Abstract
Antibodies specific to the non-structural proteins of viruses are detected in virus-infected animals and show promise as a reliable diagnostic marker for virus infections. We examined the potential use of two non-structural proteins of fowl adenovirus (FAdV)-based, 100K and 33K, enzyme-linked immunosorbent assays (ELISAs) in the diagnosis of FAdVs. We cloned and expressed the 100K and 33K non-structural protein genes of the FAdVs in the pGEX-4T-1 plasmid vector. Purified 100K and 33K proteins alone or in combination were used as antigens in ELISAs. Antibodies specific to the 100K and 33K non-structural proteins were detected in chickens experimentally infected with FAdVs, but not in chickens vaccinated with inactivated FAdVs. In contrast, the agar gel precipitation (AGP) test detected FAdV-specific antibodies in 70.3% of the vaccinated chickens, suggesting that the non-structural protein-based ELISA could be used in the differential diagnosis of infected and vaccinated chickens. To further validate the 100K and 33K-based ELISA (100K-33K-ELISA) method, we compared its sensitivity and specificity with that of a whole virus-based ELISA and an AGP test in detecting FAdV-specific antibodies in 350 field samples. The results showed that the 100K-33K-ELISA exhibited a higher sensitivity than the AGP test and a comparable sensitivity and specificity to the whole virus ELISA. Overall, the 100K-33K-ELISA method is sensitive, specific and can be used to distinguish an acute FAdV infection from an inactivated virus-based vaccination response.
Introduction
Fowl adenoviruses (FAdVs) belong to the genus Aviadenovirus of the family Adenoviridae. FAdVs cause a wide range of diseases including respiratory infection, inclusion body hepatitis, infectious hydropericardium, Angara disease and hydropericardium syndrome (Winterfield et al., Citation1973; Dhillon & Winterfield, Citation1984; Mazaheri et al., Citation1998; Nakamura et al., Citation1999). FAdVs encode 10 major structural proteins (pII, pIII, pIV, pIIIa, pVIII, pV, pVII, pX, µ, and TP) and 11 non-structural proteins (E1A, E1B, ADP [E3], E4, pol, DBP [E2A], pIVaII, 52/55K, EP, 100K, and 33K). The 100K non-structural protein is necessary for the efficient translation of viral late mRNA species (Hayes et al., Citation1990). The 33K non-structural protein plays a role in capsid assembly (Morin & Boulanger, Citation1984). The 100K and 33K proteins are both highly immunogenic and antisera generated against them have been used to study their cellular localization (Gambke & Deppert, Citation1981) .
A polymerase chain reaction (PCR) and a whole virus-based enzyme-linked immunosorbent assay (ELISA) are used in the diagnosis of FAdVs (Dawson et al., Citation1980; Xie et al., Citation1999). The ELISA is highly sensitive compared with the traditional agar gel precipitation (AGP) method. A previous study has demonstrated that a recombinant protein-based ELISA greatly reduces non-specific binding reactions and appears to have a higher correlation with serum neutralization titres than the conventional whole virus-based ELISA (Liu et al., Citation2002). Additionally, a non-structural protein-based ELISA has been used successfully to distinguish vaccinated from infected animals for a number of viruses including foot-and-mouth disease virus (Lu et al., Citation2007), avian reovirus (Xie et al., Citation2010) and influenza A virus (Ozaki et al., Citation2001). To our knowledge, a recombinant protein-based ELISA for FAdVs has not so far been reported. In this paper, we investigated the potential use of two non-structural proteins, 100K and 33K, in the development of a recombinant protein-based ELISA for FAdVs. Since inactivated FAdV vaccines have been used in some countries, including China, the successful development of a non-structural protein-based ELISA would not only distinguish an acute infection from a vaccine-induced response, but could also be used as an alternative to a whole virus-based ELISA in clinical diagnosis. Compared with AGP and whole virus ELISA, a recombinant protein-based ELISA should be safer and exhibit higher sensitivity and specificity.
