Abstract
This study explores the influence of ethanol on particulate matter (PM) emissions from gasoline direct injection (GDI) vehicles, a technology introduced to improve fuel economy and lower CO2 emissions, but facing challenges to meet next-generation emissions standards. Because PM formation in GDI engines is sensitive to a number of operating parameters, two engine calibrations are examined to gauge the robustness of the results. As the ethanol level in gasoline increases from 0% to 20%, there is possibly a small (<20%) benefit in PM mass and particle number emissions, but this is within test variability. When the ethanol content increases to >30%, there is a statistically significant 30%–45% reduction in PM mass and number emissions observed for both engine calibrations. Particle size is unaffected by ethanol level. PM composition is primarily elemental carbon; the organic fraction increases from ∼5% for E0 to 15% for E45 fuel. Engine-out hydrocarbon and NOx emissions exhibit 10–20% decreases, consistent with oxygenated fuel additives. These results are discussed in the context of the changing commercial fuel and engine technology landscapes.
Copyright 2012 American Association for Aerosol Research
INTRODUCTION
Three areas related to motor vehicles and air quality are experiencing major changes. The first is fuel composition. Recent energy policy decisions, such as the 2007 Energy Independence and Security Act, mandate increased reliance on renewable fuels, directives to enhance national security and ameliorate climate change impacts (U.S. Environmental Protection Agency Citation2007). This implies increased blending of ethanol into conventional gasoline fuel. Roughly 90% of gasoline sold in the United States currently contains nearly 10% ethanol (E10) (U. S. Energy Information Administration Citation2011). This will increase following the United States Environmental Protection Agency (EPA) partial waiver to allow E15 fuel use in 2007+ model year vehicles (U. S. Environmental Protection Agency Citation2010).
The second is the growth of gasoline direct injection (GDI) engine technology, aimed to offer fuel economy and CO2 emissions benefits (Fraser et al. Citation2009; Yi et al. Citation2009). Direct injection of gasoline into the cylinder allows better combustion control, for example, multiple fuel injections and charge-air cooling. But it risks incomplete fuel volatilization and impingement onto piston and cylinder surfaces, exacerbating particulate matter (PM) emissions. The third is regulatory; California Air Resources Board (ARB) and EPA are both contemplating next-generation emissions standards which would lower tailpipe PM emissions from 10 mg/mi to 6 mg/mi, and then 3 mg/mi, over the next decade (California Air Resources Board Citation2010).
Consequently, it is important to examine the interplay and potential synergies between fuel composition and engine technology in efforts to reduce emissions. There are ongoing investigations of ethanol's effects on fuel systems, evaporative emissions, and gaseous emissions (Durbin et al. Citation2007; Kar and Cheng Citation2009; Knoll et al. Citation2009; Coordinating Research Council Citation2011), but few gasoline engine studies have examined its impact on PM emissions. The paucity of data is presumably because stoichiometric combustion in spark ignition engines naturally produces very low PM emissions, a few milligrams per mile (Maricq et al. Citation1999), and because GDI is a new technology. One exception is the effort by Aikawa et al. (Citation2010) to create a PM index based on fuel properties, which is of interest for GDI because of the potential to help model air fuel mixing and sooting propensity.
Ethanol effects on GDI particulate emissions have been reported by Storey et al. (Citation2010) and He et al. (Citation2010), who observed reductions of about 30% for E20 fuel over the Federal Test Procedure (FTP) drive cycle. However, the detailed characterizations, such as particle number, size, and composition, were undertaken at steady-state engine operation, whereas cold and hot starts and transients are typically of more interest for gasoline engines. Work by Chen et al. (Citation2010) showed that PM emissions can either increase or decrease with ethanol content depending on fuel injection timing. Such results point out a difficulty in investigating potential fuel benefits, namely that these might be masked by adjustments in engine calibration when the fuel is changed. Other properties of fuel besides ethanol content can also impact PM emissions; thus, Khalek et al. (Citation2010) noted higher PM levels from a GDI vehicle operated on a commercial E10 fuel relative to two E0 fuels, but attributed this to a higher volatility in the base gasoline.
