Abstract
A number of investigations have examined the impact of the use of biodiesel on the emissions of carbon dioxide and regulated emissions, but limited information exists on the chemical composition of particulate matter from diesel engines burning biodiesel blends. This study examines the composition of diesel particulate matter (DPM) emissions from a commercial agriculture tractor burning a range of biodiesel blends operating under a load that is controlled by a power take off (PTO) dynamometer. Ultra-low sulfur diesel (ULSD) fuel was blended with soybean and beef tallow based biodiesel to examine fuels containing 0% (B0), 25% (B25), 50% (B50), 75% (B75), and 100% (B100) biodiesel. Samples were then collected using a dilution source sampler to simulate atmospheric dilution. Diluted and aged exhaust was analyzed for particle mass and size distribution, PM2.5 particle mass, PM2.5 organic and elemental carbon, and speciated organic compounds. PM2.5 mass emissions rates for the B25, B50, and B75 soybean oil biodiesel mixtures had 20%–30% lower emissions than the petroleum diesel, but B100 emissions were about 40% higher than the petroleum diesel. The trends in mass emission rates with the increasing biodiesel content can be explained by a significant decrease in elemental carbon (EC) emissions across all blending ranges and increasing organic carbon (OC) emissions with pure biodiesel. Beef tallow biodiesel blends showed similar trends. Nevertheless, it is important to note that the study measurements are based on low dilution rates and the OC emissions changes may be affected by ambient temperature and different dilution conditions spanning micro-environments and atmospheric conditions. The results show that the use of biodiesel fuel for economic or climate change mitigation purposes can lead to reductions in PM emissions and a co-benefit of EC emission reductions. Detailed speciation of the OC emissions were also examined and are presented to understand the sensitivity of OC emissions with respect to biodiesel fuel blends.
Copyright 2012 American Association for Aerosol Research
INTRODUCTION
Environmental concerns and energy security issues have prompted legislation and regulatory actions spurring demand for alternative fuels, such as biodiesel. The greatest driving force for the use of biodiesel blends is the need to have a fuel that meets the future environmental and energy security needs while also meeting market demands for cost and engine operating performance; historically, large roadblocks for the acceptance of alternative fuels (Akinci et al. Citation2008). Biodiesel has many positive attributes associated with its use, but by far the most noted attribute highlighted by fleet managers is the similar operating performance to conventional diesel fuel (Carraretto et al. Citation2004) and the lack of changes required to facilities and maintenance procedures to implement the use of biodiesel fuel (Lapuerta et al. Citation2008). Worldwide, agricultural tractors are being fueled by biodiesel due to its lower commercial price compared to petroleum diesel fuel, and in many cases because it can be easily produced from vegetable oil, animal fat, or even used cooking oil (Refaat Citation2009). The fast spread of biodiesel usage around the globe has led to special interest in the effects the fuel could have on noncarbon dioxide emissions, and the subsequent human health and climate change impacts (Morris and Jia Citation2003). The use of biodiesel in diesel engines has been shown to cause a decrease in particulate matter, hydrocarbons and carbon monoxide, while NOx emissions tend to increase (Schumacher et al. Citation1996; Frey et al. Citation2008). Other studies have identified size distributions of fine particles from biodiesel as being different from those of petroleum diesel; in general, biodiesel emits less accumulation mode PM, but more particles with small aerodynamic diameters (Kim et al. Citation2008; Lin et al. Citation2008; Heikkila et al. Citation2009). Few studies have focused on chemical speciation of organic aerosols emitted by diesel engines using biodiesel; however, there are studies showing a relation between the Soluble Organic Fraction (SOF) and the oxygenation level of biodiesel (Lapuerta et al. Citation2003). Others show gas and particulate phase polycyclic aromatic hydrocarbons (PAHs) emission rates from diesel engines, all concluding that using biodiesel results in substantial decreases in those emissions (Durbin et al. Citation2000; Borras et al. Citation2009; Chien et al. Citation2009). In 2004, 25 million gallons of B100 were sold. By 2005, that number had tripled. Today, approximately 600 fleets nationwide are using biodiesel blends in their diesel engines, and biodiesel is available in its various blends at approximately 800 locations across the US (USEPA Citation2009). This study shows the detailed chemical speciation of organic aerosols emitted by various biodiesel blends from a nonroad diesel engine that is not equipped with after-treatment technology. Currently in the USA, Europe, and several countries in Asia, there are regulations that effectively require all new onroad diesel engines to have after-treatment. Nevertheless, due to the long life-time of nonroad diesel engines and the slower implementation of emissions standards for nonroad engines, a significant number of nonroad diesel engines will continue to operate for at least the next two to three decades without after-treatment; unless regulation force controls to be added as retrofits. The particulate matter speciation covered in this manuscript includes organic carbon (OC), elemental carbon (EC), n-alkanes, hopanes and steranes, fatty acid methyl esters, and PAHs. This study provides an understanding of how biodiesel blends will impact the composition of particulate matter emissions from diesel engines without after-treatment devices and provides PM2.5 emissions estimates from agricultural tractors, which are important sources of pollutants in areas where agriculture operations are major economic activities.
