzer Citation2002; Tiemann et al. Citation2004; Sherwood and Wylie Citation2015). This distributional expansion and increase in abundance in Illinois resulted in downlisting the fish's conservation status from state-endangered (IESPB Citation2015) to state-threatened (IESPB 2000).
Currently, little is known about the spawning and reproductive ecology of H. amblops (Pflieger Citation1997; Kansas Fish Committee Citation2014). Documenting such ecological traits is necessary to understand the continued success of the species throughout its range, especially Illinois, where populations in the Wabash River (e.g. Sherwood and Wylie Citation2015) and Kankakee River drainages (e.g. Tiemann et al. Citation2004) represent a unique lineage (Berendzen et al. Citation2008). Our objectives were to determine the spawning season of H. amblops in Illinois and evaluate environmental correlates for spawning individuals. Our results help provide crucial information in the conservation of this imperiled fish.
Methods
Study area
Our study occurred in the Salt Fork Vermilion River (Wabash River drainage) at the University of Illinois' Richter Research Area in Vermilion County, Illinois (). The Salt Fork basin experienced several anthropogenic disturbances in the late 1800s and early 1900s, including domestic sewage, industrial and agricultural pollution, impoundments, siltation, and dredging (Baker Citation1922; Larimore and Smith Citation1963; Smith Citation1968). However, those disturbances subsided, and the physicochemical conditions in the basin have improved (Page et al. Citation1992; Larimore and Bayley Citation1996); subsequently, the Salt Fork has been rated as a 'Highly Valued Aquatic Resource' (Bertrand et al. Citation1996). As a result of improved conditions, the fish assemblage in the Salt Fork basin appears to be recovering, including the range expansion of several rare taxa like H. amblops (Tiemann Citation2008; Sherwood and Wylie Citation2015; Tiemann et al. Citation2015; Tiemann et al. Citation2020; Tiemann et al. Citation2021).
Sampling
We performed near-weekly surveys for H. amblops at 10 points along the Salt Fork in May–June 2019 and May–June 2021, as stream conditions allowed. Sampling points, which remained constant throughout the study, represented different habitat types, such as riffle, run, pool segments with varying levels of woody debris, water velocities, and substrate composition. However, we encountered no aquatic vegetation during our surveys. Of these 10 points, 5 were below, 2 within, and 3 above a large riffle. We sampled each point with three consecutive 10-15 m seine hauls using a 3.1 m minnow seine. We recorded the total length (TL; mm) and reproductive status (i.e. males emitting milt or having minute tubercles [rough texture when feeling the top of the head]; females with swollen, firm bellies) of each H. amblops taken in each pass for each point. Water temperatures were <14 °C at the onset of sampling during both years, and sampling was performed until evidence of spawning had ceased (i.e. males lost tubercles and no longer emitted sperm when milted or females' stomachs were in a flaccid, empty state).
We measured water depth (mm) with a meter stick and water velocity (cm/sec) at 50% stream depth using a FloWatch Flowmeter (JDC Electronic, Switzerland) before surveys at each point. We visually categorized substrate composition as percent silt, sand, gravel/pebble, cobble, and boulder based upon a modified Wentworth scale (Cummins Citation1962; McMahon et al. Citation1996; Mullner et al. Citation2000). We then defined a dominant substrate type for each site based on these scores. In 2019, we recorded surface water temperature using a YSI Professional Plus multiparameter instrument (YSI Incorporated, Yellow Springs, OH) after each sample. In April 2021, we deployed a HOBO Pendant MX data logger (Onset Computer Corporation, Bourne, MA) after all sampling efforts when the water temperature was 8 °C. We attached the datalogger to a brick using a zip-tie and then staked it to the streambed using rebar downstream of all sampling locations and set it to record temperatures continuously at hourly intervals.