The goal of this paper is to examine how ethanol–gasoline blends impact PM emissions from GDI vehicles. Six fuels are examined, ranging from E0 (base gasoline) to E45 (45% ethanol). The study utilizes the FTP drive cycle to include the important effects of cold start and transient operation. It addresses measurement variability both by repeat tests and the use of three metrics of PM emissions: mass, number, and elemental/organic carbon composition. The issue of sensitivity to engine parameters is handled in two ways: First, we conduct testing at two different engine calibrations to assess the consistency of ethanol's impact on emissions. Second, we compare the vehicle exhaust results to observations from a study of ethanol–gasoline blend diffusion flames (Maricq Citation2011).
EXPERIMENTAL METHODS
Test Vehicle and Fuels
The test vehicle is a light-duty truck equipped with a 3.5-L V6 gasoline turbocharged direct injection engine. It is representative of current GDI products, but contains prototype elements, such as the engine calibrations tested here. It has a compression ratio of 9.8:1 and independent variable cam timing. The fuel injectors are side-mounted and deliver single-fuel pulses, except for split injection (two pulses) during crank and early cold start operation. Exhaust aftertreatment consists of a three-way catalyst to control hydrocarbon (HC) and NOx emissions.
The study uses four fuels: certification test gasoline (E0), a commercial E10 fuel similar to that expected for future certification, a commercial pump grade E10, and a commercial E100 fuel used for blending. Their properties are listed in . E100 and E0 were splash-blended to produce the E17, E32, and E45 fuels. All fuels were analyzed by gas chromatography to verify ethanol content. Fuel changes were done by draining the tank, filling with new fuel, and running the vehicle through the FTP drive cycle prior to testing. Emissions were measured over the FTP cycle, consisting of three phases: (1) cold start, (2) urban, and (3) hot start. E0 tests were conducted first and last to confirm that no changes in vehicle emissions performance occurred.
TABLE 1 Fuel properties
PM Sampling and Measurement
The vehicle was tested on a 48-inch single roll, AC electric, chassis dynamometer. The experimental setup is illustrated in . Vehicle exhaust was sampled in two ways: (1) directly from the tailpipe and (2) through a full-flow constant volume sampling (CVS) dilution tunnel, as per the regulatory method (except to substitute quartz filter EC/OC analysis for Teflo filter gravimetric PM mass). In our CVS system, exhaust is diluted with a “remote mix T” connected to the tailpipe via a short (∼1 m) extension. The dilution air is filtered, temperature- and humidity-controlled (38°C and −9°C dew point), and actively regulated to maintain a constant total flow of exhaust plus dilution air. This was set to 9.34 m3/min for E0, E10, and E17 fuels, but raised to 19.8 m3/min for E32 and E45 because of increased exhaust humidity. The diluted exhaust travels via a ∼7-m, 25.4-cm-diameter, conductive coated Teflon tube to a 30.4-cm-diameter stainless steel tunnel.
FIG. 1 Schematic diagram of the experimental setup. Solid arrows show exhaust and diluent flows. Dashed arrow indicates diluted exhaust flow. (Color figure available online).
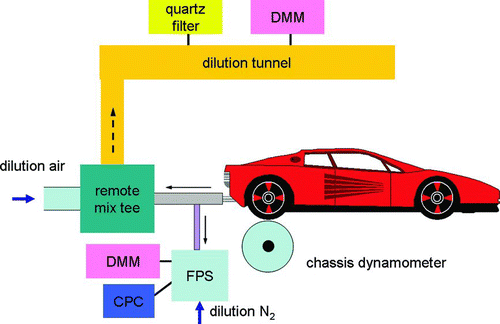
Direct tailpipe sampling employs a Dekati Fine Particle Sampler (FPS) originally developed to provide standardized dilution conditions for studying nucleation mode formation (Ntziachristos and Samaras Citation2010). It uses a coaxial perforated tube diluter that allows room temperature dilution, but avoids thermophoretic deposition of PM from hot exhaust. This approach contrasts with the European Union solid particle counting method, which is designed to remove nucleation mode particles by hot dilution and evaporation (Giechaskiel et al. Citation2008). Instead, the FPS samples both semivolatile and solid particles. It was used at a dilution factor of 25–30. A Dekati ejector pump provides 8.5 times secondary dilution for particle number counting. Room temperature nitrogen from liquid boil-off supplies the diluent for both the FPS and the ejector pumps.
