Abstract
The present work is a chronological study of the pathogenesis of three attenuated serotype 1 Marek's disease (MD) virus strains (RM1, CVI988 and 648A80) that provide high protection against MD but have been attenuated by different procedures and induce different degrees of lymphoid organ atrophy. All studied strains replicated in the lymphoid organs (bursa,x thymus and spleen) and a peak of replication was detected at 6 days post inoculation (d.p.i.). Differences, however, were observed among vaccine strains. RM1 strain replicates more in all lymphoid organs compared with CVI988 and 648A80 strains. In addition, replication of RM1 in the thymus did not decrease after 6 d.p.i. but continued at high levels at 14 d.p.i. and until the thymus was completely destroyed. Lung infection occurred very early after infection with all of the three vaccines and the level of replication was similar to that found in the lymphoid organs. Infected cells were very large and appeared scattered in the lung parenchyma and in the parabronchial lining. The study of the target cells for the early infection in cell suspensions of blood and spleen showed that both non-adherent cell populations (enriched in lymphoid cells) and adherent cells (enriched in monocytes/macrophages) supported MD virus infection. Infection in adherent cells was especially high at very early stages of the infection (3 to 6 d.p.i.). Atrophy of lymphoid organs is a major drawback in the production of highly protective vaccines against MD. A better understanding of the mechanisms associated with lymphoid organ atrophy will aid in overcoming this problem.
Introduction
Marek's disease (MD) is a lymphoproliferative disease of chickens that causes important economic losses to the poultry industry. MD is induced by an α-herpesvirus, Marek's disease virus (MDV). MD has been successfully controlled by vaccination since 1968 (Churchill & Biggs, Citation1968). The constant evolution of MDV towards more virulence, however, jeopardizes the efficacy of MD vaccines and forces the poultry industry to keep looking for better methods of control.
Attenuated serotype 1 MDV strains are currently the most protective vaccines available (reviewed in Gimeno, Citation2004). Attenuation of MDV strains is traditionally accomplished by serial passages in cell culture. Commercially available serotype 1 MDV vaccines have been attenuated by serial passages in cell culture. However, attenuation of the oncogenic properties of MDV can also be achieved by modifying the MDV genome. Insertion of the long terminal repeat (LTR) region of the reticuloendotheliosis virus (REV) into the MDV genome results in attenuation of the oncogenic properties of MDV (Witter et al., Citation1997; Kim et al., Citation2011). Deletion of the meq gene from very virulent strain Md5 results in loss of oncogenicity (Lupiani et al., Citation2004).
Attenuation of MDV strains in cell culture not only decreases the oncogenic properties of MDV but also decreases its in vivo replication ability (Schat et al., Citation1985; Dudnikova et al., Citation2009). Infection of lymphocytes in vitro is impaired after MDV attenuation in cell culture and this might contribute to the decreased in vivo replication (Schat et al., Citation1985). Most attenuated serotype 1 MDV strains do not induce an early cytolytic infection in the lymphoid organs (Schat et al., Citation1985; Gimeno et al., Citation2001). Attenuated serotype 1 MDV strains can be isolated from blood and spleen by the traditional plaque assay and can be detected in blood, spleen, and feather pulp by real-time polymerase chain reaction (PCR) (Schat et al., Citation1985; Witter et al., Citation1995; Gimeno et al., Citation2004; Baigent et al., Citation2007; Dudnikova et al., Citation2009). However, the cellular tropism of these strains as well as the nature of their interactions with the host cells is still poorly understood.
Insertion of the LTR region of REV into the MDV genome resulted in loss of the oncogenic properties of MDV but did not affect in vivo replication (Witter et al., Citation1997). The RM1 strain of MDV does not induce neoplasia even in very susceptible chickens lacking maternal antibodies but it leads to very severe atrophy of lymphoid organs (Witter et al., Citation1997). It is not yet clear why insertion of LTR leads to abrogation of oncogenicity. Liu and coworkers demonstrated that insertion of REV LTR perturbed transcription of the SORF2 gene (Liu et al., Citation2001). Kim et al., (Citation2011) recently demonstrated that insertion of REV LTR into a bacterial artificial chromosome clone of MDV alters expression of other nearby MDV genes. A better understanding of the pathogenesis of the RM1 strain might help to elucidate the mechanisms behind the attenuation of this strain.
