Abstract
We characterized the properties of combustion aerosol produced at different operating conditions of a mini-CAST burner that was treated in a Catalytic Stripper (CS) operating at 300°C. The goal was to establish a methodology for the production of soot particles resembling those emitted from internal combustion engines. Thermo-optical analysis of samples collected on Quartz filters revealed that the particles contained semi-volatile material that survived the CS. The amount of semi-volatile species strongly depended on the operating conditions ranging from less than 10% to as high as 30% of the particle mass. The mini-CAST operating conditions were also found to have a strong effect on the effective particle density (ρe ). The ρe , for example, ranged from as low as 0.3 to 1.05 g/cm3 for mondisperse 80 nm particles, although the mass-mobility exponent remained relatively constant (2.1–2.25). These differences are indicative of differences in the primary particle diameter, which was estimated to range between 8.5 and 34 nm depending on the operating conditions. The different types of particles produced were also found to exhibit different affinities for butanol but also different light absorption per mass of elemental carbon which can, therefore, lead to inconsistencies in aerosol instrumentation calibrations (e.g., condensation and optical particle counters, photoacoustic sensors). The work highlights the importance of establishing a detailed and well-defined method in using the mini-CAST-CS approach for instrument calibration in ways mimicking various engine combustion sources.
Copyright 2013 American Association for Aerosol Research
INTRODUCTION
Combustion generated particles are the major constituent of anthropogenic ambient particulate matter (PM) (Weijer et al. Citation2011). They have been associated with adverse health effects (Pope et al. Citation2002) and are considered strong climate forcers (UNEP/WMO Citation2011), stimulating legislative authorities worldwide to regulate their emissions at their sources. PM limits are already established in all major transport sectors (on-road, locomotive, marine, and aviation) but also for stationary and off-road applications.
Traditionally, combustion generated PM emissions have been measured via collection on filters and subsequent gravimetric quantification (Berg Citation2003). The tightening of the emission standards over the years has triggered a series of research initiatives to improve the filter-based method (USEPA Citation2008). Even with such improvements, concerns have risen regarding the sensitivity of the filter-based methods in quantifying the true emissions at very low levels (Giechaskiel et al. Citation2012). Accordingly, alternative, more sensitive, techniques have been pursued to supplement or even replace filter-based methods. An important development in this direction has been the introduction of a non-volatile Particle Number (PN) standard in Europe for the certification of diesel (EC Citation2007) and gasoline (EC Citation2012) Light Duty Vehicles (LDVs) as well as diesel (EC Citation2009) Heavy Duty Engines (HDEs). A similar PN methodology accompanied by a soot mass determination with either photoacoustic sensors or laser-induced incandescence sensors is currently under evaluation for the replacement of the currently applicable smoke number procedure in aircraft jet engines (SAE Standard AIR589 Citation2012). A simplified PN procedure was already adopted in Switzerland for the periodic inspection of construction equipment (VAMV Citation2006). While the U.S. EPA has not pursued the non-volatile particle approach, interest in nonvolatile and volatile PN is not off the table, and efforts are under way to better understand the benefits of adopting such approaches. Meanwhile, an arsenal of aerosol instrumentation (including photoacoustic sensors, diffusion chargers and quartz crystal microbalances) is also under evaluation (Boucher et al. Citation2012; Mamakos et al. 2012) or even accepted (USEPA Citation2008; USEPA Citation2010) for application in In-Service-Conformity (EU) and In-Use-Compliance (USA) of Heavy Duty Vehicles (HDVs) with Portable Emission Measurement Systems.
All of these developments in the legislation necessitate the establishment of traceable calibration procedures for a wide range of aerosol instruments. Appropriate selection of the calibration aerosol is of crucial importance, as the instrument response may strongly depend on particle chemistry (Giechaskiel et al. Citation2011) and morphology (Biskos et al. Citation2004; Mamakos et al. Citation2013). Ideally, the calibration aerosol should resemble that of the emission source, but there is an inherent difficulty in identifying such a reference aerosol given the strong dependence of engine exhaust aerosol properties on the engine operating conditions (Mathis et al. Citation2005; Olfert et al. Citation2007), fuel (Tzamkiozis et al. Citation2011) and engine types (Mathis et al. Citation2004).
Flame burners have been recognized as an attractive calibration aerosol source (Maricq and Xu Citation2004; Ghazi et al. Citation2013; Rissler et al. Citation2013). Among them, the Combustion Aerosol Standard (CAST) generator and the more compact mini-CAST (Jing Citation1999) are the most widely used (Schindler et al. Citation2004; Cossette et al. Citation2010; Gysel et al. Citation2012). The operating principle of the CAST generator is based on the quenching of a diffusion propane flow using nitrogen. While this premature treatment of the flame facilitates sampling, it also freezes the soot formation process at an early stage raising concerns regarding the physicochemical resemblance of the produced particles to that of automotive exhaust aerosols. Some studies though, have actually identified strong relationships between CAST operating conditions and the morphology of the produced soot particles (Konstandopoulos et al. Citation2011).
Despite the widespread use of the CAST and the mini-CAST, a detailed characterization of the properties of the produced combustion particles is still lacking. In this study, we have performed dedicated measurements to investigate the influence of the mini-CAST operating parameters on the characteristics of the produced soot. Seven mini-CAST operational conditions (set points) were selected, producing similar mobility weighted size distributions. Measured properties included the effective density, organic-elemental carbon (EC) partitioning, affinity for butanol, and photoacoustic responses. Selected samples were also collected for a more detailed morphology characterization with Transmission Electron Microscopy (TEM) and soot structure analysis using Raman Spectroscopy, the results of which will be presented in detail elsewhere. This research was conducted to support the development of a combustion aerosol generator that can simulate engine exhaust. However, the collected results also provide valuable information for atmospheric aerosol studies, since CAST aerosol is also employed for the calibration of black carbon (BC) measuring techniques (Gysel et al. Citation2012).