Three PM emissions metrics are examined: (1) mass, (2) elemental/organic carbon (EC/OC), and (3) total particle number. Engine-out HC and NOx emissions are also reported. They are measured using Horiba analyzers based on flame ionization detection (FID) and chemiluminescence detection, respectively.
PM mass is determined by Dekati Mass Monitor (DMM) using a combination of electrical mobility and aerodynamic particle size measurements (Mamakos et al. Citation2006). Particles are charged in a corona discharge, segregated by mobility (D50 = 50 nm), and those penetrating the mobility classifier enter a cascade impactor. The resulting electrical currents and aerodynamic and mobility size information yield estimates for the quantity, volume, and effective density of particles, which are combined to calculate second-by-second PM mass concentration. Two DMMs were used, one at the tailpipe and the second at the CVS tunnel.
EC/OC particulate mass is determined by sampling diluted exhaust through prebaked 47-mm-diameter quartz filters, followed by thermal analysis with a Horiba MEXA 1370PM (Akard et al. Citation2004). The filters are heated to 980°C, first under nitrogen and then with oxygen present. CO2 from the oxidation of material evolved under nitrogen is equated with organic carbon, whereas that produced with oxygen is attributed to elemental carbon. The OC mass includes a correction for hydrogen content assuming an H/C ratio of 1.85. A correction is also made for gas phase adsorption, which amounts to about 0.5 mg/mi (Maricq et al. Citation2011). Unlike the IMPROVE and NIOSH methods (Chow et al. Citation2001), there is no correction for pyrolysis, which impacts interpretation of EC/OC values. But the total PM mass compares well with gravimetric data (Akard et al. Citation2004).
Total particle number concentration is measured via TSI 3010 CPC (condensation particle counter). The lower size cutoff, 50% count efficiency, is 12 nm. This is nearly a factor of two smaller than the 23-nm cutpoint adopted by the EU for their solid particle method. The CPC counting efficiency at 70 and 100 nm was calibrated by electrometer to 100%.
Many of the E0 and E10 tests included tailpipe PM measurements by an electrical low-pressure impactor (ELPI) (Keskinen et al. Citation1992). This is a cascade impactor that measures second-by-second aerodynamic size distributions by first charging the particles in a corona discharge and then recording the electrical currents from the impactor stages. Previous work has shown that analysis of diesel particulate matter ELPI data using a fractal-like effective density results in PM mass and geometric mean mobility diameter estimates in good agreement with gravimetric and scanning mobility particle sizer data (Maricq et al. Citation2006).
RESULTS
Four engine calibrations (engine computer control of fuel pressure, fuel injection and spark timing, etc.) were initially examined with E0 fuel and found to have FTP cycle-weighted average PM emissions in the range of 3–7 mg/mi. Two of these near the proposed 3 mg/mi LEV III standard were selected for further study, labeled A and B. These differ in that calibration A produces lower cold start but higher urban and hot start PM relative to calibration B. Three to four repeat tests were performed with calibration A for each fuel; whereas, one to three were conducted with calibration B. The calibrations were not altered between fuels, except to adjust the amount of fuel needed to maintain a stoichiometric air/fuel ratio. The two calibrations show similar PM emissions trends with ethanol level; therefore, calibration A data are presented next, whereas those for calibration B are included in Supplementary Information.
PM Mass and Number Emissions
illustrates tailpipe particle number emission rates over the cold and hot start FTP phases. Mass emissions (Figure S2 in Supplementary Information) exhibit a similar pattern.
FIG. 2 Transient particle number emissions from the GDI test vehicle for the cold and hot start phases of the FTP drive cycle. Data are recorded by direct tailpipe sampling. Top panel: E0 fuel. Bottom panel: E45 fuel. (Color figure available online).