MD vaccines attenuated in cell culture do not induce lymphoid organ atrophy (Schat et al., Citation1985; Gimeno et al., Citation2001). However, MDV strains attenuated by the insertion of REV LTR (Witter et al., Citation1997; Kim et al., Citation2011) and those attenuated by deletion of the oncogene meq induce severe atrophy in the lymphoid organs when inoculated in chickens lacking maternal antibodies against MDV (Lupiani et al., Citation2004; Lee et al., Citation2010;). Remarkably, those strains are also found to confer very high protection against early challenge with very virulent plus (vv +) MDV (Witter et al., Citation1997; Witter & Kreager, Citation2004; Lee et al., Citation2010; Kim et al., Citation2011). Lymphoid organ atrophy is a major drawback of these vaccine strains as they cannot be licensed due to the regulatory requirements of testing in antibody-negative chickens without affecting the lymphoid organs (Witter & Kreager, Citation2004). Understanding the mechanism leading to lymphoid organ atrophy and the relationship with high protection will aid in the development of better vaccines that lack this deleterious feature.
The objective of the present work was to compare replication ability of three highly protective vaccines (RM1, CVI988, and 648A80) that induce different degrees of lymphoid organ atrophy. The specific objective of this work was to determine whether differences in tissue tropism and/or severity of the early cytolytic infection were related to lymphoid organ atrophy.
Materials and Methods
Chickens
Chickens were MD-susceptible F1 progeny (15×7) of Avian Disease and Oncology Laboratory line 15I5 males and line 71 females (Bacon et al., Citation2000). They were from unvaccinated breeder hens, free of antibodies to MDV and Herpesvirus of turkey (HVT) as well as to avian leucosis virus, REV and other common poultry pathogens.
Viruses
Three attenuated serotype 1 MDV strains were used. Strain CVI988 was naturally poorly oncogenic (mild) (Rispens et al., Citation1972) and has been further attenuated by 42 passages in chick embryo fibroblasts (Rhone Merieux obtained in 1991 from Select Laboratories). Strain 648A80 originated from a vv + strain 648A (Witter, Citation1997) and was attenuated by 80 serial passages in chick embryo fibroblasts (Witter, Citation2002). Neither CVI988 nor 648A80 induce lymphoid organ atrophy when inoculated in chickens lacking maternal antibodies against MDV (Gimeno et al., Citation2004). Strain RM1 originated from an oncogenic strain JM/102W (Sevoian et al., Citation1962) and was attenuated by insertion of the REV LTR region into its genome (Jones et al., Citation1996). Strain RM1 induces severe lymphoid organ atrophy in chickens lacking maternal antibodies against MDV (Witter et al., Citation1997). All three strains used in this study conferred high protection against early challenge with vv + MDV; however, strain RM1 protected better than the other two strains in a previous study (Witter & Kreager, Citation2004).
Pathology
Necropsy of every chicken was conducted before collection of samples to evaluate gross lesions. Lymphoid organ atrophy was subjectively scored from 0 to 4 based on severity.
Real-time PCR
DNA was extracted from tissues using a Puregene DNA Isolation Kit (Gentra System, Minneapolis, Minnesota, USA). Each sample was amplified with primers specific for the glycoprotein B (gB) gene of MDV as well as with primers specific for the chicken glyceraldehyde-3-phosphate dehydrogenase (GAPDH) gene as previously described (Gimeno et al., Citation2005). The respective forward and reverse primers were TM.5 (5′-CGGTGGCTTTTCTAGGTTCG-3′) and TM.3 (5′-CCAGTGGGTTCAACCGTGA-3′) for amplification of the gB gene, and GAPDH-TM.5 (5′-GGAGTCAACGGATTTGGCC-3′) and GAPDH-TM.3 (5′-TTTGCCAGAGAGGACGGC-3′) for amplification of the GAPDH gene. Amplifications were done using the GeneAmp 5700 (Applied Biosystems, Foster City, California, USA) in a 25 µl PCR reaction containing 50 ng DNA, 0.2 µM each primer and a SYBR green PCR master mix that contained the appropriate buffers, nucleotides and Taq polymerase (Applied Biosystem, Warrington, UK). The reaction consisted of 40 cycles with 95°C denaturation for 15 sec and a 60°C combined annealing/extension time for 60 sec. Fluorescence was acquired at the end of the annealing/extension phase. The melting curves were obtained at the end of amplification by cooling the sample at 20°C/sec to 60°C and then increasing the temperature to 95°C at 0.1°C/sec. The number of viral DNA copies was estimated by the use of a standard curve. Standards used in this study were serial dilutions of the cosmid SN16 that includes a complete copy of the MDV gene gB. Results are expressed as log10(number of viral DNA copies + 1).
