Introduction
Until recently, traditional radiobiology was based on the theory that the effects of radiotherapy present themselves only locally, through direct and indirect DNA damage [Citation1]. Recently, after reports on radiation effects outside the irradiated volume, the local effects were denominated as targeted effects, and the distant ones as non-targeted effects [Citation2]. There are two types of non-targeted effects, depending on the site of their occurrence and the relationship between the irradiated and non-irradiated tumor:
The Radiation-Induced Abscopal Effect (RIAE) is a systemic effect of local radiation extended outside the treated volume. Basically, this means that tumor regression is observed at distant untreated sites.
The Radiation-Induced Bystander Effect (RIBE) is a radiobiological effect-transmission that happens when irradiation of only a part of the tumor induces regression of the whole tumor.
Both phenomena have only been sporadically clinically observed, as they were occasional and unintentional. That said, is it possible to induce them intentionally, whenever needed? We have spent the past 7 years testing new ideas in attempts to induce an intentional induction of non-targeted effects from ionizing radiation.
In this letter, we describe the first clinical experience of inducing bystander and abscopal effects by exclusively targeting the hypoxic segment of the tumor with a high, single dose of radiation. The experience confirms the validity of this strategy, and could be the start of a new era in radiotherapy.
Translation of riae/ribe results from pre-clinic to clinic
In vitro, radiation-conditioned medium-transfer experiments using the A-549, H-460 human lung cancer cells, and their hypoxic clones, created for this purpose were behind the clinical studies [Citation3]. Cells were irradiated in normoxic or hypoxic conditions with 10 Gy single doses and the cell growth and survival were monitored by a real time cell electronic sensing system and a colony forming assay, respectively. The studies showed strong correlations between the tumor oxygen status and the RIAE/RIBE-intensity, indicating that the hypoxic tumor segment could be a key-factor for the intentional induction of the non-targeted effects of radiation. Furthermore, some results indicated that partial irradiation of the tumor could be even more effective in inducing strong RIAEs/RIBEs.
Target definition
A simulation computed tomography (CT) scan was fused with a positron emission tomography (PET) with 2-deoxy-2-[fluorine-18]fluoro-d-glucose (18F-FDG PET) in combination with contrast enhanced CT for the definition of the hypoxic tumor segment, the so called ‘bystander tumor volume’ (BTV) ()). Each BTV was defined individually. First, by using the contrast-CT, we divided the tumor into three segments:
contrast—enhanced (vascularized) peripheral tumor segment,
contrast—unenhanced (non-vascularized, necrotic) tumor segment,
contrast—hypoenhanced (hypovascularized) tumor segment as an up to a maximum of 5 mm junctional zone between the central-necrotic and the remaining peripheral-vascularized tumor segments.
Figure 1. Radiobiology of the bystander effect. (A, B) The figure summarizes the main features of the reported radiation technique for the induction of the bystander effect by partially irradiating only the hypoxic tumor segment. An 18F-FDG PET in combination with a contrast enhanced CT, previously fused with the simulation CT, was used for the definition of the hypoxic tumor segment, a so called ‘bystander target’ (a smaller contour). This area, which includes the junctional region between the necrotic tumor segment (central region) and the contrast-enhanced and PET-positive remainder peripheral tumor (a bigger contour that was not targeted for irradiation), was targeted with high-dose radiation. The arrows represent the bystander signals (pellets) released by the irradiated hypoxic segment, which transmit damage to the remainder of the un-irradiated part of the tumor, causing regression of the whole tumor, both in the targeted and non-targeted region.
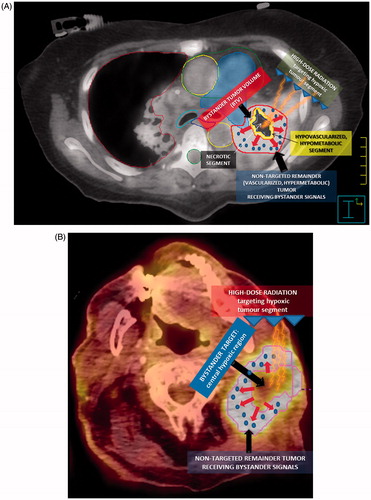
Second, PET was used for definition of a hypometabolic junctional zone between the necrotic tumor segment and the peripheral hypermetabolic tumor segment. A Standardized Uptake Value (SUV) of 3 defined the boundary since a steep increase at a SUV of around 3–4 was seen moving from the necrotic toward the peripheral hypervascularized tumor. This volume was then subtracted from the peripheral remaining hypermetabolic tumor segment to create the BTV containing a hypovascularized and hypometabolic tumor segment with a SUV between 0 and 3. The BTV was then irradiated with a single fraction without taking into account the clinical target volume (CTV) or the planning target volume (PTV); no margins were added to the BTV for these treatments.
