ABSTRACT
Veterinary vaccines contribute to food security, interrupt zoonotic transmissions, and help to maintain overall health in livestock. Although vaccines are usually cost-effective, their adoption depends on a multitude of factors. Because poultry vaccines are usually given to birds with a short life span, very low production cost per dose is one important challenge. Other hurdles are to ensure a consistent and reliable supply of very large number of doses, and to have flexible production processes to accommodate a range of different pathogens and dosage requirements. Most poultry vaccines are currently being produced on primary avian cells derived from chicken or waterfowl embryos. This production system is associated with high costs, logistic complexities, rigid intervals between harvest and production, and supply limitations. We investigated whether the continuous cell lines Cairina retina and CR.pIX may provide a substrate independent of primary cell cultures or embryonated eggs. Viruses examined for replication in these cell lines are strains associated with, or contained in vaccines against egg drop syndrome, Marek's disease, Newcastle disease, avian influenza, infectious bursal disease and Derzsy's disease. Each of the tested viruses required the development of unique conditions for replication that are described here and can be used to generate material for in vivo efficacy studies and to accelerate transfer of the processes to larger production volumes.
Introduction
Animal infectious diseases are a cause for losses to biodiversity (Fey et al., Citation2015) and a risk to human health due to zoonotic potentials of the causative agents (Sehgal, Citation2010; Häsler et al., Citation2013; Jones et al., Citation2013). Infectious diseases that threaten livestock can also lead to economic damages and contribute to persistent poverty (Normile, Citation2008; Narrod et al., Citation2012; World Health Organization, Citation2013). Epidemiological vulnerability in the poultry sector is enhanced by the high density of birds in industrial farming, a large global population of at least 19 billion chickens and 1 billion ducks, opportunities for contact between free-range and migratory birds, potential for coinfection with different pathogens and different strains of the same pathogen, and legal and illegal trade with live birds and poultry products across continents (Cavanagh, Citation2007; Galloway et al., Citation2007; van den Berg, Citation2009; Hosseini et al., Citation2010; Conan et al., Citation2012).
Vaccination of humans (Ehreth, Citation2003; Andre et al., Citation2008) and birds (Zinsstag et al., Citation2007; Vos et al., Citation2014) can be an efficacious and economic intervention for the control of infectious diseases. Veterinary vaccination is also perceived as the ethically and often epidemiologically preferred approach to avoid mass slaughter and culling of birds (O'Brien & Zanker, Citation2007; Clifton, Citation2011). However, the pressure to provide affordable veterinary vaccines is very high, and whether a vaccine is considered to be cost-effective depends on zoonotic capacity, virulence and transmissibility of the pathogen in animal populations, geographic area, number of required doses, potential effects on livestock productivity, and whether consumer worries can reduce demands for an agricultural product (McLeod & Rushton, Citation2007; Narrod et al., Citation2012; Monath, Citation2013; Fitzpatrick et al., Citation2014). One consequence of these considerations is that vaccine production is optimized for industrial, but less so for non-commercial and semi-intensive poultry keepers.
Dependence on embryonated eggs for production of many poultry vaccines may contribute to this bias. Farmers interviewed for a study about chicken farming in Ethiopia reported that vaccines were “only intermittently available and then only in inappropriate volumes”, and that vaccine production “was undertaken by a local facility, but this institution faced challenges procuring regular supplies of the raw materials (particularly Specified Pathogen Free eggs) necessary to maintain production” (quotations are verbatim from Sambo et al., Citation2015). Affordability and supply security may also be affected by dependence on eggs. One-third of the total production costs for certain avian vaccines is reported to be incurred by the preparation of the primary chicken cells (Osterrieder & Schumacher, Citation2002) and safe disposal of solid biohazardous waste that accumulates if vaccines are being produced in egg cavities requires additional resources (Ackland et al., Citation1985). Rigid intervals between husbandry, harvest of eggs, and inoculation with vaccine seed must be accommodated (Uscher-Pines et al., Citation2008) and there is an elevated risk of contamination with environmental and endogenous agents (Böni et al., Citation1996; Enserink, Citation2004; Chua & Chen, Citation2010).
We have investigated an alternative option for the production of poultry vaccines that may be suitable in environments with limited resources as well as in an industrial setting that can manipulate very large volumes. The described technologies are based on the continuous cell lines Cairina retina (CR) and CR.pIX that originate from embryonal primary retina cells of a domesticated Muscovy duck (Jordan et al., Citation2009a). Immortalization was achieved by liposomal transfection of an expression plasmid for the E1A and E1B genes of the human adenovirus 5 (hAdV-5). The CR.pIX cell line was derived from established CR cells by stable transfection of an additional expression plasmid that encodes pIX. This protein contributes to the stability of the adenoviral capsids but may also have regulatory effects (Parks, Citation2005; Vellinga et al., Citation2005). We observed previously that expression of pIX in CR cells renders the cells more sensitive to geldanamycin, an inhibitor that is specific for activated Hsp90 (Jordan et al., Citation2009b). Expression of pIX may thus be able to augment or interfere with virus propagation because the infectious cycle of some viruses intersects with heat shock responses that are mediated by Hsp90 (Hung et al., Citation2002; Connor et al., Citation2007).
The cells were shown to be free of adventitious agents, and sensitive assays for reverse transcriptase activity suggest that the anatine cell lines (as opposed to galline material (Böni et al., Citation1996)) do not release active endogenous retroviral particles (Sandig & Jordan, Citation2008; Jordan et al., Citation2013). The cell lines have furthermore been adapted to proliferation in suspension in chemically defined media, and propagation of recombinant and wild type viruses of different families to high yields has been demonstrated in single-cell suspension and adherent culture formats (Jordan & Sandig, Citation2008; Lohr et al., Citation2009; Jordan et al., Citation2011; Lohr et al., Citation2012; Mészáros et al., Citation2014).
A potential replacement for embryonated eggs should be permissive for a similarly wide spectrum of viruses as the conventional substrate. This publication describes production parameters for viruses and vaccine strains from different viral families implicated in poultry diseases, namely egg drop syndrome (EDS), infectious bursal disease (IBD), Marek's disease (MD), Newcastle disease (ND), avian influenza, and Derzsy's disease. The developed protocols can be used in subsequent studies for transfer of the processes to larger volumes and for preparation of material to investigate efficacy of candidate vaccines in vivo.
Material and methods
Cells and viruses
The CR and CR.pIX cell lines and the development of the chemically defined media have been described previously (Jordan et al., Citation2009a; Jordan et al., Citation2011). The CD-U3 and CD-VP4 media used in this study were manufactured by Biochrom (catalogue numbers F9185 and F9127, respectively). Experiments were performed with viruses of the China Institute of Veterinary Drug Control (Beijing, China), Ceva Phylaxia (Budapest, Hungary), Hungarian Academy of Sciences (Budapest, Hungary), and the Friedrich-Löffler-Institut (Insel Riems, Germany).
Determination of infectious units
Infectious titres of Newcastle disease virus (NDV) and H9N2 were determined on Vero cells. 1.5 × 106 cells in DMEM:F12 medium containing 2 mM GlutaMAX I (both Gibco) and 5% foetal calf serum (Biochrom) were seeded into CellBIND 96-well plates (Corning) at 100 µl of cell suspension. The medium was replaced on the following day against DMEM:F12 containing 2 mM GlutaMAX I and 1.5 µg/ml trypsin (type IX-S, Sigma T0303), but no foetal calf serum. Serial dilutions in steps of 10 of NDV or H9N2-containing virus samples were prepared in DMEM:F12 medium free of serum, and 10 µl each of the dilutions were added to the Vero cultures. Virus replication was allowed at 37°C for 72 h.
Detection of NDV replication was facilitated by immunostaining: the cells were fixed in methanol for 10 min, allowed to dry to completion, and rehydrated with PBS containing 0.05% Tween-20. NDV antiserum (GD Animal Health Deventer, the Netherlands) was added to a dilution of 1:2000 in PBS containing 1% foetal calf serum and incubated for 1 h at room temperature. After two washes with PBS, secondary antibody (anti-chicken, Alexa Fluor 488 labelled, host rabbit, Dianova, 303-545-003 at 1 µg/µl) was added at a dilution of 1:2000 for 2 h at ambient temperature or overnight at 4°C. Infected wells were identified by fluorescence after two washes with PBS. Calculation of TCID50 values was performed according to Spearman and Kärber using the implementation of the Food and Agriculture Organization (Bross, Citation1950; Litamoi et al., Citation1996).
Infectious titres of egg drop syndrome virus (EDSV) were determined on CS (Carinia somite) cells, a fibroblastoid and strictly adherent relative of the CR cell line (Jordan et al., Citation2009a). Similar to titration of NDV, indicator cells were seeded (at 1 × 106 cells/plate) one day prior to infection. Virus samples were applied in 10-fold serial dilutions without change of medium or addition of trypsin, and replication was allowed for up to 5 days at 37°C. Identification of infected wells was facilitated by immunostaining with primary antibody (GD Animal Health, VLDIA050) at 1:2000 and secondary antibody conjugated to Alexa Fluor 488 at 1:2000 after fixation with methanol.
Infectious bursal disease virus (IBDV) titres were determined on adherent CR.pIX cells. Infection was performed with samples in 10-fold serial dilutions, one day after the indicator cells were seeded at 4 × 106 per 96-well plate. Wells with cytopathic effect (CPE) were identified microscopically (without immunostaining) 3 days post infection. As for EDSV, a change of medium or addition of trypsin was not performed to titre this virus.
The parvoviruses seeds were originally titrated on goose embryo fibroblasts and the passages described here were titrated on CR.pIX cells. Virus replication was allowed for 7 days after application of the 10-fold serial dilutions. Cytopathic effects can be difficult to detect and infection therefore was verified by detection of viral antigens with enzyme-linked immunosorbent assays (ELISA). Quantitative ELISA was performed in a modification of (Palya et al., Citation2009) with polyclonal antibodies against duck or goose parvoviruses (GPVs), respectively, that were biotinylated and used in a sandwich method with streptavidin-labelled peroxidase for visualization.
Haemagglutination assay
Haemagglutination units (HAU) as correlates for number of viral particles were determined with chicken erythrocytes and serial dilutions of infected cell suspensions in PBS in steps of 2. Erythrocytes from 7 ml of a 25% complete blood suspension in Alsever buffer (E200, Labor DrMerk & Kollegen GmbH, Ochsenhausen, Germany) were washed thrice in cold PBS and diluted in PBS to a concentration that yields an optical density of 2.9–3.1 at 576 nm. This suspension was stored for up to one week at 4°C. Centrifugation for the washing steps was performed at room temperature with 500 × g, brake set to low, for 5 min each.
The assay was performed in round-bottom 96-well microtitre plates. The first row of the plate was filled with 200 µl of the sonicated (NDV) or crude culture supernatant (H9N2) and all other rows received 100 µl of PBS. Next, 100 µl of virus sample was added to the second row, the pipetting tips were replaced and the suspension mixed with fresh tips. This two-fold dilution was continued serially into the next row, and, for samples with high potency, continued into a second plate. Each well thus contained 100 µl of diluted virus sample and was subsequently supplemented with 100 µl of the erythrocyte suspension. This volume was mixed by pipetting and the turbid solution allowed to stand undisturbed for 60 min at ambient temperature (22°C). HAU/100 µl are given by the highest dilution where formation of the characteristic dot was not yet visible.
Some samples were further homogenized by sonication with a sonotrode H3 (Hielscher, Germany) of 3 mm diameter powered by an UP400S device at 50% pulse and 50% amplitude for 5 min (100 ml). This step increased HA titres of NDV by 2 to 4 dilution steps but was not performed in all experiments.
Detection and titration by qPCR
DNA was isolated with the QIAamp DNA Blood Mini kit (Qiagen). Purified DNA was eluted into 100 µl, and 5 µl thereof were added to PCR reactions. Alternatively, DNA was isolated from 20 µl of infected cell culture suspension by addition of 5 µl of QuickExtract DNA Extraction Solution 1.0 (101094, Biozym). This sample was heated to 65°C for 15 min and to 98°C for 5 min. After dilution with 50 µl of water, 5 µl therefrom was added to a PCR reaction with a final volume of 25 µl.