Immunohistochemistry
An avidin–biotin–peroxidase complex (Vectastain ABC kit; Vector Laboratories, Burlingame, California, USA) was used for immunohistochemistry. To study MDV replication in the lymphoid organs and in the lung, the monoclonal antibodies (mAb) H19 and T65 specific to the pp38 were used. mAb H19 specifically recognizes pp38 antigen of all serotype 1 MDV strains but CVI988 (Lee et al., Citation1983), and it was used at a working dilution of 1:3,200. mAb T65 specifically recognizes pp38 antigen of strain CVI988 and was used at a working dilution of 1:15,000 (Silva & Lee, Citation1984). Viral antigen expression was subjectively scored from 0 to 4 based on the frequency of positive cells.
Cell suspension
Buffy coats from blood samples and splenic cell suspensions were obtained as described previously (Gimeno et al., Citation2004). Both buffy coats and suspensions of splenocytes were inoculated into 35-mm tissue culture plates and incubated at 41°C for 4 h. Supernatants were collected afterwards and placed in fresh tissue culture plates. Original tissue culture plates were washed with phosphate-buffered saline five times to separate non-adherent cells and avoid contamination of the non-adherent cells. All tissue culture plates were incubated at 41°C overnight. Supernatant from plates containing non-adherent cells was collected, cells were centrifuged, and DNA was extracted. Supernatants of plates containing adherent cells were discarded and plates were washed 10 times with phosphate-buffered saline. DNA extraction from the adherent cells was conducted in the tissue culture plates. Adherent cell populations, separated using this technique, have been shown to include 81.5% monocyte-derived macrophages and 8% thrombocytes (Kaspers et al., Citation1993). The non-adherent cell populations have been shown to be enriched in lymphocytes (Rabinowitz, Citation1964).
Experimental design
One-day-old 15×7 chickens were randomly divided into four groups of 60 chickens each, and kept in Horsfall–Bauer isolation units. Three of the groups were inoculated subcutaneously with 10,000 plaque-forming units (PFU) of one of the three strains of viruses used in the study (RM1, CVI988, 648A80). The remaining group was a non-inoculated control group. Six chickens per group were euthanized at 1, 3, 6, 14, and 26 d.p.i. Samples of the bursa, thymus, spleen (days 1, 3, 6, 14, and 26) and lung (days 3, 6, and 14) were collected for quantifying viral DNA load by real-time PCR and for demonstrating viral protein by immunohistochemistry. Sampling times were selected to cover periods before the early cytolytic infection (1 and 3 d.p.i.), during early cytolytic infection (6 d.p.i.), and after early cytolytic infection (14 and 26 d.p.i.). Samples were stored at −70°C until they were processed. Blood and spleen samples were collected at each time point (1, 3, 6, 14, and 26 d.p.i.) from an additional six chickens per group to make cell suspensions. All chickens were handled according to the guidelines of the USDA Avian Disease and Oncology Laboratory Animal Care and Use Committee.
Statistical analysis
Data were analysed with the statistical program Statistica (Stat Soft, Tulsa, Oklahoma, USA). Comparison between groups was conducted by a one-way analysis of variance test. In all cases the Scheffé test was used for post hoc analysis. Results were considered statistically significant at P<0.05.
Results
Replication in the lymphoid organs
The three evaluated vaccines replicated in the lymphoid organs (spleen, bursa, and thymus) within a few days after infection (). All vaccines were detected (viral DNA load and pp38 expression) in lymphoid organs as early as 3 d.p.i. and reached the peak of replication at 6 d.p.i. Both vaccine DNA load and pp38 expression decreased by 14 d.p.i. and a second wave of replication was detected at 26 d.p.i. Differences, however, were found between vaccine strains and between lymphoid organs.
Table 1. Viral DNA load by real time PCR and pp38 expression in lymphoid organs1
The RM1 strain replicated earlier and at higher levels in all lymphoid organs at every time point compared with the CVI988 and 648A80 strains. In chickens inoculated with RM1, the high level of viral DNA load and pp38 antigen expression in the thymus was particularly remarkable not only at 3 and 6 d.p.i. but also at 14 d.p.i. (a,b). Unfortunately, the RM1 strain induced very severe atrophy of the thymus in all inoculated chickens and no thymus tissue could be collected for immunohistochemistry at 26 d.p.i. At 14 d.p.i., no pp38 expression was detected in chickens inoculated with CVI988 and 648A80. Little or no pp38 expression was detected at 26 d.p.i. in any of the evaluated tissues.
