ABSTRACT
The majority of agricultural and forest production systems are typically simplified systems and therefore thought to be sensitive to disturbance. Ecosystems might be less sensitive to disturbance if complexity increases. The resource concentration hypothesis predicts that complexity makes it more difficult for herbivores to find and exploit their food. The natural enemy hypothesis predicts less damage in diverse environments because enemy abundance and diversity remain higher and more stable in environments providing more alternative food sources. We explore the effect of tree diversity in two types of pine stands differing in tree diversity. We ask whether European pine sawfly (Neodiprion sertifer) larval group distribution and larval mortality, imposed by specialist natural enemies, is affected by tree diversity. We find fewer larval groups on pine trees surrounded by non-host trees, indicating that fewer egg-batches were laid. Mortality rates by specialist enemies are not affected by tree diversity but parasitism rates are likely to be higher in larger trees. Herbivores are inhibited by increased tree diversity and density, whereas natural enemies seem to be unaffected, partly increasing the resistance of more diverse forest stands against insect damage. Thus, managing for more mixed forests could mitigate risk of herbivore damage.
Introduction
Agricultural and forest production systems are typically simplified systems and therefore thought to be sensitive and prone to disturbance. Pimentel (Citation1961) observed that insect pest outbreaks most often happen in simplified ecosystems. Currently, managed forests often are monocultures, thus typical representatives of simplified ecosystems. Therefore, changing forest management method should benefit the provision of ecosystem services, like natural control of insect pests (Schuler et al. Citation2017) and create forests that will withstand or have the capacity to recover from biotic and abiotic disturbances (Björkman et al. Citation2015). In forestry, mixed stands have become an attractive alternative to the prevailing monoculture forestry, partly as a response to the demand to better fulfill multiple goals in modern forestry (Felton et al. Citation2016).
The effect of forest management related to natural disturbances, like pest insect or pathogen attacks, are often considered in the context of increasing species diversity, where an overall positive effect is found (Jactel et al. Citation2017). Recent meta-analyses show that in both agricultural (Crowder and Jabbour Citation2014) and forestry systems (Jactel et al. Citation2005; Jactel and Brockerhoff Citation2007; Castagneyrol et al. Citation2014) the damage caused by phytophagous insects is lower in more diverse systems in comparison to more simplified systems. The ecological theory behind the positive effects of diversity is the concept of “Associational Resistance” (Root Citation1973), which encompasses two potential mechanisms by which diversity could lead to increased resistance to disturbance. This theory can be applied in forest management using the assumption that increased diversity in the managed forest will lead to an increase in forest resistance and resilience against insect pests (Klapwijk et al. Citation2016).
The concept of associational resistance can be separated into two hypotheses (Tahvanainen and Root Citation1972; Root Citation1973). The first is the resource concentration hypothesis, proposing that the probability of a phytophagous insect locating and remaining on a potential host is reduced when non-host plants surround the potential host plant (Underwood et al. Citation2014), either through the “dilution effect” or through the chemical or physical masking of the focal plant (Hamback et al. Citation2014). The mechanisms underlying associational resistance could comprise neighbor plant effects on the survival or behavior of herbivores and their natural enemies on the focal plant, plant-plant competition altering focal plant traits and differences in relative abundance of focal and neighboring plants (Barbosa et al. Citation2009).
The natural enemy hypothesis (Root Citation1973) states that increased diversity will increase natural enemy pressure due to the increased presence of alternative prey, in turn, increasing natural enemy density and diversity (Stiling et al. Citation2003). Thus, increased diversity at the plant level will increase the diversity of higher trophic levels, as alternative food resources and alternative prey are thought to be present in higher abundance in more diverse habitats (Riihimäki et al. Citation2005).
Evidence mounting in forestry systems shows the potential positive effect of resource diversity on the control of phytophagous pest insects through increased associational resistance linked to effects on natural enemies. Parasitism pressure on larvae of the forest tent caterpillar (Malacosoma disstria) in Canada is lower in monoculture aspen stands compared to forest stands with multiple tree species (Nixon and Roland Citation2012). Empirical research conducted in the Vale of York, UK, has shown that Ichneumonid parasitoid abundance and diversity is higher in woodlands with increased numbers of deciduous tree species (Fraser et al. Citation2007). It is also found that increased tree diversity benefits generalist an egg parasitoid, Trichogramma minutum, by providing alternative hosts for this multi-voltine parasitoid species (Kemp and Simmons Citation1978) attacking the spruce budworm (Choristoneura fumiferana). Understanding the basic conditions underlying both the “resource concentration” and the “natural enemy” hypothesis will lead to more informed predictions concerning when these hypotheses will and will not apply.
One of the mechanisms involved is the searching behavior of both herbivorous insects and natural enemies and how these are affected by tree diversity in a forest stand. Both the herbivore and its natural enemies need to navigate through “landscapes” at various scales with different levels of complexity. Searching for host plants or prey is a multi-step process, which involves searching for suitable patches, identifying host trees and accepting or rejecting the potential host (Hassell and Southwood Citation1978). Theoretically, it is shown that patch immigration rates depend on host location strategies based on either patch size (number of host plants or size of the host plant) or cues (e.g. volatiles) that indicate the amount of resource available (Andersson et al. Citation2013). The search strategy is proposed to be hierarchical at a spatial scale; first, the host plant is located and second, the prey is located within the prey host plant (Vinson Citation1976). This means that the structure of the habitat surrounding the host plant could affect the success with which herbivores locate their host tree (Dulaurent et al. Citation2012) and also the success with which the prey is located by predators, for example, parasitoids (Bezemer et al. Citation2010).