Quantitative PCR against EDSV was performed using the Power SYBR Green PCR Master Mix (Applied Biosystems) in an ABI 7000 unit. Primers were added to 70 nM each per reaction and real-time PCR was launched with a single incubation for 10 min at 95°C, followed by 40 cycles of 94°C for 15 seconds and 60°C for 1 min. The primer sequences were TgA CTC Cgg TCC TTC TAA CAC A and TCA Cgg CAA CTg gTT TAA Tgg for detection of the E1A gene used to immortalize the avian host cell as internal reference (Jordan et al., Citation2009b), and gAg CgT gAT CAA gTC gAT gg and CAC TTA CCg CgA gCA TAT CC against the polymerase of EDSV (designed using GenBank sequence with accession number Y09598). Amplification of the genomic DNA was calculated via an adaptation described previously for relative quantification of gene expression (Pfaffl, Citation2001). We first determined the differences in the ct values obtained with primers against viral genome and against cellular DNA relative to the first data point, usually 2 h post infection. The differences were raised to the power of 2, assuming a uniform efficiency of 2.0 for each cycle of amplification, and the ratios of changes of viral to cellular DNA, each relative to the first data point, is the burst number.
Herpesvirus of turkey (HVT) titres were calculated similarly, but here, because of the inoculation with infected primary chicken embryo fibroblasts (CEFs) that do not contain a cellular reference, we did not normalize to an arbitrary initial time of infection, but rather depicted the ratio of viral to cellular DNA (as “genome equivalents”) for each passage. The primers used to detect E1A were the same that were used in the EDSV study and primers against the cellular pIX gene were TTg TgA CTg ACT TTg CTT TCC and AgA gCC gTC AAC TTg TCA TC, primers added to 90 nM each. The primer sequences were TCg gCg CCC TTT gAT ATA CT and TAg Agg AAg TgT Tgg gCA gg (against the HVT012 gene of Meleagrid herpesvirus 1 (MeHV-1), Genbank AF291866), and TCCgTCgTgAATTTgTACgC and CgA CAA TTC CAC CTT Agg Cg (against the MDV011 gene of Gallid herpesvirus 2 (GaHV-2), Genbank DQ530348).
RNA-extraction was performed with the Innu PREP RNA Mini kit (Analytik Jena) and cDNA transcribed with the Cloned AMV First-Strand cDNA-Synthesis kit (Invitrogen) using random hexamers. PCR was performed with 2.5 µl of the cDNA in 25 µl reactions with 94°C for 180 s, followed by 35 cycles of 94°C for 30 s, 57°C for 30 s and 72°C for 60 s. Conventional PCR was performed with 2.5 µl sample in 25 µl reactions with the thermocycler programmed to 94°C for 180 seconds, followed by 30 cycles at 94°C for 30 s, 55°C for 30 s and 72°C for 60s. A fragment of IBDV genomic RNA segment B was amplified with primers gAC gAg TTC CTA gCC gAg Tg and CTg gCT gTg gCT AgA Agg AC. The primers bind to what appear to be conserved islands determined by alignment of GenBank sequences JQ411013 (isolate Hungary 903/78), GQ451331 (strain HLJ-0504), JX682710 (isolate QL), FJ695139 (Chinese strain YS07), and JX682712 (strain ZZ-11). H9N2 genome was quantified with AAg CCg AAA TCC Tgg gAA Tg and CTC CCT CTC AAA gTC gTA TC against segment 5 encoding NP (Genbank DQ067440), and infectious bronchitis virus (IBV) was detected with TgT TgT gCC AgT CCT CTC AT and gTT TCA CAg TAg CAg AgC gg against the ORF1a gene of strain H120 (Genbank FJ888351).
Lectin staining
Adherent cells were allowed to grow on microscope cover slips, suspension cells were collected with 200 × g for further processing. The cells were fixed with 1% formaldehyde in PBS and incubated with 30 µg/ml of biotinylated agglutinin of Sambucus nigra (Vector Laboratories, Burlingame, CA, USA, B-1305) to detect α 2,6 bonds, or of Maackia amurensis (B-1265) to detect α 2,3 bonds on the surface of the plasma membrane (the cells were not permeabilized). Binding was allowed for 45 min at ambient temperature and protected from light. The cells were washed once with PBS and the bound lectins were visualized with streptavidin conjugated to Texas Red (016-070-084) or fluorescein (DTAF, both from Jackson Immuno Research, #016-010-084). DAPI (4′,6-diamidino-2-phenylindol) in methanol was added to 1 µg/ml as a nuclear counterstain. The cells were washed once more with PBS prior to fluorescence microscopy.
Suspension cell cultivation
Suspension cultures were maintained in a shaking incubator (HT Multitron Cell, Infors AG, Bottmingen, Switzerland) on a rotating platform with amplitude of 5 cm and rotation speed of 180 min−1. CO2 atmosphere was set to 8% and temperature to 37°C. All culture vessels, shake tubes (Tubespin 50, TPP Techno Plastic Products AG, Switzerland) or baffeled shake flasks (Corning, NY, USA), were equipped with 0.2 µm filtered lids to allow gas exchange. Culture volumes were maintained at 20–50% of the vessel size. Trypsin (type IX-S, Sigma T0303, from a 1 mg/ml, 16 U/µl solution) was added for propagation of H9N2 to a concentration of 0.5 µg/ml at the time of infection and every 24 h thereafter. The culture tolerated 5 µg/ml of recombinant or porcine trypsin without apparent effects on viability (data not shown).
The DASBox (DASGip, Eppendorf, Hamburg, Germany) bioreactor units were equipped with a Marine impeller with three blades and 60–250 ml working-volume vessels. Gas mixing was performed with N2, air, CO2 and O2, pH was adjusted with CO2 and 1 M Na2CO3. Inoculation was usually performed to 1 × 106 cells/ml in CD-U3 medium and the culture was allowed to proliferate for 3 days to approximately 4 × 106 cells/ml. The parameters for the cell proliferation phase were 37°C culture temperature, 60% DO (dissolved oxygen) saturation in the medium, 180 rpm for the impeller, and a pH gradient that decreased from 7.25 to 7.00 units in the cell culture during cell proliferation. The pH was usually kept at 7.1 units during infection.
Variations included addition of one volume of virus production medium (100 ml, fed at a rate of 40 ml/h) 2 days after infection to cultures infected with EDSV, and 1/6th volume at the time of infection with NDV. Propagation of NDV virus was furthermore supported by feeding recombinant trypsin (rTrypsin, Novozym 6395020) into the infected culture from a solution kept at 4°C with an activity adjusted such that a feeding rate of 0.17 ml/h (4 ml per day) resulted in a final concentration of 8 U/ml of culture volume each day. Recombinant or porcine trypsin were equivalent in activity in shake tube experiments (data not shown). The incubation temperature was set to 35°C for propagation of NDV.
Results and discussion
Egg drop syndrome
Egg drop syndrome ’76 is caused by a member of the Atadenovirus genus in the Adenoviridae family (Adair et al., Citation1979), viruses with double-stranded genomic DNA enclosed by an icosahedral capsid without lipid membranes. Synonymous names for EDSV are duck adenovirus 1 (DAdV-1) and Duck atadenovirus A (the name proposed by the ICTV). The natural hosts appear to be ducks and geese and the virus has a world-wide distribution. Clinical disease in poultry often is subacute and only evident in a drop of egg production (hence, the “egg drop”) and disturbances in shell pigmentation and quality. Transmission of DAdV-1 occurs horizontally via orally ingested fomites and also vertically.
Adherent CR.pIX cells are permissive for DAdV-1 but overt CPE does not develop before 5 days post infection and sometimes is too weak for clear identification of infection ((a)). A subpassage of the infected cells usually led to more pronounced CPE and significantly elevated titres (data not shown). This observation suggests that DAdV-1 may require an active cell cycle and may have difficulties to spread in cultures that have settled into the plateau phase. Augmentation of virus replication by subcultivation may also indicate that mixing and reseeding of the cell monolayer disperses infectious units that are not easily shed into the supernatant.
Figure 1. Propagation of DAdV-1 in CR.pIX cell lines. (a) Infection of adherent cell monolayers is visible only by immunofluorescence staining 3 days PI but virus spreads efficiently until 5 days PI. (b) Efficient replication in suspension cultures requires addition of an aggregation-inducing medium 2 days PI. The reference is a culture without feed, SCS was given cell proliferation medium and AGG virus production medium 2 days PI. Experiment was performed in bioreactors with 110 ml culture volume. (c) Effect of MOI on infectious yields (left panel) and amplification of genomic DNA (right panel). The viral genome burst number was calculated from the ratios of viral genome to cellular E1A gene for each time point relative to the ratios at day 0. The numbers in the legend refer to cell density × 106 at the time of infection. Shown are the mean values of duplicates. (d) Propagation at 33°C does not release infectious units but appears to lead to a measurable amplification of antigen (HAU, right panel). Optimal temperature was determined to be 37°C. The experiment was performed in bioreactors with 100 ml culture volume. (e) The final process scaled to bioreactors with 100 ml culture volume. Comparison of yields of infectious units, HA activity and genome amplification in bioreactors and shake flasks. The reference shown was performed in shake flasks in triplicates with 50 ml culture volume each, error bars indicate standard deviation. CPE, cytopathic effect; PI, post infection; SCS, single-cell suspension; AGG, suspended aggregate; HAU, HA units.
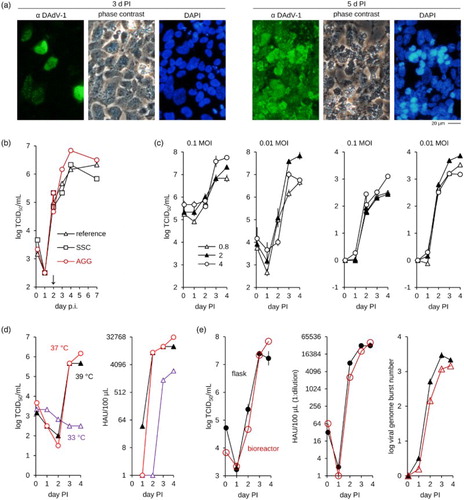
DAdV-1 replication in suspension cultures was investigated because such production processes can be scaled more easily to large volumes and are therefore preferred to adherent cultures. However, yields of DAdV-1 in single-cell suspensions were below those obtained in the adherent cultures. We have observed such a phenomenon previously during the development of a production process for highly attenuated poxviruses (Jordan et al., Citation2011). These viruses were difficult to produce in single-cell suspensions presumably because infectious units appear to be transmitted to a large extent by cell-to-cell contacts. An additional culture format was therefore developed, based on a second chemically defined medium (called the virus production medium) that was designed to induce tight cell aggregates at the time of infection (Jordan et al., Citation2011). Recombinant poxviruses that express GFP were shown to spread efficiently within these suspended aggregates. DAdV-1 production may also benefit from this culture format if spread of cell-associated infectious units contribute to yields. To test this model we compared yields after feed with the virus production medium at the time of infection, and two days or three days post infection. Cell proliferation medium (that maintains the single-cell culture format) was added at the same time points to parallel cultures as reference. Highest titres were obtained if virus production medium was added 2 days post infection (PI) ((b)), neither earlier nor later. We currently have no satisfactory explanation for this observation and speculate that inoculation and production phases may have different requirements for DAdV-1 in our cell and medium combination. Virus attachment to host cells may be more efficient in single-cell suspensions in the initial phase of production as few viruses (at low multiplicity of infection (MOI)) are inoculated into the culture. Subsequent virus generations are released to much higher MOI relative to the uninfected cells. In this later phase it may be beneficial to induce aggregates that include a single or very few infected cells and a majority of yet uninfected, potential host cells. If the ratio of infected (still viable) and uninfected cells is important for productive aggregate induction then such a scenario would also explain the importance of timing.
