Abstract
In this study we analyzed the sampling time effect on the overall performance of seven portable impactors (SMA MicroPortable, BioCulture, Microflow, MAS-100, Millipore Air Tester, SAS Super 180, and RCS High Flow) as well as a stationary impactor (BioStage) when collecting airborne bacteria and fungi from 2 to 30 minutes indoors and outdoors. Their performance was compared against the reference BioStage impactor collecting samples for 2 minutes. When bacteria were collected outdoors, the average concentration ratio of all test samplers relative to the reference sampler was 0.64, but it decreased to 0.04 when bacteria were collected for 2 min first and subsequently exposed to particle-free air for 28 min (30 min total sampling time). For fungi, the average concentration ratio decreased from 0.35 to 0.03 under the same conditions. When the test impactors sampled particle-free air first and then collected bioaerosols outdoors for 2 min, the concentration ratio for bacteria averaged for all test samplers decreased from 0.77 (agar plates not exposed to particle-free air and particles collected for 2 min) to 0.04 (agar plates exposed to particle-free air for 28 min first and then particles collected for 2 min). For fungi, the relative recovery averaged for all samplers decreased from 0.45 to 0.03 under the same conditions. The effects of sampling time and impactor model were statistically significant for all experimental conditions. Thus, the data obtained in this study suggest that when impactors are used for the collection of airborne bacteria and fungi, sampling times should be as short as reasonably possible to minimize the under-representation of airborne microorganism concentration which could be a factor of 10 or higher for prolonged sampling times.
INTRODUCTION
Exposure to airborne biological agents, such as bacteria and fungi, may result in a wide range of respiratory and other health disorders in occupational and general populations (CitationDouwes et al. 2003). It was estimated that 250 million episodes per year of respiratory infection in the United States could be attributed to bioaerosol exposure (CitationCox and Wathes 1995). The relationship between the microbial contamination of air and negative health effects, including lung irritation, rhinitis, cough and asthma, has been reported in many studies (CitationBurge 1990; CitationSpengler et al. 1993; CitationKoskinen et al. 1995; CitationFung and Hughson 2003; CitationHerr et al. 2003; CitationFung and Hughson 2008).
Presence of bioaerosols in indoor and outdoor environments can be detected using a variety of available bioaerosol samplers that employ sampling mechanisms such as filtration, impaction, impingement and electrostatic precipitation. Among the available samplers, those employing the method of impaction seem to be among the most popular and they are used to collect airborne biological particles on agar plates for cultivation (CitationYao and Mainelis 2007a), glass slides for microscopy analysis (CitationKuhn et al. 2005), filter and aluminum foil for total carbon analysis (CitationBauer et al. 2008). The use of impactors to collect viable particles offers the advantage of directly collecting particles on agar plates for subsequent incubation and counting of formed colonies without the need for post-sampling processing as in the case of using impingers. In fact, the Andersen viable bioaerosol impactor (Graseby Andersen, Atlanta, GA) has long been regarded as a sampler of choice for enumerating viable airborne microorganisms (CitationJensen et al. 1992) and has been recommended and used as a reference sampler in several bioaerosol studies (CitationJones et al. 1985; CitationChatigny et al. 1989). In the past few years, portable (battery-operated) impactors have become popular for the collection of culturable bioaerosols. Such devices do not require heavy external pumps and feature high sampling flow rates. Portable impactors have been used in a number of studies to collect bacteria and fungi indoors and outdoors (CitationMehta et al. 1996; CitationMehta 2000; CitationBellin and Schillinger 2001; CitationAn et al. 2003). Our recent study investigated various performance parameters of seven portable impactors (SMA MicroPortable, BioCulture, Microflow, Microbiological Air Sampler (MAS-100), Millipore Air Tester, SAS Super 180, and RCS High Flow) when collecting polystyrene latex particles and biological particles in a controlled laboratory environment (CitationYao and Mainelis 2006a, Citation2006b, Citation2007b). The laboratory study found that the impactors' cut-off size and ability to collect and recover various species of bacteria and fungi could vary substantially depending on the impactor model. In fact, the impactors' cut-off size, or d 50, ranged from 1.2 to 7.0 μ m. In a field study involving the same portable impactors it was found that a majority of them underperformed compared to a BioStage impactor (SKC Inc., Eighty Four, PA), which is an equivalent to the Andersen N-6 viable impactor (CitationYao and Mainelis 2007a).