Materials and Methods
Viruses
The FAdV strain CELO obtained from the China Institute of Veterinary Drug Control, Beijing, China, was used in this study. The virus was grown on chicken embryo liver cells prepared from 14-day-old specific pathogen free (SPF) embryos (Merial Inc., Beijing, China) as described previously (Meulemans et al., Citation2001). At 48 h after infection, cells and supernatant were harvested and subjected to three cycles of freezing and thawing. Culture supernatant was collected after centrifugation at 10,000 × g for 15 min. The virus titre was determined in 96-well microtitre plates as described previously (Chen et al., Citation2011).
Serum samples
SPF chicken embryos were purchased from Merial Inc. and used for the production of SPF chickens in our laboratory. The 110 SPF chickens were randomly divided into three groups. One group of 30, 4-week-old chickens was inoculated intranasally with log10 5.5 median tissue culture infectious doses (TCID50)/ml CELO virus using a nebulizer, every 2 weeks for a total of three times. Blood was collected 2 weeks after the third inoculation. Another group of 30, 2-week-old SPF chickens was vaccinated with log10 5.5 TCID50/ml inactivated FAdV intramuscularly every 2 weeks for a total of three times. Blood samples were collected 2 weeks after the third vaccination. FAdV was inactivated with 0.1% formaldehyde at 37° C overnight and then emulsified in mineral oil composed of equal volumes of Span-80 (GOODWAY, Shanghai, China) and 10# white oil (SINOPEC, Hangzhou, China). The efficacy of the inactivated vaccine has been reported previously (Wei et al., Citation2011a, Citationb). Fifty SPF chickens were used as negative controls. Blood samples were collected at 10 weeks of age. Animal protocols used in this study were approved by the Institutional Animal Care and Use Committee (Approval # A001-2010).
Control antisera
Serum samples known to be positive for infectious bursal disease virus, Newcastle disease virus, egg drop syndrome and avian influenza virus were stored at the Guangxi Veterinary Research Institute.
Cloning of the 100K and 33K genes into the pGEX-4T-1 plasmid vector
DNA extraction from CELO virus was performed as described previously (Xie et al., Citation1999). For the 100K gene, the forward primer (5’-CGGAATTCTCATCGAAAATGGCAGACAAGAT3-’) and the reverse primer (5-'TTGAATTCCCCGAGACGCCATTTAGGTA-3’) were designed and used to amplify the 1062 base pairs of 100K-encoding gene. Primers for 33K (forward, 5’-CCGGAATTCTCTCTCCCTCATTTCT-3’; reverse, 5’-CCGGAATTCCTTGATAGCATCGCTCTTC-3’) were used to amplify the 288 base pairs of 33K-encoding gene (accession number U46933.1). PCR amplification was performed with the PCR master mix kit (TIANGEN, Beijing, China) by using 1 µl (20 ng) DNA template and 50 pmol each primer in a 25 µl reaction volume by following the manufacturer's protocol with the following cycling times and temperatures: 94°C for 5 min and 35 cycles of 94°C for 1 min, 55°C for 1 min and 72°C for 1 min. The PCR product was digested with ECoRI, and then ligated into ECoRI-digested pGEX-4T-1 (GE Healthcare, Piscataway, NJ, USA). The ligation mixture was transformed into Escherichia coli BL21 competent cells. Plasmids containing 100K or 33K genes were identified by PCR and confirmed by DNA sequencing (TAKARA, Dalian, China).
Expression and purification of recombinant 100K-GST and 33K-GST fusion proteins
Recombinant bacteria were grown in 5 ml Luria broth (LB) medium containing 100 µg/ml ampicillin overnight at 37°C. The culture was then added to 1000 ml LB medium containing ampicillin (100 µg/ml) and cultured for approximately 3 h in an orbital shaker at 37°C. When the optical density at 600 nm value of the culture reached 0.55 to 0.65, 1 mM isopropyl β-d-1-thiogalactopyranoside (Sigma, St Louis, MO, USA) was added to induce the expression of cloned 100K and 33K genes. After incubation for 5 h at 30°C, the recombinant glutathione S-transferase (GST) fusion proteins and GST protein were purified using the following procedure. First, the concentrated cell pellets were resuspended in 10 ml phosphate-buffered saline (PBS) supplemented with lysozyme to a final concentration of 100 mg/ml. The mixture was incubated at room temperature for 15 min and sonicated on ice until the solution was no longer viscous. The mixture was then clarified by centrifugation at 10,000 × g for 15 min. The supernatant containing the solubilized protein was collected for subsequent binding to GST-agarose resin (GST binding resin; Glutathione Sepharose™, 4B, lot 0074574011; GE Healthcare) according to the manufacturer's instructions. The protein concentration was determined according to the Lowry method (Lowry et al., Citation1951). The purified 100K-GST and 33K-GST proteins were diluted 1:1 in glycerol and stored at −70°C for use as antigens in the ELISA.