The next set of optimizations focused on MOI and cell density at the time of infection ((c)) and revealed that a MOI of 0.01 (as opposed to 0.1) at intermediate cell densities (2 × 106/ml) should be optimal for subsequent refinements. Because of the relatively slow replication kinetic we also used qPCR to follow the dynamic of genome replication in parallel to that of the infectious units. Using a calculation described previously for relative quantification of gene expression (Pfaffl, Citation2001) we confirmed a robust amplification of the viral genome over almost 4 orders of magnitude under the selected conditions ((c)).
Replication at different temperatures (33°C, 37°C and 39°C) was studied with the intention to further improve viral titres ((d)). DAdV-1 replicated to high titres at 37°C and 39°C (the core temperature of ducks is 41°C) but appeared not to be able to be propagated efficiently at 33°C. We also observed ((d)) a surprising discrepancy between infectious and HAU that suggests that a measurable amount of non-infectious particles with HA activity are formed at 33°C. Replication may be slightly faster at 39°C because HA activity indicative of particle formation is higher 24 h PI but this headstart appears not to be maintained at later time points. Stability of infectious units appears to be slightly lower at the higher temperature and therefore the optimal temperature for production of DAdV-1 was chosen as 37°C.
An overlay of the optimized process in flasks and a bioreactor ((e)) confirms scalability and good replication of DAdV-1 at MOI of 0.01 with 2 × 106 CR.pIX cells/ml, and at 37°C. Maximum yields were 6.8 × 107 TCID50/ml, > 1:215 HAU/100 µl and 3000-fold amplification of the viral genome (relative to 2 h PI).
Newcastle disease
NDV (also referred to as serotype 1 avian paramyxovirus, APMV-1) is a negative-stranded RNA virus of the Avulavirus genus in the Paramyxoviridae family. Infectious activity requires processing of the F0 precursor protein in the viral envelope by host proteases. Specificity of the protease is strongly influenced by the number of basic amino acids at the cleavage site and contributes to the pathotype of the strains (Collins et al., Citation1993). Avirulent and lentogenic strains carry monobasic cleavage sites that are processed by trypsin-like proteases. Mesogenic and velogenic strains express polybasic cleavage sites that are accessable to a greater diversity of proteases, including, in particular, subtilisin-like proteases. This property broadens tissue tropism and allows for systemic virus spread inducing greater damage to organs and tissues (Nagai et al., Citation1979). Velogenic strains cause a highly contagious and notifiable disease that is associated with a significant economic burden (OIE, Citation2013a). NDV has a very broad host range among avian species but expresses only marginal zoonotic potential. The rare infections in humans even by velogenic strains are usually restricted to a mild and transient conjunctivitis or laryngitis (Boseret et al., Citation2013).
The vaccines against ND consist of live, vectored live and inactivated preparations of strains with low virulence (OIE, Citation2013a). Live or vectored live vaccines are often preferred because they can be applied directly in the hatchery using automated vaccination equipment. NDV has also the highly promising capacity to replicate in human tumours and is being tested to augment oncolytic therapies against neoplastic diseases (Zamarin & Palese, Citation2012). However, because this inherent oncolytic activity correlates with virulence in birds, research on and application of NDV-based virotherapy is being legally restricted by 9 CFR Part 121 in the USA (Zamarin & Palese, Citation2012; OIE, Citation2013a).
Most NDV vaccines are being produced in embryonated eggs that naturally contain the proteases required for activation of lentogenic viruses (Muramatsu & Homma, Citation1980; Rott et al., Citation1980; Zhirnov et al., Citation1985). As expected, exogenous trypsin had to be added to CR.pIX cultures to enable secondary replication cycles of the lentogenic NDV strain LaSota ((a)). However, infection of CR.pIX suspension cultures initially yielded NDV at the lower range of titres reported for other continuous cell lines even in the presence of trypsin. Although titres are difficult to compare because infectious units are given in different units it appears that volumetric yields are generally higher in eggs than in cell cultures (>109 EID50/ml in eggs (Cowen & Braune, Citation1988; Li et al., Citation2012), 108.5 PFU/ml in LSCC-H32 and secondary chicken embryo cells (Roth & Kaaden, Citation1985), 106.1 to 107 TCID50/ml in QT35 (Cowen & Braune, Citation1988) and DF-1 cells (Li et al., Citation2012), respectively).
Figure 2. Production of NDV in CR.pIX cultures. (a) Lentogenic NDV enters CR.pIX cells but cannot spread without an exogenous supply with protease. (b) Adaptation of NDV by passaging on CR suspension cells in 10 ml cultures. Note increase of infectious units between passage 4 and 5. Passaging was performed without intermediate titrations. The average MOI determined retrospectively was 0.1. (c) Differences in the kinetic of replication of the isolates at different passage levels, P4 and P9. Infection was performed with MOI of 10−4 in parallel bioreactors with a culture volume of 140 ml. (d) Influence of MOI on replication and yields, determined in shake flasks with 30 ml culture volumes. (e) Suspension cultures in a bioreactor infected with NDV. Addition of virus production medium CD-VP4 to one-sixth of the culture volume induces small aggregates. CPE is evident 24 h PI. (f) Sonication further increases HA yields. Experiment was performed in bioreactors with 110 ml culture volume in triplicates. PI, post infection.
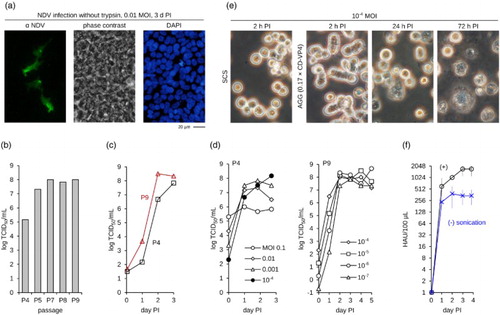
Trypsin is not expected to be inhibited because the chemically defined cell proliferation medium for CR and CR.pIX cells has a very low protein content (10 ng/ml of recombinant IGF) and no hydrolysates or other substances are present that may inhibit proteases. We therefore performed passaging of NDV in CR cell suspensions to investigate whether low titres in cell cultures are due to adaptation of our strain of NDV to eggs. A 1000-fold increase in infectious titres was indeed observed between passages 4 and 7 and appeared to remain stable for at least two additional passages ((b)). A direct comparison of virus isolates at passage levels 4 and 9 indicated that replication kinetics has also increased ((c)). We observed no decrease in titres even at 9 × 106 cells/ml indicating that NDV production may be possible also at very high densities (there is no cell density effect, (Lohr et al., Citation2012) and references therein) although this may not further increase absolute yields. Further optimization resulted in a process with incubation temperature of 33–35°C, pH of 7.1 units, and cell density of 2 × 106/ml. The optimal trypsin concentration is 0.5 µg/ml trypsin type IX-S (or 8 U/ml of recombinant trypsin) and should be maintained by daily or continuous supplementation.
A very low (rather than higher) MOI appears to be the most critical parameter for production of the NDV at passage level 4 ((d)). Titres remained above 108 TCID50/ml for virus at passage level 9 at MOI from 10−4 down to 10−7. Infectious units with virus at passage level 9 in the improved process are in the upper range of those reported for continuous cell lines and replication at lowest MOI spans almost nine orders of magnitude. However, haemagglutinating (HA) activity was still low with maximum values usually in the range of 1:265–1:512/100 µl. To further improve yields we paid more attention to the clumping of the cells that sometimes occurred 24 h post infection. This phenomenon may reflect formation of syncytia and provides an additional mechanism for spread of infectious units (Ebert et al., Citation2004). We therefore added virus production medium to further augment the aggregate formation (Jordan et al., Citation2011), but we also sonicated the final lysate to separate clusters of cell debris and viruses, speculating that the true HA titres are decreased proportionally to the number of particles bound within the aggregates. These additional steps are compatible with scalable processes in bioreactors ((e) and (Jordan et al., Citation2011)) and did improve HA yields fourfold ((f)). The final process consists of supplementation with one-sixth of the culture volume with virus production medium and sonication of the complete lysate to obtain maximum HA levels of 1:1024–1:2048 at day 3.
Avian infectious bronchitis
Avian IBV belongs to the Coronaviridae, and therein to the Gammacoronavirus genus in the Coronavirinae subfamily. Gammacoronaviruses have been described to infect a variety of avian species worldwide (Chen et al., Citation2013) but have not been implicated in any human infections (OIE, Citation2013b). IBV is disseminated by aerosol and fomites and mainly causes damage in the respiratory and the uro-genital tract (Cavanagh, Citation2007). Overt disease has been observed only in galliforms (Beaudette, Citation1950; OIE, Citation2013b) but waterfowl appear to be susceptible and may serve as asymptomatic reservoir for IBV if chickens and ducks are farmed together (Liu et al., Citation2005). Vaccinations regimes typically include live attenuated and killed vaccines but infectious bronchitis appears to be a disease that is not easily controlled. The attenuated strains are generated by serial passages in eggs, and the final passage level of a vaccine virus is selected by balancing the safety and efficacy of the vaccine candidate virus (OIE, Citation2013b).
Inoculation of CR or CR.pIX cell monolayers with IBV strains M41, H120, and 11518/2010 (a strain originating from a 13-day-old broiler with severe lympho-histiocytic interstitial nephritis) did not result in any signs of CPE (data not shown). IBV is known to replicate in embryonated eggs but not in continuous cell lines (Zhang et al., Citation2012 and references therein). The attenuated strain Beaudette, that was obtained by serial passaging in embryonated chicken eggs (Beaudette & Hudson, Citation1937), is an exception with a broad cell tropism but reduced value as a vaccine strain.
Coronaviruses are a family of large enveloped viruses with prominent surface (S) proteins or spikes. One barrier for permissivity maps to the S protein of IBV that is processed by cellular proteases into two subunits, S1 and S2 (Chen et al., Citation2007; Madu et al., Citation2007; Belouzard et al., Citation2012). The S1 subunit mediates attachment to the target cell (Casais et al., Citation2003) and appears to contain the dominant positions for neutralizing epitopes and escape mutants (Cavanagh, Citation2003; De Wit et al., Citation2011). The S2 subunit cooperates with the cognate S1 in receptor recognition and is responsible for fusion of viral and plasma membranes (Cavanagh, Citation2007; Promkuntod et al., Citation2013). The receptors of IBV are not yet known but glycosylated structures, especially α-2,3-linked sialic acids, appear to be required for infection (Cavanagh, Citation2007; Belouzard et al., Citation2012). Both, α-2,3 and α-2,6 configurations are available on CR and CR.pIX cells ((a)).
We attempted adaptation of the M41 and H120 strains to our culture system. Adaptation, however, requires at least low-level replication of the viruses so that suitable mutations can accumulate. Speculating that entry of viruses into the cells is the main block we also tested different mammalian cell lines (hoping for pseudoaffinity Zhang et al., Citation2012) and added polymers (such as polybrene, poly-L-lysine and polyethylenimine) known to augment interaction of viruses (or lipid vesicles) with plasma membranes (Davis et al., Citation2004) ((a)). Potential infection was assayed by RT-PCR with primers against the ORF1a polyprotein.
Figure 3. No infection with IBV strains M41 and H120. (a) Adherent cultures of BHK (from the Syrian hamster), R05T (from the Egyptian fruit bat Jordan et al., Citation2009a) and CR.pIX cells were inoculated with IBV at MOI of 1 also in presence of charged polymers with the intention to improve infection (Davis et al., Citation2004). Potential entry of virus was analysed by RT-PCR. A comparison of infection of adherent (ADH) and single-cell suspension (SCS) CR.pIX cultures initially suggested that suspension cultures may be susceptible. (b) Strong signal in the suspension culture for 6 days but reduced signal of the first passage (performed with the culture 3 days post inoculation). (c) Complete loss of signal in inoculations with lysate from the previously inoculated CR.pIX culture. Passage 1 culture shown in (b) was lysed and used to inoculate fresh CR.pIX cells. No signal indicative of replication-competent virus could be detected suggesting that all earlier signals were caused by the virus from the initial inoculation.