Figure 1. Immunohistochemical demonstration of replication of the RM1 strain in the thymus and lung at 6 and 14 d.p.i. 1a: Expression of pp38 antigen in the thymus of a chicken 6 days after subcutaneous (s.c.) vaccination with 10,000 PFU of RM1. Bar = 120 µm. 1b: Expression of pp38 antigen in the thymus of a chicken 14 d.p.i. with 10,000 PFU of RM1 by the s.c. route. Bar = 120 µm. Please note that the thymic tissue has marked depletion of lymphocytes and the pp38 expression is limited to the remnant lymphoid tissues. Arrowheads show the islets of positive cells. 1c: Expression of pp38 antigen in the lung of a chicken 6 d.p.i. with 10,000 PFU of RM1 by the s.c. route. Bar = 40 µm. Please note the distribution of positive cells in the parabronchi (asterisks) and within the interstitium. 1d: Expression of pp38 antigen in the lung of a chicken 6 d.p.i. with 10,000 PFU of RM1 by the s.c. route. Parabronchi are indicated with asterisks. Bar = 60 µm.
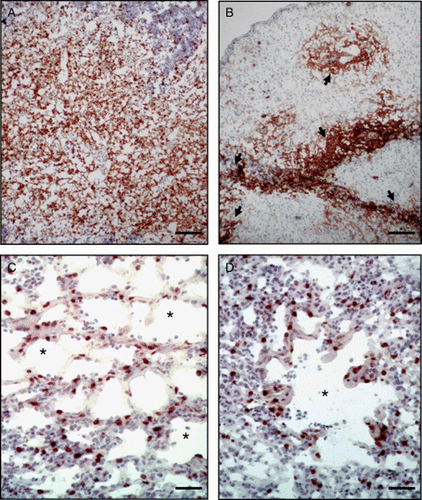
Lymphoid organ atrophy
None of the chickens inoculated with CVI988 or 648A80 developed atrophy of the bursa or of the thymus at any time point. Chickens inoculated with RM1 did not show any lymphoid organ atrophy at 1, 3, and 6 d.p.i. However, bursal atrophy was detected in 50% of the chickens at 14 d.p.i. (2 + ) and at 26 d.p.i. (2 + ). Thymic atrophy was detected in 100% of the chickens at 14 d.p.i. (3 + ) and 26 d.p.i. (4 + ).
Replication in the lung
Both viral genome and viral antigen pp38 of the three evaluated vaccines were detected in the lung (). However, RM1 strain was detected earlier (3 d.p.i.) and replicated more (P < 0.05) at each time point than the other two vaccine strains (CVI988 and 648A80). Replication in the lung was higher at 6 d.p.i. than at 3 and 14 d.p.i. Expression of pp38 antigen in the lung of chickens vaccinated with RM1 was detected in cells lining the parabronchi and within the interstitium (c,d). Distribution of pp38+ cells was very homogeneous throughout the pulmonary parenchyma of chickens vaccinated with RM1. On the other hand, expression of pp38 antigen in the lung of chickens vaccinated with CVI988 and 648A80 was detected in few scattered cells (data not shown).
Figure 2. Vaccine DNA load in the lung of chickens vaccinated with 10,000 PFU of CVI988, RM1, and 648A80 strains by the subcutaneous route at 1 day of age. The load of viral DNA is expressed as the log of the number of genome copies per 50 ng total DNA. Data represent the average (and standard error) of six chickens per group and per time point. The same letters above the bars indicate that no statistically significant differences were found in the analysis of variance test within the same time point (P<0.05).
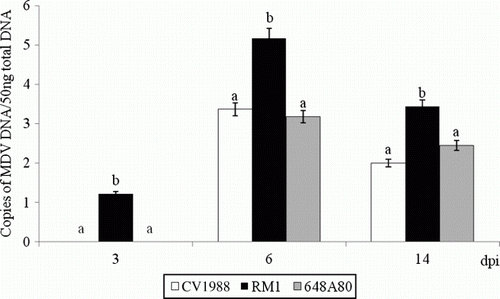
Target cells in the blood and spleen
Infection of adherent and non-adherent cells in the blood and spleen was evaluated (). The viral genome of the three evaluated vaccines was detected in both adherent and non-adherent cells at 3, 6, 14, and 26 d.p.i. No viral DNA was found at 1 d.p.i. in any of the evaluated cell subpopulations. The load of vaccine DNA in chickens vaccinated with strain RM1 was higher than in chickens inoculated with strains 648A80 and CVI9888 in all of the evaluated cell subpopulations at 3, 6, and 26 d.p.i. (an exception to this was adherent splenocytes at 6 d.p.i. and adherent blood cells at 26 d.p.i.). The load of viral DNA in adherent cell subpopulations was higher at 3 d.p.i., equal at 6 d.p.i., and lower at 14 and 26 d.p.i. than the load of viral DNA detected in non-adherent cells.