In order to understand whether or not using mixed stands, instead of monocultures, will inhibit the herbivore and benefit the natural enemy, we set out to collect data reflecting the behavior of an herbivore and its natural enemies in either diverse (mixed stands; i.e. more than one species) or simple (monoculture) forest stands. We use a specialist herbivore, Neodiprion sertifer Geoff., and its fairly specialized natural enemies, i.e. parasitoids, and compare their effectiveness in host use (pine trees and prey, N. sertifer larvae, respectively) in the two different types of forest stands.
The present study was conducted at two different locations in Sweden; both locations suffered outbreak densities of N. sertifer. The main aim of the study was to assess the effect of monoculture versus mixed species stands (i.e. tree diversity) on herbivore presence and parasitism rates in two different types of forest stands dominated to a various extent by Scots pine (Pinus silvestris L.). The difference between the two types of stands, from the perspective of the pine-specialized herbivore (N. sertifer), was the abundance of non-host tree species in the stands (mostly birch, spruce, juniper). Based on previous research, parasitism rates could be expected to be higher in the more diverse stands, i.e. stands where in addition to pine trees also other tree species like birch and rowan are present (Fraser et al. Citation2007; Jactel & Brockerhoff Citation2007; Nixon and Roland Citation2012). But within stands, parasitism rates should be higher in trees that are easier to locate, i.e. not hidden by non-host trees (Bezemer et al. Citation2010). The following questions are being asked:
Does higher tree diversity at stand level affect the number of pine sawfly larval groups and their parasitism rates?
How does the tree diversity (number of tree species) surrounding a host tree affect the number of pine sawfly larval groups in the tree and their parasitism rates?
From these two questions follows the expectation that both larval group distribution and parasitism rates are affected by the presence of non-host trees, and that the number of larval groups could be expected to be lower and that the parasitism rates could be expected to be higher in stands with non-host trees present.
Materials and methods
Study sites
The study was carried out at two different localities, in two different years in Sweden (). Both localities suffered high (outbreak) densities, when the study was performed. The first locality is 40 km North from Uppsala, central Sweden, where data was collected in 4 sites that are relatively close together (distance between 0.3 and 3 km). This locality will be referred to as “Uppland” (central point; 60°11.015′N, 17°29.056′E). The second locality is referred to as “Småland” (central point; 57°24.870′N, 15°25.383′E), located in southern Sweden, approximately 300 km south from Uppsala, where four sites included in the study were relatively far apart (distance min 19 km and max 69 km). In Småland, the outbreak of N. sertifer, during the time of the data collection, had an area of approx. 200,000 ha, started in 2008 and ended in 2011. The Småland area has a relatively large proportion of mixed forest stands. At both localities, the mixed forest stands are associated with a more herbaceous understory, e.g. grasses and ferns. In the monoculture pine stands, the understory is mostly dominated by lichen, lingonberry and bilberry. The stands in Uppland had typically a higher tree density compared to Småland (; (A)). The average percentage pine trees (number of pine trees / total number of trees) around the focal trees in high diversity sites in Småland is 63% (0.63 ± 0.32; mean ± standard deviation) and in low diversity sites 93% (0.93 ± 0.27). In Uppland, the percentage pine in low diversity sites is 86% (0.86 ± 0.26) and in high diversity sites 71% (0.71 ± 0.24). When a stand has more than 80% pine it is usually classified as a monoculture, i.e. low diversity, stand (Toumey and Korstian Citation1947). The most common deciduous trees were birch, rowan and aspen, other coniferous non-host tree species are spruce (common) and juniper (less common). Generally, the presence of coniferous non-host trees is higher in the Småland compared to Uppland but only in the high diversity sites.
Figure 1. Map of Sweden with the locations Uppland and Småland and the distribution of the sites within the locations. The scale of the maps and inlays are given in kilometres.
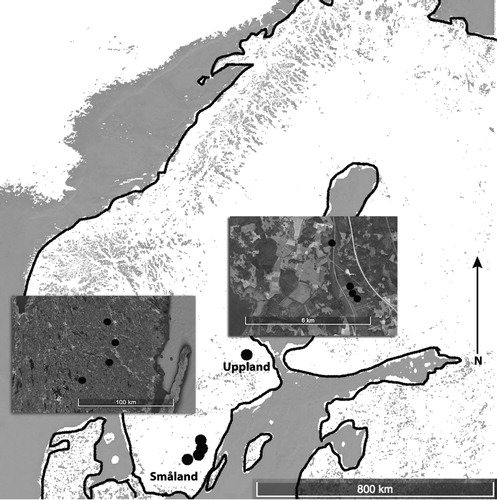
Figure 2. Interval plots for the variables tree density, number of pine trees, number of non-host trees and DBH per site, mean and 95% confidence interval. The sites from Uppland are represented by the gray symbols and the sites from Småland are represented by the black symbols. The light grey symbols represent the raw data. (A) Average number of trees in 4 m diameter around the focal tree; (B) The number of pine trees in the 4 m diameter around the focal tree; (C) The number of deciduous trees in 4 m diameter around the focal tree; (D) The number of non-host (deciduous and coniferous) trees in 4 m diameter around the host tree; (E) The number of larval groups counted in the focal tree; (F) Parasitism rates of the collected group of larvae in the focal tree.