To further confirm the expression of the recombinant GST fusion proteins, western blotting was performed using serum collected from either FAdV-infected chickens or vaccinated chickens. Briefly, the membrane was washed three times in PBS containing 0.05% Tween 20 (PBST) (20 sec/wash) and then incubated with goat anti-chicken IgG-horseradish peroxidase conjugate (KPL Inc., Gaithersburg, MD, USA) for 30 min at room temperature. Following three washes with PBST at room temperature, the membrane was incubated with the DAB Color Development Kit (TIANGEN).
Indirect 100K-ELISA, 33K-ELISA, and100K-33K-ELISA
To determine the optimal concentrations of recombinant proteins for use in the ELISA, checkerboard titrations were carried out for the 100K-GST and 33K-GST fusion protein alone and in combination using known FAdV-positive and FAdV-negative sera. Purified 100K and 33K proteins were used at a series of dilutions ranging from 10.8 to 1.4 µg/ml and 12 to 2 µg/ml, respectively, in 0.05 M carbonate buffer (pH 9.6). To increase the specificity and sensitivity of the ELISA, different combinations of 100K-GST and 33K-GST fusion proteins were tested. One hundred microlitres of antigen was added to the ELISA plate and incubated at 4°C overnight. The wells were washed three times with PBST, and then blocked with 50 µl blocking buffer (PBS, 0.05% Tween 20, and 2.5% bovine serum) for 1 h at 37°C to saturate all unbound sites. Coated plates were then washed three times with PBST. Chicken sera were diluted in a series of dilutions ranging from 1:50 to 1:400 using 1% bovine serum albumin, then added at 100 µl/well and incubated for 1 h at 37°C. After incubation, the plates were washed three times. One hundred microlitres per well of a 1:3000 dilution of horseradish peroxidase-labelled goat anti-chicken IgG (KPL, Inc.) was added, and the plates were incubated for 1 h at 37°C and then washed three times. The colour was developed using the chromogen/substrate mixture TMB/H2O2 (Sigma). After 10 min, the reaction was stopped by the addition of 2.0 M H2SO4. The absorbance was read at 450 nm using a plate reader. Duplicate positive and negative control chicken sera were included on each plate. In addition, 5 µg/ml purified GST protein was used as a coating antigen and served as a negative control. For the whole virus ELISA, CELO virus was purified by the sucrose density gradient centrifugation method and diluted 10-fold for use as the coating antigen.
To determine the cut-off value, 50 known negative serum samples and 30 known positive serum samples were tested using the 100K-ELISA, 33K-ELISA and 100K-33K-ELISA to calculate the average and standard deviation (SD) of the optical density at 450 nm values. The cut-off value was calculated using the formula: cut-off values = ±3SD.
Serum samples from commercial chicken flocks
Three hundred and fifty serum samples were collected from Guangxi province. Two hundred sera were randomly collected from chicken farms that were experiencing FAdV infections in some counties of the province during 2010. One hundred and fifty chickens that were known to be FAdV-negative were vaccinated with an inactivated commercial FAdV vaccine (Xinxing, Nanning, China). Blood samples were collected 6 weeks after vaccination. These serum samples came from different chicken farms. The sera were tested for FAdV by the 100K-ELISA, 33K-ELISA, 100K-33K-ELISA, GST-ELISA, AGP and whole virus ELISA developed in our laboratory. The detection rate of each method was determined.
Results
Expression and purification of 100K-GST and 33K-GST fusion proteins
Analysis of the purified proteins by SDS-PAGE revealed the expression of 100K-GST and 33K-GST fusion proteins with an approximate molecular mass of 64.5 kDa and 36.7 kDa respectively (). The concentrations of the 100K-GST and 33K-GST fusion proteins were 1.08 mg/ml and 0.6 mg/ml, respectively. The blurred band below the 33K-GST protein in was thought to be a degraded form of 33K-GST protein, possibly due to the ultrasonic homogenization procedure used. The degraded smaller protein was not detected in the western blotting analysis (), suggesting that the truncated 33K-GST protein did not react with FAdV antiserum. Thus it was not expected to affect the ELISA.