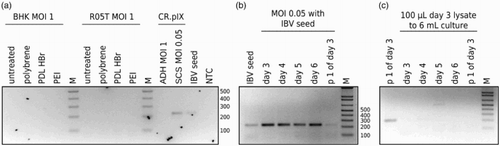
We detected a signal for genomic RNA in infected suspension cultures. This signal was maintained for one week, could be rescued into one subpassage, but was lost with the second subpassage ((b) and 3(c)). In adherent cultures we did not observe any signals indicative of entry of any viruses. One important difference in the handling of adherent and suspension cultures was that adherent cultures were washed 2 h after inoculation with seed virus. Although interference by bovine serum present in the medium for adherent cultures is a formal possibility, we consider that detection of the input virus is the most parsimonious explanation for transient detection of viral genome and consistent with similar observations and reports on virus stability in the literature (Beaudette, Citation1950; Coria & Peterson, Citation1971).
In conclusion, CR and CR.pIX cell lines appear not to be permissive for, and probably not even susceptible to, infection with the tested IBV strains. An important limitation of our study is that we had no susceptible primary cultures or cell-adapted IBV strains to test sensitivity of our assays or activity of the vaccine samples. Propagation of virus at low levels in rare niduses may have escaped undetected.
Adaptation may be successful starting with a strain such as Beaudette. However, this may not necessarily lead to a vaccine because cross-protection of strains that have been extensively propagated ex vivo appears to be reduced (OIE, Citation2013b). S1-vectored vaccines (Cavanagh, Citation2007) with viral carriers that can be propagated in the Muscovy duck cell lines (Jordan et al., Citation2011) may yield alternative control mechanisms against infectious bronchitis.
Marek's disease
MD is a neoplastic disease caused by some herpesviruses of the Mardivirus genus. The type species is referred to as GaHV-2 or Marek's disease virus (MDV). Other species in this genus include Gallid herpersvirus 3 (GaHV-3) and MeHV-1, also referred to as HVT. Infections with MDV lead to substantial economic losses each year, while GaHV-3 and HVT are non-pathogenic. MDV replicates in the skin and feather follicles and is disseminated horizontally via inhalation of fomites. The fulminant disease is caused by a subset of infected T cells that are transformed into lymphomas surprisingly fast, usually within 3–4 weeks. Infiltration of neurons can cause progressive paralysis of legs and wings whereas diffuse lymphomatosis of visceral organs often presents as an acute disease associated with high mortality (OIE, Citation2010; Couteaudier & Denesvre, Citation2014).
Vaccines against MD are based on live-attenuated strains of GaHV-2 (e.g. Rispens strain), non-pathogenic GaHV-3, and more importantly on non-pathogenic HVT. HVT is also widely used as vector virus, and with appropriate insert genes HVT represents the new generation of poultry vaccines being able to provide good protection against several important avian diseases such as ND, avian influenza, infectious bronchitis or infectious laryngotracheitis. Protection against MD appears to require that vaccine viruses establish persistent latent infections (Baigent et al., Citation2005). However, immunity is not sterile so that coinfection and reinfection with vaccine and virulent strains can occur with the effect that properties of field viruses appear to shift under selective pressures (Nair, Citation2005). Effective control of MD is also confounded by the fact that the genetic background of the flocks can also influence susceptibility for MDV (Witter et al., Citation2005) and because maternal antibodies appear to confer only a limited protection against mardivirus infection (Müller et al., Citation2012).
The vaccines are usually supplied as viable cells in liquid nitrogen that have to be revitalized prior to application because mardiviruses remain cell-associated throughout the replication cycle. Only some HVT preparations are available in a lyophilized (cell-free) formulation. Production is usually performed in CEFs and occasionally in duck embryo fibroblasts (OIE, Citation2010). Continuous cell lines reported to be permissive for mardiviruses include the DF-1 chicken cell line (Geerligs et al., Citation2008) and the QM7 quail muscle cell line (Schumacher et al., Citation2001). The QT-35 quail fibrosarcoma cell line is also permissive at least for HVT but, surprisingly, appears to harbour also a previously uncharacterized latent virus possibly related to GaHV-2 (Yamaguchi et al., Citation2000). Another cell line reported to be permissive is the chronically infected lymphoblastoid cell JM-1 that was derived from chickens infected with MDV and that can transmit this virus to CEFs in cocultivation experiments (Munch et al., Citation1978).
However, there are also unpredictable restrictions in the permissivity for mardiviruses in vitro. For example, MDV was shown not to replicate in the chicken cell line LSCC-H32 that is permissive for a wide spectrum of other viruses (Roth & Kaaden, Citation1985), and only a small fraction of cells, 5%, were positive for viral antigens in the chronically infected JM-1 cultures (Munch et al., Citation1978). Gene expression of latent MDV-like virus in QT-35 cells could be reactivated by superinfection with HVT but recovery of infectious units was not possible by cocultivation with CEFs or primary chicken kidney cultures (Yamaguchi et al., Citation2000). Isolation was only successful in vivo by inoculation of QT-35 cells into chicken embryos (Yamaguchi et al., Citation2000).
We infected adherent CR and CR.pIX cultures with different mardiviruses, including HVT, a vector HVT that expresses an antigen to protect against NDV (Palya et al., Citation2012) (Vectormune ND, Ceva Animal Health), and Rispens. The results shown in (a) and 4(b) were obtained by inoculation with revitalized CEF cells carrying Vectormune ND where MOI indicates ratio of infected fibroblasts to CR or CR.pIX. Propagation of HVT was tracked by qPCR against the viral genome and the cellular DNA.
Figure 4. Mardivirus propagation in adherent CR and CR.pIX cells. (a) CR and CR.pIX cells were inoculated with the freshly revitalized Vectormune ND preparation at the indicated ratios (e.g. MOI of 0.1 corresponds to 1 CEF of the vaccine for every 10 cells of the anatine continuous cell line). The cultures were subpassaged twice per week by fivefold dilution. DNA was isolated at each passage and qPCR was performed with primers against mardiviruses and against the E1A gene of the continuous cell line to normalize results to the number of host cells. CR cells are fully permissive for mardiviruses but several attempts failed to establish a productive infection in CR.pIX cells. (b) Immunofluorescence staining against HVT parent virus of Vectormune ND and the cellular E1A gene demonstrates extensive spread of HVT in a culture that has been subpassaged nine times. (c, d) Chronic infections could be maintained for 30 passages. CPE is characterized by extensive cell damage and large syncytia. Results in (a), (b) and (c, d) are from independent repeat experiments. (e, f) Both, CR and CR.pIX cells were fully permissive for the Rispens strain (GaHV-2). This virus induced strong CPE also in CR.pIX cells but replicated to lower levels. The dashed lines between passage 2 and 3 (CR only) and 5 and 6 (both cell lines) indicate addition of one-tenth of uninfected cells because cytopathic effect appeared to be too strong to continue subpassaging. The genome equivalents of Rispens were also determined with primers against pIX in the CR.pIX cultures; as expected, pIX could not be detected in CR cultures and the corresponding genome equivalents are therefore not shown.
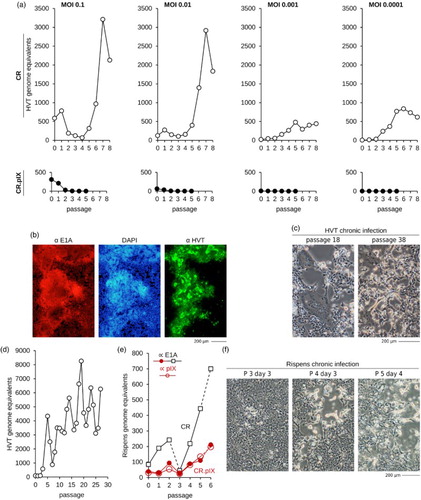
CR cells were fully permissive for HVT ((a)–(d)) and Rispens (((e) and 4(f)) and infection led to a progressively expanding CPE that was characterized by frequent plaque formation (centrally dislodged cell layer framed by highly refractile cells) or large syncytia. Appearance of CPE was delayed and was often negligible in the first three passages. Chronic infections were readily established and one culture was HVT-positive for at least 30 passages until CPE developed to an extent that required addition of uninfected cells prior subpassage ((c) and 4(d)). Because of the delayed CPEs we did not titrate virus by TCID50 protocols but quantified virus propagation via qPCR. The qPCR cycle threshold levels for the cellular E1A gene in the full lysates were used to normalize the number of viral genomes to the number of cells in the culture. With this quantitative measurement, that should be independent of cell numbers, we observed what appears to be a periodicity in the kinetic of virus propagation. The maximum yields and kinetic we observed for MDV and Rispens in adherent CR cultures compared well with previous results in chickens that measured a maximum of 108 HVT genomes per 106 feather follicle cells (Baigent et al., Citation2005).
CR.pIX cells were not permissive for HVT but allowed productive propagation of Rispens with strong cytopathic effect yet lower titres compared to CR cells ((a) and 4(e)). Especially the block in HVT replication is very surprising because CR.pIX and CR cells differ only by the expression of the pIX protein (Jordan et al., Citation2009a), a minor structural and presumably also regulatory factor of human adenoviruses (Parks, Citation2005; Vellinga et al., Citation2005). We have proposed previously that pIX may also augment binding of Hsp70 and Hsp90 in the avian cell line (Jordan et al., Citation2009b). The resulting activation of the heat shock response can be beneficial for replication of some viruses (Glotzer et al., Citation2000; Hung et al., Citation2002) but may also elevate antiviral responses as signalling cascades of the two pathways communicate (Melville et al., Citation1999; Salminen et al., Citation2008). Mardiviruses have also been reported to interact with Hsp70 (Zhao et al., Citation2009; Wang, Citation2011), and may depend on this factor for entry into chicken embryo fibroblasts (Wang, Citation2011). By directing Hsp70 into complexes with Hsp90, pIX may interfere with availability of Hsp70 at the cell surface in a way that can be partially compensated by Rispens but not by HVT. Alternatively, pIX may indirectly cause an elevated state of innate immunity. HVT does not code for several virulence factors that are present in Rispens (Tulman et al., Citation2000; Afonso et al., Citation2001) and may therefore be unable to block the cellular defences.
Avian influenza
Avian influenza viruses (AIVs, Influenzavirus A genus, Orthomyxoviridae family) have the capacity to cause serious outbreaks in poultry farms. They are also known for a high zoonotic and dangerous pandemic potential (Li et al., Citation2008; Richard et al., Citation2014). Migratory waterfowl maintain a global subclinical reservoir that allows perpetuation of all sixteen haemagglutinin (HA, designated as H1–H16) and nine neuraminidase (NA, N1–N9) subtypes of AIVs that are currently known to infect birds (Sonnberg et al., Citation2013). This reservoir, together with trade and exchange of poultry products, is a source for repeated infection of livestock (Kim et al., Citation2009).
Tropism and host range are mainly determined by the HA and NA proteins in the viral envelope and by PB2, a subunit of the viral RNA polymerase (Tscherne & García-Sastre, Citation2011). The majority of strains that are circulating in wild and domestic birds are of low pathogenicity AIV (LPAIVs) (Sonnberg et al., Citation2013). However, strains derived from H5 and H7 viruses carry an increased risk of naturally acquiring mutations that cause a transition from low to high pathogenicity AIV (HPAIV) (Veits et al., Citation2012). Morbidity and mortality in humans is influenced by tropism (viruses that infect the lower respiratory tract tend to cause more severe disease than those that replicate in the upper regions), interaction with innate immunity (the case fatality rate is higher for strains that induce hypercytokinemia or “cytokine storms”), and antigenic drift (pandemics are more severe if strains have escaped from population immunity by antigenic drift) (Kobasa et al., Citation2007; Gambotto et al., Citation2008; Tscherne & García-Sastre, Citation2011).