Data analysis
We converted date to a numerical day of year variable to examine potential seasonality. Next, we transformed all continuous variables into z-scores, representing normal deviates on the same scale. We constructed probability distributions for each variable using univariate kernels to determine shape and variation in the sampled data. We then calculated Pearson correlation coefficients and variance inflation factors among variables to assess multicollinearity. Using the brms package (Bürkner Citation2017, Citation2018, Citation2021), we constructed Bayesian linear models and set the estimation conditions at 50,000 iterations, 4 MCMC chains, a 10,000 iteration warmup, and all initial parameter estimates at zero (no effect). We used generic weakly informative priors for all β parameters as normal(0,1) and the intercept as Student's t(3,0,2.5). To determine if the probability of spawning H. amblops, we constructed an additive model using dominant substrate, depth, velocity, and day of year. Unfortunately, we could not use temperature in the model due to the differences in how temperature was recorded between years. We then calculated R2s adapted for Bayesian regression methods (Gelman et al. Citation2019) implemented in the Rstan package (Stan Development Team Citation2021). To determine the effects of each variable, we examined their posterior distributions of parameters and then calculated conditional effects in package brms. All analyses were conducted using R version 4.1.2 (R Core Team Citation2021). Model diagnostics followed recommendations from Gabry et al. (Citation2019), and all graphics were constructed/redrawn with the R packages ggplot2 (Wickham Citation2019) and patchwork (Pedersen Citation2020) from ggplot2 or bayesplot (Gabry and Mahr Citation2021) objects.
Results
We captured 48 H. amblops in 2019 and 84 in 2021. Of those 132 fish, 100 (N = 42 in 2019, N = 58 in 2021) were classified as spawning, evidenced by tubercled, milting males and ripe females. The sex ratio of spawning individuals was 2 M:1F, and both spawning males and females ranged from 54-84 mm TL. We captured spawning individuals when water temperatures were between 18–22 °C (; ). In 2019, stream conditions (i.e. high water) prevented us from sampling from June 13-28, after which all adults seemed to be post-spawned based upon loss of tubercles and no sperm emitted when milted for males or females with flaccid stomachs. In 2021, water temperatures reached 24 °C by early June, and spawning appeared to have been completed by our June 3 sample (; ).
Figure 2. Dates sampling occurred for Hybopsis amblops in 2019 and 2021, with summaries of total spawning fish captured, water temperature (surface in 2019 and benthic in 2021), water depth (cm), and velocity (m/sec). Whiskers represent the 95% C.I., box represents 1 standard error, and dark horizontal line represents the mean values for the 10 transects.
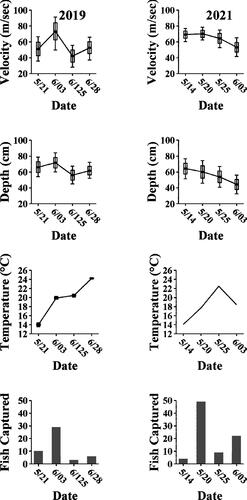
Table 1. Summary statistics for the covariates of numerical day of year (DOY), velocity (m/sec), depth (cm), and temperature (°C) for all Bigeye Chub (Hybopsis amblops) spawning surveys conducted within the Salt Fork Vermilion River sub-basin, Illinois, in 2019 and 2021 combined by dominant substrate (Sand or Gravel) and whether breeding individuals were observed.
We collected spawning H. amblops in 5 of the 10 points sampled in 2019 and 2021, including upstream (e.g. the tail of a pool), within, and downstream (e.g. a run) of the large sandy gravel/cobble riffle. Spawning H. amblops was found in depths varying from 30 to 95 cm (mean: 58.8 ± 18.7 cm SD) and water velocities ranging from 2 to 90 cm/s (mean: 40.7 ± 28.7 cm/s SD; ; ). Only depth and velocity and the number of spawning H. amblops were significantly correlated (). Deeper water tended to be flowing at a faster velocity (p ≪ 0.001), and more spawning H. amblops were captured in shallower water (p = 0.02; ).
Figure 3. Pearson correlation coefficients among sampling site water depth (cm), velocity (m/sec), day of year, and total spawning Bigeye Chub (Hybopsis amblops) captured in 2019 and 2021.