Table 2. Load of viral DNA in cell suspensions of blood and spleen 1
Discussion
The present study evaluated the replication of three attenuated MDV strains in the lymphoid organs and lungs of chickens following subcutaneous inoculation. The three vaccine strains used in this study are highly protective against early challenge with vv + MDV, although strain RM1 conferred higher protection than CVI988 and 648A80 in a previous study (Witter & Kreager, 2004). The three evaluated vaccines replicated in the bursa, thymus, spleen, and lung. In addition, all of them were detected not only in the non-adherent subpopulation (enriched in lymphoid cells) of blood and splenocytes but also in the adherent subpopulation (enriched in monocytes/macrophages). Differences, however, were found in the dynamics of infection, the extent of replication, and the tissue tropism among vaccines. Pathogenesis of CVI988 and 648A80 strains was similar to what has been previously described for other attenuated strains (Schat et al., 1985). Replication of those vaccines (both DNA and expression of pp38) was first detected in the spleen (3d.p.i.) followed by the bursa, thymus, and lung (6d.p.i.). At 14 and 26 d.p.i., CVI988 and 648A80 DNA was still detected in all examined tissues but there was none to minimal pp38 expression. Replication of RM1 strain had unique features. The replication of RM1 strain differed not only from CVI988 and 648A80 but also from what has been described for other attenuated strains or even oncogenic strains (Schat et al., Citation1985; Gimeno et al., Citation2001; Reddy et al., Citation2002). The RM1 strain seemed to have a particular tropism for thymus. RM1 DNA and pp38 expression were detected in the thymus as early as 3 d.p.i. Both viral DNA load and pp38 expression of RM1 were very high at 6 and 14 d.p.i. It is remarkable that RM1 induced such a severe atrophy in the thymus and by 14 d.p.i. most of the thymus had been depleted of lymphoid cells. However, expression of pp38 was still very high in the scant remnant lymphoid cells. This contrasts with the pathogenesis of oncogenic strains that have a transient replication in the lymphoid organs with a peak at 5 to 6 d.p.i. and absence of antigen expression at 14 d.p.i. (Calnek et al., Citation1970; Adldinger & Calnek, Citation1973; Payne & Rennie, Citation1973). It also contrasted with the replication of RM1 in other lymphoid organs (i.e. bursa) where no antigens were detected at 3 and at 14 d.p.i.
Mechanisms by which the RM1 strain has lost the ability to induce neoplasia are still poorly understood. Liu et al. (Citation2001) demonstrated that insertion of LTR resulted in abnormal transcription of SORF2, which on the other hand interacts with the growth hormone. These authors suggested that this could influence the ability of the virus to induce neoplasia. Alteration in the expression of other MDV genes in the proximity of REV LTR insertion has been reported recently (Kim et al., Citation2011). It has been demonstrated that the lack of oncogenicity and the severe atrophy of lymphoid organs is due to the insertion of the REV LTR. Insertion of LTR in other MDV strains has been reported (Kim et al., Citation2011). In all cases, the resulting strains lack oncogenic properties and induce severe atrophy of the thymus in chickens lacking maternal antibodies. Our results indicate that RM1-induced thymic atrophy is due, at least partially, to the remarkable tissue tropism of RM1 for the thymus. RM1 not only reached the thymus earlier than other MDV strains but also replicated more and for longer duration. It is possible that insertion of REV LTR into the MDV genome impaired, or negatively affected, the ability of RM1 to establish latency in T cells. A negative effect on the ability to establish latency has been also suggested in MDV strains lacking oncogene meq (Lupiani et al., Citation2004). Both vaccines with REV LTR insertion and those with deletion of oncogene meq induced lymphoid organ atrophy in chickens lacking maternal antibodies and are considered to confer the highest protection against challenge with vv + MDV (Witter & Kreager, Citation2004; Lee et al., Citation2010). Further studies to better understand the molecular mechanisms behind this altered tissue tropism are warranted.
In a previous study, we demonstrated that highly protective MD vaccines replicate better in the lymphoid organs than low-protective MD vaccines (Gimeno et al., Citation2004). The results of the present work demonstrate that there are differences in the degree of replication among highly protective vaccines. The RM1 strain replicated much more and for a longer time in the thymus than CVI988 and 648A80. Such strong viral replication led to lymphoid organ atrophy and this is a major drawback for licensing those strains as vaccines in most countries. While strong replication seems to be related to protection (Gimeno et al., Citation2004), atrophy of lymphoid organs is not. The RM1 strain protects equally well against vv + MDV challenge in chickens with or without maternal antibodies against MDV (Witter & Kreager, Citation2004). However, lymphoid organ atrophy only occurs when RM1 strain is administered to chickens lacking maternal antibodies against MDV (Witter et al., Citation1997). Current regulations require vaccine safety tests be conducted in specific-pathogen-free chickens lacking maternal antibodies against MDV (USDA, Citation2009). Unfortunately the most protective vaccines that have been developed recently induce severe lymphoid organ atrophy in chickens lacking maternal antibodies against MDV (Witter & Kreager, Citation2004; Lee et al., Citation2010). It is therefore imperative that the mechanisms behind the lymphoid organ atrophy are understood, so highly protective vaccines that do not induce lymphoid organ atrophy may be developed.
