Abstract
Regulatory methods for the measurement of particulate matter (PM) mass emissions have traditionally been gravimetric. Modern diesel engines equipped with aftertreatment systems, especially Diesel Particulate Filters (DPFs), however, emit much smaller amounts of particulate matter as compared to traditional diesel engines and emit particulate matter with variable compositions. These changes have led to difficulties in measuring PM emissions rates from modern diesel engines using gravimetric methods. Issues associated with diesel PM mass measurement, such as the semi-volatile nature of PM, the interactions with components in the dilution air such as water and ammonia, and the possibility of sampling artifacts, have counteracted a singular focus on mass measurements. These inherent problems may warrant some alternative approaches to characterizing emissions, using methods related to mass and impacts of emissions that can be more accurately defined. The present study provides a comparison and relative precision of several alternative mass measurement methods employed to measure the mass emissions of particulate matter from diesel engines with low and ultra-low levels of emissions. The methods of measurement reviewed in this study include two gravimetrically based methods, a chemically reconstructed mass method, and an integrated particle size distribution (IPSD) method. The mass measurements were consistent at low emission levels but the chemical speciation and IPSD methods achieved closer agreement and were more precise at ultra-low emission levels. Although mass measurement is a NIST-traceable quantity, alternative methods may present a new paradigm that better characterizes engine emissions in an atmospherically relevant manner.
1. INTRODUCTION
Gravimetric analysis has been the standard method for measuring diesel particulate matter (PM) mass emissions (CitationCode of Federal Regulations 2006; European Parliament Directive 2005). As diesel engines evolve, however, PM mass emissions decrease, rendering PM mass difficult to measure through gravimetric methods. Diesel Particulate Filters (DPFs) present an increased challenge for gravimetric analysis as they further decrease PM mass emissions (CitationLiu et al. 2003; CitationWarner et al. 2003). The United States Environmental Protection Agency (U.S. EPA) has developed 40 CFR Part 1065, which addresses detectability issues associated with ultra-low PM emissions used for engine certification (CitationCode of Federal Regulations 2005). The method includes specifications for weighing room conditions, buoyancy corrections, balance performance, electrostatic charge, and filter handling. In Europe, other sensitive methods such as particle counting have attracted interest. The UN/ECE PMP has developed method protocols and coordinated round-robin validation testing. Preliminary results indicate that detection for particle number counting methods exceeds gravimetric measurements by a factor of 20, even with the exclusion of nuclei mode particles smaller than 23 nm (GRPE-PMP-15–2 2005). Other studies have also shown that implementation of aftertreatment systems, such as DPFs, alters emissions compositions (CitationLev-on et al. 2002; CitationLiu et al. 2008a). In comparison to the high-PM emissions of older engines, the ultra-low PM emissions of modern engines have been shown to exhibit a less stable composition (CitationBirch and Cary 1996; CitationKleeman et al. 2000; CitationLiu et al. 2008b). The solid fraction of PM in ultra-low emissions is largely filtered by the DPF, leaving an increased organic fraction and yielding a relative enrichment in semi-volatile organic matter. The volatility of organic particles determines the probability that a compound exists in the gas or particle phase. Understanding particle volatility can improve the determination of the chemistry and phase-partitioning of diesel PM species (CitationHuffman et al. 2009). The partitioning of volatile and semi-volatile organics is sensitive to a variety of factors including temperature, vapor pressure, availability of competing adsorbates and residence time, making mass emissions unstable (CitationAbdul-Khalek et al. 1999). Filter-based measurement of ultra-low emissions (ULE) is further complicated by filter face velocity, dilution air quality, flow-rate variation, tunnel blank method, large background-to-emissions ratio, and filter adsorption issues (CitationCadle and Malawa 1990; CitationSchauer et al. 1999a). Adsorption of semi-volatile material onto the filter is difficult to measure because it is counteracted by volatilization of semi-volatile material from the filter (CitationFitz 1990) and penetration of accumulation-mode particles through the filter, especially during initial soot loading stage and for substrates with a high porosity (CitationLiu et al. 2003, Citation2005). The presumption that decreasing emissions levels in conjunction with variable sampling conditions have made gravimetric mass measurement difficult is confirmed by the lack of repeatability among different labs and among different experiments within the same lab. Additionally, zero- and negative-gravimetric mass measurements are not rare with modern engine emissions, indicating the need for alternative methods of particulate mass emissions measurements.