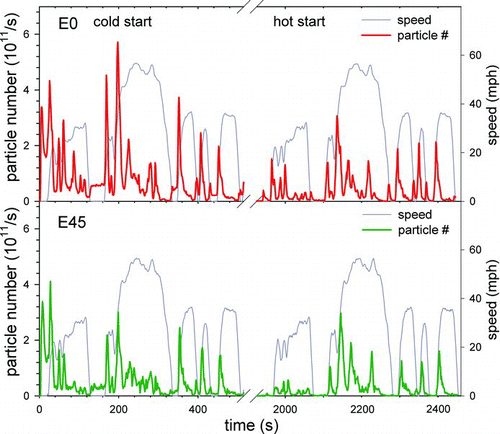
When measured at the tailpipe, the particle concentrations recorded by DMM or CPC are multiplied by the time-aligned exhaust flow volume to derive emissions rates. Concentrations recorded via CVS sampling are simply scaled by the dilution tunnel flow. Not surprisingly, PM emissions correlate with vehicle acceleration owing to the increased fueling. But one also observes smaller emissions peaks during decelerations, likely a consequence of fuel shut-off. Emissions with E45 fuel are consistently below those for E0, but the decrease is not uniform, as seen from the accentuated reduction in particle emissions at the beginning of the hot start.
The effect of ethanol on PM emissions is summarized by and S3. These portray five parallel measurements: (1) mass from the tailpipe DMM, (2) mass from CVS tunnel DMM, (3) EC/OC mass from CVS, (4) particle count at tailpipe, and (5) ELPI PM mass for a subset of tests. The 1σ error bars represent test-to-test variability. Differences between the five methods reflect measurement uncertainty. This includes both systematic and random effects, but the data scatter points to random noise as the major contributor at these low emissions levels. The variability between the five PM methods is comparable to test-to-test variability in any given method. No statistically significant differences are observed between direct tailpipe and CVS sampling.
FIG. 3 GDI vehicle exhaust particle number and mass emissions versus ethanol content of fuel. Emissions are measured over the three-phase FTP drive cycle using calibration A. Symbols = mass. Lines = particle number. E0 data recorded at the beginning and end of the study are distinguished by plotting them slightly below and above 0% ethanol, respectively. Error bars are 1σ of the test repeatability. (Color figure available online).
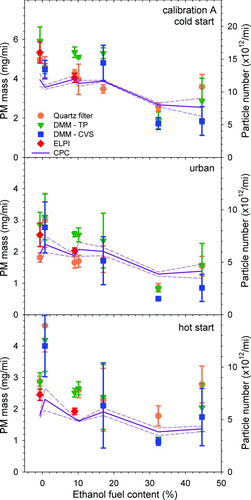
All three metrics indicate a statistically significant reduction in particulate emissions with E32 and E45 fuels compared to base gasoline, relative to the average measurement uncertainty of approximately ±0.7 mg/mi. The decrease from E0 to these fuels is on average ∼30% by particle number and ∼45% by mass. This distinction is likely within the uncertainty, but could also originate from differences in nuclei mode particle emissions. Since E85 fuel further reduces PM (not part of this study), the small increase from E32 to E45 is likely from vehicle variability.
and S3 suggest a small (∼20%) PM benefit for the lower ethanol blends relative to E0, but the data are mixed. Averaged over the parallel measurements, PM mass decreases 10–30% from E0 to E10 fuel using calibration A, but then remains constant from E10 to E17. For calibration B, there is a 10–20% PM increase from E0 to E10, but a ∼10% decrease from E0 to E17 fuel. However, the individual DMM and EC/OC data are not always consistent in their trends for the lower-ethanol blends, reflective of the difficulties in measuring PM at the ∼1 mg/mi level. Particle number measurements show a similar circa 20% improvement from E0 to E17 fuel. But even with somewhat lower variability than the PM mass data, this ∼20% falls within the overall measurement uncertainty.
FIG. 4 FTP engine-out (feedgas) total hydrocarbon and NOx emissions versus fuel ethanol content for calibration A. Initial and final E0 tests are distinguished as in . (Color figure available online).