Replication of serotype 1 MDV vaccines in the lung has been reported previously (Gimeno et al., Citation2004). It has been demonstrated recently that pulmonary replication of MD vaccines is influenced by the efficacy of the vaccine and age/route of vaccination but not by the dose of vaccine used (Gimeno & Cortes, Citation2010). Co-infection with oncogenic virus resulted also in an increased replication of vaccine virus (Haq et al., Citation2010). Results of this work confirm that the three highly protective vaccines used in this study can replicate in the lung very early after subcutaneous vaccination. However, differences in the level of replication were detected among vaccines. Load of vaccine DNA and pp38 expression were much higher in the lung of chickens vaccinated with RM1 than with CVI988 or 648A80. The RM1 strain replicated extensively in the lung and infected the cells lining the parabronchi and within the pulmonary interstitium. The pattern of expression of pp38 in the lung of chickens inoculated with RM1 resembled those after infection with oncogenic strains (Purchase, Citation1970; Adldinger & Calnek, Citation1973; St Hill et al., Citation2004; Baaten et al., Citation2009). Purchase (Citation1970) detected MDV antigens in epithelial cells lining the air capillaries of lungs 5 days after intra-abdominal inoculation with various oncogenic MDV strains. Adldinger & Calnek ( 1973) infected chickens via inhalation and demonstrated the presence of MDV antigens in lungs during the first week of infection using an immunofluorescence technique. St Hill et al. (Citation2004) detected the DNA of an oncogenic MDV in lungs of chickens following 3, 5, 7, and 10 d.p.i. Baaten et al. ( 2009) demonstrated infection of macrophages and B cells in the lung of chickens infected intratracheally with oncogenic MDV. The nature of cells supporting infection with oncogenic and attenuated serotype 1 MDV is still unknown, and further studies using double-staining techniques like immunofluorescence/immunohistochemistry or in situ hybridization will be needed.
MDV strains suffered several changes during attenuation in cell culture (Witter & Schat, Citation2003). A reduced ability to replicate in lymphoid cells has been reported in MDV strains attenuated in cell culture (Shek et al., Citation1982; Schat et al., Citation1985). In this study, we have made an effort to separate the lymphoid cell population (non-adherent) from the non-lymphoid cells (adherent) in buffy coats and splenocytes. Our results indicate that the three evaluated vaccines infected both adherent as well as non-adherent cell subpopulations. The load of viral DNA detected in adherent cells was higher at 3 d.p.i., equal at 6 d.p.i., and lower at 14 and 26 d.p.i. than the load of viral DNA detected in non-adherent cells. However, these results should be interpreted with caution because viruses might have been reactivated during cell culture. The cell type has an important effect on the ability of the virus to reactivate. Therefore, our results indicated that vaccine virus was present in both cell subpopulations but it is not possible to compare the load of viral DNA in adherent and non-adherent cells.
The results of the present work demonstrate that the three highly protective vaccines replicate in the bursa, thymus, spleen, and lung within a few days of vaccination. Strains CVI988 and 648A80 originate from very different MDV strains and they differ greatly in the number of passages in cell culture. However, the dynamics and extent of replication of both strains was very similar and neither of them induced lymphoid organ atrophy. On the other hand, the RM1 strain exhibited an extraordinary tropism for the thymus that resulted in severe thymic atrophy. Further studies on the pathogenesis of RM1 in the thymus are warranted and could be very useful to elucidate molecular mechanisms behind lymphoid organ atrophy that will aid in the development of better vaccines without this detrimental feature.
Acknowledgements
The authors thank Ms Barbara Riegle for technical assistance and Dr Arun Pandiri for helpful critical review.
References
- Adldinger , H.K. and Calnek , B.W. 1973 . Pathogenesis of Marek's disease: early distribution of virus and viral antigens in infected chickens . Journal of the National Cancer Institute , 50 : 1287 – 1298 .
- Baaten , B.J. , Staines , K.A. , Smith , L.P. , Skinner , H. , Davison , T.F. and Butter , C. 2009 . Early replication in pulmonary B cells after infection with Marek's disease herpesvirus by the respiratory route . Viral Immunology , 22 : 431 – 444 .