Previous PM speciation studies have revealed that both inorganic and organic species, including elemental carbon (EC), metallic ash, inorganic ions, and particulate organic matter (OM), constitute the majority of PM mass emissions of heavy-duty diesel engines (CitationSchauer et al. 1999b; CitationLev-on et al. 2002; CitationStetter et al. 2005). Assuming most engine emissions are comprised of similar constituents, the mass of each may be summed together to formulate a chemically reconstructed mass (CitationKweon et al. 2002; CitationZielinska et al. 2004; CitationSchauer et al. 2008). Measuring the EC and organic carbon (OC) fractions of low concentrations of PM is possible through the thermal optical method (TOM), a NIOSH-based measurement (CitationBirch and Cary 1996; CitationWarner et al. 2003; CitationKweon et al. 2003). Trace metals and inorganic ions can also be measured chemically via inductively coupled plasma mass spectroscopy and ion chromatography, respectively.
PM emissions can further be characterized by particle sizing using mechanisms such as differential electrical mobility and low-pressure impaction, reducing the problems associated with filter collection (CitationZhang and McMurry 1987; CitationMaricq et al. 2006). A particle sizer continuously collects detailed information about the size distribution and number concentration of particles. The particle number data may be converted to mass data using a density distribution, which in turn can be integrated to calculate the total mass of particles emitted during a single test; this method of measurement will be called Integrated Particle Size Distribution (IPSD).
The present study assesses traditional gravimetric and alternative methods of mass emissions rate measurement from diesel engines. These methods and their reproducibility are compared for low emissions from a 2004 engine without a DPF and ultra-low emissions from a 2007 engine with a DPF. The mass measurement methods include: a chemically reconstructed method based upon PM composition, an integrated particle size distribution method using data obtained from a particle size spectrometer, the EPA gravimetric mass method for 2004 engine certification, and an improved gravimetric method for ultra-low emissions which is consistent with EPA 40 CFR Part 1065. Results from at least three replicate runs for each engine configuration are compared to evaluate the precision and sensitivity of each measurement method. The non-gravimetric methods examined in this article are not intended as a substitute for CFR Part 1065, but may present a new paradigm that may characterize engine emissions in an atmospherically relevant manner.
2. EXPERIMENTAL METHODS
2.1. Test Equipment and Procedures
Two commercial heavy-duty diesel engines, A, a 2004-model and B, a 2007-model, were tested. Both engines employed exhaust gas recirculation (EGR) in order to reduce emissions of nitrogen oxides (NOX). Engine A was tested due to its known capacity to produce low levels of particulate mass emissions in compliance with 2004 EPA regulations. Engine B was tested due to its known capacity to produce ultra-low levels of PM mass emissions in compliance with 2007 EPA regulations. The fuel used for the two engines had a sulfur content of 308.5 ppm and 9.2 ppm, respectively. Both engines initially underwent a two-hour warming period performed at steady-state ISO mode 8 (CitationReciprocating internal combustion engines 1996). They were then operated under the Federal Test Procedure (FTP) in order to assess mass measurement methods under transient engine conditions. The FTP is a 1200-second cycle which simulates typical heavy-duty diesel on-road engine operating conditions through three different driving phases: New York Non-Freeway, Los Angeles Freeway, and Los Angeles Non-Freeway (CitationCode of Federal Regulations 2001). In an attempt to achieve detection limits of each method, Engine B was operated under three FTP cycles per sample collection. Sample collection was repeated at least three times for each engine configuration so that measurement variance and precision could be determined.
The DPF system employed with Engine B consists of two stages: a diesel oxidation catalyst (DOC) followed by a catalyzed soot filter (CSF). The CSF has performed with a PM filtration efficiency of greater than 90% (CitationChatterjee et al. 2001; CitationWarner et al. 2003; CitationLiu et al. 2003). The DOC is a flow-through catalyst which oxidizes much of the soluble organic fraction and converts NO to NO2 to assist regeneration (CitationJohnson and Kittelson 1994). The conversion of NO into NO2 can also occur in the CSF. Regeneration is a secondary combustion event necessary for removing the soot layer that creates an increased pressure drop across the CSF (CitationSuresh et al. 2000). During testing with Engine B, separate data were collected for non-regeneration and regeneration conditions. Sharp pressure drops and temperature increases, measured by sensors, indicated the onset of regeneration. Regeneration was also confirmed by comparing pressure and temperature data to pre-established computer profiles.