EXPERIMENTAL
In this section, we describe briefly the main components of the experimental setup employed in the measurement campaign. More detailed information can be found in Section A of the online supplemental information.
Aerosol Generation
Soot particles were produced in a mini-CAST burner (model 5200, Jing Ltd.) configured with an external Mass Flow Controller (MFC – model PMF-52, Jing Ltd.). In the mini-CAST the oxidation air is supplied coaxially to the stream of the fuel (propane), resulting in a diffusion flame (Jing Citation1999). A flow of nitrogen (up to 10 NlpmFootnote 1 ), quenches the diffusion flame, freezing the soot formation process at a stage were soot concentration is rather high. The quenched particle stream can be further diluted by means of mixing with conditioned air (up to 20 Nlpm).
In the configuration tested, the burner can operate with propane flows up to 0.15 Nlpm and oxidation synthetic air of up to 3 Nlpm. The primary means of controlling the peak of the particle size distribution for a given propane/air mixture is by premixing the propane with nitrogen (with an adjustable nitrogen flow between 0 and 0.5 Nlpm).
In total seven operating conditions were tested which are listed in . The selection was based on the desire to produce aerosols of similar size distributions (geometric mean diameter of ∼80 nm and geometric standard deviation of ∼1.6 – ) using overally lean, stoichiometricFootnote 2 , and rich mixtures. A 5 Nlpm of synthetic air was employed at all conditions tested to provide sufficient oxygen for efficient removal of volatile species by the Catalytic Stripper (CS) (see below).
TABLE 1 Operating conditions and properties (elemental carbon content, EC, geometric mean mobility diameter, dg , and standard deviation, sg ; estimated primary particle size, dpp , experimentally determined mass mobility exponent, Dm , and effective particle density at 85 nm, ρe@85 ) of the aerosol produced at different mini-CAST operating conditions
The combustion aerosol produced in the mini-CAST was subsequently treated in a CS () optimized for minimum particle losses at the total mini-CAST flow rate (14–15.5 Nlpm) (Khalek Citation2007). It was constructed from a ceramic monolith with an alumina/zeolite washcoat that utilized platinum as the precious metal catalyst. The CS was externally heated to achieve an outlet temperature of 300°C (wall temperature of ∼350°C).
FIG. 1 Schematic of the experimental setups employed for the characterization of mini-CAST particles. (Color figure available online.)
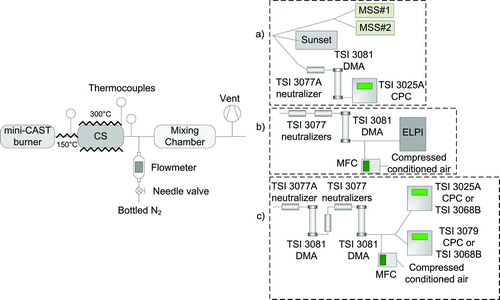
A 60 Nlpm nitrogen flow was added downstream of the CS (providing an additional dilution of ∼5:1) to cool the aerosol and minimize particle losses. An ageing chamber provided a residence time of approximately 10 s and allowed sufficient mixing before particle sampling.
Aerosol Instrumentation
Number-Weighted Mobility Size Distribution
A TSI 3934 Scanning Mobility Particle Sizer (SMPS) was employed to monitor the number-weighted mobility size distributions (). It incorporated a 85Kr neutralizer of ∼6 mCi activity (7 years old 10 mCi source – TSI 3077A) and a TSI 3081 Differential Mobility Analyzer (DMA). The number concentrations of the size-classified particles were measured with a TSI 3025A Condensation Particle Counter (CPC). The DMA operated at sheath and sample flow rates of 15 and 1.5 lpm and a scan time of 300 s, covering a mobility size range of 6–240 nm. The SMPS signals were inverted using TSI's AIM 9.0 software, which also takes into account diffusion losses inside the instrument. The size classification accuracy of the DMA was verified at the beginning of the experimental campaign using polystyrene latex spheres of 100 nm nebulized in a BGI Collison atomizer.
Organic/Elemental Carbon Partitioning
A Sunset Semi-Continuous Organic Carbon(OC)/EC Aerosol Analyzer (model 3F) was also employed to quantify the OC and EC fractions of the produced combustion aerosol (Birch and Cary Citation1996) (). Samples were collected on a quartz filter and subsequently subjected to the NIOSH 5040 thermal-optical protocol (NIOSH Citation1999). In the NIOSH 5040 protocol the filter is initially exposed to a helium environment at four different temperature steps (namely 250, 500, 650, and 850°C) where the different OC fractions are desorbed from the filter and subsequently oxidized to Carbon Dioxide (CO2) in a manganese dioxide oven. The amount of CO2 released, and therefore, the OC content, is measured with a nondispersive infrared analyzer. In a second temperature cycle, the residual EC is oxidized in a helium-oxygen carrier gas and quantified in the same way as OC. At the end of each run, a known volume of methane is injected and analyzed, to serve as an internal calibration standard. The instrument also quantifies the amount of carbon char produced by pyrolitic conversion of OC, by means of monitoring the laser transmittance through the filter during the analysis. Some samples were also collected upstream of the CS to assess the performance of the latter in removing volatile species. The accuracy of the Sunset Analyzer in quantifying the amount of Total Carbon (TC) was verified regularly during the campaign using sucrose solutions at five concentration levels covering the TC masses measured (∼10 to 40 ug) during the mini-CAST experiments (less than ±10% difference).