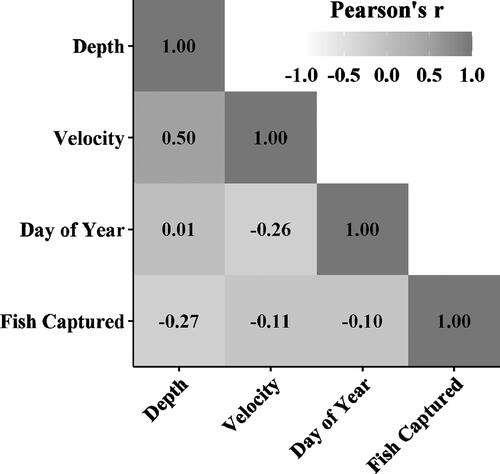
Model diagnostics
The probability density functions for all continuous variables showed nearly normal distributions with a day of year having multiple modes – reflective of the short, four-week sampling period (Supplemental 1). All variables had relatively thin tails suggesting we have undersampled these regions and should expect more uncertainty when examining predictions and effects at their respective extremes (Supplemental 1). All variables had relatively low variance inflation factors (well below 5); thus, multicollinearity was likely not an issue, and we could employ models using all four variables (Supplemental 2). Trace plots showed all MCMC chains quickly stabilized from the initial values of zero (Supplemental 3). Chains for depth, day of year, and velocity overlapped zero, and all chains show greater uncertainty in estimations (Supplemental 3). Pareto smoothed importance sampling found all data points well below the 0.5 threshold (Supplemental 4), showing practical MCMC convergence rates and reliable error estimates (Vehtari et al. Citation2017, Citation2020). The posterior probability check suggests our global model of the factors affecting the probability of H. amblops breeding has good predictability (Supplemental 5). The posterior probability distributions for the β-parameters of velocity, day of year, and depth have 95% Credible Intervals overlapping zero, meaning we may find little support for their influence on the probability of H. amblops spawning (Supplemental 6).
Predictive model
Overall, our model of the factors affecting H. amblops spawning was weakly informative (R2Bayes = 0.112, 95% C.I. − 0.057,0.161), suggesting variation was better explained by variables we did not account for (e.g. water temperature). As evidenced from model diagnostics, there is more uncertainty (larger 95% credible intervals), at the tails of our sampling efforts, particularly for later in the season, shallower and deeper depths, and higher water velocities (; ). However, H. amblops had a higher probability of spawning over gravel substrates than sand ; (). In general, there was a lower probability of spawning when water velocity was slower, but the probability increased with faster water velocities (; ). The probability of H. amblops spawning increased temporally, with the greatest probability occurring near the sampling period's ends (; ). Finally, despite a slight relationship, the effects of depth were weak and bounded by greater uncertainty (; ).
Figure 4. Bayesian conditional effects of dominant substrate, water velocity (m/sec), day of year, and water depth (cm) on the probability Bigeye Chub (Hybopsis amblops) breeding, with the gray bands representing the 95% Credible Intervals.
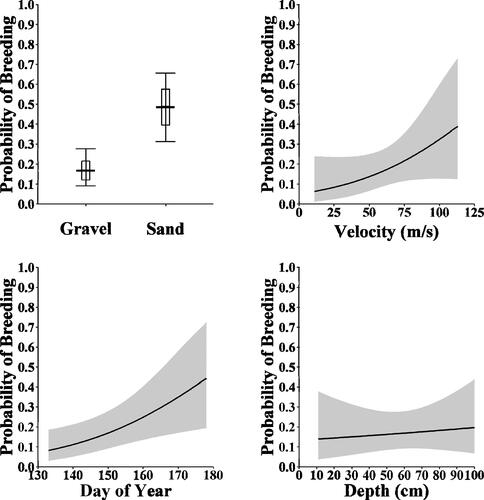
Table 2. Bayesian regression model parameter estimates, estimated error, 95% Credible Intervals, and bulk and tail estimated sample sizes for Bigeye Chub (Hybopsis amblops) spawning surveys conducted within the Salt Fork Vermilion River sub-basin, Illinois, in 2019 and 2021.