Radiotherapy technique
A stereotactic radiotherapy plan with multiple coplanar static beams was calculated on the free-breathing CT scan (PINNACLE3, Philips, Fitchburg, USA). The planned radiation dose was 10 Gy in a single fraction prescribed to the 70%-isodose line (Dmax 14 Gy at isocenter). Treatment was delivered by 6 MV FFF (flattening filter free) photon beams. For field conformation, a micro-multileaf collimator (leaf width of 5 mm at isocenter, Elekta Oncology Systems, Crawley, UK) was used. Technical features are summarized in Supplemental Table S1. As for the dose constraints for various critical organs, we used those proposed by AAPM Task Group 101. Before every treatment, a kilovoltage cone-beam CT was carried out with the XVI system implemented on a VERSA HD linear accelerator (Elekta) for correcting patient's setup. Cone-beam CT scan was registered with the simulation CT scan by using an automatic so-called bone-matching algorithm, a chamfer-matching algorithm implemented in the XVI software.
Patients
Seven patients were treated, six with 10 Gy single fraction and one with 21 Gy in three fractions (Dmax 29 Gy at isocenter) due to previous irradiation of a nearby region. The treated patients’ main characteristics are summarized in . Three of seven patients previously received chemotherapy, and one was treated with immunotherapy but not within the 2 months prior to radiotherapy. They all developed disease progression after systemic treatment before the radiotherapy. No patient received any systemic treatment concomitant with radiotherapy or after.
Table 1. Main characteristics of seven patients treated at radiotherapy institute ‘KABEG Klinikum, Klagenfurt am Wörthersee’.
The first evaluation of response was performed when significant regression of symptoms was recorded (average 2–3 weeks), followed by repeated CT scans every 2 months. The Response Evaluation Criteria in Solid Tumors (RECIST) were used.
Results
The average BTV volume (mean: 65.6 cm³, range: 42.6–90.2 cm³) was about 30% of the GTV (gross tumor volume, mean: 212.9 cm³, range: 132.5–298.2 cm³; mean diameter: 7.9 cm, range: 7–10 cm). The average maximum SUV (SUVmax) of the treated lesions was 19.3 (range: 15.2–26.7), while the BTV, considering exclusively its junctional (hypovascularized) segment, was 2.7 (range: 1–3) which represented 15% of SUVmax among the treated lesions; excluding the junctional region of the BTV and considering only its inner-necrotic part, SUVmax < 1.
At the time of analysis, three patients had died, only one of them because of systemic disease progression 2 months after radiotherapy while the other two patients died due to causes other than cancer. The median follow-up was 6 months (range, 2–9 months). Among all seven patients, a significant RIBE was observed, with an intense tumor regression after an average of 3 weeks. Additionally, a significant RIAE was observed in two patients (); Supplementary Figure S1(B, D)). Overall, the response rate for the relief of the symptoms and mass reduction was 100% (2 complete and 5 partial responses). The symptoms, for which the patients underwent radiotherapy, were under control at their last follow up and the irradiated lesions did not regrow. When downsizing was achieved (after an average of 4 weeks) with the maximum tumor regression at that time (average tumor shrinkage 60%), we proceeded with conventional palliative irradiation (20 Gy in 5 fractions) of almost all remaining lesions to achieve maximum control among those bulky masses. No patient experienced any acute or late toxicity of any grade.
Figure 2. (A) A very voluminous squamous cell carcinoma of the right lung, with a maximum diameter of 10 × 9 cm at the time of the radiotherapy. (B) The arrow showing the asymptomatic mediastinal lymphadenopathies. (C) An induction of the bystander effect with a high-dose partial irradiation of the gross tumor volume (GTV, bigger contour) by targeting the central hypoxic region (smaller area). The centrally located isodose-line corresponds to 10 Gy. (D) A dramatic regression of the treated lesion only 3 weeks later. (E) An abscopal effect among the untreated mediastinal lymphadenopathies.