Infection with AIVs of HPAIVs and H5 or H7 LPAIVs are notifiable worldwide (OIE, Citation2014). However, non-H5, non-H7 LPAIVs still can be epidemiologically and economically important for poultry rearing. For example, viruses of strain H9N2, that are investigated in greater detail below, are common in most parts of Asia and the Middle East. They contribute significantly to disease in gallinaceous poultry and may lead to marked mortality rates in concert with secondary bacterial infection or adverse environmental conditions such as high ambient temperatures (Bano et al., Citation2003). H9N2 viruses in China have also served as donors of gene segments in the creation of reassortant influenza viruses such as H7N9 and H10N8 that revealed increased zoonotic potential (Wan & Perez, Citation2007; Wan et al., Citation2008; Monne et al., Citation2013; Sun & Liu, Citation2015).
AIV infections can cause substantial economic disruptions in poultry production and trading. Control is achieved by a combination of monitoring and outbreak containment strategies that include restriction in poultry movements, culling of infected birds, and vaccination (Capua & Marangon, Citation2006; OIE, Citation2014). Vaccination of birds against notifiable AIV infections is legally restricted in many countries. If vaccination is performed, the preferred preparations contain inactivated (not live attenuated) viruses using LPAIVs (not HPAIVs) as production seed (OIE, Citation2014). More recently, vectored vaccines such as fowlpox, Newcastle or HVT viruses expressing the HA and NA gene of specific AIV subtypes are being deployed (Kapczynski et al., Citation2015). Such vaccines are manufactured based on embryonated chicken eggs or in CEFs. A wide variety of strains have been used for vaccine purposes depending on the subtype causing acute problems in various parts of Asia, North America, and North Africa (OIE, Citation2014). Problems that are sometimes associated with the current production system are low yields for certain strains calling for expensive concentration steps to increase potency, considerable amounts of egg shells and embryo remnants that remain as biohazardous solid waste, and dependence on a continuous supply of embryonated eggs free of adventitious agents (Ackland et al., Citation1985; Enserink, Citation2004; OIE, Citation2014). Improved control over supply of embryonated eggs is achieved by constructing breeding facilities immediately adjacent to the production site, but this comes at substantial costs (Hoa et al., Citation2011). We have therefore tested replication of H9N2 as a highly representative LPAIV subtype in suspension cultures of CR.pIX cells to provide an additional production substrate that is independent of primary material ().
Figure 5. Replication of LPAIV H9N2 isolates in CR.pIX cultures. (a) Both sialic acid receptor configurations for influenza A viruses are uniformly available on CR.pIX suspension cells. Lectins of S. nigra and M. amurensis have different affinities for the terminal α-glycosidic sialic acid configurations and were used to stain the membranes of the indicated cell lines. The initial pool of CR cells appeared not to be uniformly positive for the 2,6 configuration. (b) Very fast replication of the two H9N2 isolates in the CR.pIX suspension cells to high titres in presence of low and high amounts of trypsin (shake flasks, 50 ml culture volumes, MOI of 0.001) The dashed line indicates level of input virus, infectious units during the eclipse in 2 h PI samples were below limit of detection. (c) Infectious and HA activity of passage 10 of the two isolates as function of MOI. Error bars indicate standard deviation of four values of out of two independent experiments in shake tubes at 15 ml culture volumes. ND, not done; values for MOI of 10−9 and 10−10 were below limit of detection.
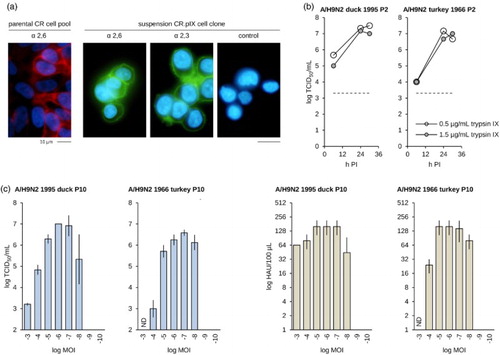
Because the host spectrum of AIVs is partially determined by preference for 2,3 or 2,6 α-glycosidic linkage at the terminal sialic acid (Ito et al., Citation1998) we first characterized surface glycosylation of the CR and CR.pIX cell lines using lectins of S. nigra and M. amurensis. Avian isolates tend to recognize 2,3 α-glycosidic bonds that predominate in the gastrointestinal and respiratory tract of ducks and chickens whereas human isolates tend to recognize the 2,6 α-configuration that predominates in the human upper respiratory tract (Shinya et al., Citation2006; Gambotto et al., Citation2008). Whereas early passage cell pools are not uniformly positive for the 2,6 α-configuration (some cells do not react with S. nigra lectin) we observe both configurations in the final CR.pIX cell clone ((a)). This result suggests that AIV isolates should be able to replicate in CR.pIX cultures independent of a mammalian or avian origin, a property that has also been described for the Madin-Darby canine kidney (MDCK) cell line that is considered a gold standard in influenza virus research (Hatakeyama et al., Citation2005; Donis et al., Citation2014).
Orthomyxo viruses require proteolytic activation of the viral receptor, the HA protein, very similar to the paramyxoviruses (Muramatsu & Homma, Citation1980; Rott et al., Citation1980; Zhirnov et al., Citation1985). The proteases contained in embryonated eggs are replaced by exogenous trypsin in cell cultures. We observed good replication of the two H9N2 isolates in the presence of 0.5 and 1.5 µg/ml of trypsin, well below the 5 µg/ml as maximum amount of trypsin that is tolerated by CR.pIX ((a)). Replication of both isolates (duck/1995 and turkey/1966) at passage level 2 appeared to be very fast so that maximum titres in the range of 5 × 107 TCID50/ml and 1:256 HAU/100 µl were obtained within 24 h of infection. However, although both isolates are H9N2 strains they differed in maximum yields, a property that was maintained also after 10 passages in the CR.pIX cells ((c)). Permissivity of CR.pIX cells appears to be very high as both isolates established productive infections to maximum titres at MOI as low as 10−7 ((c)).
AIVs isolated with the help of cell lines have been proposed to be suitable as vaccine seeds (Donis et al., Citation2014). The HA and infectious activities of the LPAIV propagated in CR.pIX cells in true suspension cultures in chemically defined medium without microcarriers and at extremely low MOI compare favourably to the results reported with H9N2 in MDCK cells (Donis et al., Citation2014; Ren et al., Citation2015).
Infectious bursal disease
IBDV is a member of the Birnaviridae, genus Avibirnavirus. The disease caused by IBDV, IBD or Gumboro disease, is a serious economic burden to the poultry industry (Berg, Citation2000). Only chickens appear to be susceptible to overt disease; turkeys, ducks, and other poultry appear not to be affected by IBDV (OIE, Citation2008). Infected chickens develop an acute depression of the humoral immune response due to extensive lesions in the bursa of Fabricius. Such birds are more susceptible to secondary infections and fail to respond adequately to routine vaccination (Müller et al., Citation2012).
The viral capsids are icosahedral with diameters of 60–70 nm. They are not enveloped and contain two segments of double-stranded RNA of 2.8 and 3.4 kbp, respectively, coding for five proteins. The viral polymerase (VP1) and a nonstructural protein proposed to contribute to dissemination of the virus (VP5) are expressed without further processing. The two structural proteins (VP2 and VP3) are released by the viral protease (VP4) contained in the autocatalytically active tripartite polyprotein. The infectious units are very stable in the environment (Müller et al., Citation2012). Control of IBDV is furthermore complicated by the evolution of the pathogen towards greater antigenic diversity and increased virulence (OIE, Citation2008; Müller et al., Citation2012). The preferred vaccine is live IBDV that has to be monitored for a correct level of attenuation: viral replication should be high enough to induce protective immunity also in the presence of maternal antibodies and against the emerging variant field strains but below levels that may lead to immunosuppression. The challenges are being addressed by immune-complex vaccines, HVT-vectored VP2, and by prime/boost regimes using live and killed vaccines for high value birds such as breeder hens.
IBDV (strain B87) replicated rapidly in adherent monolayers of CR.pIX cells ((a)). We observed prominent comet formation indicative of efficient release of viruses 24 h PI followed by widely scattered and overt CPE 48 h PI. The results in adherent cultures are confirmed by the high yields of infectious units in various suspension culture formats ((b)): IBDV replicated in single-cell suspensions as efficiently as in cultures with induced aggregates that were designed to support propagation of cell-associated viruses (Jordan et al., Citation2011). Titres were also not increased by lysis of the cells in either of the culturing system suggesting that none or negligible infectious units remain within the host cell at the end of the replication cycle.
Figure 6. Replication of IBDV in CR.pIX cells. (a) Immunofluorescence staining of infected adherent cell monolayers. IBDV replicates fast and causes formation of large comets. (b) Similar yields independent of culture format or harvest suggests robust replication. Experiment was performed in suspension cells at 2 × 106 cells/ml in 8 ml culture volume and MOI of 0.05. SN, supernatant; SCS, single-cell suspension; AGG, suspended aggregates. (c) Replication kinetic depending on MOI (left panel, performed in duplicates, 2 × 106 cells/ml in 8 ml culture volume) and cell density at infection (right panel, shown are values of two independent experiments, each in duplicates; MOI 0.05 in 8 ml culture volume).
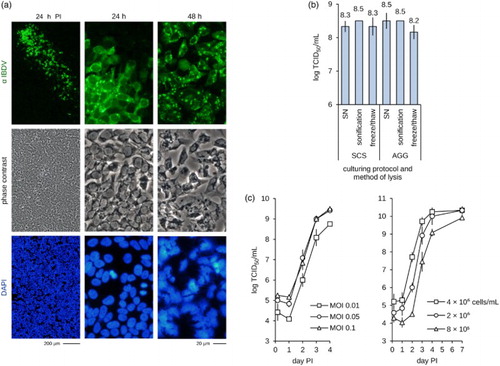
Propagation of IBDV in single-cell suspension cultures was robust with high titres at MOI of 0.1 or 0.05 (lower MOI did not increase yields) and at intermediate to high cell densities (2–4 × 106 CR.pIX cells/ml). The observed maximum yield was 3 × 1010 TCID50/ml at day four post infection.
Derzy's disease
Derzsy's disease appears to affect only geese and Muscovy ducks, and has been described in Europe, Asia, and North America (Tatár-Kis et al., Citation2004 and references therein). It is a highly contagious and economically damaging disease caused by strains of GPV and Muscovy duck parvovirus (MDPV), species in the Dependovirus genus in the Parvoviridae family. The small non-enveloped viruses are shed in large amounts by acutely infected birds and remain stable against environmental and thermal stress (Schettler, Citation1973). Disease can be subclinical in adult birds but is usually fatal in birds in the first month after hatching. The preferred vaccines are based on live-attenuated viruses produced in primary duck embryo cells, and recently, a transformed (tumorigenic in nude mice) cell line (Kisary et al., Citation1978; EMA, Citation2014; Maurin-Bernaud et al., Citation2014).
We have previously described replication of a GPV (Mészáros et al., Citation2014) and here briefly confirm this result with an extension to successful propagation of MDPV (). Although they are closely related, the two viruses had profoundly different properties. GPV was propagated easily and reached a maximum titre of 107.5 TCID50/ml within one passage whereas MDPV required three subcultivations of the infected, but apparently healthy cells until CPE was visible and maximum titres of 106.1 TCID50 were obtained ((b)). Another difference is that yields are higher if infection with GPV was performed with a low MOI whereas titres of MDPV benefited from infection with a greater MOI. Although antigen yields are difficult to compare between the two viruses because different antisera with different binding properties have to be used in quantification by ELISA, increased infectious titres appear to correlate with increased antigen yields for GPV and MDPV ((a) and 7(b)).
Figure 7. Propagation of goose (GPV) and MDPV in adherent CR.pIX cultures. (a) Yields of parvovirus antigens after infection with MOI of 0.1 or 0.01. (b) Infectious titres of the parvoviruses in CR.pIX cells. GPV replicates to expected titres within the first passage, MDPV replicates to high titres after three passages of apparently healthy cell monolayers. All values shown were obtained from complete cryogenic lysates 3 days post infection.
