Abstract
We propose a significant correction to be applied on the modeling that appears in the article “Modeling and Validation of Nanoparticle Charging Efficiency of a Single-Wire Corona Unipolar Charger” by Chien et al., published in Aerosol Science and Technology 45(12), pages 1146–1179. It concerns the boundary condition based on Peek’s law, because the geometry of the main component of the corona charger is a needle and not a wire. The effects of adopting a more realistic modeling could be very important. Boundary conditions are a key factor in the resolution of the present problem, because they affect the results a lot. This could explain why the modeling results in that article do not fit very well the experimental ones. Our proposed modification could improve them.
Copyright 2014 American Association for Aerosol Research
In the referenced article (Chien et al. 2011), the analysis of a corona charger by comparing experimental data to numerical simulations is carried out. They affirm that the ions are generated by using a “single-wire” electrode.
Actually, the title of the article should use “needle” instead of “wire,” since the behavior of the two objects is quite different. Electrically, it would be a wire if it were clamped at both ends. In the present case, it is more correct to call it a needle.
The numerical analysis of the problem done in the cited article is quite extensive and exhaustive regarding to the fluid analysis, well combined with the Poisson equation and the charge convection along the region of study.
However, the approach of a boundary condition for the ionic charge density, essential for the simulation, is not completely correct, with potentially important effects on the final results.
The mentioned boundary condition is based on the fact that the electric field in a conductor when corona starts, Eonset, is the one resulting from the Peek’s law (Adamiak and Atten Citation2004), expressed in Equation (10) of the referenced article, which is valid for wires:
[1] where Eonset has units of kV/cm, r is the radius of the wire in cm, and δ is a dimensionless correction factor for temperature and pressure.
The previous expression is approximately valid for other geometries when using an equivalent radius of curvature, re (Adamiak and Atten Citation2004). For example, for a hemispherical needle tip re = 0.5rw, being rw the radius of the cylindrical part.
In the referenced article, the shape of the tip of the needle is not given. It could be one of the shapes A, B, or C, shown in .
Case A is the smoothest, with re = 0.5rw. Cases B and C have equivalent radii of curvature, smaller than that of case A, therefore the corona onset fields verify:
[2] It seems, consequently, that the corona discharge occurs easily in the cylindrical part, then in the case A, and so on. However, not only the onset field has to be taken into account, but also the actual electric field due to the distribution of charges on the conductor surface.
In electrostatic equilibrium (just before the onset occurs), for any two points 1 and 2 on the conductor surface, it is followed approximately (Plonus Citation1978):
[3] where E represents the modulus of the electric field and r the curvature at the point of study.
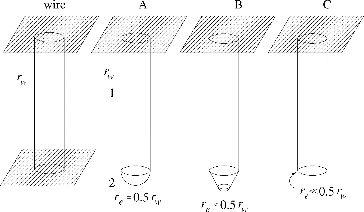
The reality is more complex, and there is no universal formulation to solve the problem (Liu Citation1987; Dubé et al. Citation1989; Torres et al. Citation1989). In any case, points with small radius of curvature will have large fields, since their charge density is larger.
Equation (Equation3[3] ) shows that the electric field increases inversely proportional to r at any point of a conductor surface, while Peek’s law (Equation1
[1] ) implies that the corona onset field increases inversely proportional to the square root of r. Therefore, it will be reached before the boundary imposed by the Peek’s equation by geometries with smaller r.
Using the Peek’s law (Equation1[1] ) with the simplest geometry (case A) to create a discharge at the tip of the needle, we have (with δ = 1)
[4]
[5]
On the other hand, from (Equation3[3] ), it must be fulfilled EA2 ≈ 2E1A, since the equivalent curvature is reduced to the half at the tip. Following the assumptions of the original article, when E1 reaches the value of (Equation4
[4] ), then it should be EA2 = 420 kV/cm, much greater than that given by (Equation5
[5] ).
On the contrary, if the limit imposed by the Peek’s law, given by (Equation5[5] ), is reached at the tip of the needle, then
, and thus corona discharge is not produced in the lateral side of the needle (the “wire” part).
This effect is even more evident in cases B and C: the limit of the Peek’s law is always reached sooner at the tip than on the cylindrical part of the needle electrode, since the ionic charge generated at the tip of the needle is much greater than that generated on the side of the needle (negligible).