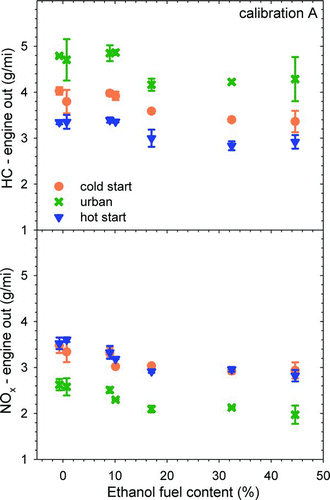
shows that engine-out HC and NOx emissions exhibit similar dependences on fuel ethanol content. The decreases are more modest, about 20%. For calibration A, they occur already for the E17 blend, but calibration B data in Figure S4 indicate the decreases to occur above E17. The HC decrease should be interpreted with caution. Adding ethanol to gasoline changes HC composition, increasing the proportion of alcohols and aldehydes. These compounds are less efficiently detected by FID, which by itself can lead to an apparent emissions reduction. Additional measurements to correct under-determination of these compounds were not conducted in this study.
PM Mode and Size
Engine exhaust particles have an agglomerate morphology; thus, their size is characterized by the notion of an equivalent diameter. The DMM employs a combination of mobility and aerodynamic analysis, but does not directly measure either equivalent diameter. Rather, we derive estimates of geometric mean mobility diameter by assuming a bimodal lognormal distribution of particle number concentration versus mobility diameter and fitting the DMM impactor and mobility currents to the calculated currents. This is similar to the procedure described previously for the ELPI (Maricq et al. Citation2006). The number of adjustable parameters is reduced to three by fixing the nucleation mode geometric mean diameter to 20 nm, its standard deviation to 1.3, and by assigning the universal value of 1.8 to the accumulation mode geometric standard deviation (Harris and Maricq Citation2001). Best fits of the DMM data and a typical OC density of 0.8 g/cm3 yield nucleation mode masses increasing from 2% to 5% of the total PM as the ethanol content rises. Choosing a different nucleation mode diameter or standard deviation alters the calculated mass, but it remains a small fraction of the total PM mass.
The influence of ethanol level on accumulation mode diameter is illustrated in . This shows three estimates of the geometric mean mobility diameter (μg ): (1) ELPI, (2) fits of DMM currents, and (3) calculated from the PM mass and number measurements via:
EquationEquation (1) assumes a log-normal mobility distribution of N 0 particles with geometric mean μg and standard deviation σg , an aggregate morphology with mass-mobility exponent Df = 2.3, a primary particle density of ρ 0 = 2 g/cm3, and a primary particle diameter of d 0 = 20 nm typical of engine soot (Maricq et al. Citation2006). Fits of DMM data yield mean mobility diameters of ∼150 nm, roughly double the size normally expected for combustion engines. This discrepancy is systematic but independent of the agreement between DMM and filter-based PM mass values. and S3 show that PM mass measurements from the two DMMs, ELPI, and EC/OC agree within the test-to-test variability. The question of size is discussed further in the Supplementary Information. Here, scaling the DMM values by 0.5 provides a consistent estimate of mean mobility equivalent particle diameter. The results reveal that accumulation mode particle diameter is essentially independent of ethanol level. For the E0–E17 fuels, average size may decrease a bit (∼5 nm) from cold start to warmed-up operation and from calibration A to calibration B (Figure S5). For E32 and E45, such changes are within measurement uncertainty.
EC/OC Composition
and S6 plot the elemental and organic carbon fractions of the PM emissions versus ethanol blend. EC is clearly the predominant component and follows the same trend as total PM mass, namely it decreases slightly from 0% to 17% ethanol, but falls by ∼45% for E32 and E45. In contrast, the OC component increases from about 0.1 mg/mi to 0.4 mg/mi from E0 to E45.
FIG. 6 Elemental carbon/organic carbon PM emissions versus fuel ethanol. (Color figure available online).