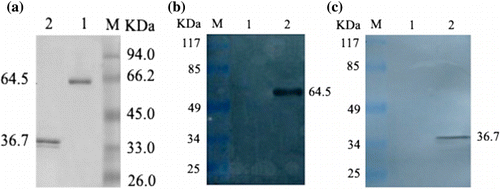
To further confirm the expression of the expected proteins, western blotting analysis was used. As shown in , the purified proteins reacted strongly with FAdV-positive serum, but not with FAdV-negative serum (data not shown).
Optimization of 100K-ELISA, 33K-ELISA and 100K-33K-ELISA
Checkerboard titrations were used to determine the optimal conditions for the 100K-ELISA, 33K-ELISA and 100K-33K-ELISA. Representative results are shown in . The optimal concentrations for 100K, 33K and 100K-33K fusion proteins were 5.4 µg/ml, 6 µg/ml, and 5.4 + 6 µg/ml, respectively. The serum dilutions of 1:100 gave a clear difference in absorbance values between the positive and negative sera.
Table 1. Optimal concentrations of recombinant proteins and of serum dilutions used in ELISAs as determined by checkerboard titration assays.
Determination of the cut-off values
The average and the SD of 50 known negative sera collected from 10-week-old SPF chickens were used to calculate the cut-off values using the formula: cut-off values = ±3SD. The cut-off values for the 100K-ELISA, 33K-ELISA and 100K-33K-ELISA were 100K-ELISA > 0.345, 33K-ELISA > 0.316, and 100K-33K-ELISA > 0.342.
Detection of FAdV-specific antibodies with non-structural protein-based ELISAs and AGP
The 100K-ELISA, 33K-ELISA and 100K-33K-ELISA were used to detect FAdV-specific antibodies in sera collected from the experimentally infected and vaccinated chickens. All three ELISAs were able to detect antibodies in all the sera from chickens experimentally infected with FAdV, whereas the AGP test only detected antibodies in 62.1% of the serum samples from the experimentally infected chickens. Interestingly, the 100K-ELISA, 33K-ELISA and 100K-33K-ELISA failed to detect any antibodies in the sera collected from experimentally vaccinated chickens, whereas by the AGP method antibodies were detected in 70.3% of the chickens experimentally vaccinated with FAdV. The results suggest that the 100K and 33K non-structural protein-based ELISA could differentiate the response to FAdV vaccination from that following an acute infection.
Validation of the non-structural protein-based ELISA for use to test serum samples from the field
A total of 350 field serum samples were collected from different farms that were either experiencing FAdV infection or were vaccinated using inactivated FAdV. The presence of FAdV-specific antibodies in these samples was examined by AGP, the whole virus ELISA developed in our laboratory and the non-structural protein-based ELISAs. The results are summarized in . The non-structural protein-based ELISA only detected antibodies in samples collected from infected farms, but not from vaccinated farms, further confirming the specificity of these ELISAs in distinguishing the response to infection from that following vaccination. The detection rates for the 100K-ELISA, 33K-ELISA and 100K-33K-ELISA were 35.5% (71/200), 32.5% (65/200) and 38% (76/200) respectively, while the detection rates for the whole virus ELISA and the AGP were 40.5% (81/200) and 26.5% (53/200) respectively. The 100K-33K-ELISA showed the highest sensitivity among the three non-structural protein-based ELISAs, but this was a little lower than that found using the whole virus ELISA. The optical density values for these sera ranged from 0.45 to 1.8. This may reflect the extent of virus activity in the chickens and the timing of sample collection. Moreover, the detection rate for the GST-ELISA was 0%, indicating that the results obtained with the recombinant protein-base ELISAs were not influenced by the GST-target protein. The 100K-ELISA, 33K-ELISA and 100K-33K-ELISA showed no cross-reaction with known positive serum samples against infectious bursal disease virus, Newcastle disease virus, egg drop syndrome or avian influenza virus (data not shown), which indicated that these indirect ELISAs are specific for the detection of FAdV antibodies.