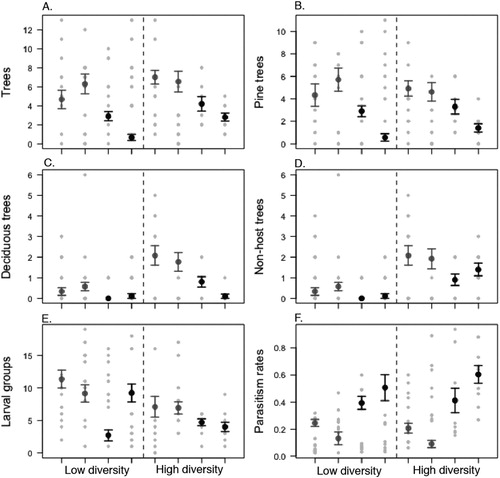
Table 1. The mean and standard error for each stand (low and high diversity) for the total number of trees, presence of non-host, deciduous and pine trees in the 4 m diameter around the focal tree, for the size of the focal trees measured in diameter at breast height (DBH; cm), the number of larval groups in the focal tree and parasitism rates (calculated as number of parasitoid emerged divided by the total successfully emerged individuals).
Ecology of Neodiprion sertifer Geoff. (Hymenoptera: Diprionidae)
The European pine sawfly, N. sertifer, a specialist on pine, (Pinus spp) is a uni-voltine species that exhibits irregular outbreak dynamics mostly in Scots pine stands in Sweden. The adults hatch in late August and early September after the summer diapause, and females lay their eggs in a single oviposition event, usually close to the location at which they emerged. The eggs are laid on the needle flush of the current year in a batch of 30 – 120 eggs (Gur’yanova Citation2006) regularly distributed over several pairs of needles on one shoot. The larvae hatch during late April and early May. Once hatched, they commence feeding on the needles that their eggs were laid on and then continue feeding on the previous years’ needles. They will only feed on the new flush of needles when the tree has otherwise been totally defoliated. Between the end of June and the beginning of July, the larvae go through their final ecdysis and become pre-pupae that will leave the tree and find a place in the litter or soil to pass through the summer diapause and undergo pupation, which happens in the beginning of August. There is accumulated information on specialist parasitoids (Pschorn-Walcher Citation1965, Citation1967; Griffiths Citation1975; Pschorn-Walcher Citation1987), predation by ants (Olofsson Citation1992), cocoon predation by small mammals (Hanski and Parviainen Citation1985; Hanski Citation1987; Olofsson Citation1987; Kouki et al. Citation1998), the impact and transmission of nuclear polyhedrosis virus (NPV) during outbreaks (Olofsson Citation1989a, Citation1989b) and mortality factors in general (Olofsson Citation1987). The species is distributed over North America and Eurasia and exhibits periodical outbreaks over its entire range.
Pine sawfly and parasitoid data collection
Uppland 2009
In each site, 15 trees were haphazardly selected with the main criterion that a larval group would be present. The minimal distance between the two pairs of trees was 5 m and the maximal distance was 235 m. At least 50 larvae in fourth or fifth instar, were collected per selected tree by collecting the larvae dropping from the tree after beating the tree stem, using this method allowed for the collection of larvae potentially originating from different larval groups in the same tree. The larvae were taken back to the laboratory and reared until spinning of the cocoons. In September, the number hatched sawflies were counted, as well as the hatched parasitoids. The remaining cocoons were placed outside sheltered from precipitation to facilitate successful overwintering of the remaining parasitoids/sawflies. In spring, the hatched parasitoids/sawflies were counted again and the cocoons of which sawfly or parasitoid had not successfully emerged were dissected to determine the fate of the sawfly.
Småland 2011
In each site, up to 10 trees were haphazardly selected with at least one larval group present. The minimal distance between selected trees within a site was 5 m and the maximum difference was 150 m. At fourth instar, the larval group was covered with mesh sleeve cover and after spinning of the cocoons the whole group was collected and taken back to the laboratory. The same procedure for rearing the cocoons was followed as for the larvae collected from Uppland.
Stand variables collected
For the selected trees the diameter at breast height (DBH) was measured and the number of larval groups in the tree was counted. This is easily done at the beginning of larval feeding as first instar larvae leave very distinct curled, dried remains of the needles on which the eggs hatched and the newly hatched larvae have fed.
For each tree, the number of trees in a diameter of 4 m (area of 13 m2) was counted and the representation of tree species was registered, divided into pine, non-host coniferous (typically juniper and spruce) and non-host deciduous trees (most often birch).