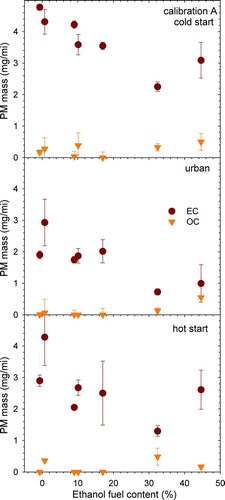
The low OC fraction is consistent with the small (<5%) nucleation mode mass determined from DMM data. However, this result should not be interpreted too literally. First, pyrolysis during thermal evolution of the OC introduces a bias toward a higher EC/OC ratio. Second, the ∼0.5-mg/mi correction for gaseous HC adsorption by quartz filters is only approximate. Nevertheless, OC constitutes a small fraction of the GDI vehicle PM emissions.
DISCUSSION
Overall, the effects of ethanol blends on GDI vehicle PM emissions described above agree with previous work. The data in Storey et al. (Citation2010) show a 30% PM decrease for E20, but as for the present study, this decrease lies within measurement uncertainty. In He et al. (Citation2010), there is likewise no clear distinction between E0 and E10, but they report a statistically significant 20% PM reduction for E20. Interestingly, He et al. (Citation2010) observe bimodal size distributions in their fast mobility particle sizer data, with peaks at 10 nm and 70 nm. The latter value coincides with the ∼70-nm mean accumulation mode mobility diameter depicted in . They further report that a three-way catalyst reduces nucleation mode emissions, consistent with the present DMM data, which indicate that this mode contributes little to the total PM mass from the three-way catalyst-equipped test vehicle.
The present study of GDI vehicle exhaust PM reveals interesting features not typically associated with gasoline vehicles: (1) a high fraction of elemental carbon and (2) a correlation between particle mass and number emissions. Normally, gasoline vehicle PM is considered primarily organic in nature; for example, EPA's Kansas City Study reports that OC accounts for about 80% of the particulate emissions (U.S. Environmental Protection Agency Citation2008). The explanation is that tight control of air/fuel stoichiometry allows little chance for sooting conditions to develop and, therefore, the observed PM largely derives from organic combustion byproducts and fugitive low-volatility fuel and lube components. But this reasoning applies to port fuel injection, where the fuel is vaporized at the intake port. Direct injection provides less opportunity for fuel vaporization and increases the likelihood of fuel impingement onto piston and cylinder surfaces, and the resulting combustion of liquid fuel produces soot. HC precursors to organic PM, though, are removed by the three-way catalyst, leaving the tailpipe PM with a high EC/OC content.
and 7S demonstrate the correlation between particle number and mass emissions. The ratio of ∼2 × 1012 particles/mg for E0–E17 fuels is the same as found for solid particles emissions from both GDI and diesel vehicles (Kirchner et al. Citation2010; Maricq et al. Citation2011). Since in the present work, we did not purposefully remove liquid droplets, this similarity indicates that there is virtually no nucleation mode. Apparently, pool fires and liquid droplet combustion in GDI engines produce PM sufficiently similar to the 60- to 80-nm geometric mean mobility diameter soot in diesel exhaust to yield a comparable number to mass correlation (Harris and Maricq Citation2001). The increase of the ratio toward 4 × 1012 particles/mg in some tests, particularly E32, suggests the possibility of a small nucleation mode.
The high soot content and likely formation by liquid fuel combustion suggest that a comparison of GDI vehicle PM to soot in ethanol–gasoline diffusion flames may be interesting (Maricq Citation2011). These flames fall into two characteristic groups: (1) open flames, orange in color and emitting soot from their tips, and (2) closed flames, yellow in color with no smoke emitted from the tip. E0 and E20 flames belong to the first group. They exhibit little difference in how soot size and number density develop with height of the flame. E50 is similar, but shows signs of reduced soot formation. In contrast, the E85 flame falls into group 2. In effect, ethanol blend combustion fundamentally follows a similar trend as found in the GDI vehicle emissions, namely a minimal impact on soot up to about E20, but then, larger reductions for high-level blends.