Discussion
Our study offers insight into the spawning period of one of Illinois' rarest fishes, the Bigeye Chub. Before our study, the spawning season of H. amblops was simply stated as 'late spring to early summer' (Pflieger Citation1997; Kansas Fish Committee Citation2014). Our project in the Vermilion River basin, which is at the northern edge of the fish's range, suggests H. amblops spawns between mid-May and mid-June as the water temperature remains consistently between 18–22 °C. A study by B. Stallsmith (University of Alabama-Huntsville, pers. coms.) in the Flint River system in Alabama, which is at the southern edge of the fish's range, found a peak period for mature oocyte development occurred when water temperatures were between 17–21 °C.
Our predictive model showed H. amblops had a higher probability of spawning over gravel substrates and moderate water velocities. The five points where spawning H. amblops were captured occurred within a sandy gravel run downstream of the riffle, often in areas away from the thalweg (i.e. near stream margins). While there were differences in substrate composition among sampling points, the streambed was relatively homogenous, with sandy gravel dominating every sampling point; very little silt or boulder was present. Our results agree with others that H. amblops occurs in sandy gravel runs at the base of riffles (i.e. Warren and Burr Citation1988; Pflieger Citation1997) and is likely because the fish body shape is heavily influenced by stream flow (Foster et al. Citation2015; Akin and Geheber Citation2020).
We examined discharge readings from the nearest U.S. Geological Survey (USGS) National Water Information System stream monitoring gage (No. 03336900). While there were minor high-water events in early May in both 2019 and 2021 as water temperatures approached 17 °C, we are hesitant to speculate whether elevated discharge or floods were also a cue to spawn for H. amblops, as has been reported for other minnows (Starrett Citation1951; Hodgskins et al. Citation2016). The gage is located approximately 50 river km upstream of our study site, and several large tributaries flow into the Salt Fork downstream and therefore would not be a true representation of discharge at the project area. Future studies could incorporate a discharge variable into their predictive models to possibly improve predictability.
We did not attempt to observe H. amblops spawning in nature, so we cannot provide additional specifics to the spawning ecology of the species. Given the imperiled status of H. amblops, we did not sacrifice individuals to determine fecundity. Johnston (Citation1999) speculated Hybopsis spp. are broadcast spawners, and the Fishtraits Database (Frimpong and Angermeier Citation2009) suggested H. amblops is likely a lithophilic spawner. If true, lithophilic species often have stringent requirements for optimal spawning conditions (e.g. the composition of spawning substrates, water temperature, and water velocity), which could make H. amblops susceptible to physicochemical conditions and hydro-geomorphological alterations (van Treeck et al. 2000).
Over the last few decades, Bayesian methods are becoming more readily accessible to ecologists (McCarthy Citation2007) and have been used to answer numerous ecological questions (Mollenhauer et al. Citation2020). Their wider use can be tracked to high computational demands being alleviated (Dorazio Citation2016) and group efforts on packages and programming like brms, rstan, and Stan (Bürkner Citation2017, Citation2018, Citation2021; Stan Development Team Citation2021). Bayesian methods can make strong use of prior data or information (e.g. McCarthy and Masters Citation2005; Banner et al. Citation2020) and provide solid inference at even smaller sample sizes (Dorazio Citation2016). Thus, Bayesian methods are tractable when dealing with conservation biology, and the low sample sizes often observed with rare species (Wade Citation2000; Poirazidis et al. Citation2004; Kindsvater et al. Citation2018). Unfortunately, not even the strength of Bayesian analysis can correct for poor descriptor variables. Although our study did not have great predictive strength, it helps provide important information on the timing and microhabitat preference for spawning H. amblops, which can then be used for conservation efforts of this critically imperiled fish.