Therefore, this demonstrates that the corona effect occurs only at the tip of the electrode in the case discussed in the article. Some real examples certifying this can be found in several photos appearing in Okuyama and Fujimoto (Citation1985).
Nonetheless, Chien et al. could check the exact shape of the tip, for using the correct curvature radius in the Peek’s law, with the aim of improving the results of the modeling.
The drag flow may cause that certain ions are transported along the lateral side of the needle, but since the base of the needle is placed at a point that stops the flow stream, this contribution appears to be minimal (although calculations must be done). Thus, the charge concentration maps shown in Figures 7c and d of Chien et al. (Citation2011) would not be completely valid: there exists higher concentration of charge at the tip than along the side of the electrode.
Note that the numerical model of Chien et al. (Citation2011) is based on a previous model of Lin and Tsai (Citation2010), which is developed for wires clamped at both ends, thus not showing a tip. In this second case, the model is obviously more accurate, because the Peek’s law is correctly applied.
In addition, Equation (9) of the referenced article is valid only for wires but not for needle tips, therefore, the charge density, ρi, 0, at the tip cannot be certainly known. Another equation is needed to replace that one, and in any case, a significant increase in the number of mesh points in the numerical simulation near the tip of the needle is needed.
For needles it is not possible to know a priori the electric field and the injected charge on the injecting electrode. A methodology, which uses the Kaptzov’s assumption (the electric field at every electrode point remains constant at the value predicted by the Peek’s law, by adequately increasing the injected charge), can be iteratively used, as exposed in Khaddour et al. (Citation2006) and more developed in Khaddour (Citation2006). A similar method is exposed in Adamiak and Atten (Citation2004).
Following Khaddour procedure, by using dimensionless variables (see the cites before for the details), the charge density near the electrode is calculated by
[6] where A is a large constant (Khaddour proposes a value of 100 or higher). Equation (Equation6
[6] ) must be iterated, recalculating the electric field at the electrode surface, E(surfacepoints), until convergence (within some level of accuracy, e.g., 0.1% or less with dimensionless variables). This methodology replaces Equation (9) of the referenced article.
These considerations in the simulation could explain the relatively large errors between the simulations and the experimental data shown, for example, in of the article.
In conclusion, we consider that the approach with a boundary condition given by the Peek’s law for wires, combined with Equation (9) from the referenced article, is inaccurate and the simulation should be repeated with the assumptions herein. Then the new results could be compared with the experimental ones. Nevertheless, we think this modification would improve significantly the modeling in Chien et al. (Citation2011), making it more realistic.
REFERENCES
- Adamiak, K., Atten, P. (2004). Simulation of Corona Discharge in Point-Plane Configuration. J. Electrostat., 48: 85–98.
- Chien, C.-L., Tsai, C.-J., Chen, H.-L., Lin, G.-Y., Wu, J.-S. (2011). Modeling and Validation of Nanoparticle Charging Efficiency of a Single-Wire Corona Unipolar Charger. Aerosol Sci. Technol., 45: 1468–1479.
- Dubé, M., Morel, M., Gauthier, N., Barrett, A.J. (1989). Comment on “Relation Between Charge Density and Curvature of Surface of Charged Conductor.”Am. J. Phys., 57: 1047–1048.
- Khaddour, B. (2006). Modélisation du Champ Électrique Modifié Par la Charge D’espace Injectée. Ph.D. Thesis, Institut National Polytechnique de Grenoble (INPG), France.
- Khaddour, B., Atten, P., Coulomb, J.-L. (2006). Electrical Field Modified by Injected Space Charge in Blade-Plate Configuration. IEEE Trans. Magn., 42: 651–654.
- Lin, G.-Y., Tsai, C.-J. (2010). Numerical Modeling of Nanoparticle Collection Efficiency of Single-Stage Wire-in-Plate Electrostatic Precipitators. Aerosol Sci. Technol., 44: 1122–1130.
- Liu, K.-M. (1987). Relation Between Charge Density and Curvature of Surface of Charged Conductor. Am. J. Phys., 55: 849–852.
- Okuyama, F., Fujimoto, Y. (1985). Formation Mechanism of the So-called Corona Figure. IEEE Trans. Plasma Sci., PS-13: 10–15.
- Plonus, M. (1978). Applied Electromagnetics. McGraw-Hill, New York.
- Torres, M., González, J.M., Pastor, G. (1989). Comment on “Relation Between Charge Density and Curvature of Surface of Charged Conductor.”Am. J. Phys., 57: 1044–1046.