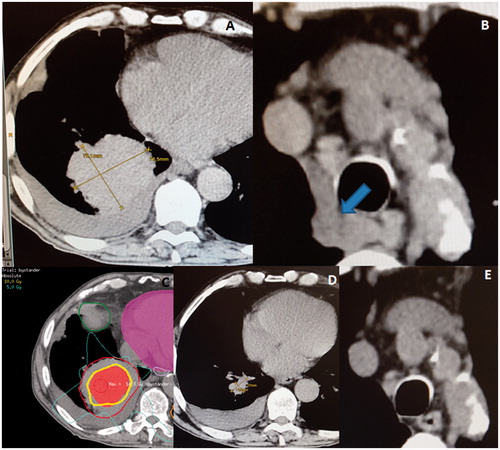
From our seven cases, we selected the following 3 for this report, which best exemplify the feasibility, and efficacy of this therapeutic option.
Case 1
A 56-year-old female, with no co-morbidity, with a centrally located adenosquamous right-lung carcinoma and partial atelectasis underwent two cycles of chemotherapy with Cisplatin/Pemetrexed, followed by radiotherapy to the right hilus with four conformal fields, 42 Gy in 3 Gy fractions (Supplemental Figure S2(A)). A follow-up CT after 4 months showed an incomplete tumor regression and significant radiation pneumonitis. No further chemotherapy was administered. Six months later, the patient presented again with significant dyspnea due to progression of the disease to the left hilus; the lesion diameter was 6 × 6 cm and it induced a complete left-lung atelectasis (Supplementary Figure S2(B)). She was no longer suitable for chemotherapy; therefore, re-irradiation was planned with the intent to dis-obstruct the main bronchus. By using seven conformal beams, this lesion was re-irradiated partially by targeting its central hypoxic segment, which corresponded to 30% of the total GTV volume (Supplementary Figure S3(A)). The total dose was 21 Gy in three 7 Gy-fractions, prescribed to the 70% isodose-line (Dmax 29Gy). Two months later, a follow-up CT showed a 70% regression of the treated lesion and an almost complete regression of the atelectasis (Supplementary Figures S3(B) and S4(A–C)).
Case 2
A 78-year-old male with a history of prostate cancer (which had been treated with radiotherapy 8 years earlier), an adenocarcinoma of the ascending colon (for which he underwent a right hemicolectomy and adjuvant chemotherapy 1 year earlier), now presented with a voluminous left neck metastasis (Supplementary Figure S5(A)), from a squamous cell carcinoma of the right ear that was previously resected in combination with a modified radical neck dissection. The 9 × 7 cm large lesion was un-resectable due to its infiltration of the cervical vertebra bodies. In addition, he also presented with a single right lung metastasis. Chemotherapy was not an option. Due to increasing pain, he was treated with radiotherapy, 10 Gy in a single fraction to the 70% isodose-line (Dmax 14 Gy) to 30% of the centrally located volume of this lesion, corresponding to the hypoxic tumor segment (Supplementary Figure S5(B)). Only 2 weeks later, tumor regression was observed (about 50%), which started from the lesion’s non-irradiated periphery towards the irradiated center (Supplementary Figures S5(C) and 6(A, B)). The patient had no longer any pain.
Case 3
A 73-year-old male, previously resected for a malignant melanoma and with a history of hypothyroidism and nicotine/alcohol abuse, was diagnosed with a right lower-lung lobe squamous cell carcinoma. His tumor was very voluminous, causing dyspnea and pain. At that time, the carcinoma measured 10 × 9 cm (). Furthermore, there were also mediastinal lymphadenopathies that, because asymptomatic, were not subjected to treatment (), and two small metastases in the contralateral lung. Because of a poor performance status, both resection and systemic therapy were unsuitable. Due to the important symptoms, the multidisciplinary tumor board recommended palliative irradiation of the lung lesion. Also for this patient we chose a single 10 Gy-fraction to the 70% isodose-line, prescribed to 30% of the hypoxic tumor segment (). Four weeks later, a dramatic regression was observed; the tumor had reduced to 2 cm (), and the dyspnea and pain completely disappeared. Furthermore, the untreated mediastinal lymphadenopathies also disappeared ().