Based on the presence of deciduous trees, the sites were grouped into two categories based on “low” or “high” tree diversity (B; (C,D)). The number of pine trees surrounding the focal tree and the size of the focal trees was not different between sites (). Thus, stand type, low and high diversity stands, can be used as an explanatory variable to compare the effects on herbivores and natural enemies. To estimate the within-stand effect of the total of trees in the 4 m diameter around the focal tree was used as a measure of tree density. Within-stand tree diversity was determined by the number of deciduous trees and non-host coniferous trees in the 4 m diameter around the focal tree. As the number of larval groups in a tree could be related to size (DBH) of the tree, we also calculated the de-trended number of larval groups in a tree by subtracting the predicted values of the regression between the number of larval groups and DBH from the observed values (Crawley Citation2012, p. 791).
Data analyses
The differences in larval group numbers and parasitism rates are compared for the low and high diversity forest stands to test if there is a difference between stands with different tree diversity. In addition to these analyses, we test within-stand difference by using the total number of pine, non-host coniferous and deciduous trees and the total number trees in the 4 m diameter around the focal trees in multiple regressions.
Among-stand effect of diversity
We compared the different locations for the number of larval groups in the focal tree and parasitism rates (response variables) by using generalized linear mixed models with penalized quasi-likelihood (glmmPQL; package MASS in R; Venables and Ripley Citation2002) with explanatory variables stand diversity (high and low) and location (Uppland and Småland). Site identity was included as a random grouping variable. We used de-trended number of larval groups for DBH to eliminate the effect of DBH on the number of larval groups in a tree and again parasitism rates. For the larval group data, a Poisson distribution (Crawley Citation2012, p. 561) was used as it is an analysis of count data. For the parasitism rates, a Binomial distribution (Crawley Citation2012, p. 560) was used as the data are recorded in a number of successes (hatched parasitoids) and failures (number of hatched sawflies). The minimum adequate model was obtained using the principles of model simplification.
Within-stand effects of diversity
For this analysis, we used generalized linear mixed models with penalized quasi-likelihood again (glmmPQL; package MASS in R; Venables and Ripley Citation2002) with site-identity nested in location included as random grouping factor. To estimate the effect of within-stand tree diversity the larval group numbers (count; Poisson) and parasitism rates (proportion; binomial) were used as response variable in the model. The DBH, number of pine and non-host trees surrounding the focal tree and all possible interactions were included as explanatory variables in the model and possible interactions. To separate the effect of tree species, we ran the model again with the number of pine, non-host coniferous and deciduous trees, and all possible interactions. The third model looked at the effect of the number of trees in the 4 m diameter around the focal tree (also referred to as tree density). The number of trees is the count of the trees surrounding the focal tree in a diameter of 4 m. For the last two models, de-trended larval group density was used to eliminate the effect of tree height (DBH). Similar models were run for the parasitism rates. All models included the number of larval groups in the tree. The first model included both DBH and the count of the larval groups as explanatory variable. The second and third model included the de-trended number of larval groups as explanatory variable. The minimum adequate model was obtained using the principles of model simplification.
Results
Among-stand effects of diversity
The locations do differ; the larval group densities are higher in Uppland compared to Småland and the parasitism rates are higher in Småland compared to Uppland (; ). For the larval group density in high diversity stands is lower (5.4 ± 0.4 larval groups per tree; mean ± std.error) compared to low diversity stands (8.3 ± 0.6 larval groups per tree). The interaction between diversity (high and low) and the location is not significant for either of the response variables.
Figure 3. Comparison of the two locations for (A) number of larval groups (mean and standard error of the mean) and (B) parasitism rates (mean and standard error of the mean). The sites from Uppland are represented by the gray symbols and the sites from Småland are represented by the black symbols. The light grey symbols represent the raw data ().
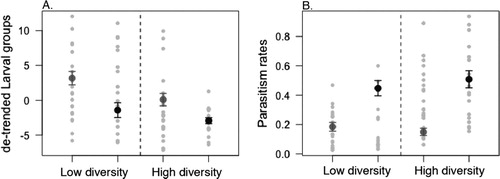
Table 2. Comparison for the locations for the different levels of tree diversity using generalized linear mixed model (glmmPQL; package MASS in R; Venables and Ripley Citation2002) with number of larval groups de-trended for DBH as response with a gaussian error distribution or parasitism rates and a binomial error distribution (i.e. estimates are given in logits).
Within-stand effects of diversity
The number of pine trees surrounding the focal tree negatively affected the larval group density in the focal tree. (A; (A)). The interaction between the number of non-host trees and DBH indicates that large pine trees surrounded by many non-host trees will have more larval groups compared to a small tree surrounded by the same number of non-host trees.
Figure 4. The relationship between (A) The number of larval groups in a tree plotted against DBH, the size of the symbols represents the number of non-host trees in a 4 m diameter around the focal tree (A); (B) the number of larval groups in a tree (de-trended for DBH) plotted against the number of pine trees in a 4 m diameter. The size of the symbols depicts the number of non-host trees in the 4 m diameter (B); (C) the number of larval groups plotted against the total number of trees in 4 m diameter. The line represents the trend for the total dataset as estimated by the model (C). For all graphs, the black symbols represent the observations from the sites in Småland and gray symbols represent the observations from the sites in Uppland.