One of the performance characteristics of the commercially available portable impactors that has not been very widely explored is the sampling time. In general, it has been a common assumption that impactors' long sampling times lead to prolonged drying stress through dehydration of the collected microorganisms, which causes damage and inactivation (CitationHensel and Petzoldt 1995). The long sampling time could also lead to particle bounce due to desiccation of agar and substantial decrease in collection efficiency (CitationJuozaitis et al. 1994). Some studies use sampling times in the order of a few minutes (CitationJuozaitis et al. 1994; CitationFang et al. 2005; CitationKalogerakis et al. 2005; CitationYao and Mainelis 2007a), while other use sampling times as long as 60 min (CitationLi 1999). In high concentration environments sampling time could be limited to just a few seconds to avoid sample overload and masking bias (CitationChang 1995; CitationRinsoz et al. 2008). Potential decrease in impactors' performance due to extended sampling time also depends on the relative humidity of the sampled air and its ability to desiccate the agar medium and already collected microorganisms. The effect of humidity and sampling time has been offered as a potential explanation of some of the anomalies observed in ambient bioaerosol sampling (CitationNevalainen 1989). A laboratory study with sensitive bacteria Pseudomonas fluorescens found that total recovery of the bacteria at RH = 30% decreased from 1.5% to approximately 0.3% when sampling time was extended from 10 s to 30 min (CitationThompson et al. 1994). When the experiments were repeated at RH = 90%, total recovery of P. fluorescens had high variability and no substantial influence of sampling time was observed. Conversely, another laboratory study investigating collection of Escherichia coli and Bacillus subtilis bacteria by the Andersen 1-stage impactor, the MAS-100 portable impactor and the Burkard sampler concluded that sampling times < 40 min do not to significantly influence bacterial recoveries (CitationLi and Lin 1999).
The studies referenced above have been performed in laboratory environments with only a few selected organisms, while multiple microorganism species would be encountered in any field investigation. In addition, to the best of our knowledge there are virtually no data on the effect of sampling time and the performance of portable microbial impactors (except a study using MAS-100 as referenced above). Since the majority of available literature indicates that long sampling times would damage the collected organisms, the quantitative information on the optimal sampling time window would be useful for field professionals employing portable samplers.
Thus, as a continuation of our study into the performance of portable microbial impactors, here we analyzed whether the sampling time affects the overall performance of seven portable impactors mentioned above when collecting airborne bacteria and fungi from 2 to 30 min indoors and outdoors. In addition, to better ascertain the factors responsible for likely decrease in the number of recovered colonies with prolonged sampling times, we separately investigated the effects of desiccation damage to the already collected microorganisms as well as the effect of agar desiccation prior to collecting the microbial particles. Since an Andersen impactor is often regarded as a sampler of choice and is used in many investigations, a one-stage Andersen impactor (N-6)—equivalent microbial impactor was also included in our study and operated alongside the portable impactors. A second such impactor served as a reference and its sampling time was limited to two minutes.
MATERIALS AND METHODS
Microbial Samplers
The experiments were performed with seven portable impactors and two Andersen (N-6)—equivalent impactors. All of the used impactors collect microorganisms directly on agar which is then incubated to determine the number of formed colony forming units (CFUs). The battery-operated portable impactors included BioCulture (A.P. BUCK Inc., Orlando, FL), MAS-100 (EMD Chemicals, Inc., Gibbstown, NJ), Microflow (Aquaria srl, Lacchiarella, Italy), Millipore Air Tester (Millipore Corp., Billerica, MA), RCS High Flow (Biotest Diagnostics Corp., Denville, NJ), SAS Super 180 (Bioscience International, Inc., Rockville, MD), and SMA MicroPortable (Veltek Associates, Inc, Phoenixville, PA). The impactors' sampling flow rates, number of impaction nozzles, amount of agar used and cut-off sizes are presented in .
TABLE 1 Physical characteristics of tested portable microbial samplers
The investigation of cut-off sizes of these impactors as well as of the factors affecting their performance when sampling bacteria and fungi under controlled laboratory conditions have been described in earlier studies (CitationYao and Mainelis 2006a; CitationYao and Mainelis 2006b). The amount of agar used to operate each impactor was the same as used in earlier studies and reflects either a manufacturer's recommendations or was determined to yield lower cut-off sizes in those earlier studies. In addition to the portable impactors, we used two one-stage Andersen impactor (N-6)—equivalent microbial samplers: BioStage impactors (SKC Inc., Eighty Four, PA). One of them served as a reference sampler, while the other was operated in parallel to the portable impactors. The BioStage impactor is a replicate of the last stage of Andersen six-stage impactor, which has a cutoff size of 0.65 μ m (CitationWüst et al. 2003), and was used with 50 mL agar. The Andersen viable bioaerosol impactor (Graseby Andersen, Atlanta, GA) has been recommended and used as a reference sampler in several bioaerosol studies (CitationChatigny et al. 1989) and thus its equivalent was included as a reference sampler.
Sampling Protocol and Determination of Samplers' Performance
Since it is not completely clear whether the desiccation of already collected microorganisms or desiccation of agar prior to collecting the microorganisms is a more substantial causative factor for a decrease in sample CFUs, the sampling procedures were divided into three separate protocols.