Light Absorption
Two AVL Micro Soot Sensors (MSS) were also employed sampling in parallel during the campaign (). The MSS operates on the photoacoustic principle. The aerosol passes through a resonator cell were it is exposed to an intensity-modulated 808 nm laser beam (at the resonance frequency of the cell) that is preferentially absorbed by soot particles leading to a periodic heating and cooling of the surrounding carrier gas (Schindler et al. Citation2004). This is manifested as a periodic sound wave which is measured by a sensitive microphone. Accordingly, the response of the MSS is proportional to the light absorption coefficient and concentration of the particles, i.e., BC mass concentration, and is calibrated against the insoluble mass fraction of CAST particles (Schindler et al. Citation2004). Each MSS instrument was connected to a conditioning unit providing a fixed dilution ratio of 3:1 at the sampling point. This dilution with air was necessary in order to avoid large changes in the speed of sound of the carrier gas (being predominantly N2 at the sampling point) that could affect the cell resonance frequency (Fiedler and Hess Citation1989) and accordingly the MSS response (less than ∼2.5% effect at the dilution setting of 3:1 employed – Section B in the supplemental information). The diluted samples were transferred to the measurement cells through 2 m long heated (at 47°C) sampling lines. The two MSS units were calibrated against the manufacturer's certified absorption windows and their responses were corrected for the different zero levels.
Affinity for Butanol
The affinity of mini-CAST particles for butanol was assessed by means of measuring their size-dependent detection efficiencies in a TSI 3790 CPC fully compliant with the European PN regulations (CitationUNECE Regulation 83). The low saturation ratios employed in this particular model, constitutes its detection efficiency very sensitive to particles of different affinity for butanol (Giechaskiel et al. Citation2011). A Tandem DMA setup () was employed in these experiments to minimize multiply charged artifacts that otherwise could be significant over the size range examined (25–60 nm) given the relatively large peak diameter of the assessed mini-CAST aerosols (∼80 nm). The two DMAs (both TSI 3081) operated at sheath and sample flow rates of 15 and 1.5 lpm, respectively. The first DMA was equipped with a ∼6 mCi activity 85Kr neutralizer while the second DMA employed a ∼1.2 mCi and a ∼1 mCi 85Kr neutralizers (model TSI 3077) in series. The size classified particles (with single charged particles constituting more than 97% of the total charged fraction) were then mixed with 1 Nlpm purified (dehumidified, HEPA filtered) air supplied by a MFC and their number concentration measured with the TSI 3790 CPC and a TSI 3025A CPC sampling in parallel. The 3025A CPC served as a reference counter as it had a nominal 100% counting efficiency over the investigated size range (23–50 nm). The slopes of the two CPCs were determined through calibrations against a TSI 3068B electrometer. For these calibrations, we employed monodisperse mini-CAST particles in the 80–160 nm size range, produced with the same Tandem-DMA setup with the electrometer replacing one of the CPCs ().
Effective Density
The effective density of the produced particles was measured in dedicated experiments using a TSI 3081 DMA and an Electrical Low Pressure Impactor (ELPI) in tandem (Maricq and Xu Citation2004) (). The ELPI operated on normal impactor stages without an electrical filter stage. Particles were first neutralized with two TSI 3077 bipolar chargers (with radioactive strength of ∼1.2 and a ∼1 mCi) connected in series and then classified in a TSI 3081 DMA, operating at sheath and sample flow rates of 10 and 1 lpm, respectively. The classified particles were mixed with conditioned make-up air supplied by a MFC at 8.5 Nlpm before entering the ELPI.
The effective densities were calculated following the procedure described by Maricq and Xu (Citation2004). In brief, the ELPI responses were simulated using the instrument Kernel functions (Marjamäki et al. Citation2005), that in addition to impaction account for secondary collection mechanisms and specifically, diffusion, image charge and space charge losses. Given that the primary collection mechanism (impaction) depends on the aerodynamic particle size, an estimate of the effective particle density (ρe ) is required to translate mobility diameter (dB ) to aerodynamic (da ) (Kelly and McMurry Citation1992; Equation (S5) in the supplemental information):
RESULTS
Volatility
summarizes the organic fraction speciation of mini-CAST aerosol as determined with the Sunset analyzer. For these particular tests, some samples were also collected directly at the outlet of the mini-CAST (Section A in the supplemental information), in order to assess the performance of the CS. The OC is apportioned into four fractions based on the amount of CO2 released over the four OC stage temperature ramps (21–250°C, 250–500°C, 500–650°C, and 650–850°C), corrected for the pyrolyzed material based on the laser transmittance dip at each temperature step.
FIG. 2 OC fraction of PM samples collected upstream (grey borderlines) and downstream (black borderlines) of the CS at the different operating conditions of the mini-CAST. The four stacked bars illustrate the fractions of OC desorbed at the four temperature steps in Helium environment, corresponding to peak temperatures of 250, 500, 650, and 850°C.
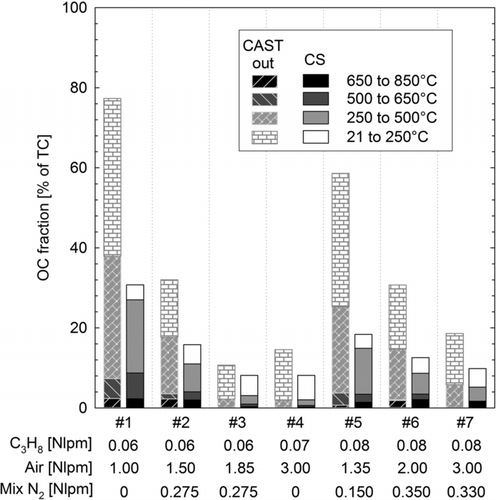
The EC content of the particles that were not treated in the CS ranged from as low as 23% to as high as 89%. The addition of oxidation air at a given propane flow rate was found to reduce the relative quantity of the desorbed OC and at the same time increase its volatility. When the air flow exceeded the chemically correct amount (conditions 3, 4, and 7), nearly 80% of the OC was desorbed at 250°C. At all other conditions, approximately half of the OC was released above 250°C. No clear effect of the amount of nitrogen diluting the propane flow (mix N2) could be identified. Similar trends were observed in the tests of Saathoff et al. (Citation2004) with the original CAST burner, who reported OC fractions of as high as 80% at overall rich mixtures, with nearly half of them being released at temperature above 350°C.