The present study suggests that substantial PM emissions benefits are not expected for low-level ethanol blends; at least not more than the ∼0.7-mg/mi measurement uncertainty. But, neither is there a PM disadvantage as the commercial light-duty fuel composition moves to E10, and possibly E20. The specific conclusions from this study might change as GDI engine designs evolve, but the reproducibility of the fuel effects at two different calibrations, plus the similar behavior in flames, suggests a measure of robustness to these conclusions.
uast_a_648780_sup_23255521.zip
Download Zip (70.1 KB)Acknowledgments
The authors are grateful to Sherry Mueller for measuring ethanol fuel content and Carolyn Hubbard for helping acquire the E100 fuel. We thank Joel Richert for help with the Horiba Mexa-1370PM and Mike Loos, Adolfo Mauti, and Jamie Taylor for their test cell support.
[Supplementary materials are available for this article. Go to the publisher's online edition of Aerosol Science and Technology to view the free supplementary files.]
REFERENCES
- Aikawa , K. , Sakurai , T. and Jetter , J. J. 2010 . Development of a Predictive Model for Gasoline Vehicle Particulate Matter Emissions . SAE Techical Paper No. 2010-01-2115. SAE, Warrendale, PA
- Akard , M. , Oestergaard , K. , Chase , R. E. , Richert , J. F. O. , Fukushima , H. and Adachi , M. 2004 . Comparison of an Alternative Particulate Mass Measurement with Advanced Microbalance Analysis . SAE Technical Paper No. 2004-01-0589. SAE, Warrendale, PA
- California Air Resources Board. 2010 . Preliminary Discussion Paper – Proposed Amendments to California's Low-Emission Vehicle Regulations – Particulate Matter Mass, Ultrafine Solid Particle Number, and Black Carbon Emissions . http://www.arb.ca.gov/msprog/levprog/leviii/meetings/051810/pm_disc_paper-v6.pdf
- Chen , L. , Braisher , M. , Crossley , A. , Stone , R. and Richardson , D. 2010 . The Influence of Ethanol Blends on Particulate Matter Emissions from Gasoline Direct Injection Engines . SAE Technical Paper No. , 2010-01-0793. SAE, Warrendale, PA
- Chow , J. C. , Watson , J. G. , Crow , D. , Lowenthal , D. H. and Merrifield , T. 2001 . Comparison of IMPROVE and NIOSH Carbon Measurements . Aerosol Sci. Technol., , 34 : 23 – 34 .
- Coordinating Research Council. 2011 . Reports E77-2c, Study to Determine Evaporative Emission Breakdown, Including Permeation Effects and Diurnal Emissions, Using E20 Fuels on Aging Enhanced Evaporative Emissions Certified Vehicles (2010) and E90-2a, Evaluation of Inspection and Maintenance OBD II Data to Identify Vehicles That May Be Sensitive to E10+ Blends (2011) . Available at http://www.crcao.com/publications/emissions/index.html
- Durbin , T. D. , Miller , J. W. , Younglove , T. , Huai , T. and Cocker , K. 2007 . Effects of Fuel Ethanol Content and Volatility on Regulated and Unregulated Exhaust Emissions for the Latest Technology Gasoline Vehicles . Environ. Sci. Technol., , 41 : 4059 – 4064 .
- Fraser , N. , Blaxill , H. , Lumsden , G. and Bassett , M. 2009 . Challenges for Increased Efficiency Through Gasoline Engine Downsizing . SAE Int. J. Eng. , 2 : 991 – 1008 .
- Giechaskiel , B. , Dilara , P. , Sandbach , E. and Andersson , J. 2008 . Particle Measurement Programme (PMP) Light-Duty Inter-Laboratory Exercise: Comparison of Different Particle Number Measurement Systems . Meas. Sci. Technol. , 19 : 095401
- Harris , S. J., and Maricq , M. M. 2001 . Signature Size Distributions for Diesel and Gasoline Engine Exhaust Particulate Matter . J. Aerosol Sci. , 32 : 749 – 764 .
- He , X. , Ireland , J. C. , Zigler , B. T. , Ratcliff , M. A. , Knoll , K. E. Alleman , T. L., . 2010 . The Impacts of Mid-Level Biofuel Content in Gasoline on SIDI Engine-Out and Tailpipe Particulate Matter Emissions . SAE Technical Paper No. 2010-01-2125. SAE, Warrendale, PA
- Kar , K. , and Cheng , W. K. 2009 . Speciated Engine-Out Organic Gas Emissions from a PFI-SI Engine Operating on Ethanol/Gasoline Mixtures . SAE Technical Paper No. 2009-01-2673. SAE, Warrendale, PA
- Keskinen , J. , Pietarinen , K. and Lehtimäki , M. 1992 . Electrical Low Pressure Impactor . J. Aerosol Sci. , 23 : 353 – 360 .