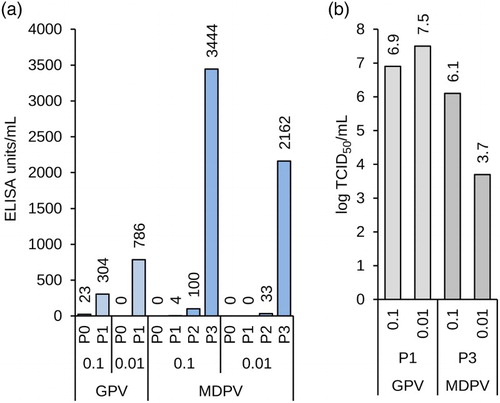
In summary, the yields for both GPV and MDPV compare favourably to a vaccine dose of 2.6–4.8 log10 TCID50. The level of antigen as measured by ELISA is furthermore similar to the level of antigen obtained after infection of duck eggs. CR.pIX cells may therefore be able to replace the currently predominating primary cells as host substrate also for the production of vaccines based on avian parvoviruses.
Conclusions
A delicate balance between costs, economic benefits, and potential to reduce zoonotic threats guides the decision on whether a given vaccine is or is not to be used for agricultural birds with a short life span. One complication introduced into these considerations for poultry vaccines is that industrial farmers and those with few resources both require a diversity of vaccines, but that robust and cost-effective production appears to be skewed primarily towards industrial use.
Most poultry vaccines are manufactured with material derived from embryonated chicken and duck eggs of a high quality. Dependence on embryonated eggs introduces costs and sometimes fragile logistics into vaccine production processes. Such issues are avoided if a continuous cell line permissive for a broad selection of vaccine strains and suitable for vaccine production would be available. CR and CR.pIX are well-characterized continuous cell lines for research and for manufacturing of vaccines (Jordan et al., Citation2013). They are available as adherent cultures and in suspension format in chemically defined media without dependence on bovine sera or other animal-derived components. There have also been no indications of any adventitious agents in exhaustive series of specific and general diagnostic assays. We now extend earlier studies on permissivity for various pathogens to include poultry vaccine candidates against EDS, MD, ND, infectious bronchitis, avian influenza, IBD, and Derzsy's disease. Some of the viruses required unique adaptations to the production process but all, with exception of the tested isolates of IBV, could be propagated to high titres in the anatine continuous cell lines.
Additional studies are required for the development of novel poultry vaccine production processes based on the CR and CR.pIX cell lines. One topic is scale-up to larger bioreactors or transfer to different bioreactor types such as cultivation at high cell-density with help of perfusion. A change of the production substrate may also affect level of attenuation of live vaccines and degree of protection conferred by antigens in killed vaccines (World Health Organization, Citation1998). Another important aspect that requires investigation is therefore characterization in vivo of safety and immunogenicity of poultry vaccines derived from the anatine cell lines.
Conflicts of interest
Authors IJ, KJ, KH, VL, ZP, EG-S, YF, PG, and VS are employed by companies involved in vaccine production.
Acknowledgements
The authors wish to thank Banghui Zhang for support and critical discussions.
ORCID
Ingo Jordan http://orcid.org/0000-0003-2711-7252
References
- Ackland, N.R., Tannock, G.A. & Young, I.F. (1985). A device for the nondestructive decontamination of large volumes of infected egg waste. Applied and Environmental Microbiology, 49, 920–924.
- Adair, B.M., McFerran, J.B., Connor, T.J., McNulty, M.S. & McKillop, E.R. (1979). Biological and physical properties of a virus (strain 127) associated with the egg drop syndrome 1976. Avian Pathology, 8, 249–264. doi: 10.1080/03079457908418350
- Afonso, C.L., Tulman, E.R., Lu, Z., Zsak, L., Rock, D.L. & Kutish, G.F. (2001). The genome of turkey herpesvirus. Journal of Virology, 75, 971–978. doi: 10.1128/JVI.75.2.971-978.2001
- Andre, F.E., Booy, R., Bock, H.L., Clemens, J., Datta, S.K., John, T.J., Lee, B.W., Lolekha, S., Peltola, H., Ruff, T.A., Santosham, M. & Schmitt, H.J. (2008). Vaccination greatly reduces disease, disability, death and inequity worldwide. Bulletin of the World Health Organization, 86, 140–146. doi: 10.2471/BLT.07.040089
- Baigent, S.J., Smith, L.P., Currie, R.J.W. & Nair, V.K. (2005). Replication kinetics of Marek's disease vaccine virus in feathers and lymphoid tissues using PCR and virus isolation. Journal of General Virology, 86, 2989–2998. doi: 10.1099/vir.0.81299-0
- Bano, S., Naeem, K. & Malik, S.A. (2003). Evaluation of pathogenic potential of avian influenza virus serotype H9N2 in chickens. Avian Diseases, 47, 817–822. doi: 10.1637/0005-2086-47.s3.817
- Beaudette, F.R. (1950). Infectious bronchitis (differential characteristics from Newcastle disease). Canadian Journal of Comparative Medicine and Veterinary Science, 14, 24–27.
- Beaudette, F.R. & Hudson, C.R. (1937). Cultivation of the virus of infectious bronchitis. Journal of the American Veterinary Medical Association, 90, 51–60.
- Belouzard, S., Millet, J.K., Licitra, B.N. & Whittaker, G.R. (2012). Mechanisms of coronavirus cell entry mediated by the viral spike protein. Viruses, 4, 1011–1033. doi: 10.3390/v4061011
- van den Berg, T. (2009). The role of the legal and illegal trade of live birds and avian products in the spread of avian influenza. Revue Scientifique et Technique (International Office of Epizootics), 28, 93–111.
- Berg, T.P. (2000). Acute infectious bursal disease in poultry: a review. Avian Pathology, 29, 175–194. doi: 10.1080/03079450050045431
- Böni, J., Stalder, J., Reigel, F. & Schüpbach, J. (1996). Detection of reverse transcriptase activity in live attenuated virus vaccines. Clinical and Diagnostic Virology, 5, 43–53. doi: 10.1016/0928-0197(95)00159-X
- Boseret, G., Losson, B., Mainil, J.G., Thiry, E. & Saegerman, C. (2013). Zoonoses in pet birds: review and perspectives. Veterinary Research, 44, 36. doi: 10.1186/1297-9716-44-36
- Bross, I. (1950). Estimates of the LD 50: a critique. Biometrics, 6, 413–423. doi: 10.2307/3001785
- Capua, I. & Marangon, S. (2006). Control of avian influenza in poultry. Emerging Infectious Diseases, 12, 1319–1324. doi: 10.3201/eid1209.060430
- Casais, R., Dove, B., Cavanagh, D. & Britton, P. (2003). Recombinant avian infectious bronchitis virus expressing a heterologous spike gene demonstrates that the spike protein is a determinant of cell tropism. Journal of Virology, 77, 9084–9089. doi: 10.1128/JVI.77.16.9084-9089.2003
- Cavanagh, D. (2003). Severe acute respiratory syndrome vaccine development: experiences of vaccination against avian infectious bronchitis coronavirus. Avian Pathology, 32, 567–582. doi: 10.1080/03079450310001621198
- Cavanagh, D. (2007). Coronavirus avian infectious bronchitis virus. Veterinary Research, 38, 281–297. doi: 10.1051/vetres:2006055
- Chen, G.-Q., Zhuang, Q.-Y., Wang, K.-C., Liu, S., Shao, J.-Z., Jiang, W.-M., Hou, G.-Y., Li, J.-P., Yu, J.-M., Li, Y.-P. & Chen, J.-M. (2013). Identification and survey of a novel avian coronavirus in ducks. PloS One, 8, e72918. doi: 10.1371/journal.pone.0072918
- Chen, H.Y., Guo, A.Z., Peng, B., Zhang, M.F., Guo, H.Y. & Chen, H.C. (2007). Infection of HeLa cells by avian infectious bronchitis virus is dependent on cell status. Avian Pathology, 36, 269–274. doi: 10.1080/03079450701447291
- Chua, J.V. & Chen, W.H. (2010). Bench-to-bedside review: vaccine protection strategies during pandemic flu outbreaks. Critical Care, 14, 218. doi: 10.1186/cc8891
- Clifton, M. (2011). How to eradicate canine rabies: a perspective of historical efforts. Asian Biomed, 5, 559–568.
- Collins, M.S., Bashiruddin, J.B. & Alexander, D.J. (1993). Deduced amino acid sequences at the fusion protein cleavage site of Newcastle disease viruses showing variation in antigenicity and pathogenicity. Archives of Virology, 128, 363–370. doi: 10.1007/BF01309446
- Conan, A., Goutard, F.L., Sorn, S. & Vong, S. (2012). Biosecurity measures for backyard poultry in developing countries: a systematic review. BMC Veterinary Research, 8, 240. doi: 10.1186/1746-6148-8-240
- Connor, J.H., McKenzie, M.O., Parks, G.D. & Lyles, D.S. (2007). Antiviral activity and RNA polymerase degradation following Hsp90 inhibition in a range of negative strand viruses. Virology, 362, 109–119. doi: 10.1016/j.virol.2006.12.026
- Coria, M.F. & Peterson, J.K. (1971). Adaptation and propagation of avian infectious bronchitis virus in embryonic turkey kidney cell cultures. Avian Diseases, 15, 22–27. doi: 10.2307/1588383
- Couteaudier, M. & Denesvre, C. (2014). Marek's disease virus and skin interactions. Veterinary Research, 45, 36. doi: 10.1186/1297-9716-45-36
- Cowen, B.S. & Braune, M.O. (1988). The propagation of avian viruses in a continuous cell line (QT35) of Japanese quail origin. Avian Diseases, 32, 282–297. doi: 10.2307/1590815
- Davis, H.E., Rosinski, M., Morgan, J.R. & Yarmush, M.L. (2004). Charged polymers modulate retrovirus transduction via membrane charge neutralization and virus aggregation. Biophysical Journal, 86, 1234–1242. doi: 10.1016/S0006-3495(04)74197-1
- Donis, R.O., Influenza Cell Culture Working Group, Influenza Cell Culture Working Group, Davis, C.T., Foust, A., Hossain, M.J., Johnson, A., Klimov, A., Loughlin, R., Xu, X., Tsai, T., Blayer, S., Trusheim, H., Colegate, T., Fox, J., Taylor, B., Hussain, A., Barr, I., Baas, C., Louwerens, J., Geuns, E., Lee, M.-S., Venhuizen, O., Neumeier, E. & Ziegler, T. (2014). Performance characteristics of qualified cell lines for isolation and propagation of influenza viruses for vaccine manufacturing. Vaccine, 32, 6583–6590. doi: 10.1016/j.vaccine.2014.06.045
- Ebert, O., Shinozaki, K., Kournioti, C., Park, M.-S., García-Sastre, A. & Woo, S.L.C. (2004). Syncytia induction enhances the oncolytic potential of vesicular stomatitis virus in virotherapy for cancer. Cancer Research, 64, 3265–3270. doi: 10.1158/0008-5472.CAN-03-3753
- Ehreth, J. (2003). The value of vaccination: a global perspective. Vaccine, 21, 4105–4117. doi: 10.1016/S0264-410X(03)00377-3
- EMA. (2014). WC500167255: CVMP Assessment Report for Parvoduk (EMEA/V/C/002740). International non-proprietary name: live attenuated Muscovy duck parvovirus. EMA/103539/2014.
- Enserink, M. (2004). Influenza: crisis underscores fragility of vaccine production system. Science, 306, 385. doi: 10.1126/science.306.5695.385
- Fey, S.B., Siepielski, A.M., Nusslé, S., Cervantes-Yoshida, K., Hwan, J.L., Huber, E.R., Fey, M.J., Catenazzi, A. & Carlson, S.M. (2015). Recent shifts in the occurrence, cause, and magnitude of animal mass mortality events. Proceedings of the National Academy of Sciences of the United States of America, 112, 1083–1088.