As expected, the efficiency of the CS depended on the volatility of the OC, removing up to 96% of the more volatile fraction released in the 21–250°C temperature range. The removal efficiency of the CS for this fraction is somehow underestimated owing to some gas adsorption artifacts (most probably from material previously adsorbed on the walls of the ageing chamber), verified when filtering the stream sampled by the Sunset Analyzer (Section C in the supplemental information). The CS was also found to remove 30%–60% of the less volatile OC released in the 250–500°C temperature range. A limited number of additional tests conducted at condition 6 (not presented in ), with the CS operating at an elevated wall temperature of 500°C effectively removed 97% of this less volatile fraction.
Effective Density and Primary Particle Size
summarizes the effective densities determined with the Tandem DMA-ELPI setup for mini-CAST particles produced at the different settings examined. The right-hand panel also includes measured effective densities for emery oil droplets, employed to validate the methodology (the experimental setup is described in Section A of the supplemental information). The retrieved emery oil densities averaged at 815 kg/m3 over all sizes examined (60–260 nm), with individual differences from the bulk value of 819 kg/m3 confined to less than ±7%. These differences are within the estimated experimental error of ±12% over the same size range reported by Maricq and Xu (Citation2004).
FIG. 3 Measured effective densities of emery oil (star symbols) and different mini-CAST particles. The curves illustrate the fitted effective particle densities of DLCAs using the mobility-primary particle size fit suggested by Sorensen (Citation2011) (as described in Section D of the supplemental information). The primary particle size (dpp ) that best reproduced the different experimental data is shown next to the curves. For the calculations, a material density of 2 g/cm3 was assumed for soot.
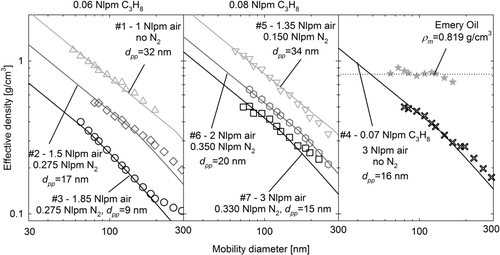
The measured effective densities of mini-CAST particles were found to exhibit an approximately power-law decrease with increasing mobility diameter, indicative of fractal-like structures. Exponents of the power-law fits that best reproduce the experimental data ranged between 0.71 and 0.9. These figures translate to mass-mobility exponentsFootnote 3 of 2.1 (3–0.9) to 2.31 (3–0.71) (), which are indicative of Diffusion Limited Cluster Aggregates (DLCAs) exhibiting a fractal dimension of ∼1.8 (Sorensen Citation2011). Similar mass-mobility exponents were determined in experimental studies on premixed ethylene soot (2.15 ± 0.1 in Maricq and Xu Citation2004), diesel (2.2 ± 0.1 in Maricq and Xu Citation2004; 2.33–2.41 in Park et al. Citation2003; 2.22–2.48 in Olfert et al. Citation2007), and direct injection gasoline (2.35 ± 0.1 in Maricq and Xu Citation2004) exhaust aerosols.
The effective density values while within the range of published data, were found to be strongly affected by the operating conditions of the mini-CAST. In absolute terms, the effective density varied from as high as 0.9 g/cm3 (condition 5) to as low as 0.3 g/cm3 (condition 3) at 85 nm, decreasing in magnitude with increasing the amount of oxidation air.
These large differences in the absolute levels of the effective densities are indicative of differences in the size of primary particles composing the aggregate. Recently, Sorensen (Citation2011) reviewed studies addressing the mobility of DLCAs and derived a fit relating mobility diameter with primary particle size (dpp ) and number of primary particles (Npp ). As shown in Section D of the supplemental information, this fit allows for an estimation of the primary particle diameter from the measured effective particle densities. also includes the effective particle density curves calculated from this analysis, for the primary particle sizes that best reproduce our experimental data. The fitted primary particle sizes ranged from as low as 8.7 nm at condition 3 to as high as 34.1 nm at condition 5. The resulting curves were found to capture very well the shape of the experimental data up to 200 nm, with individual differences being smaller than the experimental uncertainty of ±12%. At larger mobility diameters and fitted primary sizes smaller than 20 nm, the difference grows larger as under these conditions the low pressure of the ELPI leads to an overestimation of the effective particle density (Section D in the supplemental information).
Such large differences in the primary particle size were also verified in some TEM samples collected at the three operating conditions at 0.08 Nlpm C3H8 flow (). An analysis of more than 100 aggregates at each operating condition (presented in detail elsewhere – Seong et al. Citation2013), suggested an average primary size decreasing from 32 nm to 11 nm as the air flow rate increased from 1.5 Nlpm to 3 Nlpm. These figures are in relatively good quantitative agreement with the estimates from the Tandem DMA-ELPI data (34 nm and 15 nm, respectively).
FIG. 4 TEM images of particles produced at operating conditions 5 (0.08 Nlpm C3H8, 1.35 Nlpm air), 6 (0.08 Nlpm C3H8, 2 Nlpm air), and 7 (0.08 Nlpm C3H8, 3 Nlpm air).