- Khalek , I. A. , Bougher , T. and Jetter , J. J. 2010 . Particle Emissions from a 2009 Gasoline Direct Injection Engine Using Different Commercially Available Fuels . SAE Technical Paper No. 2010-01-2117. SAE, Warrendale, PA
- Kirchner , U. , Vogt , R. and Maricq , M. 2010 . Investigation of EURO-5/6 Level Particle Number Emissions of European Diesel Light Duty Vehicles . SAE Technical Paper No. 2010-01-0789. SAE, Warrendale, PA
- Knoll , K. , West , B. , Huff , S. , Thomas , J. , Orban , J. and Cooper , C. 2009 . Effects of Mid-Level Ethanol Blends on Conventional Vehicle Emissions . SAE Technical Paper No. 2009-01-2723. SAE, Warrendale, PA
- Mamakos , A. , Ntziachristos , L. and Samaras , Z. 2006 . Evaluation of the Dekati Mass Monitor for the Measurement of Exhaust Particle Mass Emissions . Environ. Sci. Technol. , 40 : 4739 – 4745 .
- Maricq , M. M. 2011 . Soot Formation in Ethanol/Gasoline Fuel Blend Diffusion Flames . Combust. Flame , 159 : 170 – 180 .
- Maricq , M. M. , Podsiadlik , D. H. and Chase , R. E. 1999 . Examination of the Size-Resolved and Transient Nature of Motor Vehicle Particle Emissions . Environ. Sci. Technol. , 33 : 1618 – 1626 .
- Maricq , M. M. , Szente , J. , Loos , M. and Vogt , R. 2011 . Motor Vehicle PM Emissions Measurement at LEV III Levels . SAE Int. J. Eng. , 4 : 597 – 609 .
- Maricq , M. M. , Xu , N. and Chase , R. E. 2006 . Measuring Particulate Mass Emissions with an Electrical Low Pressure Impactor . Aerosol Sci. Technol. , 40 : 68 – 79 .
- Ntziachristos , L. , and Samaras , Z. 2010 . The Potential of a Partial-Flow Constant Dilution Ratio Sampling System as a Candidate for Vehicle Exhaust Aerosol Measurements . J. Air Waste Manage. Assoc. , 60 : 1223 – 1236 .
- Storey , J. M. , Barone , T. , Norman , K. and Lewis , S. 2010 . Ethanol Blend Effects on Direct Injection Spark-Ignition Gasoline Vehicle Particulate Matter Emissions . SAE Technical Paper No. 2010-01-2129. SAE, Warrendale, PA
- U. S. Energy Information Administration . 2011 . Petroleum & Other Liquids: Weekly Supply Estimates . Available at http://www.eia.doe.gov/dnav/pet/pet_sum_sndw_dcus_nus_w.htm
- U. S. Environmental Protection Agency . 2007 . Renewable Fuel Standard . Available at http://www.epa.gov/otaq/fuels/renewablefuels/index.htm
- U. S. Environmental Protection Agency . 2008 . Analysis of Particulate Matter Emissions from Light-Duty Gasoline Vehicles in Kansas City . Available at http://www.epa.gov/oms/emission-factors-research/420r08010.pdf
- U. S. Environmental Protection Agency . 2010 . EPA Announces E15 Partial Waiver Decision and Fuel Pump Labeling Proposal . Available at http://www.epa.gov/otaq/regs/fuels/additive/e15/420f10054.htm
- Yi , J. , Wooldridge , S. , Coulson , G. , Hilditch , J. , Iyer , C. O. Moilanen , P. , . 2009 . Development and Optimization of the Ford 3.5L V6 EcoBoost Combustion System . SAE Int. J. Eng. , 2 : 1388 – 1407 .