- Fitzpatrick, M.C., Hampson, K., Cleaveland, S., Mzimbiri, I., Lankester, F., Lembo, T., Meyers, L.A., Paltiel, A.D. & Galvani, A.P. (2014). Cost-effectiveness of canine vaccination to prevent human rabies in rural Tanzania. Annals of Internal Medicine, 160, 91–100. doi: 10.7326/M13-0542
- Galloway, J.N., Burke, M., Bradford, G.E., Naylor, R., Falcon, W., Chapagain, A.K., Gaskell, J.C., McCullough, E., Mooney, H.A., Oleson, K.L.L., Steinfeld, H., Wassenaar, T. & Smil, V. (2007). International trade in meat: the tip of the pork chop. Ambio, 36, 622–629. doi: 10.1579/0044-7447(2007)36[622:ITIMTT]2.0.CO;2
- Gambotto, A., Barratt-Boyes, S.M., de Jong, M.D., Neumann, G. & Kawaoka, Y. (2008). Human infection with highly pathogenic H5N1 influenza virus. Lancet, 371, 1464–1475. doi: 10.1016/S0140-6736(08)60627-3
- Geerligs, H., Quanz, S., Suurland, B., Spijkers, I.E.M., Rodenberg, J., Davelaar, F.G., Jongsma, B. & Kumar, M. (2008). Efficacy and safety of cell associated vaccines against Marek's disease virus grown in a continuous cell line from chickens. Vaccine, 26, 5595–5600. doi: 10.1016/j.vaccine.2008.07.080
- Glotzer, J.B., Saltik, M., Chiocca, S., Michou, A.I., Moseley, P. & Cotten, M. (2000). Activation of heat-shock response by an adenovirus is essential for virus replication. Nature, 407, 207–211. doi: 10.1038/35025102
- Häsler, B., Gilbert, W., Jones, B.A., Pfeiffer, D.U., Rushton, J. & Otte, M.J. (2013). The economic value of one health in relation to the mitigation of zoonotic disease risks. Current Topics in Microbiology and Immunology, 365, 127–151.
- Hatakeyama, S., Sakai-Tagawa, Y., Kiso, M., Goto, H., Kawakami, C., Mitamura, K., Sugaya, N., Suzuki, Y. & Kawaoka, Y. (2005). Enhanced expression of an alpha2,6-linked sialic acid on MDCK cells improves isolation of human influenza viruses and evaluation of their sensitivity to a neuraminidase inhibitor. Journal of Clinical Microbiology, 43, 4139–4146. doi: 10.1128/JCM.43.8.4139-4146.2005
- Hoa, L.K., Hiep, L.V. & Be, L.V. (2011). Development of pandemic influenza vaccine production capacity in Viet Nam. Vaccine, 29 ( Suppl. 1), A34–36. doi: 10.1016/j.vaccine.2011.04.118
- Hosseini, P., Sokolow, S.H., Vandegrift, K.J., Kilpatrick, A.M. & Daszak, P. (2010). Predictive power of air travel and socio-economic data for early pandemic spread. PloS One, 5, e12763. doi: 10.1371/journal.pone.0012763
- Hung, J.-J., Chung, C.-S. & Chang, W. (2002). Molecular chaperone Hsp90 is important for vaccinia virus growth in cells. Journal of Virology, 76, 1379–1390. doi: 10.1128/JVI.76.3.1379-1390.2002
- Ito, T., Couceiro, J.N., Kelm, S., Baum, L.G., Krauss, S., Castrucci, M.R., Donatelli, I., Kida, H., Paulson, J.C., Webster, R.G. & Kawaoka, Y. (1998). Molecular basis for the generation in pigs of influenza A viruses with pandemic potential. Journal of Virology, 72, 7367–7373.
- Jones, B.A., Grace, D., Kock, R., Alonso, S., Rushton, J., Said, M.Y., McKeever, D., Mutua, F., Young, J., McDermott, J. & Pfeiffer, D.U. (2013). Zoonosis emergence linked to agricultural intensification and environmental change. Proceedings of the National Academy of Sciences of the United States of America, 110, 8399–8404. doi: 10.1073/pnas.1208059110
- Jordan, I., Horn, D., Oehmke, S., Leendertz, F.H. & Sandig, V. (2009a). Cell lines from the Egyptian fruit bat are permissive for modified vaccinia Ankara. Virus Research, 145, 54–62. doi: 10.1016/j.virusres.2009.06.007
- Jordan, I., Vos, A., Beilfuss, S., Neubert, A., Breul, S. & Sandig, V. (2009b). An avian cell line designed for production of highly attenuated viruses. Vaccine, 27, 748–756. doi: 10.1016/j.vaccine.2008.11.066
- Jordan, I., Lohr, V., Genzel, Y., Reichl, U. & Sandig, V. (2013). Elements in the development of a production process for modified vaccinia virus Ankara. Microorganisms, 1, 100–121. doi: 10.3390/microorganisms1010100
- Jordan, I., Northoff, S., Thiele, M., Hartmann, S., Horn, D., Höwing, K., Bernhardt, H., Oehmke, S., von Horsten, H., Rebeski, D., Hinrichsen, L., Zelnik, V., Mueller, W. & Sandig, V. (2011). A chemically defined production process for highly attenuated poxviruses. Biologicals: Journal of the International Association of Biological Standardization, 39, 50–58. doi: 10.1016/j.biologicals.2010.11.005
- Jordan, I. & Sandig, V. (2008). Cell Line for Propagation of Highly Attenuated Alphaviruses. US Patent WO/2009/156155, pp. 1–42. Priority Date 25 June 2008.
- Kapczynski, D.R., Esaki, M., Dorsey, K.M., Jiang, H., Jackwood, M., Moraes, M. & Gardin, Y. (2015). Vaccine protection of chickens against antigenically diverse H5 highly pathogenic avian influenza isolates with a live HVT vector vaccine expressing the influenza hemagglutinin gene derived from a clade 2.2 avian influenza virus. Vaccine, 33, 1197–1205. doi: 10.1016/j.vaccine.2014.12.028
- Kim, J.-K., Negovetich, N.J., Forrest, H.L. & Webster, R.G. (2009). Ducks: the “Trojan horses” of H5N1 influenza. Influenza and Other Respiratory Viruses, 3, 121–128. doi: 10.1111/j.1750-2659.2009.00084.x
- Kisary, J., Derzsy, D. & Meszaros, J. (1978). Attenuation of the goose parvovirus strain B. Laboratory and field trials of the attenuated mutant for vaccination against Derzsy's disease. Avian Pathology, 7, 397–406. doi: 10.1080/03079457808418293
- Kobasa, D., Jones, S.M., Shinya, K., Kash, J.C., Copps, J., Ebihara, H., Hatta, Y., Kim, J.H., Halfmann, P., Hatta, M., Feldmann, F., Alimonti, J.B., Fernando, L., Li, Y., Katze, M.G., Feldmann, H. & Kawaoka, Y. (2007). Aberrant innate immune response in lethal infection of macaques with the 1918 influenza virus. Nature, 445, 319–323. doi: 10.1038/nature05495
- Li, F.C.K., Choi, B.C.K., Sly, T. & Pak, A.W.P. (2008). Finding the real case-fatality rate of H5N1 avian influenza. Journal of Epidemiology and Community Health, 62, 555–559. doi: 10.1136/jech.2007.064030
- Li, J., Hu, H., Yu, Q., Diel, D.G., Li, D. & Miller, P.J. (2012). Generation and characterization of a recombinant Newcastle disease virus expressing the red fluorescent protein for use in co-infection studies. Virology Journal, 9, 227. doi: 10.1186/1743-422X-9-227
- Litamoi, J., Palya, V.J., Sylla, D. & Rweyemamu, M.M. (1996). Quality Control Testing of Contagious Bovine Pleuropneumonia Live Attenuated Vaccine. Standard operating procedure 2: Estimation of viable Mycoplasma Content of CBPP Vaccines (Microtitration Method).
- Liu, S., Chen, J., Chen, J., Kong, X., Shao, Y., Han, Z., Feng, L., Cai, X., Gu, S. & Liu, M. (2005). Isolation of avian infectious bronchitis coronavirus from domestic peafowl (Pavo cristatus) and teal (Anas). The Journal of General Virology, 86, 719–725. doi: 10.1099/vir.0.80546-0
- Lohr, V., Genzel, Y., Jordan, I., Katinger, D., Mahr, S., Sandig, V. & Reichl, U. (2012). Live attenuated influenza viruses produced in a suspension process with avian AGE1.CR.pIX cells. BMC Biotechnology, 12, 79. doi: 10.1186/1472-6750-12-79
- Lohr, V., Rath, A., Genzel, Y., Jordan, I., Sandig, V. & Reichl, U. (2009). New avian suspension cell lines provide production of influenza virus and MVA in serum-free media: studies on growth, metabolism and virus propagation. Vaccine, 27, 4975–4982. doi: 10.1016/j.vaccine.2009.05.083
- Madu, I.G., Chu, V.C., Lee, H., Regan, A.D., Bauman, B.E. & Whittaker, G.R. (2007). Heparan sulfate is a selective attachment factor for the avian coronavirus infectious bronchitis virus Beaudette. Avian Diseases, 51, 45–51. doi: 10.1637/0005-2086(2007)051[0045:HSIASA]2.0.CO;2
- Maurin-Bernaud, L., Goutebroze, S., Merdy, O., Chanay, A., Cozette, V. & Le Gros, F.-X. (2014). Efficacy of a new attenuated duck parvovirosis vaccine in Muscovy ducks. The Veterinary Record, 175, 281. doi: 10.1136/vr.102584
- McLeod, A. & Rushton, J. (2007). Economics of animal vaccination. Revue Scientifique Et Technique (International Office of Epizootics), 26, 313–326.
- Melville, M.W., Tan, S.L., Wambach, M., Song, J., Morimoto, R.I. & Katze, M.G. (1999). The cellular inhibitor of the PKR protein kinase, P58(IPK), is an influenza virus-activated co-chaperone that modulates heat shock protein 70 activity. The Journal of Biological Chemistry, 274, 3797–3803. doi: 10.1074/jbc.274.6.3797
- Mészáros, I., Tóth, R., Bálint, A., Dán, A., Jordan, I. & Zádori, Z. (2014). Propagation of viruses infecting waterfowl on continuous cell lines of Muscovy duck (Cairina moschata) origin. Avian Pathology, 43, 379–386.
- Monath, T.P. (2013). Vaccines against diseases transmitted from animals to humans: a one health paradigm. Vaccine, 31, 5321–5338. doi: 10.1016/j.vaccine.2013.09.029
- Monne, I., Hussein, H.A., Fusaro, A., Valastro, V., Hamoud, M.M., Khalefa, R.A., Dardir, S.N., Radwan, M.I., Capua, I. & Cattoli, G. (2013). H9N2 influenza A virus circulates in H5N1 endemically infected poultry population in Egypt. Influenza and Other Respiratory Viruses, 7, 240–243. doi: 10.1111/j.1750-2659.2012.00399.x
- Müller, H., Mundt, E., Eterradossi, N. & Islam, M.R. (2012). Current status of vaccines against infectious bursal disease. Avian Pathology, 41, 133–139. doi: 10.1080/03079457.2012.661403
- Munch, D., Hohlstein, L. & Sevoian, M. (1978). In vitro establishment of Marek's disease herpesvirus-transformed productive and nonproductive lymphoblastoid cell lines. Infection and Immunity, 20, 315–318.