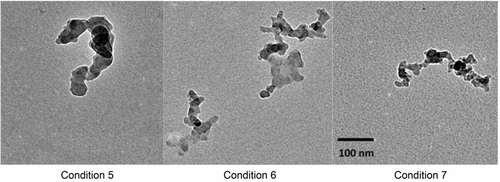
FIG. 5 Counting efficiencies of the TSI 3790 CPC against emery oil (star symbols) and different mini-CAST particles. The certification values by TSI, determined using emery oil, are also shown (crosses).
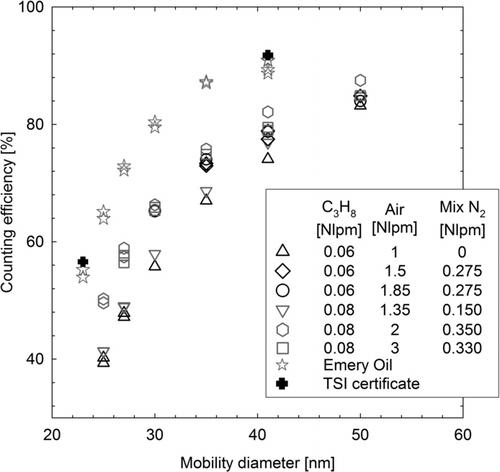
FIG. 6 Comparison of the total particle mass determined with the Sunset analyzer (assuming a hydrogen/carbon ratio of 1.85:1), the airborne particle mass calculated by convoluting the measured number weighted size distributions with the corresponding effective density profiles (), the EC mass determined with the Sunset Analyzer and the mass concentrations recorded by the two MSS. An example case for G-DI exhaust particles is also included in the chart.
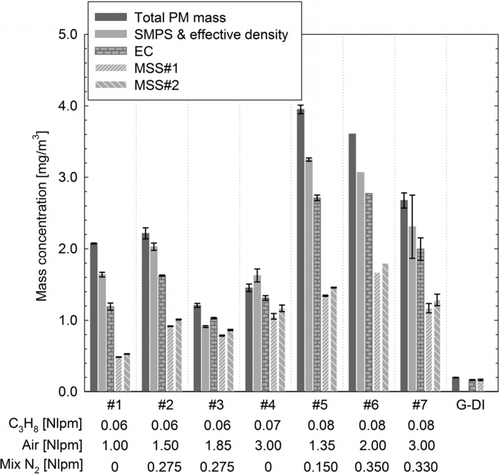
Affinity for Butanol
summarizes the experimentally determined counting efficiencies of the TSI 3790 for emery oil and mini-CAST particles. The measured emery oil efficiencies agreed to within −1% to −5% of the calibration certificate values. Mini-CAST particles were less efficiently detected by the CPC, in good agreement with what has been reported in the literature (Wang et al. Citation2010; Giechaskiel et al. Citation2011). More interestingly though, the data revealed that the counting efficiencies also depended on the operating conditions of the mini-CAST. More specifically, the particles produced at conditions 1 and 5 (Table 1) were less effectively detected from those produced at all other operating points examined. The difference in counting efficiency ranged from −17% to −22% at 25 nm to −6% to −12% at 35 nm and the results were practically indistinguishable above 50 nm. These two operating conditions also gave considerably larger primary particle sizes pointing towards a morphology effect on the activation energy barrier (i.e., affecting the contact line Mamakos et al. Citation2013). Differences in the chemistry of the particle surface and/or adsorbed volatile material could also have contributed to the different affinity for butanol.
Light Absorption
summarizes the mass concentrations determined with the two MSS to the EC concentration quantified with the Sunset Analyzer. The figure also includes the total airborne particle mass determined by convoluting the SMPS distributions with the effective density profiles () and the total PM mass derived from the Sunset Analyzer assuming a hydrogen/carbon ratio of 1.85:1 for the organic fraction. All mass concentrations were expressed at normal conditions (0°C and 1 atm) for these comparisons.
The indications of the two MSS units differed by ∼8% irrespective of the test condition, a figure which according to the manufacturer lies within the maximum expected calibration uncertainty of 10%. Both units though, were found to systematically underestimate the EC concentrations. In five out of the total seven conditions examined, the concentrations detected by the MSS was nearly half (40%–60%) of the thermooptically determined EC. The difference was reduced to 16%–24% and 11%–20% at conditions 3 and 4, respectively, but no clear association with a single mini-CAST operating parameter could be identified for this improved correlation.
Repetition of the tests (not shown in ) at condition 6 with the CS operating at 500°C (that removed 97% of the semivolatile material desorbed in the 250–500°C – ), did not improve the agreement (difference remained at ∼40%). Therefore, the reduced sensitivity of the MSS was not related to the presence of semivolatile species. It is also highly unlikely that this difference originates from uncertainties in the partitioning of the EC/OC content of the PM, specific to the temperature protocol used (Chow et al. Citation2001). Analysis of samples collected at conditions 4 and 6, using the IMRPOVE protocol (that reaches up to 500°C in helium environment) yielded equivalent results (less than ±2% difference) to those determined following the NIOSH protocol employed in the main campaign.
The observed discrepancy between the MSS and the EC, rather reflects differences in the absorption properties of particles produced at different mini-CAST operating conditions. The mass absorption coefficient of soot particles is known to depend on their structural properties and specifically on the amount of graphitization (Bond and Bergstrom Citation2006) which strongly depends on the type of flames and the particle residence times in the flames. The differences in the primary particle size of the particles () could also have contributed somehow on the apparent differences in the light absorption coefficient (Bond and Bergstrom Citation2006).