Samples for both the ultra-low emissions gravimetric method and chemical reconstruction method were collected using a source dilution sampling system consisting of a primary dilution tunnel, a secondary-micro diluter, a residence time chamber (RTC), and multiple sampling trains (CitationLiu et al. 2008b). The exhaust gas from the engine was piped into a critical flow venturi-constant volume system (CFV-CVS) tunnel where it was mixed with pre-, activated carbon-, and HEPA-filtered air at a rate of 1.298 m3/s. The dilution ratio of filtered air-to-exhaust ranged from about 4:1 to 7:1 in the CFV-CVS tunnel. A partial sample was isokinetically extracted from the primary dilution tunnel into the secondary-micro diluter where PM-, moisture-, and hydrocarbon-filtered air was added for secondary dilution. From the secondary-micro diluter, the diluted exhaust flowed into the residence time chamber which was calibrated to have 30 s of residence time to age and uniformly distribute the sample. Several ports are affixed to the sampling section of the RTC to allow for the simultaneous collection of multiple samples. Each of the ports contains a probe which is inserted into the RTC and oriented in the direction of flow to ensure isokinetic and isoaxial sampling. Each sampling train includes a PM2.5 cyclone to pre-classify PM emissions. Samples were collected using 47 mm baked quartz filters, polytetrafluoroethylene (PTFE) membrane filters, polyurethane foam (PUF) cartridges, XAD cartridges, and thermal desorption tubes. Samples for the IPSD method were taken from the CFV-CVS tunnel to an ageing chamber and were further diluted by two micro diluters before being scanned by a TSI Model 3090 Engine Exhaust Particle Sizer (EEPS). The dilution ratio following the CFV-CVS tunnel was calculated to be 185:1 using the CO2 and NOX concentrations from raw and diluted exhaust samples. For the pre-2007 gravimetric method, the sample passed through the CFV-CVS tunnel to a secondary-micro diluter manufactured by Horiba Instruments Inc and was collected on 70 mm Teflon impregnated glass fiber (TIGF) filters.
2.2. Measurement Methods
The first method of mass measurement was gravimetric-based and was consistent with the EPA pre-2007 method. This method utilized TIGF filters which were conditioned and then weighed in a temperature- and humidity-controlled room before and after filter loading. The second method, the ultra-low emissions (ULE) method, was also gravimetric and involved the use of PTFE membrane filters. Each filter set was conditioned and then weighed before and after filter loading in a temperature-, humidity-, draft-, and electrostatically controlled weighing room. Both gravimetric methods utilized a microbalance and the weighing room for the ULE method additionally included a robotic filter-handling apparatus. The robotic filter-handling apparatus improves measurements by minimizing vibrations and movements, providing additional stability, and removing human and handling errors.
The third mass measurement method is based upon a chemical reconstruction of the total mass emissions, calculated by adding the mass of each of the primary PM constituents, including EC, trace metals, inorganic ions, and particulate OM (CitationSchauer et al. 1999b). Quantification of the primary PM constituents was performed by the Wisconsin State Laboratory of Hygiene using a variety of established chemical analysis methods. The elemental and organic carbons were analyzed using a thermal optical method based upon the work of CitationBirch and Cary (1996). In brief, each quartz filter sample was heated in a helium atmosphere which evolved the OC present within the sample and then formed CO2 through oxidation, reduced to methane, and quantified by a flame ionization detector. The quartz filter samples were then heated a second time in a helium and oxygen atmosphere to remove any remaining carbon from the filter. The final EC mass was determined after applying a correction for pyrolysis carbon formed during prior analysis. From the OC measurement, the total OM mass was calculated by correcting for non-absorbed gaseous OC through the use of two filters in series as well as a correction for oxygen, hydrogen, and nitrogen organic components (CitationKolodziej et al. 2007). The OC uncertainty was determined by the formula 0.05 *massOC + 0.2 and the range of values of uncertainty used in this article was 1.7–17.8 μg/filter. In addition, teflon filter sets were collected and analyzed for trace metals, semi-metals, and inorganic ions. Trace metals and semi-metals were analyzed by inductively coupled plasma mass spectroscopy after the particulate matter samples were digested using a microwave digestion with a mixture of hydrochloric, hydrofluoric, and nitric acids. Another set of teflon filters was extracted in water and inorganic ions were quantified using ion chromatography (CitationSchauer et al. 2006). Blank substrates were collected to account for contamination from dilution air, background, handling, storage, and transportation during all tests.
The final mass measurement method, Integrated Particle Size Distribution method, implemented a TSI model 3090 Engine Exhaust Particle Size spectrometer which was described in detail by CitationJohnson et al. (2004). Particles passed through a charging region, joined particle-free sheath air, and flowed into a column containing an axial high-voltage central rod with 22 electrode rings forming the outer wall. The charged particles were deflected radially outward, separated by their differential electrical mobility, and collected on the electrode rings. Electrical mobility increases as particle size decreases, thus small particles were deflected to the electrode rings near the top of the column while large particles were deflected outward further downstream. The particle number concentration was then determined by the electrical current collected on the series of electrode rings. For the present study, the EEPS was set to scan particles ranging from 5.6 to 560 nm in diameter across 16 channels per decade every second. The minimum detection limit of the EEPS reflects the RMS noise, while the maximum detection limit correlates with the highest tolerance for electrical current. Since the total amount of charge depends on the particle size, both the maximum and minimum detection limits vary with particle diameter. The minimum detection rate additionally changes with averaging time. For example, the minimum detection limit for the particle sizes examined in this article varies approximately from 240 to 2.1 particles/cm3 for an averaging time of one second (CitationOperation and Service Manual 2006). In order to convert the particle size-resolved number distributions measured by the EEPS to a size-resolved mass distribution, it was necessary to select an appropriate density distribution.