Table 2. Comparison of different methods for detecting FAdV-specific antibodies in field samples.
Discussion
Several methods have been developed to detect antibodies against FAdVs, including AGP (Cowen, Citation1987), virus neutralization (Calnek et al., Citation1982), and ELISA (Dawson et al., Citation1980). Because the ELISA technique has a high level of sensitivity and reproducibility and allows for automation, it is the method of choice for screening a large number of serum samples.
Whole virus lysate-based ELISAs have been widely used to detect the virus-specific antibody response. However, non-specific reactions are more likely to occur using the whole virus lysate-based ELISA as has been shown for reoviruses (Islam & Jones, Citation1988). Furthermore, the use of whole virus lysates as the coating antigen leads to the danger of spreading live virus particles. Previous reports have demonstrated that using two proteins as the coating antigens in ELISAs used for detecting antibodies greatly reduces non-specific binding reactions and gives a higher correlation with serum neutralization assays than does the conventional whole virus-based ELISA (Islam & Jones, Citation1988; Liu et al., Citation2002). Non-structural proteins are normally expressed abundantly in virus-infected cells, but they are not detected in the virions (Krug & Etkind, Citation1973). It has also been reported for influenza virus and reovirus that a non-structural protein-based ELISA might be able to distinguish the vaccination response from that following an acute viral infection (Ozaki et al., Citation2001; Xie et al., Citation2010).
In this study, we cloned two non-structural protein genes (100K and 33K) of CELO FAdV into a pGEX-4T-1 vector to generate recombinant GST fusion proteins. The potential use of these two GST fusion proteins in capturing antibodies specific to the two non-structural proteins was evaluated in experimentally infected and in vaccinated chickens. The results showed that the 100K-ELISA, 33K-ELISA and 100K-33K-ELISA were able to differentiate experimentally infected chickens from vaccinated ones, while the conventional whole virus-based ELISA and the AGP test could not distinguish an acute infection response from that following vaccination. All 30 sera collected from experimentally infected chickens were found to be positive by these ELISAs. In contrast, none of the experimentally vaccinated chickens were positive for FAdV. Overall, the 100K-ELISA, 33K-ELISA and 100K-33K-ELISA have advantages over the conventional whole virus-based ELISA (Dawson et al., Citation1980; Cowen, Citation1987), with less non-specific binding reactions and high sensitivity, and could be used as a differential diagnostic tool to distinguish infected chickens from birds given inactivated virus vaccines. The availability of such diagnostic tests will allow researchers to gather accurate epidemiological data on FAdVs, which in turn will greatly facilitate the regional elimination of FAdVs.
Formaldehyde can effectively inactivate a number of viruses including poliovirus, human enterovirus 71, Japanese encephalitis virus, foot-and-mouth disease virus, enteroviruses, Ross River virus, and human immunodeficiency virus (Martin et al., Citation2003; Appaiahgari & Vrati, Citation2004; Barteling & Cassim, Citation2004; Poon et al., Citation2005; Kistner et al., Citation2007; Chen et al., Citation2011). In addition, formaldehyde has been shown to be suitable for commercial vaccine production due to its low cost. In our previous study, we compared the effect of formaldehyde and β-propriolactone for the inactivation of FAdV. Treatment of FAdV with 0.1% formaldehyde or with 0.05% β-propriolactone effectively inactivated virus activity (Wei et al., Citation2011a, Citationb). Although inactivation with formaldehyde can have a protein altering effect, the data reported here show that the ELISAs we developed were not negatively influenced by the inactivation procedure; this finding is important, because all of the current FAdV vaccines used in China are inactivated by formaldehyde treatment.
We used the 100K-ELISA, 33K-ELISA and 100K-33K-ELISA to detect FAdV-specific antibodies from 350 field samples collected from Guangxi province in China. A good correlation in sensitivity and specificity was observed between the three ELISAs and also with the whole virus-based ELISA. Furthermore, these non-structural protein-based ELISAs, but not the AGP test or the whole virus-based ELISA, were capable of distinguishing infected chickens from vaccinated ones, supporting the data we obtained from the experimentally infected and vaccinated chickens. Overall, the results reported here suggest that these non-structural protein-based ELISAs can be used in screening for the prevalence of FAdV in chickens and in the differential diagnosis of acute infections and responses to vaccination. Since there are many different serotypes of FAdV (Cowen, Citation1987; McFerran & Smyth, Citation2000), it remains to be determined whether these non-structural protein-based ELISAs can be used in the detection of all of the different serotypes.