A limited number of tests conducted using G-DI exhaust (using a similar setup described in details in Section A of the supplemental information) suggested a much better agreement, with the difference being less than ±8% over three test repetitions. This is in good agreement with published data on both G-DI (Khalek et al. Citation2010) and diesel (Mamakos et al. Citation2011) exhaust, where the MSS is reported to agree with the EC concentrations to within ±10%. Since all MSS units are calibrated by the manufacturer against CAST aerosol (Schindler et al. Citation2004), it is anticipated that through appropriate selection of the operating flows the mini-CAST burner can also produce particles that better reproduce the light absorption characteristics of automotive exhaust.
The SMPS-derived mass was generally found to lie in between the EC and total PM masses determined with the Sunset Analyzer, as expected given that the determined effective particle densities also account for the volatile material contained in the particles. This consistency between SMPS-derived particle mass and the Sunset Analyzer results, come to further verify that the systematic deviations observed between the two MSS and the EC results are related to the absorption characteristics of the soot aerosol.
DISCUSSION
The operating conditions of the mini-CAST generator were found to have a strong effect on the physicochemical properties of the produced particles. Conditions characterized by low propane flows and a relative abundance of oxygen (e.g., points 3 and 4), led to freshly emitted particles exhibiting high EC content and high light absorption. By increasing the fuel rate and/or decreasing the oxidation air flow, an increasing amount of semivolatile species that survived the CS, was forming. This behavior is indicative of a rather early quenching of the flame, freezing the soot particles at a premature state exhibiting distinctly different light absorption properties.
The differences in the light absorption properties of the produced particles were reflected in the relative differences between the BC emissions measured with the MSS and the thermo-optically determined EC, which ranged from −10% to −60%. The better agreement observed for G-DI exhaust (±8%) suggests that there should exist operating conditions of the mini-CAST that better reproduce the light absorption properties of engine exhaust, since the MSS is calibrated by the manufacturer against CAST aerosol. Research is currently underway to characterize the light absorption properties of mini-CAST aerosol over a wider range of operating conditions.
The effective density measurements suggested similar mass mobility exponents (∼2.2) at all conditions tested, characteristic of diffusion limited fractal aggregates. However, large differences were observed in the absolute value of the effective density which ranged between 0.32 and 1.05 g/cm3 at the geometric mean mobility diameter (∼80 nm) of the seven settings examined. These differences are indicative of differences in the primary particle size which was estimated to range between 9 and 34 nm, in good agreement with what observed in a limited number of TEM images analyzed. These results also illustrate that the generally overlooked absolute value of the effective density may actually contain more valuable information on particle morphology than the widely employed mass-mobility exponent.
The operating conditions of the mini-CAST were also found to affect the affinity of the generated particles for butanol, manifested in large differences in the detection efficiencies of a TSI 3790 CPC, reaching ∼20% at the 50% cut-off size. Giechaskiel et al. (Citation2009) have also reported large differences in the counting efficiencies of TSI 3790 CPC at two operating conditions of a Heavy-Duty diesel engine, even though to a certain extent this was attributed to experimental uncertainties. This evidence raises concerns regarding the traceability of CPC calibrations against combustion aerosol. On the other hand, the counting efficiencies for emery oil, while not representative of combustion soot, reproduced the manufacturer's certified values to within 4%, in good agreement with other published data (Giechaskiel and Bergmann Citation2011; Mamakos et al. Citation2013).
The presence of semi-volatile material under certain operating conditions also highlights the importance of a thorough conditioning of the aerosol especially for the calibration of Volatile Particle Remover (VPR) used for the regulation of solid PN emissions in Europe. Unless a very efficient approach is employed to completely remove such species (e.g., a CS operating at elevated temperatures), the size, and shape of particles may change inside the VPR depending on the design and operating conditions of the evaporating tube (especially the wall temperature and residence time).
Overall, the results obtained in this study highlight the importance of an appropriate selection of the mini-CAST settings through a comprehensive characterization of the produced aerosol properties. Considering the different types of CAST burners available (at least four models are currently commercialized covering different mass flow rates), there is a need for a more fundamental understanding of the process of flame quenching and its effect on the properties of produced aerosol. This will help identify suitable operating conditions depending on the targeted application and will allow researchers to use this system consistently in reporting their results and/or for calibration purposes.
Supplemental_Information.zip
Download Zip (3 MB)Acknowledgments
The authors would like to acknowledge Richard Mechler, Daniel Preece, and Vinay Premnath for their assistance in setting up the experimental setup. The authors would also like to thank Dr. Matti Maricq for his thorough and careful review of our article and his insightful recommendations. Dr. H. Seong and Dr. L. O. Kyeong from Argonne National Laboratory are gratefully acknowledged for the collection of TEM samples and their analysis. Athanasios Mamakos would like to thank Dr. Yannis Drossinos for the fruitful discussions on the description of fractal particles. This work was funded by EPA under Contract No. GS-07F-6087P.
[Supplementary materials are available for this article. Go to the publisher’s online edition of Aerosol Science and Technology to view the free supplementary files.]
Notes
Nlpm stands for liters per minute at normal conditions (0°C and 1 atm).
The air flow rates corresponding to chemically correct mixtures at 60, 70, and 80 mlpm. C3H8 are 1.5, 1.75, and 2 lpm, respectively.
A number of studies have associated the fractal dimension with the exponent of power-law curves fitted to mass (or effective density) versus mobility diameter. This assumption though is not valid, as shown by Sorensen (2011), leading to an overestimation of the true fractal dimension (and also quantified in Section D of the supplemental information).
REFERENCES
- Berg , W. 2003 . Legislation for the Reduction of Exhaust Gas Emissions . Handbook of Environment Chemistry , 3 ( Part T ) : 175 – 253 .