Supplemental Material
Download MS Word (393.8 KB)Acknowledgments
We thank J. Ellis for providing access to the University of Illinois' Richter Research Area. We also thank K. Conaster, I. Hanson, J. Reinhofer, and P. Willink for assisting with sample collection. We thank A. Rahlin for providing a critical review of our Bayesian methods and interpretations and B. Stallsmith for sharing accounts of Bigeye Chub from their study in Alabama. Fishes were collected under the University of Illinois' Institutional Animal Care and Use Committee (IACUC) Protocol #19241. We conducted research under IDNR Threatened and Endangered Species Permits 3854 (2019) and 10766 (2021).
Data availability statement
The data that support the findings of this study are available from the corresponding author, JST, upon reasonable request.
Disclosure statement
No potential conflict of interest.
Additional information
Funding
Notes on contributors
Jeremy S. Tiemann
Jeremy S. Tiemann is an aquatic ecologist with the Illinois Natural History Survey.
Sarah A. Douglass
Sarah A. Douglass is a malacologist with the Illinois Natural History Survey.
Josh L. Sherwood
Josh L. Sherwood is a former fish biologist with the Illinois Natural History Survey but currently is a firefighter for the U.S. Air Force.
Andrew J. Stites
Andrew J. Stites is currently a fish biologist with the Arizona Game and Fish Department.
Michael J. Dreslik
Michael J. Dreslik is a herpetologist the Illinois Natural History Survey.
References
- Akin DR, Geheber AD. 2020. Conforming to the status flow: the influence of altered habitat on fish body-shape characteristics. Freshw Biol. 65(11):1883–1893.
- Albanese B, Angermeier PL, Peterson JT. 2009. Does mobility explain variation in colonisation and population recovery among stream fishes? Freshw Biol. 54(7):1444–1460.
- Alexander GG, Allan JD. 2006. Stream restoration in the Upper Midwest, U.S.A. Restor Ecol. 14(4):595–604.
- Baker FC. 1922. The molluscan fauna of the Big Vermilion River, Illinois, with special reference to its modification as a result of pollution by sewage and manufacturing wastes. Illinois Biol Monograph. 7:105–224. + 15 pls.
- Banner KM, Irvine KM, Rodhouse TJ. 2020. The use of Bayesian priors in ecology: the good, the bad, and the not great. Method Ecol Evol. 11(8):882–889.
- Berendzen PB, Gamble T, Simons AM. 2008. Phylogeography of the Bigeye Chub, Hybopsis amblops (Teleostei: Cypriniformes): early Pleistocene diversification and post-glacial range expansion. J Fish Biol. 73(8):2021–2039.
- Bernhardt ES, Palmer MA, Allan JD, Alexander G, Barnas K, Brooks S, Carr J, Clayton S, Dahm C, Follstad-Shah J, et al. 2005. Ecology. Synthesizing U.S. river restoration efforts. Science. 308(5722):636–637.
- Bertrand WA, Hite RL, Day DM. 1996. Biological stream characterization (BSC): biological assessment of Illinois stream quality through 1993. Biological Streams Characterization Work Group, Springfield, Illinois. pp. 44.
- Bürkner PC. 2017. BRMS: an R Package for Bayesian Multilevel Models Using Stan. J Stat Soft. 80(1):1–28.
- Bürkner PC. 2018. Advanced Bayesian Multilevel Modeling with the R Package brms. R J. 10(1):395–411.
- Bürkner PC. 2021. Bayesian Item Response Modeling in R with brms and Stan. J Stat Soft. 100(5):1–54.
- Burr BM, Cook KM, Eisenhour DJ, Piller KR, Poly WJ, Sauer RW, et al. 1996. Selected Illinois fishes in jeopardy: new records and status evaluations. Transact Illinois State Academy Sci. 89:169–186.
- Cummins KW. 1962. An evaluation of some techniques for the collection and analysis of benthic samples, with special emphasis on lotic waters. American Midland Naturalist. 67(2):477–504.
- Detenbeck NE, DeVore PW, Niemi GJ, Lima A. 1992. Recovery of temperate-stream fish communities from disturbance: a review of case studies and synthesis of theory. Environ Manage. 16(1):33–53.
- Dorazio RM. 2016. Bayesian data analysis in population ecology: motivations, methods, and benefits. Popul Ecol. 58(1):31–44.