METHODS
Testing Vehicle, Fuel Blends, and Additional Equipment
The diesel-powered tractor used for this study was a John Deere 7700 model from 1993 that was not equipped with after-treatment control technologies. This specific model is commonly used with pure petroleum diesel fuel, as well as with commercial and home-made biodiesel blends. The engine load was controlled with a power take off (PTO) dynamometer AW NEB-400 in order to simulate real world engine operation conditions. In order to keep this experiment as representative as possible, the engine test cycle included two modes: idle, for 10 min; followed by a steady operation of the engine at the manufacturers rated speed of 2100 RPM and 126 HP, for 20 min. Samples were collected after the whole testing time of 30 min. The driving cycle used for the emissions test was selected to best mimic real world operating conditions of agricultural tractors that are used to power farming activities, based on discussions with John Deere experts at the Madison Area Technical College (MATC). The fuels used for the study were commercially available Ultra Low Sulfur Diesel fuel (ULSD, B0), virgin soybean oil based biodiesel (Chevron Philips Chemical Company, The Woodlands, TX), and beef tallow based biodiesel (Nova Biofuels Seneca, Seneca, IL). Blends of ULSD with soybean oil based biodiesel (25% [B25], 50% [B50], 75% [B75] and 100% [B100] by volume), and with beef tallow based biodiesel (50 (B50T), and 100% (B100T) by volume) were tested. Three tests were performed for each fuel of soy biodiesel blend and two for tallow based biodiesel blends. Four dynamic blank tests and one loading blank test were performed to assess data quality and control. Dynamic blank tests consist of regular 30 min tests without engine exhaust entering into the sampler. Their purpose is to establish the level of contamination in the sampler and by the filtered and activated carbon scrubbed dilution air. Loading blank tests consist of loading all sampling media in the sampler and collecting them after a few minutes in order to estimate the level of contamination introduced by sample handling.
Sampling Procedure
A dilution sampler was used to dilute the engine exhaust with filtered and scrubbed ambient air to mimic real atmospheric dilution of engine exhaust. The sampler was the same as described by Hildemann et al. (Citation1989) and applied to emissions testing as described by Okuda et al. (Citation2009). In addition to filter based media, two MSP M102 model Micro-Orifice Uniform Deposit Impactors (MOUDI) were used to collect size resolved particulate matter emissions from B0, B100, and B100T fuels (Kleeman et al. Citation2000). One MOUDI was loaded with Pall (Pall Life Sciences, Ann Arbor, MI) polytetrafluoroethylene (PTFE) 47 mm, Teflon membrane filters (TEF), and the other was loaded with MSP (MSP Corporation, Shoreview, MN) aluminum foils (AF), and a quartz fiber filter (QFF) after-filter (Pall Life Sciences, Ann Arbor, MI). TEF collected with the MOUDI were analyzed for mass gravimetric PM and the aluminum foils were analyzed for mass and elemental and organic carbon (ECOC). Filter samples were collected on 47 mm quartz fiber and Teflon filters (Pall Life Sciences, Ann Arbor, MI), supported by aluminum and Teflon holders respectively, after a PM2.5 cyclone to remove coarse particles. In this study, denuded filters are considered those in which flow passed through an activated carbon-impregnated cellulose denuder (Sunset Laboratory, Inc., Tigard, OR), used to capture semivolatile organic gases, and undenuded samples were collected without passing through the earlier mentioned denuder. Figure S1 (see online supplemental files) shows a schematic of the different sampling equipment connected to the dilution stack sampler.
Analytical Techniques
TEF and aluminum foils were weighed before and after sampling in a temperature and humidity controlled room with a robotic filter apparatus to determine PM2.5 mass (Kleeman et al. Citation2000). The quartz fiber filters were baked for 12 h at 550°C before sampling, to reduce the residual concentrations of organics on the filters. OC and EC were measured by a version of the NIOSH ECOC method (Schauer et al. Citation2003), using 1.5 cm2 punches of QFF and aluminum foils. The organic mass (OM) concentration was estimated by subtracting the denuded OC mass from the difference between total PM and EC mass [OM = (PM – EC) – OC]. This value accounts for the noncarbon elements in the particulate organic species emitted in the exhaust, mainly oxygen and hydrogen. Chemical speciation of particle organics was performed by gas chromatography/mass spectroscopy (GC/MS) analysis (Sheesley et al. Citation2004). Composite samples of two or three half quartz fiber filters, depending on the number of tests performed for each test, were spiked with isotopically labeled internal standards (IS4, Levoglucosan, KPA, PMSTD10, and PMSTD11) in order to track the response of the target compounds (Sheesley et al. Citation2007). Samples were extracted with dichloromethane and acetone using sonication then concentrated with rotary evaporators and a nitrogen evaporator. The portions of 250 μL aliquots were used for quantification of PAHs, alkanes, hopanes, steranes, fatty acids, and fatty acid methyl esters. The reported emission rates have been dynamic blank subtracted, and the uncertainty was calculated by taking the square root of the sum of the squared standard deviation of the dynamic blanks plus 20% of each detected value as determined by GCMS analysis (Stone et al. Citation2008).