- Muramatsu, M. & Homma, M. (1980). Trypsin action on the growth of Sendai virus in tissue culture cells. V. An activating enzyme for Sendai virus in the chorioallantoic fluid of the embryonated chicken egg. Microbiology and Immunology, 24, 113–122. doi: 10.1111/j.1348-0421.1980.tb00569.x
- Nagai, Y., Shimokata, K., Yoshida, T., Hamaguchi, M., Iinuma, M., Maeno, K., Matsumoto, T., Klenk, H.D. & Rott, R. (1979). The spread of a pathogenic and an apathogenic strain of Newcastle disease virus in the chick embryo as depending on the protease sensitivity of the virus glycoproteins. The Journal of General Virology, 45, 263–272. doi: 10.1099/0022-1317-45-2-263
- Nair, V. (2005). Evolution of Marek's disease – a paradigm for incessant race between the pathogen and the host. The Veterinary Journal, 170, 175–183. doi: 10.1016/j.tvjl.2004.05.009
- Narrod, C., Zinsstag, J. & Tiongco, M. (2012). A one health framework for estimating the economic costs of zoonotic diseases on society. EcoHealth, 9, 150–162. doi: 10.1007/s10393-012-0747-9
- Normile, D. (2008). Rinderpest: driven to extinction. Science, 319, 1606–1609. doi: 10.1126/science.319.5870.1606
- O'Brien, D. & Zanker, S. (2007). Animal vaccination and the veterinary pharmaceutical industry. Revue Scientifique Et Technique (International Office of Epizootics), 26, 471–477.
- OIE. (2008). Chapter 2.3.12. Infectious bursal disease (Gumboro disease), OIE Terrestrial Manual, 549–565.
- OIE. (2010). Chapter 2.3.13. Marek's disease, OIE Terrestrial Manual, 1–11.
- OIE. (2013a). Chapter 2.3.14. Newcastle disease, OIE Terrestrial Manual, 1–19.
- OIE. (2013b). Chapter 2.3.2. Avian infectious bronchitis, OIE Terrestrial Manual, 1–15.
- OIE. (2014). Chapter 2.3.4. Avian Influenza, OIE Terrestrial Manual, 436–454.
- Osterrieder, N. & Schumacher, D. (2002). A Continuous Cell Line for the Production of Vaccines. WO/2003/066093 A1.
- Palya, V., Kiss, I., Tatár-Kis, T., Mató, T., Felföldi, B. & Gardin, Y. (2012). Advancement in vaccination against Newcastle disease: recombinant HVT NDV provides high clinical protection and reduces challenge virus shedding with the absence of vaccine reactions. Avian Diseases, 56, 282–287. doi: 10.1637/9935-091511-Reg.1
- Palya, V., Zolnai, A., Benyeda, Z., Kovács, E., Kardi, V. & Mató, T. (2009). Short beak and dwarfism syndrome of mule duck is caused by a distinct lineage of goose parvovirus. Avian Pathology, 38, 175–180. doi: 10.1080/03079450902737839
- Parks, R.J. (2005). Adenovirus protein IX: a new look at an old protein. Molecular Therapy: The Journal of the American Society of Gene Therapy, 11, 19–25. doi: 10.1016/j.ymthe.2004.09.018
- Pfaffl, M.W. (2001). A new mathematical model for relative quantification in real-time RT-PCR. Nucleic Acids Research, 29, e45. doi: 10.1093/nar/29.9.e45
- Promkuntod, N., Wickramasinghe, I.N.A., de Vrieze, G., Gröne, A. & Verheije, M.H. (2013). Contributions of the S2 spike ectodomain to attachment and host range of infectious bronchitis virus. Virus Research, 177, 127–137. doi: 10.1016/j.virusres.2013.09.006
- Ren, Z., Lu, Z., Wang, L., Huo, Z., Cui, J., Zheng, T., Dai, Q., Chen, C., Qin, M., Chen, M. & Yang, R. (2015). Rapid production of a H9N 2 influenza vaccine from MDCK cells for protecting chicken against influenza virus infection. Applied Microbiology and Biotechnology, 99, 2999–3013. doi: 10.1007/s00253-015-6406-7
- Richard, M., de Graaf, M. & Herfst, S. (2014). Avian influenza A viruses: from zoonosis to pandemic. Future Virology, 9, 513–524. doi: 10.2217/fvl.14.30
- Roth, S. & Kaaden, O.R. (1985). Use of chicken cell line LSCC-H32 for titration of animal viruses and exogenous chicken interferon. Applied and Environmental Microbiology, 49, 634–636.
- Rott, R., Reinacher, M., Orlich, M. & Klenk, H.D. (1980). Cleavability of hemagglutinin determines spread of avian influenza viruses in the chorioallantoic membrane of chicken embryo. Archives of Virology, 65, 123–133. doi: 10.1007/BF01317323
- Salminen, A., Paimela, T., Suuronen, T. & Kaarniranta, K. (2008). Innate immunity meets with cellular stress at the IKK complex: regulation of the IKK complex by HSP70 and HSP90. Immunology Letters, 117, 9–15. doi: 10.1016/j.imlet.2007.12.017
- Sambo, E., Bettridge, J., Dessie, T., Amare, A., Habte, T., Wigley, P. & Christley, R.M. (2015). Participatory evaluation of chicken health and production constraints in Ethiopia. Preventive Veterinary Medicine, 118, 117–127. doi: 10.1016/j.prevetmed.2014.10.014
- Sandig, V. & Jordan, I. (2008). Immortalized Avian Cell Lines for Virus Production. European Patent EP 1939281 A1, pp. 1–49. Priority Date 3 November 2003.
- Schettler, C.H. (1973). Virus hepatitis of geese. 3. Properties of the causal agent. Avian Pathology, 2, 179–193. doi: 10.1080/03079457309353795
- Schumacher, D., Tischer, B.K., Reddy, S.M. & Osterrieder, N. (2001). Glycoproteins E and I of Marek's disease virus serotype 1 are essential for virus growth in cultured cells. Journal of Virology, 75, 11307–11318. doi: 10.1128/JVI.75.23.11307-11318.2001
- Sehgal, R.N.M. (2010). Deforestation and avian infectious diseases. The Journal of Experimental Biology, 213, 955–960. doi: 10.1242/jeb.037663
- Shinya, K., Ebina, M., Yamada, S., Ono, M., Kasai, N. & Kawaoka, Y. (2006). Avian flu: influenza virus receptors in the human airway. Nature, 440, 435–436. doi: 10.1038/440435a
- Sjaak de Wit, J.J., Cook, J.K.A. & van der Heijden, H.M.J.F. (2011). Infectious bronchitis virus variants: a review of the history, current situation and control measures. Avian Pathology, 40, 223–235. doi: 10.1080/03079457.2011.566260
- Sonnberg, S., Webby, R.J. & Webster, R.G. (2013). Natural history of highly pathogenic avian influenza H5N1. Virus Research, 178, 63–77. doi: 10.1016/j.virusres.2013.05.009
- Sun, Y. & Liu, J. (2015). H9N2 influenza virus in China: a cause of concern. Protein & Cell, 6, 18–25. doi: 10.1007/s13238-014-0111-7
- Tatár-Kis, T., Mató, T., Markos, B. & Palya, V. (2004). Phylogenetic analysis of Hungarian goose parvovirus isolates and vaccine strains. Avian Pathology, 33, 438–444. doi: 10.1080/03079450410001724067
- Tscherne, D.M. & García-Sastre, A. (2011). Virulence determinants of pandemic influenza viruses. The Journal of Clinical Investigation, 121, 6–13. doi: 10.1172/JCI44947
- Tulman, E.R., Afonso, C.L., Lu, Z., Zsak, L., Rock, D.L. & Kutish, G.F. (2000). The genome of a very virulent Marek's disease virus. Journal of Virology, 74, 7980–7988. doi: 10.1128/JVI.74.17.7980-7988.2000
- Uscher-Pines, L., Barnett, D.J., Sapsin, J.W., Bishai, D.M. & Balicer, R.D. (2008). A systematic analysis of influenza vaccine shortage policies. Public Health, 122, 183–191. doi: 10.1016/j.puhe.2007.06.005
- Veits, J., Weber, S., Stech, O., Breithaupt, A., Gräber, M., Gohrbandt, S., Bogs, J., Hundt, J., Teifke, J.P., Mettenleiter, T.C. & Stech, J. (2012). Avian influenza virus hemagglutinins H2, H4, H8, and H14 support a highly pathogenic phenotype. Proceedings of the National Academy of Sciences of the United States of America, 109, 2579–2584. doi: 10.1073/pnas.1109397109
- Vellinga, J., Van der Heijdt, S. & Hoeben, R.C. (2005). The adenovirus capsid: major progress in minor proteins. The Journal of General Virology, 86, 1581–1588. doi: 10.1099/vir.0.80877-0
- Vos, A., Un, H., Hampson, K., De Balogh, K., Aylan, O., Freuling, C.M., Müller, T., Fooks, A.R. & Johnson, N. (2014). Bovine rabies in Turkey: patterns of infection and implications for costs and control. Epidemiology and Infection, 142, 1925–1933. doi: 10.1017/S0950268813002811
- Wan, H. & Perez, D.R. (2007). Amino acid 226 in the hemagglutinin of H9N2 influenza viruses determines cell tropism and replication in human airway epithelial cells. Journal of Virology, 81, 5181–5191. doi: 10.1128/JVI.02827-06
- Wan, H., Sorrell, E.M., Song, H., Hossain, M.J., Ramirez-Nieto, G., Monne, I., Stevens, J., Cattoli, G., Capua, I., Chen, L.-M., Donis, R.O., Busch, J., Paulson, J.C., Brockwell, C., Webby, R., Blanco, J., Al-Natour, M.Q. & Perez, D.R. (2008). Replication and transmission of H9N2 influenza viruses in ferrets: evaluation of pandemic potential. PloS One, 3, e2923. doi: 10.1371/journal.pone.0002923
- Wang, X.-J. (2011). Heat-shock protein 70 is associated with the entry of Marek's disease virus into fibroblast. Acta Virologica, 55, 189–194. doi: 10.4149/av_2011_03_189
- Witter, R.L., Calnek, B.W., Buscaglia, C., Gimeno, I.M. & Schat, K.A. (2005). Classification of Marek's disease viruses according to pathotype: philosophy and methodology. Avian Pathology, 34, 75–90. doi: 10.1080/03079450500059255
- World Health Organization. (1998). Reverse transcriptase activity in chicken-cell derived vaccine. Weekly Epidemiological Record/Health Section of the Secretariat of the League of Nations, 73, 209–212.
- World Health Organization. (2013). Research priorities for the environment, agriculture and infectious diseases of poverty. World Health Organization Technical Report Series, i–xiii, 1–125.
- Yamaguchi, T., Kaplan, S.L., Wakenell, P. & Schat, K.A. (2000). Transactivation of latent Marek's disease herpesvirus genes in QT35, a quail fibroblast cell line, by herpesvirus of turkeys. Journal of Virology, 74, 10176–10186. doi: 10.1128/JVI.74.21.10176-10186.2000
- Zamarin, D. & Palese, P. (2012). Oncolytic Newcastle disease virus for cancer therapy: old challenges and new directions. Future Microbiology, 7, 347–367. doi: 10.2217/fmb.12.4
- Zhang, Y., Buckles, E. & Whittaker, G.R. (2012). Expression of the C-type lectins DC-SIGN or L-SIGN alters host cell susceptibility for the avian coronavirus, infectious bronchitis virus. Veterinary Microbiology, 157, 285–293. doi: 10.1016/j.vetmic.2012.01.011
- Zhao, Y., Kurian, D., Xu, H., Petherbridge, L., Smith, L.P., Hunt, L. & Nair, V. (2009). Interaction of Marek's disease virus oncoprotein Meq with heat-shock protein 70 in lymphoid tumour cells. The Journal of General Virology, 90, 2201–2208. doi: 10.1099/vir.0.012062-0
- Zhirnov, O.P., Ovcharenko, A.V. & Bukrinskaya, A.G. (1985). Myxovirus replication in chicken embryos can be suppressed by aprotinin due to the blockage of viral glycoprotein cleavage. The Journal of General Virology, 66, 1633–1638. doi: 10.1099/0022-1317-66-7-1633
- Zinsstag, J., Schelling, E., Roth, F., Bonfoh, B., de Savigny, D. & Tanner, M. (2007). Human benefits of animal interventions for zoonosis control. Emerging Infectious Diseases, 13, 527–531. doi: 10.3201/eid1304.060381