- Birch , M. E. and Cary , R. A. 1996 . Elemental Carbon-Based Method for Monitoring Occupational Exposures to Particulate Diesel Exhaust . Aerosol Sci. Technol. , 25 : 221 – 241 .
- Biskos , G. , Mastorakos , E. and Collings , N. 2004 . Monte Carlo Simulation of Unipolar Diffusion Charging for Spherical and Non-Spherical Particles . J. Aerosol Sci. , 35 : 707 – 730 .
- Bond , T. C. and Bergstrom , R. W. 2006 . Light Absorption by Carbonaceous Particles: An Investigative Review . Aerosol Sci. Technol. , 40 : 27 – 67 .
- Boucher , T. L. , Khalek , I. A. , Laroo , C. A. and Bischnu , D. K. 2012 . Determination of the PEMS Measurement Allowance for PM Emissions Regulated Under the Heavy-Duty Diesel Engine In-Use Testing Program . SAE Int. J. Eng. , 5 : 1371 – 1386 .
- Chow , J. C. , Watson , J. G. , Crow , D. , Lowenthal , D. H. and Merrifield , T. 2001 . Comparison of IMPROVE and NIOSH Carbon Measurements . Aerosol Sci. Technol. , 34 : 23 – 34 .
- Cossette , G. , Speilvogel , J. , Carli , S. , Usarek , S. , Brandt , L. Keck , L. Comparison of two Condensation Particle Counters for Automotive Applications using a Combustion Soot Generator . 29th AAAR Conference, 25–29 October 2010 , Portland, Oregon , , USA
- EC . 2007 . “ Commission Regulation 715/2007 ” .
- EC . 2009 . “ Commission Regulation 595/2009 ” .
- EC . 2012 . “ Commission Regulation 459/2012 ” .
- Fiedler , M. and Hess , P. 1989 . “ Laser Excitation of Acoustic Modes in Cylindrical and Spherical Resonators: Theory and Applications ” . In Photoacoustic, Photothermal and Photochemical Processes in Gases, Topics in Current Physics Vol. 46 , 85 – 123 .
- Ghazi , R. , Tjong , H. , Soewono , A. , Rogak , S. R. and Olfert , J. S. 2013 . Mass, Mobility, Volatility, and Morphology of Soot Particles Generated by a McKenna and Inverted Burner . Aerosol Sci. Technol. , 47 : 395 – 405 .
- Giechaskiel , B. and Bergmann , A. 2011 . Validation of 14 Used, Re-Calibrated and New TSI 3790 Condensation Particle Counters According to the UN-ECE Regulation 83 . J. Aerosol Sci. , 42 : 195 – 203 .
- Giechaskiel , B. , Mamakos , A. , Andersson , J. , Dilara , P. , Martini , G. Schindler , W. 2012 . Measurement of Automotive Nonvolatile Particle Number Emissions within the European Legislative Framework: A Review . Aerosol Sci. Technol. , 46 : 719 – 749 .
- Giechaskiel , B. , Wang , W. , Gilliland , D. and Drossinos , Y. 2011 . The Effect of Particle Chemical Composition on the Activation Probability in n-Butanol Condensation Particle Counter . J. Aerosol Sci. , 42 : 20 – 37 .
- Giechaskiel , B. , Wang , W. , Horn , G. G. , Spielvogel , J. , Gerhart , C. Southgate , J. 2009 . Calibration of Condensation Particle Counters for Legislated Vehicle Number Emission Measurements . Aerosol Sci. Technol. , 43 : 1164 – 1173 .
- Gysel , M. , Laborde , M. , Mensah , A. A. , Corbin , J. C. , Keller , A. Kim , J. 2012 . Technical Note: The Single Particle Soot Photometer Fails to Reliably Detect PALAS Soot Nanoparticles . Atmos. Meas. Tech. , 5 : 3099 – 3107 .
- Jing , L. Standard Combustion Aerosol Generator for Calibration Purposes . 3rd ETH Conference on Combustion Generated Nanoparticles , Zurich 9–10 August, 1999
- Kelly , W. P. and McMurry , P. H. 1992 . Measurement of Particle Density by Inertial Classification of Differential Mobility Analyzer-Generated Monodisperse Aerosol . Aerosol Sci. Technol. , 17 : 199 – 212 .
- Khalek , I. A. 2007 . Sampling System for Solid and Volatile Exhaust Particle Size, Number, and Mass Emissions . SAE Int. J. Fuels Lubr. , 116 : 122 – 133 .
- Khalek , I. A. , Bougher , T. and Jetter , J. J. 2010 . Particle Emissions from a 2009 Gasoline Direct Injection Engine Using Different Commercially Available Fuels . SAE Int. J. Fuels Lubr. , 3 ( 2 ) : 623 – 637 .
- Konstandopoulos , A. G. , Daskalos , E. and Vlachos , N. The Microstructure of Soot Aggregates Produced by the Combustion Aerosol Standard (CAST) Generator . 15th ETH-Conference on Combustion Generated Nanoparticles , Zürich , , Switzerland June 26–29
- Mamakos , A. , Bonnel , P. , Perujo , A. and Carriero , M. 2012 . Assessment of Portable Emission Measurement Systems (PEMS) for Heavy-Duty Diesel Engines with Respect to Particulate Matter . J. Aerosol Sci. , 57 : 54 – 70 .