Emission Rates
Emission rates of EC, OC, mass, and speciated chemical species were calculated in mass per kilogram of CO2 in the exhaust gas. Tests for soybean biodiesel blends were performed in triplicate, and in duplicate for the tallow biodiesel blends, which is the basis for the average measurements and uncertainties shown in the results of this study. These measurements were compared with pure ULSD fuel, referred to as B0. Real time measurements of CO2 emission rates in the exhaust and in the diluted sample were taken by a TESTO 350 (Testo 350, Testo Inc., Sparta, NJ) emissions analyzer, in addition to O2, CO, NO, and NO2. Dilution ratios were calculated by comparing the emission rates of CO, NO, and NO2 in direct exhaust with the diluted sample exhaust. Dilution ratio inside the dilution chamber was calculated by dividing the raw exhaust gas concentration by the dilution chamber gas concentration as measured by the portable emissions analyzer. Concentrations values that had the best data quality based on detection limit and repeatability were selected to calculate the dilution ratio for each test. In most tests, NO concentrations showed to be more consistent. Dilution ratios calculated for each test can be found in Table S1.
RESULTS
PM Mass, EC, and OC Emissions
As part of the EC and OC emissions characterization, the effect of an activated carbon denuder was studied. Results show that for EC emissions there is no significant change in filter loading when comparing the denuded filters with the undenuded filters, confirming that activated carbon denuders only adsorb organic volatile and semivolatile species but not elemental carbon. On a mass basis, the loss of particles in a denuder is small, since most of the lost particles have very small diameters (less than 30 nm), and the mass contribution of such particles is negligible compared with particles with greater diameters (Kittelson et al. Citation2006; Cheng et al. Citation2009).
FIG. 1 Denuded and undenuded EC and OC emission rates. (a) Influence of denuding aerosol on elemental carbon (EC) emission rates, and on (b) organic carbon emission rates. Backup filters correspond to quartz fiber filters preceded by Teflon membrane filters (TEF) collecting semivolatile organics.
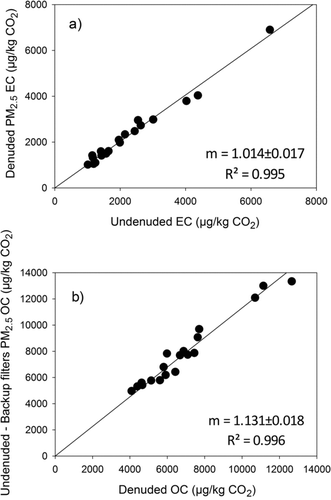
Good agreement between the denuded and undenuded filters EC measurements, with an R square value of 0.995 is shown in . On the other hand, OC filter loading was different between denuded and undenuded filters; there was an approximate 20% difference between the OC loading in those filters, as shown in Figure S2, indicating the influence of semivolatile/volatile organic partitioning in quartz fiber filters in the estimation of particle OC mass. In order to evaluate the removal of semivolatile/volatile organic species by the denuder, the OC mass in the denuded filters was compared with the OC mass in quartz fiber filters (Backup) installed on a separate sample train, before Teflon filters. The OC mass collected in denuded filters and that collected in undenuded filters minus the collected in backup filters correlated well with a simple linear regression, as observed in , indicating that the denuder removes a good portion of semivolatile/volatile organics from the OC mass collected in undenuded filters, which is in good agreement with other studies (Cheng et al. Citation2009; Okuda et al. Citation2009). Nonetheless, the use of denuders does not completely overrule the additional effect that could have the dilution of engine exhaust on the partitioning of organic species in quartz fiber filters (Lipsky and Robinson Citation2006).
In this study, we considered the denuded OC emissions as representatives of the particle phase OC. shows OC emission rates for soybean oil and beef tallow biodiesel fuels. OC emissions increased with the increased biodiesel content with the highest emission rates found with largest biodiesel content. The OM to OC ratio shows a similar trend to OC emissions, as observed in , which indicates particulate organic species emitted by pure biodiesel combustion have higher oxygen content compared to those emitted by pure petroleum diesel.
Size Resolved EC and OM Emission Rates for Tested Biodiesel Blends
The size resolved EC and OM emission rates as determined using the MOUDI samplers are shown in .
FIG. 3 Size resolved EC and OM emission rates in diesel exhaust from the combustion of (a) pure petroleum diesel, (b) pure soybean oil based biodiesel, and (c) pure beef tallow based biodiesel fuels.
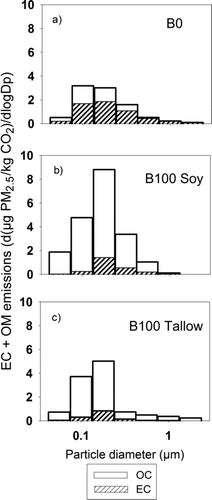
The results show that the fraction of biodiesel in the blend impacts the size distribution of ultrafine particles. The increment in particle number in the 100–200 nm particle diameter range for soybean oil biodiesel B100 is about 70% compared to B0. This increase is not as large for beef tallow biodiesel B100 (about 40%). EC decreases with pure biodiesel in the same particle diameter range, while OM increases. It is important to highlight that soybean oil and beef tallow biodiesel emissions show a similar peak in the range of 100 nm to 200 nm particle diameter.