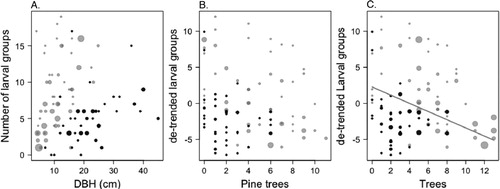
Table 3. Results of general mixed models (glmmPQL; package MASS in R; Venables and Ripley Citation2002) for the multiple regression with larval group density and parasitism rates as response.
When the larval group density was de-trended for the positive relationship with the size of the tree, the number of pine trees and the number of deciduous trees around the focal tree interact negatively (B; (B)), indicating an overall negative effect of tree density. Tested in a separate model, tree density reduces the number of larval groups in the focal tree (−0.57 ± 0.14 [slope ± std.error]; D; (C)).
Parasitism rates of larval groups showed a positive relation with the size of the tree (DBH). None of the other variables explains a significant part of the variation in parasitism rates. Much of the variability was explained by location and site (D; ). The second model with the de-trended number of larval groups, number of pine, the number of non-host coniferous trees and number of deciduous trees did not show any significant results (E), neither did tree density per se (F).
Figure 5. The relationship between parasitism rates (proportion) and the size of the tree measured in diameter at breast height (DBH). The symbols represent the fitted values for different locations (black symbols = Småland, gray symbols = Uppland). The regression line is an illustration of the trend in the fitted data for the total dataset (D).
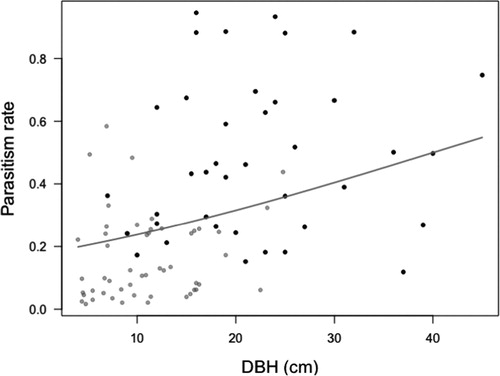
Discussion
The successful use of different forest management strategies to mitigate risk for insect damage very much depends on the ecological interactions and mechanisms that operate within-stands. Our results indicate that the presence of the specialist herbivore, N. sertifer, is lower in forest stands with higher numbers of non-host trees present in the stand. However, there is no evidence that parasitism rates are affected either negatively or positively by the number of non-host trees for N. sertifer (). In other words, the type of tree species in a stand negatively affects the herbivore but no response is found for parasitism rates. Similar patterns are found for within-stand diversity (A–F). Our results imply that diversification of forest systems, like increasing amount of mixed forests – both within- and among-stands in a landscape, could function to reduce the risk of insect damage through reducing herbivore attack. Increased number of tree species could mitigate risks for insect damage in forest stands.
In our study system, the effect of resource concentration, i.e. number of host trees, is confounded by the size of the host plant as has been found in other systems (Masumoto et al. Citation2000). The higher number of larval groups in larger trees could be a direct effect of the availability of resource (larger trees have more branches and can sustain more larval groups) on resource selection. Our results indicate that the effect of host tree size could affect the mechanisms of tree location by the sawfly females: large trees are more apparent; that this mechanism could be involved is confirmed by the significant interaction between the host tree size and the surrounding trees (non-host and pine). The response of the N. sertifer females to the size of the host tree could be interpreted as them using visual cues more than volatile cues when searching for oviposition sites (Jactel et al. Citation2011).
By removing the trend between a number of larval groups and host tree size, it became possible to assess the effects of tree diversity, showing that the number of non-host trees has a stronger negative effect on the colonization of N. sertifer compared to the negative effect of the number of pine trees. But when non-host trees were separated out in non-host coniferous trees and deciduous trees, many deciduous trees around the focal tree increased the larval groups in the trees but when there was a mixture of pine and deciduous trees, the number of larval groups in the focal tree was generally low ((B)).
As Underwood (Citation2004) showed, the distribution of the herbivores in a patch depends on the quality of the host plant as perceived by the herbivore. The dependence on host plant quality can be further moderated by the number and species of neighboring plants, resulting in associational effects (Barbosa et al. Citation2009). In our case, this means that the attractiveness of the focal tree could also depend on host plant quality. If the quality of the pine tree is negatively related to the number of trees (pine and non-pine) around the focal tree, it would mean that a focal tree surrounded by many trees would not only become less apparent but in addition, less likely be perceived as a suitable host plant.