Protocol A
The bacteria and fungi present indoors were collected with all test impactors operating simultaneously and collecting samples for different sampling periods: t S = 2, 5, 10, and 30 min. During all four different sampling periods, the reference BioStage impactor collected samples for t S = 2 min only. Overall, nine replicates over several days for both bacteria and fungi were collected for each sampling time. The relative humidity during sampling corresponded to comfortable room conditions, ≈ 45–50%. The collected samples were incubated at a room temperature and the colony forming units (CFUs) were counted after 24, 48, and 72 h. The room temperature (∼ 20–22°C) was selected because many environmental bacteria prefer temperatures from 20 to 30°C (CitationOtten and Burge 1999) and room temperature allows isolation of most fungi (CitationBurge and Otten 1999). The final number of CFUs was adjusted for positive-hole correction (except for the RCS High Flow) and fungal/bacterial concentrations were calculated as CFUs/m3. Since the test impactors feature different numbers of collection nozzles, a separate Excel sheet with correction factors was developed for each sampler based on the probability analysis (CitationFeller 1968). The calculated factors were verified against correction tables available for some samplers. Tryptic soy agar (TSA) (Becton Dickinson Microbiology Systems, Cockeysville, MD) was used for collection of bacteria, while malt extract agar (MEA) (Becton Dickinson Microbiology Systems) was used to collect fungi. Broad-spectrum media such as non-selective TSA agar supports growth of bacteria, while MEA supports the general detection of fungi (CitationBurge and Otten 1999; CitationOtten and Burge 1999; CitationReponen 2001). Since some fungi usually grow on TSA agar and some bacteria on MEA agar, when counting CFUs only bacteria were counted on TSA agar and only fungi on MEA agar. To determine the effect of sampling time on the performance of each sampler, the Concentration Ratio A, CR_A(t S , i), was determined as
Protocol B
Protocol B was designed to investigate the effect of desiccation on already collected microorganisms. Here, the seven portable samplers and two BioStage impactors were operated in parallel outdoors. Each test sampler i and the reference sampler collected bacteria or fungi simultaneously for t COLL = 2 min, then were transferred indoors and operated in a particle-free environment for t S * = t S –t COLL , where t S = 2, 5, 10, and 30 min. Thus, the minimum exposure to particle-free air was 0 min and maximum 28 min. The reference sampler was operated for t COLL = 2 min outdoors only. The particle free environment was ensured by operating the impactors inside a Class II biosafety cabinet (Nuaire Inc., Plymouth, MN) with air circulation “ON,” while the temperature and relative humidity corresponded to comfortable room conditions. The agar types, incubation conditions and counting procedures were the same as in the protocol A. The effect of microorganism desiccation was determined as the Concentration Ratio B, CR_B:
Protocol C
Protocol C was designed to investigate the effect of desiccating agar prior to the collection of the microorganisms. Here, the seven portable samplers and a test BioStage impactor were operated simultaneously in a particle-free environment first for t S * = t S –t COLL , where t S = 2, 5, 10, and 30 min and t COLL = 2 min. The particle-free environment was the same as in the protocol B. After sampling the particle-free air, the samplers were transferred outdoors and simultaneously with the reference sampler collected samples for t COLL = 2 min. The relative humidity outdoors during the sampling days ranged from 42 to 69%. Thus, before collecting the actual samples, the minimum exposure to particle-free air was 0 min and maximum 28 min. The reference sampler exposed to particle-free air. The agar types, incubation conditions and counting procedures were the same as in the protocol A. The effect of agar desiccation was determined as the Concentration Ratio C, CR_C:
STATISTICAL ANALYSIS
Two-way ANOVA tests were performed to analyze the Concentration Ratios (CR_A, CR_B, and CR_C) as a function of sampler model and sampling time. Analysis ToolPack available as an add-in for Microsoft Office Excel was used for this purpose. The p < 0.05 indicated statistical significance in all tests. The data in , , , , , represent averages and standard deviations from nine repeats for each sampler and each sampling time.
FIG. 1 Relative performance of portable impactors as a function of sampling time, t S , when sampling bacteria indoors. The data represent averages and standard deviations from nine repeats.
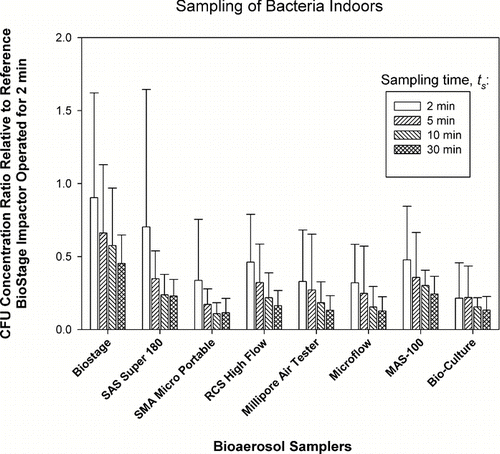
FIG. 2 Relative performance of portable impactors as a function of sampling time, t S , when sampling fungi indoors. The data represent averages and standard deviations from nine repeats.
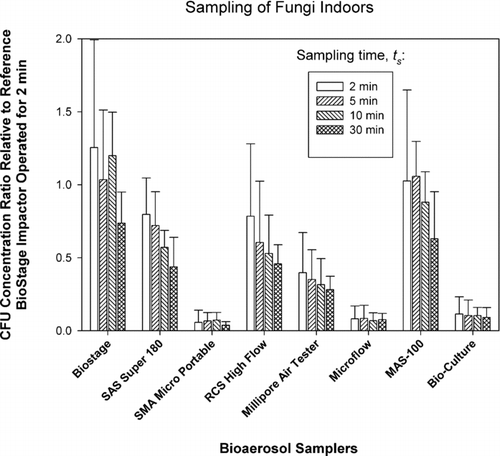
FIG. 3 Relative performance of portable impactors as a function of sampling time, t S , when sampling bacteria outdoors. The test samplers sampled outdoor air for t COLL = 2 min and then sampled particle-free air for t S −t COLL , where t S = 2, 5, 10, and 30 min. The data represent averages and standard deviations from nine repeats.