Chemical Speciation of PM2.5 Emissions for Biodiesel Blends
shows emission rates of fatty acid methyl esters, fatty acids, and other organics. B0 fuel exhaust showed no detectable fatty acid methyl ester emissions, whereas biodiesel blends (from B50 to B100) showed increases of 74% for soy biodiesel and of 64% for tallow biodiesel. N-alkane emission rates were reduced in 35% for B50 soy biodiesel, and in 82% for pure soy biodiesel compared to B0 fuel. Tallow biodiesel showed 69% reduction in n-alkane emission rates for B50 and 87% for pure tallow biodiesel. B50 soybean oil resulted in a 73% decrease in PAHs emission rates and 80% for B100 soybean oil, compared to B0. PAHs emission rates were reduced to 76% for B50 beef tallow and 84% for B100 beef tallow blends. Since hopanes and steranes species are associated with the engine lubricant oil and not with the fuel, emission rates of these species were expected to show little variation. Compared with the other analyzed species, there was not a clear variation between hopanes and steranes emission rates with the biodiesel content in fuel blends, as seen in . In the case of soybean oil based biodiesel, B50 fuel blends showed a slight decrease of about 8% in these emissions compared to B0. For B100 fuel blends there was an increase of about 20% compared to B0. At the same time, tallow based biodiesel blends showed no specific correlation either, with a 30% increase for B50, and a 19% decrease for B100 compared to B0.
FIG. 4 PM2.5 emission rates of detected dominant organic compound functional groups. (a) Fatty acid methyl esters, fatty acids, and other organics. (b) Polycyclic aromatic hydrocarbons, hopanes and steranes, and n-alkanes.
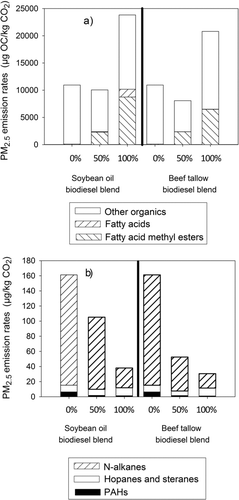
Calculated emission rates of hopanes and steranes for the different fuel blends are summarized in Table S3.
Table 1 shows emission rates and the associated uncertainties of the analyzed dominant organic functional groups.
Speciated n-alkane and fatty acid methyl ester species emission rates are shown in . The detected dominant n-alkane species were n-octadecane, n-heptadecane, n-nona- decane n-eicosane, n-hexadecane, n-norpristane, and n-henei- cosane. In general, emission rates of all these species were distributed almost evenly for all fuel blends. Blending petroleum diesel with biodiesel fuel results in clear reductions in emission rates of all detected n-alkane species; beef tallow biodiesel blends clearly showed greater reductions compared to B0. shows as dominant fatty acid methyl ester species linoleic acid, oleic acid, and hexadecanoic acid methyl esters. In this case, blending petroleum diesel with biodiesel fuel results in increasing fatty acid methyl ester emission rates, which is more significant for soybean oil biodiesel blends.
FIG. 5 PM2.5 emission rates of speciated organic compounds: (a) n-alkanes, and (b) fatty acid methyl esters.
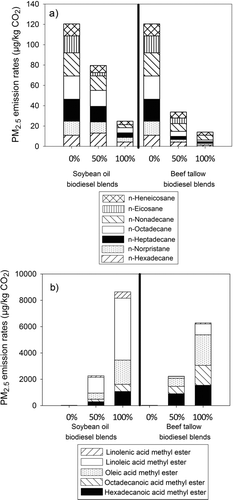
Emission rates of the speciated fatty acid methyl ester species and their content in each fuel blend are reported in .
TABLE 1 Emission rates of organic species in fine particulate matter from the combustion of different blends of petroleum diesel and soybean oil and beef tallow based biodiesel fuels
TABLE 2 Emission rates of analyzed fatty acid methyl esters in fine particulate matter from the combustion of different blends of petroleum diesel and soybean oil and beef tallow based biodiesel fuels and fatty acid methyl ester composition of the different fuel blends
shows emission rates of all speciated PAH species, with phenanthrene, pyrene, and fluoranthene as dominant constituents. Low molecular weight (LMW) PAH emission rates are considerably higher compared to those of high molecular weight (HMW) PAHs, accounting for more than 55% of total emission rates. Blending petroleum diesel with biodiesel fuel reduced LMW PAH emission rates by about 80%, using B50, and 87% for B100 soybean oil blends. HMW PAH emission rates decreased about 67% for B50 and 73% for B100 soybean oil blends, as shown in , thus decreasing the risk associated with human exposure to such harmful and often carcinogenic species. The reductions achieved by using B100 or B50 did not show great variation; indicating that there is no added value to B100 compared to B50 in terms of PAH emissions.
DISCUSSION
In general, blending petroleum diesel fuel with either vegetable or animal origin biodiesel fuel showed benefits in terms of reductions in PM2.5 emissions of the analyzed species from an agricultural tractor without any exhaust aftertreatment device. Compared with straight petroleum diesel fuel, PM2.5 EC emissions are greatly reduced by blending this fuel with biodiesel from either vegetable or animal origin. On the other hand, increasing the content of biodiesel in fuel blends does not correlate well with PM2.5 OC emissions. For B25 blends, there is a slight decrease in such emissions, whereas for B50 and B75 blends there is small increase; oddly, combustion of straight biodiesel fuel showed a significant increase in PM2.5 OC emissions, which might be attributed to the incomplete combustion of fatty acid methyl esters. This sudden reduction in the combustion reaction yield might as well be associated with the almost total lack of n-alkanes and other hydrocarbon species present in petroleum diesel fuel, which is contradictory with the fact that usually biodiesel fuels have higher cetane numbers compared with petroleum diesel fuel, and higher cetane numbers are associated with shorter ignition delays and thus more time for the combustion process to be completed. Nevertheless, there is no significant improvement in engine performance for cetane numbers higher than 55. Moreover, several scientists have agreed that engine performance is only slightly reduced by the use of biodiesel blends, even though fuel consumption increases and heating values are lower due to lower carbon contents in these fuels (Xue et al. Citation2011). Also, driving cycles, engine types and age could have a strong influence. For all these reasons, the conclusion about the results discussed earlier is that more research is substantially required before reaching any robust explanation.