At the time of analysis all three patients are still alive, 1 at 9 months and 2 at 5 months, respectively, post-radiotherapy.
Discussion
Despite that more than six decades have passed since the first report on RIAE [Citation4], its nature is still unclear. This phenomenon is often described as an immunologic response induced by radiotherapy, and an increasing number of studies call it an immune-mediated distant effect [Citation5]. Those studies were the basis for the proposed synergistic effect of concomitant radio-immunotherapy, to revive the RIAE through cancer immunomodulation, a so called in situ anti-tumor vaccine. Clinical evidence suggests that a combination of radiotherapy and immunotherapy could improve the RIAE response rates and increase survival [Citation6]. Until now, very few prospective trials [Citation6,Citation7] have used this treatment modality to elicit the immune-mediated abscopal responses.
However, other clinical [Citation8–10] and pre-clinical studies [Citation11] have shown that immunotherapy was not required to induce RIAE. Furthermore, numerous in vitro studies [Citation3,Citation12–14] have shown that a strong RIAE/RIBE can be induced by tumor signaling in response to radiotherapy, also without the immune system mediating the phenomenon. A cell–cell communication for the RIBE and secreted soluble molecules for the RIAE appear to play important roles in mediating the effects [Citation15,Citation16].
Although the RIAE likely represents an immunological response induced by radiotherapy (even more likely if induced by higher radiation doses), it is still rarely observed clinically. Considering that there are multiple published cases on RIAE, it seems that this phenomenon did not pass unobserved in the past, when immunotherapy and/or hypo-fractionated radiotherapy were rarely used; the majority of irradiated patients that manifested RIAEs was not subject to any immunotherapy. Therefore, the nature of RIAE remains controversial, and limited to three main categories: the immune system, tumor signaling, and the pseudo-abscopal effect [Citation17].
The results of in vitro studies on the most effective radiation dose able to induce RIAEs/RIBEs are very contradictory and cannot be transferred directly to the in vivo situations. No studies, until now, have had the objective of evaluating specific factors, including radiation dose that might play a role in the induction of RIAEs. Some preclinical studies [Citation18] suggest that doses greater than 8 Gy in a single fraction lead to an immunogenic tumor cell death. Other studies, like those in [Citation3, Citation14] by the author of this article, confirmed the dependence of the RIAE/RIBE-intensity on the radiation dose, concluding, however, that the inductive radiation dose depends on the tumor type. In the same experimental study [Citation3] we have tried to find a correlation between the intrinsic radio-sensitivity of a certain tumor type and its capacity to induce significant RIAE. Interestingly, the findings described an inversely proportional relationship between the intrinsic radio-sensitivity of different human lungs cancer cells and their abscopally-induced radio-sensitivity; the more radio-resistant the tumor cells-inducer of the abscopal signals are, the more sensitive it will be, if exposed to the same abscopal signals. Even if there is no proof for the correlation between those findings and the clinical observations, what is interesting here is that two of the most radio-resistant solid tumor types (the renal cell carcinoma and melanoma) are the most reported ones among clinical cases on RIAE. Conversely, the more radio-sensitive tumor cells-inducer of RIAE were much more able to ‘communicate to the unirradiated cells radio-protective’ or ‘pro-proliferative signals’. Since similar results have not yet been reported, this warrants further studies. The hypothesis is that the more radio-sensitive the cells are, the more they are able to release and transmit protective and proliferative abscopal signals. Until now, only our study has dealt with the tumor oxygen status and its relation to the RIAE/RIBE induction. The results have shown that irradiation of a part of a tumor-inductor of the RIAE/RIBE, hypoxic versus normoxic respectively, produce a more intense RIAE.
With regard to the RIBE, there is a great lack of knowledge concerning its role in clinical radiation oncology. Our knowledge on RIBEs derives mostly from preclinical studies. The RIAE and RIBE do however exist, as demonstrated here, and they can be intentionally induced by radiation, leading to partial and/or complete long lasting regression of metastatic disease [Citation19]. It is still unclear why these non-targeted effects have only been seen sporadically until now. It is possible that attention should be focused on the tumor type, its radio-sensitivity, dose and fractionation schedule, tumor volume to be targeted, stressing tumor hypoxia. It is likely that multiple factors are competing with one another, and that only a perfect combination of those factors is able to trigger a strong RIAE/RIBE [Citation3]. The immune-system is involved, but it is not the dominant component that determines the manifestation of the phenomenon, otherwise, every single metastatic patient exposed to radio-immunotherapy would manifest it. In our study, non-targeted effects were induced in all cases, but none of those patients was treated with immunotherapy. Considering how many patients are treated every day with this combination modality without manifesting RIAEs, shows that this phenomenon is still rare, despite the fact that all patients are immune-competent. Therefore, theoretically, by establishing the perfect conditions and considering the previously mentioned factors, it should be possible to intentionally induce an effective RIAE.