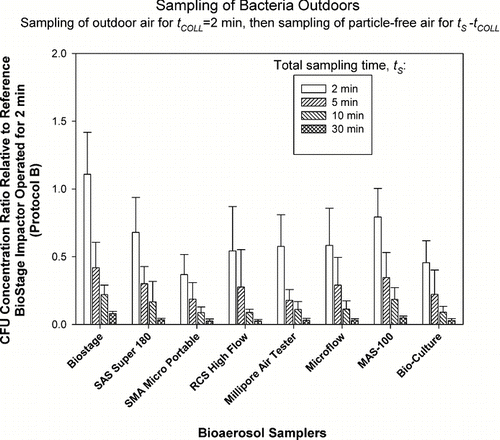
FIG. 4 Relative performance of portable impactors as a function of sampling time, t S , when sampling fungi outdoors. The test samplers sampled outdoor air for t COLL = 2 min and then sampled particle-free air for t S −t COLL , where t S = 2, 5, 10, and 30 min. The data represent averages and standard deviations from nine repeats.
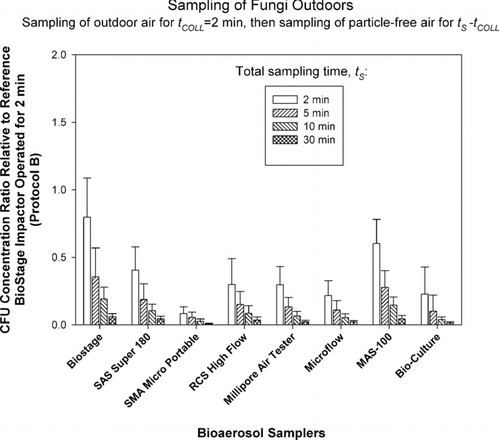
FIG. 5 Relative performance of portable impactors as a function of sampling time, t S , when sampling bacteria outdoors. The test samplers sampled particle-free air for t S − 2 min (t S = 2, 5, 10, and 30 min) and then sampled outdoor air for t COLL = 2 min. The data represent averages and standard deviations from nine repeats.
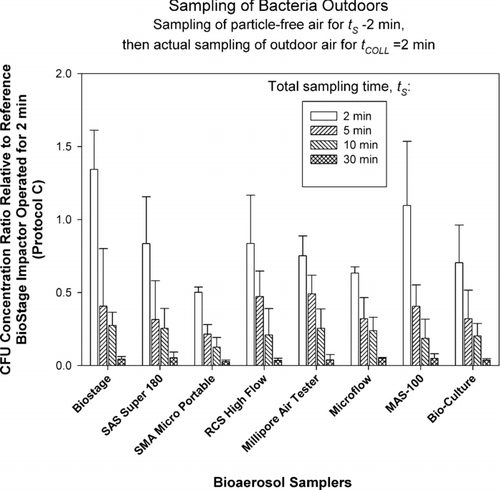
FIG. 6 Relative performance of portable impactors as a function of sampling time, t S , when sampling fungi outdoors. The test samplers sampled particle-free air for t S − 2 min (t S = 2, 5, 10, and 30 min) and then sampled outdoor air for t COLL = 2 min. The data represent averages and standard deviations from nine repeats.
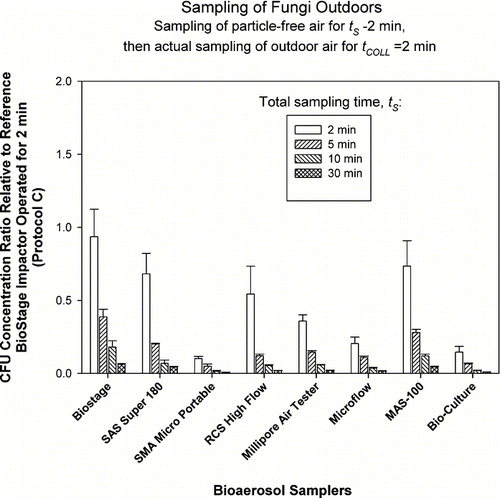
RESULTS AND DISCUSSION
The indoor concentration ratios for bacteria and fungi are presented in and . Here, the test samplers were operated for different sampling times and the obtained microorganism concentrations were compared to those measured by the reference BioStage sampler operated for t s = 2 min (protocol A). The indoor bacterial and fungal concentrations measured by the reference BioStage impactor was generally below 200 CFU/m3.
For the sampling of bacteria and sampling time t s = 2 min, the test BioStage impactor had the highest average Concentration Ratio of 0.9, while the SAS-180 had an average ratio of 0.7. The average Concentration Ratios of all test samplers gradually decreased as the sampling time increased. In fact, the average Concentration Ratio averaged for all samplers sampling bacteria for t s = 2 min was 0.45 and gradually decreased to 0.2 as sampling time increased to 30 min. When sampling fungi for t s = 2 min, the test BioStage impactor had the highest Concentration Ratio of 1.25 with MAS-100 being second highest with the Concentration Ratio of 1.02. The same ratios for the SAS-180 and RCS High Flow were approximately 0.8, and for the Millipore Air Tester 0.4. These ratios gradually declined as the sampling time increased. Somewhat surprising was very low concentration ratios exhibited by the SMA Micro Portable, the Microflow and the BioCulture samplers. However, it is consistent with their underperformance compared to other samplers (CitationYao and Mainelis 2007a). One can also see that the error bars are relatively high in . Most likely it was caused by the relatively low bacterial concentration indoors causing variability in the number of collected bacteria and the resulting Concentration Ratios. The average Concentration Ratio of all test samplers sampling fungi for t s = 2 min was 0.55 and gradually decreased to 0.35 as the sampling time increased to 30 min. For both the sampling of bacteria and fungi, the effect of sampling time t s and the impactor model were statistically significant (p < 0.0001). This result does indicate that the recovery of airborne bacteria and fungi by microbial impactors decreases as the sampling time increases.