- Bacon , L.D. , Hunt , H.D. and Cheng , H.H. 2000 . A review of the development of chicken lines to resolve genes determining resistance to diseases . Poultry Science , 79 : 1082 – 1093 .
- Baigent , S.J. , Smith , L.P. , Currie , R.J. and Nair , V.K. 2007 . Correlation of Marek's disease herpesvirus vaccine virus genome load in feather tips with protection, using an experimental challenge model . Avian Pathology , 36 : 467 – 474 .
- Calnek , B.W. , Ubertini , T. and Adldinger , H.K. 1970 . Viral antigen, virus particles, and infectivity of tissues from chickens with Marek's disease . Journal of the National Cancer Institute , 45 : 341 – 351 .
- Churchill , A.E. and Biggs , P.M. 1968 . Herpes-type virus isolated in cell culture from tumors of chickens with Marek's Disease. II. Studies in vivo . Journal of the National Cancer Institute , 41 : 951 – 956 .
- Dudnikova , E. , Vlasov , A. , Norkina , S. , Kireev , D. and Witter , R.L. 2009 . Factors influencing the attenuation of serotype 1 Marek's disease virus by serial cell culture passage and evaluation of attenuated strains for protection and replication . Avian Diseases , 53 : 63 – 72 .
- Gimeno , I.M. 2004 . “ Future strategies for controlling Marek's disease ” . In Marek's disease , Edited by: Nair , V. and Davison , F. 186 – 198 . Oxford : Elsevier .
- Gimeno , I.M. and Cortes , A.L. 2010 . Evaluation of factors influencing replication of serotype 1 Marek's disease vaccines in the chicken lung . Avian Pathology , 39 : 71 – 79 .
- Gimeno , I. , Witter , R.L. , Fadly , A.M. and Silva , R.F. 2005 . Novel criteria for the diagnosis of Marek's disease virus-induced lymphomas . Avian Pathology , 34 : 332 – 340 .
- Gimeno , I.M. , Witter , R.L. , Hunt , H.D. , Reddy , S.M. and Neumann , U. 2001 . Differential attenuation of the induction by Marek's disease virus of transient paralysis and persistent neurological disease: a model for pathogenesis studies . Avian Pathology , 30 : 397 – 409 .
- Gimeno , I.M. , Witter , R.L. , Hunt , H.D. , Reddy , S.M. and Reed , W.M. 2004 . Biocharacteristics shared by highly protective vaccines against Marek's disease . Avian Pathology , 33 : 59 – 68 .
- Haq , K. , Abdul-Caeer , M.F. , Shanmuganthan , S. , Thanthrige-Don , N. , Read , L.R. and Sharif , S. 2010 . Vaccine-induced host responses against very virulent Marek's disease virus infection in the lung of chickens . Vaccine , 28 : 5565 – 5572 .
- Jones , D. , Brunovskis , P. , Witter , R.L. and Kung , H.J. 1996 . Retroviral insertional activation in a herpesvirus: transcriptional activation of Us genes by an integrated long terminal repeat in a Marek's disease virus clone . Journal of Virology , 70 : 2460 – 2467 .
- Kaspers , B. , Lillehoj , H.S. and Lillehoj , E.P. 1993 . Chicken macrophages and thrombocytes share a common cell surface antigen defined by a monoclonal antibody . Veterinary Immunology and Immunopathology , 36 : 333 – 346 .
- Kim , T. , Mays , J. , Fadly , A. and Silva , R.F. 2011 . Artificially inserting a reticuloendotheliosis virus long terminal repeat into a bacterial artificial chromosome clone of Marek's disease virus (MDV) alters expression of nearby MDV genes . Virus Genes , 42 ( 3 ) : 369 – 376 .
- Lee , L.F. , Liu , X. and Witter , R.L. 1983 . Monoclonal antibodies with specificity for three different serotypes of Marek's disease viruses in chickens . Journal of Immunology , 130 : 1003 – 1006 .
- Lee , L.F. , Kreager , K.S. , Arango , J. , Paraguassu , A. , Beckman , B. , Zhang , H. , Fadly , A. , Lupiano , B. and Reddy , S.M. 2010 . Comparative evaluation of vaccine efficacy of recombinant Marek's disease virus vaccine lacking Meq oncogene in commercial chickens . Vaccine , 28 : 1294 – 1299 .
- Liu , H.-C. , Kung , H.J. , Fulton , J.E. , Morgan , R.M. and Cheng , H.H. 2001 . Growth hormone interacts with the Marek's disease virus SORF2 protein and is associated with disease resistance in chicken . Proceedings of the National Academy of Sciences USA , 98 : 9203 – 9208 .