shows the three size-resolved particle density correlations that were considered (CitationMaricq et al. 2000; CitationPark et al. 2003; CitationMaricq and Xu 2004). Other distributions are available, but the determination of density correlation accuracy is not within the scope of this article. The correlation from CitationMaricq and Xu (2004) was chosen for this study because it follows the general trend found in all three studies and after applying IPSD, the total mass matches closely with the ultra-low emissions gravimetric method measurements. Maricq et al.'s density distribution is defined in Equation (Equation1),
where ρ
is the effective density and Dp is the effective diameter of the particle. For the IPSD method, total mass,
, was calculated using Equation (Equation2
),
where i represents the particle size channel and ni is the total number of particles scanned in that channel.
shows the IPSD masses for particles with diameters greater than 5.6 nm, and greater than 50 nm in Engine A, using each of the density distributions. The IPSD masses derived from the three density distributions are within about 20% of the ULE gravimetric measurement for the low-emissions engine.
3. RESULTS AND DISCUSSION
3.1. Emissions Compositions in Old and Modern Engines
The composition of PM in old engines has been well studied and broadly understood. Technology advances and the use of aftertreatment in newer engines, however, alter the PM content.
, reproduced from CitationLiu et al. (2008a), shows that there is a nearly equal partitioning of the inorganic and organic species in the PM of high-PM-emitting engines, largely following findings of previous studies (CitationKleeman et al. 2000; CitationSchauer et al. 2003; CitationKittelson 1998).
FIG. 3 Chemical reconstruction analyzed from PM mass constituents of high-to-medium PM-emissions of heavy-duty diesel engines, from CitationLiu et al. (2008a).
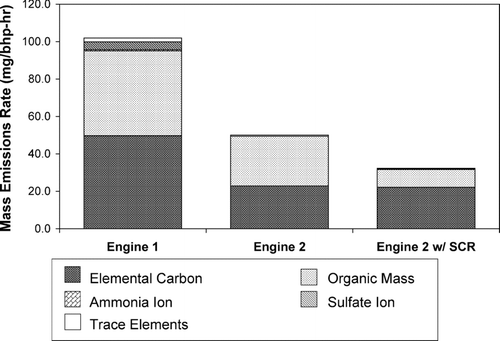
In contrast, the chemical speciation shown in shows that the compositions of ultra-low emissions are quite variable and the assumption that carbonaceous aerosol dominates the particulate matter mass is not reasonable. The very low mass collected on filters used to measure particulate matter emissions from ULE engines, along with the variable contribution of semi-volatile organic material to the particulate matter mass, leads to potentially large and variable artifacts for filter-based mass measurements (CitationStetter et al. 2005).
FIG. 4 Chemical reconstruction analyzed from PM mass constituents of ultra-low PM-emissions of heavy-duty diesel engines, from CitationLiu et al. (2008a).
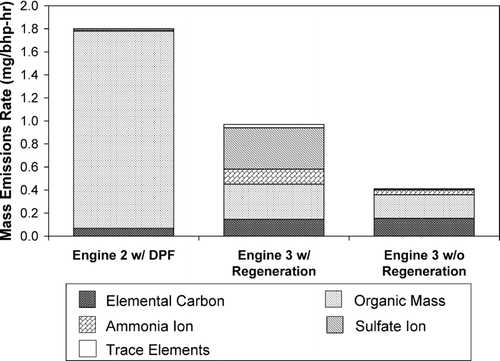
In addition to chemical composition variation, particle size composition varies in ultra-low emissions, especially comparing regeneration to non-regeneration. Kittelson defined that the nuclei mode includes particles with diameters smaller than 50 nm and the accumulation mode includes particles with diameters ranging from 50–1000 nm (1998), although he has recently redefined the nuclei mode to include only particles with diameters smaller than 30 nm (2002). The nuclei mode is thought to be composed of volatile organic and sulfur compounds formed by homogeneous and heterogeneous nucleation during cooling and dilution whereas the accumulation mode primarily consists of carbonaceous aggregates and nuclei-mode particles that enlarge by adsorbing sufficient quantities of volatile material.