In the next set of experiments (protocol B), we examined the possible effects of desiccation on the already collected microorganisms as the sampling continues. The results for outdoor sampling of bacteria and fungi are presented in and , respectively.
Here the microorganisms were collected outdoors by each sampler for t COLL = 2 min and then exposed to particle-free air for 0, 3, 8, and 28 min. According to the reference sampler, the outdoor bacterial concentrations ranged from 200 to 2,000 CFU/m3, while fungal concentrations ranged from 600 to 8,000 CFU/m3. As could be clearly seen from and , Concentration Ratios steadily and consistently declined for each sampler and for both bacteria and fungi as we went from no desiccation (t s = 2 min, t s −t COLL = 0 min) to 28 min of exposure to particle-clean air (t s = 30 min, t s −t COLL = 28 min). In fact, the Concentration Ratio for bacteria averaged for all test samplers decreased from 0.64 at t s = 2 min to 0.04 at t s = 30 min. For fungi, the situation was similar and the Concentration Ratio averaged for all samplers decreased from 0.35 to 0.03 as t s was increased from 2 min to 30 min. Two-way ANOVA analysis for bacteria and fungi indicated that that both the effect of sampling time t s and the impactor model were statistically significant (p < 0.0005). In fact, the p-values for time effect were extremely low: p < 10−39. The results obtained here clearly indicate that once microbial particles are collected, they are prone to damage as the sampling continues and they are exposed to air flow. It has been suggested that the damage and inactivation are likely due to dehydration (CitationHensel and Petzoldt 1995). If the exposure to particle-free air continues for 28 min, their recovery can be reduced by a factor as high as 10. Even short exposure of 3 min can reduce their recovery by a factor of 2.
In the third set of experiments (protocol C), we investigated the recovery of microorganisms when collection media is subjected to desiccation prior to sampling the organisms. The results for outdoor sampling of bacteria and fungi are presented in and , respectively.
Here, each of the test samplers collected particle-free air for t S * = t s −t COLL (t COLL = 2 min) resulting in particle-free air sampling of 0, 3, 8, and 28 min before collecting bacterial or fungal samples outdoors for t COLL = 2 min. The reference sampler collected microorganisms only for t COLL = 2 min and was not exposed to particle-free air. According to the reference sampler, outdoor bacterial concentrations ranged from 200 to 1,000 CFU/m3, while fungal concentrations were approximately 3,000 CFU/m3. As could be clearly seen from and , the Concentration Ratios steadily and consistently declined for each sampler and for each microorganism type as exposure of collection media to particle-free air was increased from 0 min to 28 min prior to sampling the organisms. The Concentration Ratio for bacteria averaged for all test samplers decreased from 0.77 at t s = 2 min to 0.04 at t s = 30 min. For fungi, the situation was similar and the Concentration Ratio averaged for all test samplers decreased from 0.45 to 0.03 as t s was increased from 2 min to 30 min. Two-way ANOVA analysis for bacteria and fungi indicated that that both the effect of sampling time t s and the impactor model were statistically significant (p < 0.05). The p-values for time effect were very low: p < 10–17 . The results obtained here clearly indicate that if collection media (area under the impaction nozzles) is exposed to particle-free air prior to collecting the microorganisms, the microorganism recovery could be greatly reduced. Sampling of particle- free air for 3 min can reduce the recovery of bacteria and fungi by a factor of 2–3. If the exposure to particle-free air is increased to 28 min prior to sampling the organisms, the recovery could be reduced by a factor close to 20 compared to no-exposure condition. The likely reason for such a dramatic decrease in microorganism recovery is the desiccation and hardening of agar under the impaction jet which results in particle bounce and reduced collection efficiency as suggested by earlier studies (CitationJuozaitis et al. 1994). Even if the microorganism does not bounce off and is deposited on agar it would have an impeded access to nutrients due to hardened agar surface.
The bacterial concentration ratios for outdoor samples ( and ) seem to have less sampler-to-sampler variability compared to the fungal results outdoors ( and ), especially for the 2 min samples. The lowest initial concentration ratios (2 min sampling) for fungi are observed for the samplers that have the highest d 50: SMA Microportable, Microflow and BioCulture. It is possible that due to the presence of specific bioaerosol sources the bacteria were attached to larger particles and thus were collected relatively efficiently even by the samplers that have high cut-off sizes. Fungal spores, on the other hand, were mostly present as single spores and were collected less efficiently.