From the climate change point of view, reductions in EC aerosol emissions could reduce the positive radiative forcing effect associated with diesel PM emissions (Gaffney and Marley Citation2009), and since OC aerosol species tend to have a negative radiative forcing effect (Adler et al. Citation2009) their warming effect might as well be reduced. In regards to OC aerosol emissions, there was little variation for B25, B50, and B75 blends, however, using pure biodiesel fuel (B100) resulted in increases in overall OC aerosol emission rates, mostly associated with unburned fatty acid methyl esters. Whereas prior studies have shown that PM10 emissions decrease when blending petroleum diesel fuel with biodiesel (Schumacher et al. Citation1996; Durbin et al. Citation2000; Krahl et al. Citation2002; Lapuerta et al. Citation2003; Knothe et al. Citation2006; Frey et al. Citation2008), other studies showed higher emissions of particles with smaller particle diameters from diesel engines operating on biodiesel fuel blends compared with petroleum diesel fuel, associated with factors such as the fuel composition, heating value and thermodynamic properties of species in the exhaust (Kim et al. Citation2008; Heikkila et al. Citation2009).
Few studies have focused on the chemical composition of PM emissions from the combustion of biodiesel fuel blends. Most of these studies have covered EC and OC composition analysis (Chung et al. Citation2008; Zhang et al. Citation2009), and further analyzing PAH species from the OC portion of the PM (Borras et al. Citation2009; Chien et al. Citation2009; Tsai et al. Citation2011). Having a detailed composition profile of the particles emitted by a source provides valuable information that can be used in receptor models, which helps characterizing and clearly identifying the differences in chemical composition of different source types. Elemental composition of source emissions has been used on many occasions to identify specific sources from ambient monitoring samples of airborne particles (Schauer et al. Citation1996). Prior studies have identified molecular marker profiles of diesel exhaust (Lough et al. Citation2007), which have been and are still used in the application of source apportionment models throughout the world (Stone et al. Citation2008; Miller-Schulze et al. Citation2011; Sahu et al. Citation2011); nonetheless, there is very limited information about molecular marker profiles of biodiesel exhaust (Dutcher et al. Citation2011). Even with the limitations associated with the consistency of the measurements performed in this study due to lower dilution ratios, the chemical speciation of aerosol organic species present in the exhaust of a nonroad engine operating on biodiesel blends may provide a good basis for identifying potential molecular markers of combustion of biodiesel or biodiesel blends, which can be used in source apportionment models providing accurate estimates of source contributions to atmospheric particulate matter concentrations. Fatty acids have been investigated as potential markers of biomass burning (Ballentine et al. Citation1996; Oros and Simoneit Citation2001) and even cooking sources (Hou et al. Citation2006); on the other hand, few alkyl ester species have been identified as molecular biomarkers, except from HMW wax esters used as biomarkers of biomass burning (Oros and Simoneit Citation2001). So far, fatty acid methyl esters have not been associated as potential candidates to be molecular markers of biodiesel combustion. Between the reasons for not including such species as potential markers of biodiesel combustion could be: the lack of knowledge about detailed organic aerosol chemical speciation, the wide variety of biodiesel sources, and thus, variety in fatty acid methyl ester composition (Hoekman et al. Citation2012), the frequent formation of fatty acid methyl esters from fatty acids in the atmosphere favored by acidic atmospheric environments (Ma et al. Citation2010), and their tendency to be degraded by sunlight and interactions with marine aerosols (Khoury et al. Citation2011). Notwithstanding, the emission profiles of biodiesel combustion provided by this study may be the basis for further studies regarding this topic.
This study provides a wider view of the chemical composition of carbonaceous organic aerosol emitted from a diesel engine operating on biodiesel fuel blends, limited by engine type and age, fuel type, and driving cycle, factors that may have an important influence on such emission profiles (Schauer Citation2003; Kim et al. Citation2008; Borras et al. Citation2009; Lapuerta et al. Citation2009). Based on the earlier discussion, and keeping in mind that dilution of engine exhaust also plays a very important role on specifying realistic emission profiles from sources (Lipsky and Robinson Citation2006), this study has few comparable conditions with prior studies. Although further research on the parameters mentioned earlier is required before reaching any conclusions, since the engine type used in the tractor tested in this study has been used in different nonroad vehicles other than agricultural tractors such as front loaders and graders manufactured by John Deere and widely used in construction applications, the reach of this study could be extended to such vehicles.
Dilution samplers are typically operated at dilution ratios between 20:1 and 200:1. A dilution ratio of 100:1 is generally high enough to reduce the exhaust temperature to ambient levels, but the median dilution ratio of vehicle exhaust in an urban atmosphere is around 10,000:1. Dilution ratios estimated in this study range between 15 and 30, with temperatures inside the dilution stack sampler varying from 25°C to 28°C. At these conditions, PM2.5 concentrations inside the dilution sampler ranged between 800 and 1900 μg/cm3, as shown in Table S2. Since dilution can cause changes in partitioning of semivolatile organic compounds (Pankow Citation1994; Lipsky and Robinson Citation2006; Robinson et al. Citation2007), the partitioning of semivolatile organic compounds that contribute to particulate matter organic carbon is expected to be different between these experiments, and typical atmospheric conditions. It is important to note, however, that partitioning is very sensitive to temperature and since atmospheric temperatures vary over a very wide range, there really is not singular dilution condition that can be used to represent the partitioning of the atmosphere.