shows the distribution of particle mass associated with PM size, applying both the 30 nm and 50 nm definitions of nuclei-mode particles, for each engine configuration. The accumulation mode is prevalent in both low and ultra-low non-regeneration emissions, consistent with previous findings that diesel engine-out PM mass emissions are mainly composed of accumulation-mode particles (CitationKleeman, Schauer, and Cass 2000). For the ultra-low non-regeneration emissions, the dominance of the accumulation mode may be due to the penetration of accumulation-mode particles in the Greenfield Gap, where the particles are too large for diffusion to be effective and too small for impaction or interception to be effective. Because these competing mechanisms operate in different size ranges, there will exist a particle size, usually in the range of 50–500 nm, that is responsible for the minimum efficiency of each filter (CitationHinds 1982). Regeneration induces a second combustion event in which soot particles are oxidized in the DPF and some volatile compounds pass through and nucleate to form particles downstream of the DPF. Additional organic mass and sulfate ions observed during regeneration of the ULE engine () may contribute to the increase of nuclei-mode particles, as shown in . Because nuclei-mode particles contain volatile materials, the appropriation of nuclei-mode particles measured by the EEPS into either PM mass emissions or artifacts should be investigated for the application of the IPSD method.
3.2. Gravimetric Method Comparison
shows the masses measured by gravimetric methods for each engine configuration. The first method is the pre-2007 method which utilizes two 70 mm-diameter TIGF filters in series and a corresponding sampling flowrate of 3.78 L/s. The second method is the ultra-low emissions method which utilizes two 47 mm diameter PTFE membrane filters in series with a flowrate of 0.28 L/s. Although the flowrates are substantially different, the two methods compare well for Engine A after tunnel-blank corrections were made. Ideally, the second method should offer improved precision and sensitivity, because the experimental techniques are better defined and more rigorous; for example, the exhaust is more thoroughly mixed and diluted to ambient conditions, the room used for measurement is highly conditioned and filter weighing is conducted by a robotic arm. Since the secondary dilution air for the second method is more highly filtered (through a HEPA filter, water separator, and gaseous adsorber), the second method has less dilution air blank variability. In the case of Engine A, an improvement for the ULE method can be observed in , where the standard error decreased from 1.50 to 1.31 mg/bhp-hr. However, the instability associated with DPF regeneration of Engine B counteracted this effect, as shown by the relatively large and approximately equal error bars of 0.40 and 0.34 mg/bhp-hr. For the same engine without regeneration, both gravimetric methods detected insignificant quantities and produced large error bars. For the pre-2007 method, the error bar remained below the reference value on the graph. Due to these difficulties associated with the gravimetric measurements, the methods discussed in the following sections may provide additional tools for the research community to characterize PM emissions from ultra low-emissions engines.
3.3. Chemically Reconstructed Mass Measurement Methods
displays a comparison among the three different chemical mass reconstructions and uses the ultra-low emissions gravimetric method as a reference. The three compositions of reconstruction each include EC, trace metals, and ions. Each one varies in its OM content, correcting OC with a factor of 1.2, 1.4, and 1.6, respectively. The OM is normally corrected with a 1.2 factor for vehicle emissions to incorporate atoms that are not measured during OC analyzation (CitationKleeman et al. 2000; CitationKweon et al. 2003). Although some have suggested that a 1.4 factor is the lowest reasonable estimate and a 1.6 factor appears more accurate for urban aerosol (CitationTurpin and Lim 2001), CitationBae et al. (2006) indicated that vehicle emissions have an OM-to-OC ratio closer to 1.2 as they contain much less oxygenated organics as compared to atmospheric particulate matter. shows that the OM component with the 1.2 factor correlates best with the gravimetric measurement for the first two engine configurations, while Engine B without regeneration provided the least conclusive results. In this configuration, the gravimetric method produced an almost unperceivable measurement on and contained the largest variance of the Engine B data. The decision to use the 1.2 factor was clearly justified by the other two configurations, and also agrees with the recommendations of CitationBae et al. (2006).
3.4. Integrated Particle Size Distribution Method
provides a comparison of four different mass emissions rates calculated from IPSD measurements, each with different particle size inclusions. Although issues exist when converting particle size distributions to mass, this measure was necessary in order to directly compare the four different methods. Mass represented the only available metric for comparison, since gravimetric measurements cannot be converted into chemical speciation or particle size distributions. Ultra-low emissions gravimetric method data is included for reference in . The four IPSDs integrate particles greater than 5.6 nm (the smallest channel on the EEPS), greater than 23 nm (PMP cutoff size, GRPE-PMP-15-2 2005), greater than 30 nm (exclusion of nuclei-mode defined by CitationKittelson et al. 2002), and greater than 50 nm (exclusion of nuclei mode defined by CitationKittelson 1998). The range greater than 5.6 nm was shown as a comprehensive integration of all particles measured by the EEPS in this study. The ranges greater than 30 nm and 50 nm are displayed as integrations excluding nuclei-mode particles. The range greater than 23 nm is the range chosen in Europe for future particle number regulations (European Parliament Regulation 2007). The figure shows that all IPSDs agree fairly well at low emissions and ultra-low emissions without regeneration. The nuclei mode during regeneration, however, is variable. The IPSD including all particles greater than 5.6 nm has the largest deviation from the ultra-low emissions method. The discrepancies may be due to volatile materials in the nuclei mode.