The data presented in our study clearly indicate that recovery of airborne microorganisms collected using portable impactors as well as traditional stationary impactors decreases as the sampling time increases. Once the organisms are deposited onto the collection media they are exposed to air flow over them as the sampling continues causing their dehydration and inactivation. Although a particle hitting a moist agar surface will generally adhere (CitationWilleke and Macher 1999), once the area under the impaction nozzle is exposed to air prior to depositing the organisms it becomes desiccated and hardened resulting in a microorganism bounce or reduced access to nutrients. The bounce of bacteria and fungi from hard surfaces has been observed in other studies (CitationGrinshpun 1997; CitationTrunov 2001). In fact, visual observation of the collection media after prolonged exposure to particle-free air revealed clear indentations under the sample nozzles. These indentations result not only in a harder surface, but also in an increased jet-to-plate distance which results in decreased collection efficiency (CitationYao and Mainelis 2006b). The extent of damage to the organisms would also depend on the relative humidity of the air sampled (CitationThompson et al. 1994). Therefore, the data obtained in this study for different sampling times should not be used for their absolute value as sampling circumstances and environmental conditions may differ from investigation to investigation, but as an indication that prolonged sampling times would decrease the culturability of the collected microorganisms which would negatively affect the accuracy of a microbial investigation.
It could also be noted that the extent of microorganism inactivation due to prolonged sampling times was more pronounced when organisms were collected outdoors and agar was exposed to particle-free air before or after sampling (protocols B and C) compared to sampling for different times indoors (protocol A). It is most likely due to low microorganism concentration indoors and its temporal variation which obscured the effect of sampling time. It is also possible that different microorganism composition indoors and outdoors could have contributed to their differences in susceptibility to desiccation. Nonetheless, even for protocol A, the effect of both sampling time and sampler model was statistically significant.
Overall, the results of this study are consistent with those of earlier studies indicating that longer sampling times would result in a decreased microorganism recovery as indicated by CitationJuozaitis et al. (1994) and CitationHensel and Petzoldt (1995). The data obtained here indicate that the sampling time should be as short as reasonably possible to avoid the reduced microorganism recovery. Our observation is different from the laboratory study by CitationLi and Lin (1999) who indicated that sampling times up to 40 min did not have a significant effect of microorganism recoveries. The difference in outcomes could be explained by the differences in experimental protocols and microorganisms used. CitationLi and Lin (1999) investigated B. subtilis and E. coli bacteria in a laboratory study. B. subtilis is a hardy organism (CitationSneath 1986) resistant to many adverse conditions and probably was not affected by desiccation due sampling times of 40 min or less. E. coli on the other hand is a sensitive organism (CitationAn et al. 2006) and was most likely affected by the desiccation over prolonged sampling periods. However, the reference value in the CitationLi and Lin (1999) study was the concentration of colonies in the Collison nebulizer suspension. When sensitive microorganisms are recirculated in a Collison nebulizer (20 mL of liquid recirculated about every 6 seconds (CitationMay 1973) and subjected to shear stress as their aerosolization continues, the amount of injury increases and their viability decreases. This phenomenon was demonstrated with sensitive bacteria of P. fluorescens in other studies (CitationReponen et al. 1997; CitationMainelis et al. 2005). Since the culturability of both the reference bacteria and the test airborne bacteria decreases, the effect of prolonged sampling time becomes obscured.
CONCLUSIONS
The data obtained in this study show that for all testing protocols, the effect of microbial impactor model and sampling time were statistically significant. It has also been shown that both the desiccation of the collection media and the desiccation of the already collected organisms play a role in a decreased microorganism recovery with increased sampling time. The results of our investigation suggest that when impactors are used for the collection of airborne bacteria and fungi, sampling times should be kept as short as reasonably possible to minimize under-representation of airborne microorganism concentration.
Acknowledgments
This publication was supported by the CDC/NIOSH grant DHHS-CDC1-K01-OH008029. Its contents are solely the responsibility of the authors and do not necessarily represent the official view of the sponsors. The technical assistance by EMD Chemicals, Inc. (Gibbstown, NJ), A.P. BUCK Inc. (Orlando, FL), Veltek Associates, Inc. (Phoenixville, PA), Bioscience International, Inc., (Rockville, MD), Millipore Corp., (Billerica, MA), and Biotest Diagnostics Corp., (Denville, NJ) is appreciated.
REFERENCES
- An , H. R. , Mainelis , G. and White , L. 2006 . Development and Calibration of Real-time PCR for Quantification of Airborne Microorganisms in Air Samples . Atmos. Environ. , 40 : 7924 – 7939 .
- An , H. R. , Mainelis , G. and Yao , M. 2003 . Evaluation of a High Volume Portable Bioaerosol Sampler in Laboratory and Field Environments . Indoor Air , 14 ( 6 ) : 385 – 393 .
- Bauer , H. , Schueller , E. , Weinke , G. , Berger , A. , Hitzenberger , R. , Marr , I. L. and Puxbaum , H. 2008 . Significant Contributions of Fungal Spores to the Organic Carbon and to the Aerosol Mass Balance of the Urban Atmospheric Aerosol . Atmos. Environ. , 42 ( 22 ) : 5542 – 5549 .
- Bellin , P. and Schillinger , J. 2001 . Comparison of Field Performance of the Andersen N6 Single Stage and the SAS Sampler for Airborne Fungal Propagules . Indoor Air , 11 ( 1 ) : 65 – 68 .