Within a stand, the only important feature affecting parasitism rates was the size of the focal tree: larger trees – higher rates of parasitism. The parasitism rates did not differ significantly when the means in the stands are compared. A positive effect of forest mixture has been found for (other) generalist predators like ants and spiders (Kaitaniemi et al. Citation2007). Higher predation pressure by generalist predators has a positive effect on the regulation of pest populations. However, spiders and ants are less mobile within a site than parasitoids and the area of effect of ants reduces with distance from the ants nest (Olofsson Citation1992). Perhaps, the associational effect is stronger for generalist natural enemies than relatively specialized enemies, like parasitoids, because alternative food sources could be present in adjacent trees. For the more specialized enemies, like many parasitoids, host location in a more complex habitat could inhibit their efficiency (Gols et al. Citation2005). Parasitoids are mobile and will actively search for hosts to oviposit. Based on previous research, two patterns could be expected, 1) higher parasitism rates in more simple habitats (Bezemer et al. Citation2010) or 2) higher parasitism rates in the more heterogeneous habitat based on the “natural enemy hypothesis” (Castagneyrol et al. Citation2013). Our empirical data did not confirm or reject either of the expectations. We found a strong response to the size of the focal tree in parasitism rates, suggesting that plant apparency is not only important for host plant location in herbivorous insects but also in search patterns for parasitoids. This results aligns with the hierarchical searching hypothesis (Vinson Citation1976) and seems to indicate that, the larger the resource patch for the herbivore, the higher the risk of parasitism for individual larvae within a groups is.
Based on the optimal foraging theory, the oviposition effort should increase with searching effort (Krebs Citation1977). Thus, the parasitism rate of a larval host group should decrease when surrounded by multiple groups (Klapwijk and Lewis Citation2014), but no relationship with number of groups in a tree was found here. As N. sertifer larvae are attacked by a number of different species of parasitoids (Pschorn-Walcher Citation1965, Citation1967), interference and competition between individuals of different species might affect the efficiency of the complex as a whole (Doak and Wagner Citation2015). Throughout the life cycle of N. sertifer, mortality caused by parasitoids in the egg and larval stages, walking predators like spiders and ants in the early larval stages, and small mammals in the cocoon stage are found to be important predators. The predation rates of N. sertifer cocoons imposed by small mammals are known to be positively affected by forest structure (Hanski and Parviainen Citation1985; Kollberg et al. Citation2014; Bellone et al. Citation2017). Here we found that parasitoids seemed to be affected by tree size but not tree diversity. We could, therefore, hypothesize that the mortality rates imposed by specialist predators could be habitat independent as a result of their density-dependent prey-searching mechanism and the response of generalist predators could be positively affected by habitat structure as they are more opportunistic in their predation behavior.
Our data enabled us to investigate patterns in larval group densities and parasitism rates at two different locations at two different times. We investigated patterns between sites and the data is interpreted for differences for the types of stands at the different locations. The large distance between the sites (±300 km between Småland and Uppland) will level out any variation in insect population density over time. Even the slightly different ways of collecting parasitism data will not affect the overall patterns that we observe between the stand types at the different locations as the data has been collected the same at all sites within a location. However, using the collected data in this way has disadvantages, i.e. there is variation between sites and between years that might obscure potential patterns in parasitism rates due to variance within the sites and locations. However, the consequences of applying a forest management method will last for the whole rotation time, and the mechanisms should be rather general and consistent irrespective of when a phenomenon is studied. The patterns that we found apply to two locations and can, therefore, be expected to have a certain robustness.
We conclude that, in order to get the complete picture, the effects of increasing tree diversity should be investigated for different types of predators over the whole life cycle of a pest insect. Specialist predators and generalist predators could be expected to have different responses to an increase in the number of tree species in a stand. The specialist predators are not negatively affected by increasing the tree species and can therefore still be expected to have a numerical response to the density of their host. The positive response of the generalist predators to increased diversity needs to be investigated further to understand under what conditions those will be able to exert a stronger and more constant predation pressure on the herbivore host to reduce the possibility for rapidly increasing herbivore population densities.
Acknowledgements
The authors are grateful to the Swedish Forest Agency (Skogsstyrelsen), Gunnar Isacsson, and Sveaskog, Kjell Gustavsson, for help with identifying the research sites.
Disclosure statement
No potential conflict of interest was reported by the authors.
ORCID
Maartje J. Klapwijk http://orcid.org/0000-0001-7690-045X
Additional information
Funding
References
- Andersson P, Lofstedt C, Hamback PA. 2013. Insect density-plant density relationships: a modified view of insect responses to resource concentrations. Oecologia. 173:1333–1344. doi: 10.1007/s00442-013-2737-1
- Barbosa P, Hines J, Kaplan I, Martinson H, Szczepaniec A, Szendrei Z. 2009. Associational resistance and associational susceptibility: having right or wrong neighbors. Ann Rev Ecol Evol Syst. 40:1–20. doi: 10.1146/annurev.ecolsys.110308.120242
- Bellone D, Klapwijk MJ, Björkman C. 2017. Habitat heterogeneity affects predation of European pine sawfly cocoons. Ecol Evol. 7:11011–11020. doi: 10.1002/ece3.3632
- Bezemer TM, Harvey JA, Kamp AFD, et al. 2010. Behaviour of male and female parasitoids in the field: influence of patch size, host density, and habitat complexity. Ecol Entomol. 35:341–351. doi: 10.1111/j.1365-2311.2010.01184.x
- Björkman C, Bylund H, Nilsson U, Nordlander G, Schroeder M. 2015. Forest management to mitigate insect damage in a changing climate: possibilities and uncertainties. In: Björkman C, Niemelä P, editors. Climate change and insect pests. Wallingford: CABI; p. 248–266.