Nevertheless, even if there is an overestimation of fine particle emissions due to the difference between sampling and atmospheric conditions, it is clear that the use of biodiesel blends results in reductions in emissions of n-alkane and PAH species. The results of this study showed increasing fatty acid methyl ester emissions with increasing biodiesel content in fuel blends, which may be associated with differences in fuel composition; petroleum diesel contains mainly saturated and aromatic hydrocarbons while biodiesel fuel contains mainly fatty acid methyl esters (Maricq Citation2007). The higher oxygen content in biodiesel fuel may result in better combustion yields (Lapuerta et al. Citation2009), nonetheless, fatty acid methyl ester emissions from pure biodiesel fuel (B100) were greatly increased with respect to other fuel blends. This increase can be associated with the driving cycle used, the type and age of the engine, and the lower carbon content in biodiesel which results in power loss and consequent higher fuel consumption.
From the human health point of view, reductions in particle emissions of EC and species such as PAHs, greatly reduces the risks associated with exposure to diesel exhaust, mainly for agricultural workers. Since in the past 50 years there has been a dramatic increase in agricultural tractor use (Federico Citation2005), operating agricultural tractors with biodiesel blends would significantly decrease this risk.
In summary, the results of this study may be the starting point for developing useful tools for climate modelers and policy makers concerned with the current and future use of fossil fuel alternatives.
uast_a_696315_sup_26396140.zip
Download Zip (784.7 KB)Acknowledgments
[Supplementary materials are available for this article. Go to the publisher's online edition of Aerosol Science and Technology to view the free supplementary files.]
REFERENCES
- Adler , G. , Riziq , A. A. , Erlick , C. and Rudich , Y. 2009 . Effect of Intrinsic Organic Carbon on the Optical Properties of Fresh Diesel Soot . Proc. Nat. Acad. Sci. U. S. A. , 107 : 6699 – 6704 .
- Akinci , B. , Kassebaum , P. G. , Fitch , J. V. and Thompson , R. W. 2008 . The Role of Bio-Fuels in Satisfying US Transportation Fuel Demands . Energy Policy , 36 : 3485 – 3491 .
- Ballentine , D. C. , Macko , S. A. , Turekian , V. C. , Gilhooly , W. P. and Martincigh , B. 1996 . Compound Specific Isotope Analysis of Fatty Acids and Polycyclic Aromatic Hydrocarbons in Aerosols: Implications for Biomass Burning . Org. Geochem. , 25 : 97 – 104 .
- Borras , E. , Tortajada-Genaro , L. A. , Vazquez , M. and Zielinska , B. 2009 . Polycyclic Aromatic Hydrocarbon Exhaust Emissions from Different Reformulated Diesel Fuels and Engine Operating Conditions . Atmos. Environ. , 43 : 5944 – 5952 .
- Carraretto , C. , Macor , A. , Mirandola , A. , Stoppato , A. and Tonon , S. 2004 . Biodiesel as Alternative Fuel: Experimental Analysis and Energetic Evaluations . Energy , 29 : 2195 – 2211 .
- Cheng , Y. , He , K. B. , Duan , F. K. , Zheng , M. , Ma , Y. L. and Tan , J. H. 2009 . Measurement of Semivolatile Carbonaceous Aerosols and Its Implications: A Review . Environ. Int. , 35 : 674 – 681 .
- Chien , S. M. , Huang , Y. J. , Chuang , S. C. and Yang , H. H. 2009 . Effects of Biodiesel Blending on Particulate and Polycyclic Aromatic Hydrocarbon Emissions in Nano/Ultrafine/Fine/Coarse Ranges from Diesel Engine . Aerosol Air Qual. Res. , 9 : 18 – 31 .
- Chung , A. , Lall , A. A. and Paulson , S. E. 2008 . Particulate Emissions by a Small Non-Road Diesel Engine: Biodiesel and Diesel Characterization and Mass Measurements Using the Extended Idealized Aggregates Theory . Atmos. Environ. , 42 : 2129 – 2140 .
- Durbin , T. D. , Collins , J. R. , Norbeck , J. M. and Smith , M. R. 2000 . Effects of Biodiesel, Biodiesel Blends, and a Synthetic Diesel on Emissions from Light Heavy-Duty Diesel Vehicles . Environ. Sci. Technol. , 34 : 349 – 355 .
- Dutcher , D. D. , Pagels , J. , Bika , A. , Franklin , L. , Stolzenburg , M. Thompson , S. 2011 . Emissions from Soy Biodiesel Blends: A Single Particle Perspective . Atmos. Environ. , 45 : 3406 – 3413 .
- Federico , G. 2005 . Feeding the World: An Economic History of Agriculture 1800–2000 , Princeton , NJ : Princeton University Press .
- Frey , H. C. , Rasdorf , W. , Kim , K. , Pang , G. H. and Lewis , P. 2008 . Comparison of Real-World Emissions of B20 Biodiesel Versus Petroleum Diesel for Selected Nonroad Vehicles and Engine Tiers . Transp. Res. Rec. , 2058 : 33 – 42 .