FIG. 8 Total PM mass emissions throughout FTP cycles for Engines A, B with regeneration, and B without regeneration, as measured through integrated particle size distribution (IPSD). The integrated masses compare integrations of all particles greater than 5.6 nm, 23 nm, 30 nm, and 50 nm. The ultra-low emissions gravimetric method of mass measurement method is included as a reference.
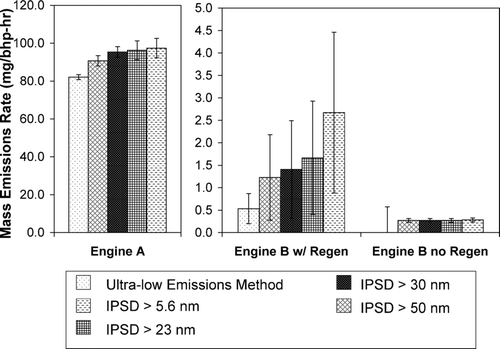
3.5. Method Comparison
shows the average PM mass emissions for all engine configurations over the FTP cycles, as measured by the pre-2007 method, the ultra-low emissions method, chemical reconstruction, and integrated particle size distribution. Several reasons explain the inclusion of only the particles larger than 50 nm for the IPSD in : this particle range achieved the closest agreement with the other measurement methods and largely resembled the other cutoff ranges of 5.6, 23, and 30 nm. Adding more particle ranges to the graph would also detract from readability. For the low PM emissions from Engine A, independent two-sample T-tests were performed for a 95% confidence interval on all of the possible method combinations. The results show that the ULE method agrees statistically with both chemical reconstruction and IPSD, while the pre-2007 method fails in those cases. When comparing Engine B to Engine A, the data reflects the greater instability associated with the regeneration conditions in the former case. For Engine B without regeneration, only the chemically reconstructed and particle size distribution methods produced detectable PM mass emissions. Background contamination has a more pronounced effect at ULE levels, thus emissions comprise a lower percentage of the total mass measured and become difficult to distinguish gravimetrically. Chemical reconstruction was likely capable of detection because it is less susceptible to the effects of temperature, humidity, and conditioning than gravimetric methods (CitationStetter et al. 2005). IPSD method was likely capable of detection because of its differential electrical mobility scanner and nearly instantaneous data collection. According to the T-tests, chemical reconstruction and IPSD had statistically similar measurements for all emissions.
3.6. Conclusions
As diesel engine PM emissions continue to decrease, it is vital for mass measurement technology to advance accordingly. Although gravimetric methods perform well at low emission levels, sensitivity concerns may exist at ultra-low emissions, where the mass-loading on the filter approaches the measurability limit. The more pronounced effect of background contamination also contributes to the increased uncertainty observed for ultra-low emissions. This study has indicated that chemical reconstruction and IPSD methods may offer more capability and sensitivity for such measurements. However, these methods are not proposed as alternatives to gravimetric measurements and assessing the relative merits of the gravimetric and non-gravimetric methods is premature. Chemical reconstruction and IPSD measurements were converted to mass only to facilitate comparisons between the four methods. Rather than replacing gravimetric methods, chemical reconstruction and IPSD may serve as a new paradigm to characterize ultra-low engine PM emissions in an atmospherically relevant manner.
Acknowledgments
The authors acknowledge Dr. Barbara Zielinska of the Desert Research Institute for her valuable discussions. We also acknowledge Devin Berg, Thaddeus Swor, Denise Ford, and Tom Wosikowski of Cummins Emission Solutions for their help with sample collection and data analysis as well as Chris Worley of the University of Wisconsin–Madison, Jeff DeMinter, Steve Strebel, and Steve Geis of the Wisconsin State Laboratory of Hygiene for their help with the chemical analysis.