Acknowledgements
This work was supported by National Bai Qian Wan Talents Engineering Foundation (945200603) and Guangxi Government Senior Scientist Foundation (2011B020).
References
- Appaiahgari, M.B. & Vrati, S. (2004). Immunogenicity and protective efficacy in mice of a formaldehyde-inactivated Indian strain of Japanese encephalitis virus grown in Vero cells. Vaccine, 22, 3669–3675. doi:10.1016/j.vaccine.2004.03.024
- Barteling, S.J. & Cassim, N.I. (2004). Very fast (and safe) inactivation of foot-and-mouth disease virus and enteroviruses by a combination of binary ethyleneimine and formaldehyde. Developmental Biology, 119, 449–455.
- Calnek, B.W., Shek, W.R., Menendez, N.A. & Stiube, P. (1982). Serological cross-reactivity of avian adenovirus serotypes in an enzyme-linked immunosorbent assay. Avian Diseases, 26, 897–906. doi:10.2307/1589878
- Chen, C.W., Lee, Y.P., Wang, Y.F. & Yu, C.K. (2011). Formaldehyde-inactivated human enterovirus 71 vaccine is compatible for co-immunization with a commercial pentavalent vaccine. Vaccine, 29, 2772–2776. doi:10.1016/j.vaccine.2011.01.094
- Cowen, B.S. (1987). A trivalent antigen for the detection of type I avian adenovirus precipitin. Avian Diseases, 31, 351–354. doi:10.2307/1590884
- Dawson, G.J., Orsi, L.N., Yates, V.J., Chang, P.W. & Pronovost, A.D. (1980). An enzyme-linked immunosorbent assay for detection of antibodies to avian adenovirus and avian adenovirus-associated virus in chickens. Avian Diseases, 24, 393–402. doi:10.2307/1589706
- Dhillon, A.S. & Winterfield, R.W. (1984). Pathogenicity of various adenovirus serotypes in the presence of Escherichia coli in chickens. Avian Diseases, 28, 147–153. doi:10.2307/1590136
- Gambke, C. & Deppert, W. (1981). Late nonstructural 100,000- and 33,000-dalton proteins of adenovirus type 2. I. Subcellular localization during the course of infection. Journal of Virology, 40, 585–593.
- Hayes, B.W., Telling, G.C., Myat, M.M., Williams, J.F. & Flint, S.J. (1990). The adenovirus L4 100-kilodalton protein is necessary for efficient translation of viral late mRNA species. Journal of Virology, 64, 2732–2742.
- Islam, M.R. & Jones, R.C. (1988). An enzyme-linked immunosorbent assay for measuring antibody titre against avian reovirus using a single dilution of serum. Avian Pathology, 17, 411–425. doi:10.1080/03079458808436459
- Kistner, O., Barrett, N., Bruhmann, A., Reiter, M., Mundt, W., Savidis-Dacho, H., Schober-Bendixen, S., Dorner, F. & Aaskov, J. (2007). The preclinical testing of a formaldehyde inactivated Ross River virus vaccine designed for use in humans. Vaccine, 25, 4845–4852. doi:10.1016/j.vaccine.2007.01.103
- Krug, R.M. & Etkind, P.R. (1973). Cytoplasmic and nuclear virus-specific proteins in influenza virus-infected MDCK cells. Virology, 56, 334–348. doi:10.1016/0042-6822(73)90310-3
- Liu, H.J., Kuo, L.C., Hu, Y.C., Liao, M.H. & Lien, Y.Y. (2002). Development of an ELISA for detection of antibodies to avian reovirus in chickens. Journal of Virological Methods, 102, 129–138. doi:10.1016/S0166-0934(02)00010-1
- Lowry, O.H., Rosebrough, N.J., Farr, A.L. & Randall, R.J. (1951). Protein measurement with the Folin phenol reagent. Journal of Biological Chemistry, 193, 265–275.