- Castagneyrol B, Giffard B, Pere C, Jactel H. 2013. Plant apparency, an overlooked driver of associational resistance to insect herbivory. J Ecol. 101:418–429. doi: 10.1111/1365-2745.12055
- Castagneyrol B, Jactel H, Vacher C, Brockerhoff EG, Koricheva J. 2014. Effects of plant phylogenetic diversity on herbivory depend on herbivore specialization. J Appl Ecol. 51:134–141. doi: 10.1111/1365-2664.12175
- Crawley MJ. 2012. The R book. Chicester: John Wiley & Sons. Ltd. doi: 10.1002/9781118448908
- Crowder DW, Jabbour R. 2014. Relationships between biodiversity and biological control in agroecosystems: current status and future challenges. Biol Control. 75:8–17. doi: 10.1016/j.biocontrol.2013.10.010
- Doak P, Wagner D. 2015. The role of interference competition in a sustained population outbreak of the aspen leaf miner in Alaska. Basic Appl Ecol. 16:434–442. doi: 10.1016/j.baae.2015.04.001
- Dulaurent AM, Porté AJ, Van Halder I, Vetillard F, Menassieu P, Jactel H. 2012. Hide and seek in forests: colonization by the pine processionary moth is impeded by the presence of nonhost trees. Agric For Entomol. 14:19–27. doi: 10.1111/j.1461-9563.2011.00549.x
- Felton A, Nilsson U, Sonesson J, et al. 2016. Replacing monocultures with mixed-species stands: ecosystem service implications of two production forest alternatives in Sweden. Ambio. 45:124–139. doi: 10.1007/s13280-015-0749-2
- Fraser SEM, Dytham C, Mayhew PJ. 2007. Determinants of parasitoid abundance and diversity in woodland habitats. J Appl Ecol. 44:352–361. doi: 10.1111/j.1365-2664.2006.01266.x
- Gols R, Bukovinszky T, Hemerik L, Harvey JA, Van Lenteren JC, Vet LEM. 2005. Reduced foraging efficiency of a parasitoid under habitat complexity: implications for population stability and species coexistence. J Anim Ecol. 74:1059–1068. doi: 10.1111/j.1365-2656.2005.01003.x
- Griffiths KJ. 1975. The biology of Lophyroplectus luteator (Hym.: Ichneumonidae) on Neodiprion sertifer (Hym.: Diprionidae) in Ontario. Entomophaga. 20:97–103. doi: 10.1007/BF02373455
- Gur’yanova T. 2006. Fecundity of the European pine sawfly Neodiprion sertifer (Hymenoptera, Diprionidae) related to cyclic outbreaks: invariance effects. Entomol Rev. 86:910–921. doi: 10.1134/S0013873806080069
- Hamback PA, Inouye BD, Andersson P, Underwood N. 2014. Effects of plant neighborhoods on plant-herbivore interactions: resource dilution and associational effects. Ecology. 95:1370–1383. doi: 10.1890/13-0793.1
- Hanski I. 1987. Pine sawfly population-dynamics – patterns, processes, problems. Oikos. 50:327–335. doi: 10.2307/3565493
- Hanski I, Parviainen P. 1985. Cocoon predation by small mammals, and pine sawfly population-dynamics. Oikos. 45:125–136. doi: 10.2307/3565230
- Hassell MP, Southwood TRE. 1978. Foraging strategies of insects. Annu Rev Ecol Syst. 9:75–98. doi: 10.1146/annurev.es.09.110178.000451
- Jactel H, Bauhus J, Boberg J, et al. 2017. Tree diversity drives forest stand resistance to natural disturbances. Curr For Rep. 3:223–243.
- Jactel H, Birgersson G, Andersson S, Schlyter F. 2011. Non-host volatiles mediate associational resistance to the pine processionary moth. Oecologia. 166:703–711. doi: 10.1007/s00442-011-1918-z
- Jactel H, Brockerhoff EG. 2007. Tree diversity reduces herbivory by forest insects. Ecol Lett. 10:835–848. doi: 10.1111/j.1461-0248.2007.01073.x
- Jactel H, Brockerhoff E, Duelli P. 2005. A test of the biodiversity-stability theory: meta-analysis of tree species diversity effects on insect pest infestations, and re-examination of responsible factors. In: Scherer-Lorenzen M, Korner C, Schulze ED, editors. Forest diversity and function: temperate and boreal systems. Berlin: Springer-Verlag; p. 235–262.