REFERENCES
- Abdul-Khalek I. S.Kittelson D. B.Brear F.The Influence of Dilution Conditions on Diesel Exhaust Particle Size Distribution Measurements1999 SAE Technical Paper No. 1999-01-1142
- Bae M. S.Schauer J. J.Turner J. R.Estimation of the Monthly Average Ratios of Organic Mass to Organic Carbon for Fine Particulate Matter at an Urban SiteAerosol Sci. Technol.200640121123–1139
- Birch M. E.Cary R. A.Elemental Carbon-Based Method for Monitoring Occupational Exposures to Particulate Diesel ExhaustAerosol Sci. Technol.199625221–241
- Cadle S. H.Mulawa P. A.Atmospheric Carbonaceous Species Measurement Methods Comparison Study: General Motors ResultsAerosol Sci. Technol.199012128–141
- Chatterjee S.McDonald C.Conway R.Windawi H.Vertin K.LeTavec C. A.Clark N. N.Gautam M.Emissions Reductions and Operational Experiences with Heavy Duty Diesel Fleet Vehicles Retrofitted with Continuously Regenerated Diesel Particulate Filters in Southern California2001 SAE Technical Paper No. 2001 01-0512
- Code of Federal RegulationsProtection of the Environment, Title 40, Parts 80 and 86U.S. Government Printing OfficeWashington2001
- Code of Federal RegulationsProtection of the Environment, Title 40, Part 1065U.S. Government Printing OfficeWashington2005
- Code of Federal RegulationsProtection of the Environment, Title 40, Parts 80 and 86U.S. Government Printing OfficeWashington2006
- European Parliament and of the Council of 28 September 2005 Directive 2005/55/EC
- European Parliament and of the Council of 20 June 2007 Regulation (EC) No. 715/2007
- Fitz D. R.Reduction of the Positive Organic Artifact on Quartz FiltersAerosol Sci. Technol199012142–148
- GRPE-PMP-15-2The 15th PMP Informal MeetingGeneva Switzerland2005
- Hinds W. C.Aerosol Technology: Properties, Behavior, and Measurement of Airborne ParticlesJohn Wiley & SonsNew York1982
- Huffman J. A.Docherty K. S.Mohr C.Cubison M. J.Ulbrich I. M.Ziemann P. J.Onasch T. B.Jimenez J. L.Chemically-Resolved Volatility Measurements of Organic Aerosol from Different SourcesEnviron. Sci. Technol2009 ASAP paper
- Johnson J. E.Kittelson D. B.Physical Factors Affecting Hydrocarbon Oxidation in a Diesel Oxidation Catalyst1994 SAE Technical Paper No. 94-1771
- Johnson T.Caldow R.Pocher A.Mirme A.Kittelson D. B.A New Electrical Mobility Particle Sizer Spectrometer for Engine Exhaust Particle Measurements2004 SAE Technical Paper No. 2004-01-1341
- Kittelson D. B.Engines and Nanoparticles: A ReviewJ. Aerosol Sci.199829575–588
- Kittelson D. B.Watts W. F.Johnson J. H.Diesel Aerosol Sampling MethodologyCoordinating Research Council2002E–43
- Kleeman M. J.Schauer J. J.Cass G. R.Size and Composition Distribution of Fine Particulate Matter Emitted from Motor VehiclesEnviron. Sci. Technol2000 34(7):1132–1142
- Kolodziej C.Wirojsakunchai E.Foster D. E.Schmidt N.Kamimoto T.Kawai T.Akard M.Yoshimura T.Comprehensive Characterization of Particulate Emissions from Advanced Diesel Combustion2007 SAE Technical Paper No. 2007-01-1945
- Kweon C.Foster D. E.Schauer J. J.Okada S.Detailed Chemical Composition and Particle Size Assessment of Diesel Engine Exhaust2002 SAE Technical Paper No. 2002-01-2670
- Kweon C.Okada S.Foster D. E.Bae M. S.Schauer J. J.Effect of Engine Operating Conditions on Particle-Phase Organic Compounds in Engine Exhaust of a Heavy-Duty Direct Injection (D.I.) Diesel Engine2003 SAE Technical Paper No. 2003-01-0342
- Lev-on M.LeTavec C.Uihlein J.Kimura K.Alleman T. L.Lawson D. R.Vertin K.Gautam M.Thompson G. J.Wayne S.Clark N.Okamoto R.Rieger P.Yee G.Zielinska B.Sagebiel J.Chatterjee S.Hallstrom K.Speciation of Organic Compounds from the Exhaust of Trucks and Buses: Effect of Fuel and After-Treatment on Vehicle Emission Profiles2002 SAE Technical Paper No. 2002-01-2873
- Liu Z. G.Skemp M. D.Lincoln J. C.Diesel Particulate Filters: Trends and Implications of Particle Size Distribution Measurement2003 SAE Technical Paper No. 2003-01-0046