- Burge , H. 1990 . Bioaerosols: Prevalence and Health Effects in the Indoor Environment . J. Allergy. Clin. Immunol. , 86 ( 5 ) : 687 – 701 .
- Burge , H. A. and Otten , J. A. 1999 . “ Fungi ” . In Bioaerosols: Assessment and Control , Edited by: Macher , J. M. 322 Cincinnati, OH : American Conference of Governmental Industrial Hygenists .
- Chang , C.-W. , Grinshpun , S. A. , Willeke , K. , Macher , J. M. , Donnelly , J. , Clark , S. and Juozaitis , A. 1995 . Factors Affecting Microbiological Colony Count Accuracy for Bioaerosol Sampling and Analysis . Am. Ind. Hyg. Assoc. J. , 56 : 979 – 986 .
- Chatigny , M. A. , Macher , J. M. , Burge , H. A. and Solomon , W. R. 1989 . “ Sampling Airborne Microorganisms and Aeroallergens ” . In Air Sampling Instruments for Evaluation of Atmospheric Contaminants , Edited by: Hering , S. V. 199 – 220 . Cincinnati : ACGIH .
- Cox , C. S. and Wathes , C. M. 1995 . Bioaerosols Handbook , Boca Raton : CRC. Lewis Publishers .
- Douwes , J. , Thorne , P. , Pearce , N. and Heederik , D. 2003 . Bioaerosol Health Effects and Exposure Assessment: Progress and Prospects . Annals of Occupational Hygiene , 47 ( 3 ) : 187 – 200 .
- Fang , Z. , Ouyang , Z. , Hu , L. , Wang , X. , Zheng , H. and Lin , X. 2005 . Culturable Airborne Fungi in Outdoor Environments in Beijing, China . Sci. Total Environ. , 350 ( 1–3 ) : 47 – 58 .
- Feller , W. 1968 . An Introduction to the Probability Theory and Its Application , New York : John Wiley and Sons .
- Fung , F. and Hughson , W. G. 2003 . Health Effects of Indoor Fungal Bioaerosol Exposure . Appl. Occ. & Environ. Hygiene , 18 ( 7 ) : 535 – 544 .
- Fung , F. and Hughson , W. G. 2008 . The Fundamentals of Mold-Related Illness: When to Suspect the Environment is Making a Patient Sick . Postgraduate Medicine , 120 ( 1 ) : 80 – 84 .
- Grinshpun , S. A. , Willeke , K. , Ulevicius , V. , Juozaitis , A. , Terzieva , S. , Donnelly , J. , Stelma , G. N. and Brenner , K. P. 1997 . Effect of Impaction, Bounce and Reaerosolization on the Collection Efficiency of Impingers . Aerosol Sci. Technol. , 26 : 326 – 342 .
- Hensel , A. and Petzoldt , K. 1995 . “ Biological and Biochemical Analysis of Bacteria and Viruses ” . In Bioaerosols Handbook , Edited by: Cox , C. S. and Wathes , C. M. New York : Lewis Publishers .
- Herr , C. E. W. , zur Nieden , A. , Jankofsky , M. , Stilianakis , N. I. , Boedeker , R. H. and Eikmann , T. F. 2003 . Effects of Bioaerosol Polluted Outdoor Air on Airways of Residents: A Cross Sectional Study . Occup. Environ. Med. , 60 : 336 – 342 .
- Jensen , P. A. , Todd , W. F. , Davis , G. N. and Scarpino , P. V. 1992 . Evaluation of Eight Bioaerosol Samplers Challenged with Aerosols of Free Bacteria . Am. Ind. Hyg. Assoc. J. , 53 ( 10 ) : 660 – 667 .
- Jones , W. , Morring , K. , Morey , P. and Sorenson , W. 1985 . Evaluation of the Andersen Viable Impactor for Single Stage Sampling . Amer. Industrial Hygiene Association J. , 46 ( 5 ) : 294 – 298 .
- Juozaitis , A. , Willeke , K. , Grinshpun , S. A. and Donnelly , J. 1994 . Impaction onto a Glass Slide or Agar versus Impingement into a Liquid for the Collection and Recovery of Airborne Microorganisms . Appl. Environ. Microbiol. , 60 ( 3 ) : 861 – 870 .
- Kalogerakis , N. , Paschali , D. , Lekaditis , V. , Pantidou , A. , Eleftheriadis , K. and Lazaridis , M. 2005 . Indoor Air Quality-Bioaerosol Measurements in Domestic and Office Premises . J. Aerosol Sci. , 36 ( 5–6 ) : 751 – 761 .
- Koskinen , O. , Husman , T. , Hyvärinen , A. , Reponen , T. and Ruuskanen , J. A., N. 1995 . Respiratory Symptoms and Infections among Children in Day Care Centers with Mold Problems . Indoor Air , 5 : 3 – 9 .
- Kuhn , R. C. , Mingyi , T. W. , Hofer , V. , Lee , M. and Russell , N. S. 2005 . Prevalence and Airborne Spore Levels of Stachybotrys spp. in 200 Houses with Water Incursions in Houston, Texas . Canadian J. Microbiol. , 51 : 25 – 28 .
- Li , C.-S. 1999 . Sampling Performance of Impactors for Bacterial Bioaerosols . Taylor & Francis , 30 : 280 – 287 .