- Foster K, Bower L, Piller K. 2015. Getting in shape: habitat-based morphological divergence for two sympatric fishes. Biol J Linn Soc Lond. 114(1):152–162.
- Frimpong EA, Angermeier PL. 2009. FishTraits: a database of ecological and life-history traits of freshwater fishes of the United States. Fisheries. 34(10):487–495.
- Gabry J, Simpson D, Vehtari A, Betancourt M, Gelman A. 2019. Visualization in Bayesian workflow. J R Stat Soc A. 182(2):389–402.
- Gabry J, Mahr T. 2021. "Bayesplot: Plotting for Bayesian Models." R package version 1.8.1, <URL: https://mc-stan.org/bayesplot/>.
- Gelman A, Goodrich B, Gabry J, Vehtari A. 2019. R-squared for Bayesian regression models. American Statistician. 73(3):307–309.
- Hodgskins K, Greenleaf S, Hillman J, Stallsmith B. 2016. Reproductive schedule of the Silver Shiner (Notropis photogenis) in the Flint River of Alabama. Southeastern Fishes Council Proceed. 56:12–31.
- Illinois Endangered Species Protection Board (IESPB). 2015. Checklist of Endangered and Threatened Animals and Plants of Illinois. Illinois: Endangered Species Protection Board, Springfield; pp. 18.
- Illinois Endangered Species Protection Board (IESPB). 2020. Checklist of endangered and threatened animals and plants of Illinois. Illinois: Endangered Species Protection Board, Springfield; pp. 10.
- Johnston CE. 1999. The relationship of spawning mode to conservation of North American minnows (Cyprinidae). Environ Biol Fish. 55(1–2):21–30.
- Kansas Fish Committee 2014. Kansas Fishes. Lawrence: University of Kansas Press; pp. 496.
- Kindsvater HK, Dulvy NK, Horswill C, Juan-Jordá M-J, Mangel M, Matthiopoulos J. 2018. Overcoming the data crisis in biodiversity conservation. Trends Ecol Evol. 33(9):676–688.
- Larimore RW, Bayley PB. 1996. The fishes of Champaign County, Illinois, during a century of alterations of a prairie ecosystem. INHS Bulletin. 35(1-5):53–183.
- Larimore RW, Smith PW. 1963. The fishes of Champaign County, Illinois, as affected by 60 years of stream changes. INHS Bulletin. 28(1-3):299–382.
- McCarthy MA. 2007. Bayesian methods for ecology. United Kingdom: Cambridge University Press.
- McCarthy MA, Masters P. 2005. Profiting from prior information in Bayesian analyses of ecological data. J Appl Ecol. 42(6):1012–1019.
- McMahon TE, Zale AV, Orth DJ. 1996. Aquatic habitat measurements (pp. 83–120). In: Murphy DR, Willis DW, editors. Fisheries techniques. 2nd ed. American Fisheries Society, Bethesda, Maryland; pp. 732.
- Mollenhauer R, Miller AD, Goff J, Brewer SK. 2020. The influence of groundwater on the population size and total length of warmwater stream fishes. Southeastern Naturalist. 19(2):308–324.
- Mullner SA, Hubert WA, Wesche TA. 2000. Visually estimating substrate composition at potential spawning sites for trout in mountain streams. J Freshw Ecol. 15(2):199–207.
- Page LM, Cummings Mayer CA, Post SL, Retzer ME. 1992. Biologically significant Illinois streams. An evaluation of the streams of Illinois based on aquatic biodiversity. Illinois Natural History Survey, Center for Biodiversity. Technical Report 1992(1). Champaign, Illinois; pp. 485
- Page LM, Retzer ME. 2002. The status of Illinois' rarest fishes and crustaceans. Transact Illinois State Academy Sci. 95:311–326.
- Pedersen TL. 2020. Patchwork: The Composer of Plots. R package version 1.1.1. https://CRAN.R-project.org/package=patchwork.
- Pflieger WL. 1997. The Fishes of Missouri. Jefferson City: Missouri Department of Conservation; pp. 371.