- Lupiani , B. , Lee , L.F. , Cui , X. , Gimeno , I.M. , Anderson , A. , Morgan , R.W. , Silva , R.F. , Witter , R.L. , Kung , H.-J. and Reddy , S.M. 2004 . Marek's disease virus-encoded Meq gene is involved in transformation of lymphocytes but is dispensable for replication . Proceedings of the National Academy of Sciences USA , 101 : 11815 – 11820 .
- Payne , L.N. and Rennie , M. 1973 . Pathogenesis of Marek's disease in chicks with and without maternal antibody . Journal of the National Cancer Institute , 51 : 1559 – 1573 .
- Purchase , H.G. 1970 . Virus-specific immunofluorescent and precipitin antigens and cell-free virus in the tissues of birds infected with Marek's disease . Cancer Research , 30 : 1898 – 1908 .
- Rabinowitz , Y. 1964 . Separation of lymphocytes, polymorphonuclear leukocytes and monocytes on glass columns, including tissue culture observations . Blood , 23 : 811
- Reddy , S.M. , Lupiani , B. , Gimeno , I.M. , Silva , R.F. , Lee , L.F. and Witter , R.L. 2002 . Rescue of a pathogenic Marek's disease virus with overlapping cosmid DNAs: Use of a pp38 mutant to validate the technology for the study of gene function . Proceedings of the National Academy of Sciences USA , 99 : 7054 – 7059 .
- Rispens , B.H. , Van Vloten , J. , Mastenbroek , N. , Maas , H.J.L. and Schat , K.A. 1972 . Control of Marek's disease in the Netherlands. I. Isolation of an avirulent Marek's disease virus (strain CVI 988) and its use in laboratory vaccination trials . Avian Diseases , 16 : 108 – 125 .
- Schat , K.A. , Calnek , B.W. , Fabricant , J. and Graham , D.L. 1985 . Pathogenesis of infection with attenuated Marek's disease virus strains . Avian Pathology , 14 : 127 – 146 .
- Sevoian , M. , Chamberlain , D.M. and Counter , F.T. 1962 . Avian lymphomatosis. I. Experimental reproduction of the neural and visceral forms . Veterinary Medicine , 57 : 500 – 501 .
- Shek , W.R. , Schat , K.A. and Calnek , B.W. 1982 . Characterization of non-oncogenic Marek's disease virus-infected and turkey-herpesvirus infected lymphocytes . Journal of General Virology , 63 : 333 – 341 .
- Silva , R.F. and Lee , L.F. 1984 . Monoclonal antibody-mediated immunoprecipitation of proteins from cells infected with Marek's disease virus and turkey herpesvirus . Virology , 136 : 307 – 320 .
- St Hill , C.A. , Silva , R.F. and Sharma , J.M. 2004 . Detection and localization of avian alphaherpesviruses in embryonic tissues following in ovo exposure . Virus Research , 100 : 243 – 248 .
- USDA-APHIS ( 2009 ) . Code of Federal Regulations . Title 9, Chapter I, part 113–330
- Witter , R.L. 1997 . Increased virulence of Marek's disease virus field isolates . Avian Diseases , 41 : 149 – 163 .
- Witter , R.L. 2002 . Induction of strong protection by vaccination with partially attenuated serotype 1 Marek's disease viruses . Avian Diseases , 46 : 925 – 937 .
- Witter , R.L. , Lee , L.F. and Fadly , A.M. 1995 . Characteristics of CVI988/Rispens and R2/23, two prototype vaccine strains of serotype 1 Marek's disease virus . Avian Diseases , 39 : 269 – 284 .
- Witter , R.L. , Li , D. , Jones , D. , Lee , L.F. and Kung , H.J. 1997 . Retroviral insertional mutagenesis of a herpesvirus: A Marek's disease virus mutant attenuated for oncogenicity but not for immunosuppression or in vivo replication . Avian Diseases , 41 : 407 – 421 .
- Witter , R.L. and Kreager , K.S. 2004 . Serotype 1 viruses modified by backpassage or insertional mutagenesis: approaching the threshold of vaccine efficacy in Marek's disease . avian Diseases , 48 : 768 – 782 .
- Witter , R.L. and Schat , K.A. 2003 . “ Marek's disease ” . In Diseases of Poultry Code , 11th ed , Edited by: Saif , Y.M. , Barnes , H.J. , Fadly , A.M. , Glisson , J.R. , McDougald , L.R. and Swayne , D.E. 407 – 465 . Ames : Iowa State University Press .