- Li , C.-S. and Lin , Y.-C. 1999 . Sampling Performance of Impactors for Bacterial Bioaerosols . Aerosol Sci. Technol. , 30 ( 3 ) : 280 – 287 .
- Mainelis , G. , Berry , D. , An , H. R. , Yao , M. S. , DeVoe , K. , Fennell , D. E. and Jaeger , R. 2005 . Design and Performance of a Single–Pass Bubbling Bioaerosol Generator . Atmos. Environ. , 39 ( 19 ) : 3521 – 3533 .
- May , K. R. 1973 . The Collison Nebulizer: Description, Performance and Application . J. Aerosol Sci. , 4 : 235 – 243 .
- Mehta , S. K. , Bell-Robinson , D. M. , Groves , T. O. , Stetzenbach , L. D. and Pierson , D. L. 2000 . Evaluation of Portable Air Samplers for Monitoring Airborne Culturable Bacteria . American Industrial Hygiene Association Journal , 61 : 850 – 854 .
- Mehta , S. K. , Mishra , S. K. and Pierson , D. L. 1996 . Evaluation of Three Portable Samplers for Monitoring Airborne Fung . Applied and Environ. Microbiology , 62 ( 5 ) : 1835 – 1838 .
- Nevalainen , A. 1989 . Bacterial Aerosols in Indoor Air , Ph.D. Dissertation Kuopio, Finland : National Public Health Institute .
- Otten , J. A. and Burge , H. A. 1999 . “ Bacteria ” . In Bioaerosols: Assessment and Control , Edited by: Macher , J. M. ACGIH .
- Reponen , T. , Willeke , K. , Ulevicius , V. , Grinshpun , S. A. and Donnelly , J. 1997 . Techniques for Dispersion of Microorganisms into Air . Aerosol Sci. Technol. , 27 : 405 – 421 .
- Reponen , T. , Willeke , K. , Grinshpun , S. and Nevalainen , A. 2001 . “ Biological Particle Sampling ” . In Aerosol Measurement: Principles, Techniques and Applications , Edited by: Baron , P. A. and Willeke , K. 751 – 778 . New York : Willey-Interscience .
- Rinsoz , T. , Duquenne , P. , Greff-Mirguet , G. and Oppliger , A. 2008 . Application of Real-Time PCR for Total Airborne Bacterial Assessment: Comparison with Epifluorescence Microscopy and Culture-Dependent Methods . Atmos. Environ. , 42 ( 28 ) : 6767 – 6774 .
- Sneath , P. H. A. 1986 . “ Endospore-Forming Gram-Positive Rods and Cocci ” . In Bergey's Manual of Systematic Bacteriology , Edited by: Sneath , P. H. A. , Mair , N. S. , Sharpe , M. E. and Holt , J. G. Vol. 2 , 1104 – 1139 . Baltimore : Williams and Wilkins .
- Spengler , J. , Neas , L. , Nakai , S. , Dockery , D. , Speizer , F. , Ware , J. and Raizenne , M. 1993 . Respiratory Symptoms and Housing Characteristics . Indoor Air 93 , 1 : 165 – 170 .
- Thompson , M. W. , Donnelly , J. , Grinshpun , S. A. , Juozaitis , A. and Willeke , K. 1994 . Method and Test System for Evaluation of Bioaerosol Samplers . J. Aerosol Sci. , 25 ( 8 ) : 1579 – 1593 .
- Trunov , M. , Trakumas , S. , Willeke , K. and Grinshpun , S. A. 2001 . Collection of Bioaerosol Particles by Impaction: Effect of Fungal Spore Agglomeration and Bounce . Aerosol Sci. Technol. , 35 : 617 – 624 .
- Willeke , K. and Macher , J. M. 1999 . “ Air Sampling ” . In Bioaerosols: Assessment and Control Edited by: Macher , J. M. 322 American Conference of Governmental Industrial Hygenists, Cincinnati, OH
- Wüst , G. , Friedl , H. , Haas , D. , Kock , M. , Pichler-Semmelrock , F. , Reinthaler , F. F. , Schlacher , R. and Marth , E. 2003 . A Comparison between Andersen (ACFM) and Reuter Centrifugal Sampler (RCS-Plus) for Indoor Sampling of Airborne Molds . Aerobiologia , 19 : 125 – 128 .
- Yao , M. and Mainelis , G. 2006a . Effect of Physical and Biological Parameters on Enumeration of Bioaerosols by Portable Microbial Impactors . J. Aerosol Sci. , 37 ( 11 ) : 1467 – 1483 .
- Yao , M. S. and Mainelis , G. 2006b . Investigation of Cut-Off Sizes and Collection Efficiencies of Portable Microbial Samplers . Aerosol Sci. Technol. , 40 ( 8 ) : 595 – 606 .
- Yao , M. S. and Mainelis , G. 2007a . Analysis of Portable Impactor Performance for Enumeration of Viable Bioaerosols . J. Occupat. Environ. Hygiene , 4 ( 7 ) : 514 – 524 .
- Yao , M. S. and Mainelis , G. 2007b . Use of Portable Microbial Samplers for Estimating Inhalation Exposure to Viable Biological Agents . J. Exposure Sci. Environ. Epidemiol. , 17 ( 1 ) : 31 – 38 .