- Kaitaniemi P, Riihimaki J, Koricheva J, Vehvilainen H. 2007. Experimental evidence for associational resistance against the European pine sawfly in mixed tree stands. Silva Fenn. 41:259–268. doi: 10.14214/sf.295
- Kemp WP, Simmons GA. 1978. The influence of stand factors on parasitism of spruce budworm eggs by Trichogramma minutum. Environ Entomol. 7:685–688. doi: 10.1093/ee/7.5.685
- Klapwijk MJ, Bylund H, Schroeder M, Björkman C. 2016. Forest management and natural biocontrol of insect pests. Forestry. 89:253–262. doi: 10.1093/forestry/cpw019
- Klapwijk MJ, Lewis OT. 2014. Spatial ecology of host–parasitoid interactions: a threatened butterfly and its specialised parasitoid. J Insect Conserv. 18:437–445. doi: 10.1007/s10841-014-9653-5
- Kollberg I, Bylund H, Huitu O, Björkman C. 2014. Regulation of forest defoliating insects through small mammal predation: reconsidering the mechanisms. Oecologia. 176:975–983. doi: 10.1007/s00442-014-3080-x
- Kouki J, Lyytikainen-Saarenmaa P, Henttonen H, Niemelä P. 1998. Cocoon predation on diprionid sawflies: the effect of forest fertility. Oecologia. 116:482–488. doi: 10.1007/s004420050613
- Krebs J. 1977. Optimal foraging – theory and experiment. Nature. 268:583–584. doi: 10.1038/268583a0
- Masumoto T, Sunahara T, Suzuki N. 2000. Effects of nonhost and host plants on insect herbivory covarying with plant size in the cruciferous plant Turritis glabra. Popul Ecol. 42:145–152. doi: 10.1007/PL00011993
- Nixon AE, Roland J. 2012. Generalist predation on forest tent caterpillar varies with forest stand composition: an experimental study across multiple life stages. Ecol Entomol. 37:13–23. doi: 10.1111/j.1365-2311.2011.01330.x
- Olofsson E. 1987. Mortality factors in a population of Neodiprion sertifer (Hymenoptera. Diprionidae). Oikos. 48:297–303. doi: 10.2307/3565517
- Olofsson E. 1989a. Transmission agents of the nuclear polyhedrosis virus of Neodiprion sertifer (Hym, Dirpionidae). Entomophaga. 34:373–380. doi: 10.1007/BF02372476
- Olofsson E. 1989b. Transmission of the nuclear polyhedrosis virus of the European pine sawfly from adult to offspring. J Invertebr Pathol. 54:322–330. doi: 10.1016/0022-2011(89)90116-X
- Olofsson E. 1992. Predation by Formica polycenia Forster (Hym, formicidae) on newly emerged larvae of Neodiprion sertifer (Geoffroy) (Hym, Diprionidae). J Appl Entomol. 114:315–319. doi: 10.1111/j.1439-0418.1992.tb01132.x
- Pimentel D. 1961. Species diversity and insect population outbreaks. Ann Entomol Soc Am. 54:76–86. doi: 10.1093/aesa/54.1.76
- Pschorn-Walcher H. 1965. The ecology of Neodiprion sertifer (Geoffr.) (Hymenoptera: Diprionidae) and a review of its parasite complex in Europe. Commonwealth Inst Biol Contr Tech Bull. 5:33–97.
- Pschorn-Walcher H. 1967. Biology of the Ichneumonid parasites of Neodiprion sertifer in Europe. Commonwealth Inst Biol Contr Tech Bull. 8:7–51.
- Pschorn-Walcher H. 1987. Interspecific competition between the principal larval parasitoids of the pine sawfly, Neodiprion sertifer (Geoff.) (Hym.: Diprionidae). Oecologia. 73:621–625. doi: 10.1007/BF00379426
- Riihimäki J, Kaitaniemi P, Koricheva J, Vehviläinen H. 2005. Testing the enemies hypothesis in forest stands: the important role of tree species composition. Oecologia. 142:90–97. doi: 10.1007/s00442-004-1696-y
- Root RD. 1973. Organization of a plant-arthropod association in simple and divers habitat - fauna of collards (Brassica oleracea). Ecol Monogr. 43:95–124. doi: 10.2307/1942161
- Schuler LJ, Bugmann H, Snell RS. 2017. From monocultures to mixed-species forests: is tree diversity key for providing ecosystem services at the landscape scale? Landscape Ecol. 32:1499–1516. doi: 10.1007/s10980-016-0422-6
- Stiling P, Rossi AM, Cattell MV. 2003. Associational resistance mediated by natural enemies. Ecol Entomol. 28:587–592. doi: 10.1046/j.1365-2311.2003.00546.x
- Tahvanainen JO, Root RB. 1972. The influence of vegetational diversity on the population ecology of a specialized herbivore, Phyllotreta cruciferae (Coleoptera: Chrysomelidae). Oecologia. 10:321–346. doi: 10.1007/BF00345736
- Toumey J, Korstian C. 1947. Foundations of silviculture upon an ecological basis. New York: John Wiley & Sons, Inc.
- Underwood N. 2004. Variance and skew of the distribution of plant quality influence herbivore population dynamics. Ecology. 85:686–693. doi: 10.1890/03-0030
- Underwood N, Inouye BD, Hamback PA. 2014. A conceptual framework for associational effects: when do neighbours matter and how would we know? Q Rev Biol. 89:1–19. doi: 10.1086/674991
- Venables WN, Ripley BD. 2002. Modern applied statistics with S. New York: Springer Science & Business Media. doi: 10.1007/978-0-387-21706-2
- Vinson S. 1976. Host selection by insect parasitoids. Annu Rev Entomol. 21:109–133. doi: 10.1146/annurev.en.21.010176.000545