- Lu, Z., Cao, Y., Guo, J., Qi, S., Li, D., Zhang, Q., Ma, J., Chang, H., Liu, Z., Liu, X. & Xie, Q. (2007). Development and validation of a 3ABC indirect ELISA for differentiation of foot-and-mouth disease virus infected from vaccinated animals. Veterinary Microbiology, 125, 157–169. doi:10.1016/j.vetmic.2007.05.017
- Martin, J., Crossland, G., Wood, D.J. & Minor, P.D. (2003). Characterization of formaldehyde-inactivated poliovirus preparations made from live-attenuated strains. Journal of General Virology, 84, 1781–1788. doi:10.1099/vir.0.19088-0
- Mazaheri, A., Prusas, C., Voss, M. & Hess, M. (1998). Some strains of serotype 4 fowl adenoviruses cause inclusion body hepatitis and hydropericardium syndrome in chickens. Avian Pathology, 27, 269–276. doi:10.1080/03079459808419335
- McFerran, J.B. & Smyth, J.A. (2000). Avian adenoviruses. Revue Scientifique et Technique, 19, 589–601.
- Meulemans, G., Boschmans, M., Berg, T.P. & Decaesstecker, M. (2001). Polymerase chain reaction combined with restriction enzyme analysis for detection and differentiation of fowl adenoviruses. Avian Pathology, 30, 655–660. doi:10.1080/03079450120092143
- Morin, N. & Boulanger, P. (1984). Morphogenesis of human adenovirus type 2: sequence of entry of proteins into previral and viral particles. Virology, 136, 153–167. doi:10.1016/0042-6822(84)90256-3
- Nakamura, K., Mase, M., Yamaguchi, S., Shibahara, T. & Yuasa, N. (1999). Pathologic study of specific-pathogen-free chicks and hens inoculated with adenovirus isolated from hydropericardium syndrome. Avian Diseases, 43, 414–423. doi:10.2307/1592638
- Ozaki, H., Sugiura, T., Sugita, S., Imagawa, H. & Kida, H. (2001). Detection of antibodies to the nonstructural protein (NS1) of influenza A virus allows distinction between vaccinated and infected horses. Veterinary Microbiology, 82, 111–119. doi:10.1016/S0378-1135(01)00366-2
- Poon, B., Safrit, J.T., McClure, H., Kitchen, C., Hsu, J.F., Gudeman, V., Petropoulos, C., Wrin, T., Chen, I.S. & Grovit-Ferbas, K. (2005). Induction of humoral immune responses following vaccination with envelope-containing, formaldehyde-treated, thermally inactivated human immunodeficiency virus type 1. Journal of Virology, 79, 4927–4935. doi:10.1128/JVI.79.8.4927-4935.2005
- Wei, Y., Xie, Z., Fan, Q., Liu, J., Xie, L., Pang, Y., Deng, X. & Xie, Z. (2011a). Development of an inactivated vaccine against infectious fowl adenovirus Group I serum type 1 strain. Progress in Veterinary Medicine, 32, 14–19.
- Wei, Y., Xie, Z., Fan, Q., Liu, J., Xie, L., Pang, Y., Deng, X. & Xie, Z. (2011b). Development of bivalent inactivated oil-emulsion vaccine for fowl adenovirus group-I. Journal of Southern Agriculture, 42, 1550–1554.
- Winterfield, R.W., Fadly, A.M. & Gallina, A.M. (1973). Adenovirus infection and disease. I. Some characteristics of an isolate from chickens in Indiana. Avian Diseases, 17, 334–342. doi:10.2307/1589217
- Xie, Z., Fadl, A.A., Girshick, T. & Khan, M.I. (1999). Detection of avian adenovirus by polymerase chain reaction. Avian Diseases, 43, 98–105. doi:10.2307/1592767
- Xie, Z., Qin, C., Xie, L., Liu, J., Pang, Y., Deng, X., Xie, Z. & Khan, M.I. (2010). Recombinant protein-based ELISA for detection and differentiation of antibodies against avian reovirus in vaccinated and non-vaccinated chickens. Journal of Virological Methods, 165, 108–111. doi:10.1016/j.jviromet.2009.12.008