- Gaffney , J. S. and Marley , N. A. 2009 . The Impacts of Combustion Emissions on Air Quality and Climate – from Coal to Biofuels and Beyond . Atmos. Environ. , 43 : 23 – 36 .
- Heikkila , J. , Virtanen , A. , Ronkko , T. , Keskinen , J. , Aakko-Saksa , P. and Murtonen , T. 2009 . Nanoparticle Emissions from a Heavy-Duty Engine Running on Alternative Diesel Fuels . Environ. Sci. Technol. , 43 : 9501 – 9506 .
- Hildemann , L. M. , Cass , G. R. and Markowski , G. R. 1989 . A Dilution Stack Sampler for Collection of Organic Aerosol Emissions - Design, Characterization and Field-Tests . Aerosol Sci. Technol. , 10 : 193 – 204 .
- Hoekman , S. K. , Broch , A. , Robbins , C. , Ceniceros , E. and Natarajan , M. 2012 . Review of Biodiesel Composition, Properties, and Specifications . Renewable Sustainable Energy Rev. , 16 : 143 – 169 .
- Hou , X. , Zhuang , G. , Sun , Y. and An , Z. 2006 . Characteristics and Sources of Polycyclic Aromatic Hydrocarbons and Fatty Acids in PM2.5 Aerosols in Dust Season in China . Atmos. Environ. , 40 : 3251 – 3262 .
- Khoury , R. R. , Ebrahimi , D. , Hejazi , L. , Bucknall , M. P. , Pickford , R. and Brynn Hibbert , D. 2011 . Degradation of Fatty Acid Methyl Esters in Biodiesels Exposed to Sunlight and Seawater . Fuel , 90 : 2677 – 2683 .
- Kim , M. Y. , Yoon , S. H. , Hwang , J. W. and Lee , C. S. 2008 . Characteristics of Particulate Emissions of Compression Ignition Engine Fueled with Biodiesel Derived from Soybean . J. Eng. Gas Turbines Power , 130 : 05280s-1 – 05280s-7 .
- Kittelson , D. B. , Watts , W. F. and Johnson , J. P. 2006 . On-Road and Laboratory Evaluation of Combustion Aerosols—Part1: Summary of Diesel Engine Results . J. Aerosol Sci. , 37 : 913 – 930 .
- Kleeman , M. J. , Schauer , J. J. and Cass , G. R. 2000 . Size and Composition Distribution of Fine Particulate Matter Emitted from Motor Vehicles . Environ. Sci. Technol. , 34 : 1132 – 1142 .
- Knothe , G. , Sharp , C. A. and Ryan , T. W. 2006 . Exhaust Emissions of Biodiesel, Petrodiesel, Neat Methyl Esters, and Alkanes in a New Technology Engine . Energy Fuels , 20 : 403 – 408 .
- Krahl , J. , Bunger , J. , Schroder , O. , Munack , A. and Knothe , G. 2002 . Exhaust Emissions and Health Effects of Particulate Matter from Agricultural Tractors Operating on Rapeseed Oil Methyl Ester . J. Am. Oil Chem. Soc. , 79 : 717 – 724 .
- Lapuerta , M. , Armas , O. and Rodriguez-Fernandez , J. 2009 . Effect of Biodiesel Fuels on Diesel Engine Emissions (vol 34, pg 198, 2008) . Prog. Energy Combust. Sci. , 35 : 383 – 383 .
- Lapuerta , M. , Fernandez , J. R. and Agudelo , J. R. 2008 . Diesel Particulate Emissions from Used Cooking Oil Biodiesel . Bioresour. Technol. , 99 : 731 – 740 .
- Lapuerta , M. , Hernandez , J. J. , Ballesteros , R. and Duran , A. 2003 . Composition and Size of Diesel Particulate Emissions from a Commercial European Engine Tested with Present and Future Fuels . Proc. Inst. Mech. Eng. Part D J. Automob. Eng. , 217 : 907 – 919 .
- Lin , Y. C. , Lee , C. F. and Fang , T. 2008 . Characterization of Particle Size Distribution from Diesel Engines Fueled with Palm-Biodiesel Blends and Paraffinic Fuel blends . Atmos. Environ. , 42 : 1133 – 1143 .
- Lipsky , E. M. and Robinson , A. L. 2006 . Effects of Dilution on Fine Particle Mass and Partitioning of Semivolatile Organics in Diesel Exhaust and Wood Smoke . Environ. Sci. Technol. , 40 : 155 – 162 .
- Lough , G. C. , Christensen , C. G. , Schauer , J. J. , Tortorelli , J. , Mani , E. Lawson , D. R. 2007 . Development of Molecular Marker Source Profiles for Emissions from On-Road Gasoline and Diesel Vehicle Fleets . Air Waste Manage. Assoc. , 57 : 1190 – 1199 .
- Ma , S. , Peng , P. , Song , J. , Bi , X. , Zhao , J. He , L. 2010 . Seasonal and Spatial Changes of Free and Bound Organic Acids in Total Suspended Particles in Guangzhou, China . Atmos. Environ. , 44 : 5460 – 5467 .
- Maricq , M. M. 2007 . Chemical Characterization of Particulate Emissions from Diesel Engines: A Review . J. Aerosol Sci. , 38 : 1079 – 1118 .