The endpoint of this preliminary study was to test the validity of our hypothesis; could significant non-targeted effects by targeting only the hypoxic segment of bulky tumors with a single-high dose of radiotherapy be induced? And our answer is yes. What is interesting here is that the radiobiological rules indicate that, for the control and eradication of a tumor lesion, it is necessary to cover the entire lesion with the prescribed dose, including its possible microscopic infiltration outside the detectable tumor (CTV), and that the prescribed dose should be as homogeneous as possible within the whole PTV (at least 95%) which is not the case with our technique. Furthermore, the larger the lesion the higher the dose should be in order to kill all cells. Unfortunately, though, large volumes are not possible to treat with tumoricidal doses, especially not if in proximity of important organs. Obviously, further research and longer follow ups will be necessary in order to confirm the effectiveness of our results. Furthermore, as the dose outside the tumor is very low by using our technique, usually less than 2 Gy, it allows for re–irradiation in case of relapse; the down staging of the voluminous tumors by employing RIBE’s has the potential to convert non-resectable into resectable lesions, and a palliative intent into a curative intent.
Currently, different PET tracers are available for the identification of hypoxia, with the fluorinated nitroimidazole derivative 18F-fluoromisonidazole or 18F-FMISO as the most widely used ones. Our Institute did not have any hypoxia-specific tracer. By using 18F-FDG PET and contrast CT, we overcome these limitations at least partially. 18F-FDG PET is not optimal to indicate hypoxic regions, but is capable of defining more aggressive tumor types, also correlated with HIF-1α expression, in patients with gastric carcinoma [Citation20], tongue cancer [Citation21], NSCLC [Citation22,Citation23] and oral squamous cell carcinoma [Citation21–24]. Further, this defined target corresponded to the central tumor region-outside the contrast-enhanced tumor segment which perfectly matched the results obtained by immunohistochemical staining of hypoxic cells using certain 2-nitroimidazoles, which typically showed increasing signal intensity as a function of increasing distance from the microvessel-carrying stroma [Citation25]. By measuring metabolic activities in three segments defined on the contrast-CT, we found that SUV among the necrotic segment (<1) and the hypermetabolic segment (average 19.3) were rather stable while unstable and with steep increase at the junction between hypovascularized and hypervascularized segments. We cannot claim that this irradiated tumor part corresponds to the hypoxic tumor, but we argue that our target structurally corresponds to an unvascularized and hypovascularized part of the tumor with very low metabolic activity.
Our simple 1-day-treatment have demonstrated high effectiveness, with no toxicity among patients affected by symptomatic and hard-to-treat tumors, showing a high potential for regional and distant non-targeted effects induction.
IONC_A_1346385_Supplementary_Information.zip
Download Zip (3.5 MB)Disclosure statement
The authors report no conflicts of interest.
References
- Cook AM, Berry RJ. Direct and indirect effects of radiation: their relation to growth. Nature. 1966;210:324–325.
- Nagasawa H, Little JB. Induction of sister chromatid exchanges by extremely low doses of alpha-particles. Cancer Res. 1992;52:6394–6396.
- Tubin S, Mansoor MA, Gupta S. Radiation and acute hypoxia-induced abscopal effects in normoxic and hypoxic conditions in human lung cancer cells. ESTRO 35, Turin, Italy, 2016: Abstract Book. Available at https://user-swndwmf.cld.bz/ESTRO-35-Abstract-book3/989.
- Mole RH. Whole body irradiation; radiobiology or medicine? Br J Radiol. 1953;26:234–241.
- Cao X. Regulatory T cells and immune tolerance to tumors. Immunol Res. 2010;46:79–93.