- Mamakos , A. , Carriero , M. , Bonnel , P. , Demircioglu , H. , Douglas , K. Alessandrini , S. 2011 . “ EU-PEMS PM Evaluation Program – Second Report - Study on Post DPF PM/PN Emissions ” . JRC Scientific Technical Report EUR 24793 EN
- Mamakos , A. , Giechaskiel , B. and Drossinos , Y. 2013 . Experimental and Theoretical Investigations of the Effect of the Calibration Aerosol Material on the Counting Efficiencies of TSI 3790 Condensation Particle Counters . Aerosol Sci. Technol. , 47 : 11 – 21 .
- Maricq , M. M. and Xu , N. 2004 . The Effective Density and Fractal Dimension of Soot Particles from Premixed Flames and Motor Vehicle Exhaust . J. Aerosol Sci. , 35 : 1251 – 1274 .
- Marjamäki , M. , Lemmetty , M. and Keskinen , J. 2005 . ELPI Response and Data Reduction I: Response Functions . Aerosol Sci. Technol. , 39 : 575 – 582 .
- Mathis , U. , Kaegi , R. , Mohr , M. and Zenobi , R. 2004 . TEM Analysis of Volatile Nanoparticles from Particle Trap Equipped Diesel and Direct-Injection Spark-Ignition Vehicles . Atmos. Environ. , 38 : 4347 – 4355 .
- Mathis , U. , Mohr , M. , Kaegi , R. , Bertola , A. and Boulouchos , K. 2005 . Influence of Diesel Engine Combustion Parameters on Primary Soot Particle Diameter . Environ. Sci. Technol. , 39 : 1887 – 1892 .
- NIOSH . 1999 . “ Method 5040 Issue 3: Elemental Carbon (Diesel Exhaust) ” . In NIOSH Manual of Analytical Methods. National institute of Occupational Safety and health Cincinnati , OH
- Olfert , J. S. , Symonds , J. P. R. and Collings , N. 2007 . The Effective Density and Fractal Dimension of Particles Emitted from a Light-Duty Diesel Vehicle with a Diesel Oxidation Catalyst . J. Aerosol Sci. , 38 : 69 – 82 .
- Park , K. , Cao , F. , Kittelson , D. B. and McMurry , P. H. 2003 . Relationship between Particle Mass and Mobility for Diesel Exhaust Particles . Environ. Sci. Technol. , 37 : 577 – 583 .
- Pope , C. A. III , Burnett , R. T. , Thun , M. J. , Calle , E. E. , Krewski , D. Ito , K. 2002 . Lung Cancer, Cardiopulmonary Mortality, and Long-term Exposure to Fine Particulate Air Pollution . J. Am. Med. Assoc. , 287 ( 9 ) : 1132 – 41 .
- Rissler , J. , Messing , M. E. , Malik , A. I. , Nilsson , P. T. , Nordin , E. Z. Bohgard , M. 2013 . Effective Density Characterization of Soot Agglomerates from Various Sources and Comparison to Aggregation Theory . Aerosol Sci. Technol. , 47 : 792 – 805 .
- Ristimäki , J. , Virtanen , A. , Marjamäki , M. , Rostedt , A. and Keskinen , J. 2002 . On-Line Measurement of Size Distribution and Effective Density of Submicron Aerosol Particles . J. Aerosol Sci. , 33 : 1541 – 1557 .
- Saathoff , H. , Blatt , N. , Gimmler , M. , Linke , C. and Schurath , U. Thermographic Characterisation of Different Soot Types . 8th ETH Conference on Combustion Generated Particles, 14–16 September, 2004 , Zurich , , Switzerland
- SAE Standard AIR589 . Nonvolatile Exhaust Particle Measurement Techniques . E-31 Aircraft Exhaust Emissions Committee , Version 2012-03-07
- Schindler , W. , Haisch , C. , Beck , H. , A. , Niessner , R. , Jacob , E. and Rothe , D. 2004 . “ A Photoacoustic Sensor System for Time Resolved Quantification of Diesel Soot Emissions ” . SAE Technical Paper 2004-01-0968
- Seong , H. , Lee , K. , Mamakos , A. , Khalek , I. A. , Giannelli , R. and Spears , M. Morphology and Nanostructures of Particles from an Engine Simulating Particle Generator . 23rd CRC Real World Emissions Workshop, 7–10 April , San Diego , , USA
- Sorensen , C. M. 2011 . The Mobility of Fractal Aggregates: A Review . Aerosol Sci. Technol. , 45 : 765 – 779 .
- Tzamkiozis , T. , Ntziachristos , L. , Mamakos , A. , Fontaras , G. and Samaras , Z. 2011 . Aerodynamic and Mobility Size Distribution Measurements to Reveal Biodiesel Effects on Diesel Exhaust Aerosol . Aerosol Sci. Technol. , 45 : 587 – 595 .
- UNECE Regulation 83
- UNEP/WMO . 2011 . Integrated Assessment of Black Carbon and Tropospheric Ozone. United Nations Environment Programme and World Meteorological Organization . Summary for Decision Makers ,
- USEPA . 2008 . Code Of Federal Regulations 40, part 1065
- USEPA . 2010 . Notice of Alternate System Approval. CISD-10–22. October 19, 2010
- VAMV . 2006 . Verordnung des EJPD über Abgasmessgeräte für Verbrennungsmotoren (VAMV) vom 19 März 2006
- Wang , X. , Caldow , R. , Sem , G. J. , Hama , N. and Sakurai , H. 2010 . Evaluation of a Condensation Particle Counter for Vehicle Emission Measurement: Experimental Procedure and Effects of Calibration Aerosol Material . J. Aerosol Sci. , 41 : 306 – 318 .
- Weijer , E. P. , Schaap , M. , Nguyen , L. , Matthijsen , J. Denier van der Gon , H. A. C. 2011 . Anthropogenic and Natural Constituents in Particulate Matter in the Netherlands . Atmos. Chem. Phys. , 11 : 2281 – 2294 .