- Miller-Schulze , J. P. , Shafer , M. M. , Schauer , J. J. , Solomon , P. A. , Lantz , J. Artamonova , M. 2011 . Characteristics of Fine Particle Carbonaceous Aerosol at Two Remote Sites in Central Asia . Atmos. Environ. , 45 : 6955 – 6964 .
- Morris , R. E. and Jia , Y. 2003 . “ Impact of Biodiesel Fuels on Air Quality and Human Health: Task 5 Report ” . In Air Toxics Modeling of the Effects of Biodiesel Fuel Use on Human Health in the South Coast Air Basin Region of Southern California , Novato , CA : National Renewable Energy Laboratory .
- Okuda , T. , Schauer , J. J. , Olson , M. R. , Shafer , M. M. , Rutter , A. P. Walz , K. A. 2009 . Effects of a Platinum-Cerium Bimetallic Fuel Additive on the Chemical Composition of Diesel Engine Exhaust Particles . Energy Fuels , 23 : 4974 – 4980 .
- Oros , D. R. and Simoneit , B. R. T. 2001 . Identification and Emission Factors of Molecular Tracers in Organic Aerosols from Biomass Burning Part 1. Temperate Climate Conifers . Appl. Geochem. , 16 : 1513 – 1544 .
- Pankow , J. F. 1994 . An Absorption Model of Gas/Particle Partitioning of Organic Compounds in the Atmosphere . Atmos. Environ. , 28 : 185 – 188 .
- Refaat , A. A. 2009 . Different Techniques for the Production of Biodiesel from Waste Vegetable Oil . Int. J. Environ. Sci. Technol. , 7 : 183 – 213 .
- Robinson , A. L. , Donahue , N. M. , Shrivastava , M. K. , Weitkamp , E. A. , Sage , A. M. Grieshop , A. P. 2007 . Rethinking Organic Aerosols: Semivolatile Emissions and Photochemical Aging . Science , 315 : 1259 – 1262 .
- Sahu , M. , Hu , S. , Ryan , P. H. , Le Masters , G. , Grinshpun , S. A. Chow , J. C., . 2011 . Chemical Compositions and Source Identification of PM2.5 Aerosols for Estimation of a Diesel Source Surrogate . Sci. Total Environ. , 409 : 2642 – 2651 .
- Schauer , J. J. 2003 . Evaluation of Elemental Carbon as a Marker for Diesel Particulate Matter . J. Expo. Anal. Environ. Epidemiol. , 13 : 443 – 453 .
- Schauer , J. J. , Mader , B. T. , Deminter , J. T. , Heidemann , G. , Bae , M. S. Seinfeld , J. H. 2003 . ACE-Asia Intercomparison of a Thermal-Optical Method for the Determination of Particle-Phase Organic and Elemental Carbon . Environ. Sci. Technol. , 37 : 993 – 1001 .
- Schauer , J. J. , Rogge , W. F. , Hildemann , L. M. , Mazurek , M. A. , Cass , G. R. and Simoneit , B. R. T. 1996 . Source Apportionment of Airborne Particulate Matter Using Organic Compounds as Tracers . Atmos. Environ. , 30 : 3837 – 3855 .
- Schumacher , L. G. , Borgelt , S. C. , Fosseen , D. , Goetz , W. and Hires , W. G. 1996 . Heavy-Duty Engine Exhaust Emission Tests Using Methyl Ester Soybean Oil/Diesel Fuel Blends . Bioresour. Technol. , 57 : 31 – 36 .
- Sheesley , R. J. , Schauer , J. J. , Bean , E. and Kenski , D. 2004 . Trends in Secondary Organic Aerosol at a Remote Site in Michigan's Upper Peninsula . Environ. Sci. Technol. , 38 : 6491 – 6500 .
- Sheesley , R. J. , Schauer , J. J. , Meiritz , M. , DeMinter , J. T. , Bae , M. S. and Turner , J. R. 2007 . Daily Variation in Particle-Phase Source Tracers in an Urban Atmosphere . Aerosol Sci. Technol. , 41 : 981 – 993 .
- Stone , E. A. , Snyder , D. C. , Sheesley , R. J. , Sullivan , A. P. , Weber , R. J. and Schauer , J. J. 2008 . Source Apportionment of fine Organic Aerosol in Mexico City During the MILAGRO Experiment 2006 . Atmos. Chem. Phys. , 8 : 1249 – 1259 .
- Tsai , J. H. , Chen , S. J. , Huang , K. L. , Lee , W. J. , Kuo , W. C. and Lin , W. Y. 2011 . Characteristics of Particulate Emissions from a Diesel Generator Fueled with Varying Blends of Biodiesel and Fossil Diesel . J. Environ. Sci. Health Part A-Toxic/Hazard. Subst. Environ. Eng. , 46 : 204 – 213 .
- USEPA . 2009 . Biodiesel, Office of Transportation and Air Quality . Available online at: http://www.epa.gov/otag/renewablefuels/42of09064.pdf
- Xue , J. , Grift , T. E. and Hansen , A. C. 2011 . Effect of Biodiesel on Engine Performances and Emissions . Renew. Sust. Energ. Rev. , 15 : 1098 – 1116 .
- Zhang , J. , He , K. B. , Shi , X. Y. and Zhao , Y. 2009 . Effect of SME Biodiesel Blends on PM2.5 Emission from a Heavy-Duty Engine . Atmos. Environ. , 43 : 2442 – 2448 .