- Golden EB, Chhabra A, Chachoua A, et al. Local radiotherapy and granulocyte-macrophage colony-stimulating factor to generate abscopal responses in patients with metastatic solid tumours: a proof-of-principle trial. Lancet Oncol. 2015;16:795–803.
- Brody JD, Ai WZ, Czerwinski DK, et al. In situ vaccination with a TLR9 agonist induces systemic lymphoma regression: a phase I/II study. J Clin Oncol. 2010;28:4324–4332.
- Wersall PJ, Blomgren H, Pisa P, et al. Regression of non-irradiated metastases after extracranial stereotactic radiotherapy in metastatic renal cell carcinoma. Acta Oncol. 2006;45:493–497.
- Tubin S, Casamassima F, Menichelli C, et al. A case report on metastatic thyroid carcinoma: radiation-induced bystander or abscopal effect? J Cancer Sci Ther. 2012;4:408–411.
- Siva S, Callahan J, Mac Manus MP, et al. Abscopal [corrected] effects after conventional and stereotactic lung irradiation of non-small-cell lung cancer. J Thorac Oncol. 2013;8:e71–e72.
- Kanagavelu S, Gupta S, Wu X, et al. In vivo effects of lattice radiation therapy on local and distant lung cancer: potential role of immunomodulation. Radiat Res. 2014;182:149–162.
- Gupta S, Zagurovskaya M, Wu X, et al. Spatially Fractionated Grid High-dose radiation-induced tumor regression in A549 lung adenocarcinoma xenografts: cytokines and ceramide regulators balance in abscopal phenomena. Sylvester Comprehensive Cancer Center, 2014:20.
- Shareef MM, Cui N, Burikhanov R, et al. Role of tumor necrosis factor alpha and TRAIL in high-dose radiation-induced bystander signaling in lung adenocarcinoma. Cancer Res. 2007;67:11811–11820.
- Tubin S, Valeriani M, Salerno G, et al. Manipulation of radiation-induced bystander effect in prostate adenocarcinoma by dose and tumor differentiation grade: in vitro study. Int J Radiat Biol. 2015;91:166–171.
- Prise KM, O’sullivan JM. Radiation-induced bystander signalling in cancer therapy. Nat Rev Cancer. 2009;9:351–360.
- Baskar R. Emerging role of radiation induced bystander effects: cell communications and carcinogenesis. Genome Integr. 2010;1:13.
- Kaminski JM, Shinohara E, Summers JB, et al. The controversial abscopal effect. Cancer Treat Rev. 2005;31:159–172.
- Schaue D, Ratikan JA, Iwamoto KS, et al. Maximizing tumor immunity with fractionated radiation. Int J Radiat Oncol Biol Phys. 2012;83:1306–1310.
- Ishihara D, Pop L, Takeshima T, et al. Rationale and evidence to combine radiation therapy and immunotherapy for cancer treatment. Cancer Immunol Immunother. 2016;66:281–298.
- Takebayashi R, Izuichi K, Yamamoto Y, et al. [18F]fluorodeoxyglucose accumulation as a biological marker of hypoxic status but not glucose transport ability in gastric cancer. J Exp Clin Cancer Res. 2013;32:34.
- Han MW, Lee HJ, Cho KJ, et al. Role of FDG-pet as a biological marker for predictiong the hypoxic status of tongue cancer. Head Neck. 2012;3:1395–1402.
- Van Baardwijk A, Dooms C, Van Suylen RJ, et al. The maximum uptake of (18)F-deoxyglucose on positron emission tomography scan correlated with survival, hypoxia inducible factor-1alpha and GLUT-1 in non-small cell lung cancer. Eur J Cancer. 2007;43:1392–1398.
- Kaira K, Okumura T, Ohde Y, et al. Correlation between 18F-FDG uptake on PET and molecular biology in metastatic pulmonary tumors. J Nucl Med. 2011;52:705–711.
- Yamada T, Uchida M, Kwang-Lee K, et al. Correlation of metabolism/hypoxia markers and fluorodeoxyglucose uptake in oral squamous cell carcinomas. Oral Surg Oral Med Oral Pathol Oral Radiol. 2012;113:464–471.
- Yeom CJ, Zeng L, Zhu Y, et al. Strategies to assess hypoxic/HIF-1-active cancer cells for the development of innovative radiation therapy. Cancers (Basel). 2011;3:3610–3631.