- Liu Z. G.Thurow E. M.Caldow R.Johnson T. R.Transient Performance of Diesel Particulate Filters as Measured by an Engine Exhaust Particle Size Spectrometer2005 SAE Technical Paper No. 2005-01-0185
- Liu Z. G.Berg D.Schauer J. J.An Analysis of Methods for Measuring Particulate Matter Mass Emissions2008a SAE Technical Paper No. 2008-01-1748
- Liu Z. G.Swor T. A.Schauer J. J.Debilzen J.Severance C. A.A Source Dilution Sampling System for Characterization of Engine Emissions under Transient or Steady-State OperationAerosol Sci. Technol2008b42270–280
- Maricq M. M.Podsiadlik D. H.Chase R. E.Size Distributions of Motor Vehicle Exhaust PM: A Comparison between ELPI and SMPS MeasurementsAerosol Sci. Technol200033239–260
- Maricq M. M.Xu N.The Effective Density and Fractal Dimension of Soot Particles from Premixed Flames and Motor Vehicle ExhaustJ. Aerosol Sci.2004351251–1274
- Maricq M. M.Xu N.Chase R. E.Measuring Particulate Mass Emissions with the Electrical Low Pressure ImpactorAerosol Sci. Technol20064068–79
- Operation and Service Manual for Model 3090 Engine Exhaust Particle Sizer Spectrometer 2006 P/N 1980494. TSI Incorporated
- Park K.Feng C.Kittelson D. B.McMurry P. H.Relationship between Particle Mass and Mobility for Diesel Exhaust ParticlesEnviron. Sci. Technol200337577–583
- Reciprocating Internal Combustion Engines—Exhaust Emission Measurement Part 4: Test Cycles for Different Engine Applications International Organization for StandardizationGeneva1996 ISO 8178-4
- Schauer J. J.Kleeman M. J.Cass G. R.Simoneit B. R.Measurement of Emissions from Air Pollution Sources. 1. C1 to C29 Organic Compounds from Meat CharbroilingEnviron. Sci. Technol1999a331566–1577
- Schauer J. J.Kleeman M. J.Cass G. R.Simoneit B. R.Measurement of Emissions from Air Pollution Sources. 2. C1 to C30 Organic Compounds from Medium Duty Diesel TrucksEnviron. Sci. Technol1999b331578–1587
- Schauer J. J.Mader B. T.Deminter J. T.Heidemann G.Bae M. S.Seinfeld J. H.Flagan R. C.Cary R. A.Smith D.Huebert B. J.Bertram T.Howeel S.Kline J. T.Quinn P.Bates T.Turpin B.Lim H. J.Yu J. Z.Yang H.Keywood M. D.ACE-Asia Intercomparison of Thermal-optical Method for the Determination of Particle Phase Organic and Elemental CarbonEnviron. Sci. Technol200337993–1001
- Schauer J. J.Lough G. C.Shafer M. M.Christensen W. F.Arndy M. F.DeMinter J. T.Park J. S.Characterization of Metals Emitted from Motor VehiclesResearch Report 133. Health Effects InstituteBoston2006
- Schauer J. J.Christensen C. G.Kittelson D. B.Johnson J. P.Watts W. F.Impact of Ambient Temperatures and Driving Conditions on the Chemical Composition of Particulate Matter Emissions from Non-Smoking Gasoline-Powered Motor VehiclesAerosol Sci. Technol2008 42(3):210–23
- Stetter J. C.Foster D. E.Schauer J. J.Modern Diesel Particulate Matter Measurements and the Application of Lessons Learned to 2007 Levels and Beyond2005 SAE Technical Paper No. 2005-01-0194
- Suresh A.Khan A.Johnson J. H.An Experimental and Modeling Study of Cordierite Traps—Pressure Drop and Permeability of Clean and Particulate Loaded Traps2000 SAE Technical Paper No. 2000-01-0476
- Turpin B. J.Lim H. J.Species Contributions to PM2.5 Mass Concentrations: Revisiting Common Assumptions for Estimating Organic MassAerosol Sci. Technol.2001351602–610
- Warner J. R.Johnson J. H.Bagley S. T.Huynh C.Effects of a Catalyzed Particulate Filter on Emissions from a Diesel Engine: Chemical Characterization Data and Particulate Emissions Measured with Thermal Optical and Gravimetric Methods2003 SAE Technical Paper No. 2003-01-0049
- Zhang X. Q.McMurry P. H.Theoretical Analysis of Evaporative Losses from Impactor and Filter DepositsAtmos. Environ.19872181779–1789
- Zielinska B.Sagebiel J.McDonald J. D.Whitney K.Lawson D. R.Emission Rates and Comparative Chemical Composition from Selected in-use Diesel and Gasoline-Fueled VehiclesJ. Air & Waste Manage. Assoc.2004541138–1150