- Poirazidis K, Goutner V, Skartsi T, Stamou G. 2004. Modelling nesting habitat as a conservation tool for the Eurasian black vulture (Aegypius monachus) in Daidia Nature Reserve, northeastern Greece. Biol Conserv. 118(2):235–248.
- R Core Team. 2021. R: A language and environment for statistical computing. Vienna, Austria: R Foundation for Statistical Computing. https://www.R-project.org/.
- Roni P, Beechie TJ, Bilby RE, Leonetti FE, Pollock MM, Pess GR. 2002. A review of stream restoration techniques and a hierarchical strategy for prioritizing restoration in pacific northwest watersheds. North Am J Fish Manage. 22(1):1–20.
- Sherwood JL, Wylie DB. 2015. Current status and historic range of one of Illinois' rarest fishes. Transact Illinois State Academy Sci. 108:43–46.
- Smith PW. 1968. An assessment of changes in the fish fauna of two Illinois rivers and its bearing on their future. Transact Illinois State Academy Sci. 61:31–45.
- Smith PW. 1971. Illinois streams: a classification based on their fishes and an analysis of factors responsible for disappearance of native species. Illinois Natural History Survey Bioll Note. 76:14. pp.
- Smith PW. 1979. The fishes of Illinois. Urbana: University of Illinois Press; pp. 314.
- Stan Development Team. 2021. RStan: the R interface to Stan. R package version 2.21.3. https://mc-stan.org/.
- Starrett WC. 1951. Some factors affecting the abundance of minnows in the Des Moines River, Iowa. Ecology. 32(1):13–27.
- Tiemann JS. 2008. Distribution and life history characteristics of the state-endangered Bluebreast Darter Etheostoma camurum (Cope) in Illinois. Transact Illinois State Academy Sci. 101:235–246.
- Tiemann JS, Fisher BE, Thomas T, Adams J, Moody-Carpenter C, Jones A, Colombo RE. 2021. First occurrence of the Streamline Chub Erimystax dissimilis and Tippecanoe Darter Etheostoma tippecanoe in Illinois and within the Vermilion River basin (Wabash River drainage). Transact Illinois State Academy Sci. 114:35–38.
- Tiemann JS, Retzer ME, Tiemann BL. 2004. Range expansion of the state-endangered Bigeye Chub Hybopsis amblops (Rafinesque) in Illinois. Transact Illinois State Academy Sci. 97:255–257.
- Tiemann JS, Sherwood JL, Stites AJ. 2020. Population expansion of the state-threatened Eastern Sand Darter, Ammocrypta pellucida (Agassiz, 1863), within the Vermilion River basin (Wabash River drainage). Illinois Transact Illinois State Academy Sci. 113:1–4.
- Tiemann JS, Taylor CA, Lamer J, Willink PW, Veraldi FM, Pescitelli S, et al. 2015. Range expansions and new drainage records for select Illinois fishes. Transact Illinois State Academy Sci. 108:47–52.
- van Treeck R, Van Wichelen J, Wolter C. 2020. Fish species sensitivity classification for environmental impact assessment, conservation and restoration planning. Sci Total Environ. 708:135–173.
- Vehtari A, Gelman A, Gabry J. 2017. Practical Bayesian model evaluation using leave-one-out cross-validation and WAIC. Stat Comput. 27(5):1413–1432.
- Vehtari A, Gabry J, Magnusson M, Yao Y, Bürkner P, Paananen T, Gelman A. 2020. "loo: Efficient leave-one-out cross-validation and WAIC for Bayesian models." R package version 2.4.1, <URL: https://mc-stan.org/loo/>.
- Wade PR. 2000. Bayesian methods in conservation biology. Conserv Biol. 14(5):1308–1316.
- Warren ML, Jr., Burr BM. 1988. Reassessment of the Illinois ranges of the Bigeye Chub, Hybopsis amblops, and the Pallid Shiner, Notropis amnis. Ohio J Sci. 88:181–183.
- Wickham H. 2019. Ggplot2: elegant graphics for data analysis. New York, NY: Springer-Verlag.