ABSTRACT
Probiotics and immunization are being widely adopted by the poultry industry with the goal of controlling Salmonella enterica. However, the interaction between these two management protocols has been sparsely studied. The present study aimed to understand the role of an Enterococcus faecium probiotic in the production of salmonella-specific IgA in layers immunized with a live vaccine. Four groups were used: “Control” (no vaccine or probiotic); “Probiotic” (which received an E. faecium product); “Vaccine” (immunized with two doses of a live attenuated S. Enteritidis vaccine); and “Vaccine + probiotic”. Faecal salmonella-specific IgA was analysed 7 and 20 days post-vaccination (dpv) boost. At 7 dpv, the “Vaccine” and “Vaccine + probiotic” groups had similar IgA levels. However, at 20 dpv, IgA levels were two times higher in the “Vaccine + probiotic” group compared to the “Vaccine” group. To understand the role of the intestinal microbiota in this finding, bacterial diversity in faeces was analysed by 16S rRNA gene sequencing. The improvement in IgA production in probiotic-treated birds was accompanied by marked changes in the faecal microbiome. Some of the main differences between the “Vaccine” and “Vaccine + probiotic” groups included reduction of Escherichia-Shigella and increases in Blautia, Anaerotruncus and Lactobacillus in the latter group. Although no direct causal link can be established from this study design, it is possible that the E. faecium probiotic induces improved antibody production following vaccination via modulation of the intestinal microbiota.
Research Highlights
A probiotic is shown to enhance the IgA response following Salmonella vaccination.
The correlation of this immune effect with the microbiota is explored.
Introduction
Vaccination of broiler breeders and layers with live attenuated Salmonella strains has become the norm in several countries. The ultimate goal of this prophylactic strategy is the reduction of the contamination of food products and of the progeny (Collard et al., Citation2008; Dórea et al., Citation2010). Protection induced by attenuated Salmonella depends on a myriad of immune responses, among which are modulation of cells recruited to the mucosae and production of local IgA (Carvajal et al., Citation2008; Dal Bérto et al., Citation2015). Mucosal IgA levels, in conjunction with other immune components, are one of the several parameters associated with protectivity following Salmonella immunization (Liu et al., Citation2001; Jawale & Lee, Citation2014).
Mucosae are important barriers against the invasion of pathogens. Among the most important procedures for maintaining integral gut health, use of growth promoter antibiotics is being gradually reduced throughout the world (Phillips et al., Citation2004). As an alternative, probiotics are being more frequently used and therefore understanding their mechanisms of action is growing (Kabir, Citation2009).
Although it seems to be the case that, occasionally, probiotics can induce improved responses to live oral vaccines, this is not consistent; to the contrary, it can also be speculated that competitive exclusion or activation of opposing immune pathways by probiotics may play a role in diminishing the immunogenicity of some replicating vaccines. Indeed, on occasions, probiotics have negatively affected vaccination outcomes (Praharaj et al., Citation2015). Vaccination and probiotics are important flock health management tools, and often are administered concomitantly or in close succession. However, with the current knowledge, it is not possible to predict how a given combination of products will interact (Kuritza et al., Citation2013; Valdez et al., Citation2014).
It is considered that efficient priming of immune responses by live vaccines requires at least moderate persistence of colonization by the immunogenic agent (Capozzo et al., Citation2004). The probiotic studied here (Enterococcus faecium) has been shown to reduce the presence of S. Minnesota in the cecum (Kuritza et al., Citation2013); this raises the possibility that simultaneous administration of the probiotic and a live salmonella vaccine could hinder the immune effects of the latter by rapidly removing it from the host.
Therefore, the aim of the present study was to elucidate if an E. faecium probiotic interacts with an attenuated Salmonella enterica Enteritidis (S. Enteritidis) vaccine. For this, IgA production against a salmonella surface antigen was assessed, and whether this immune response could be correlated with changes in the intestinal microbiota.
Materials and methods
Birds and procedures
Forty Embrapa-51 layers were divided into four groups: “Control” (no vaccine or probiotic); “Probiotic” (which received E. faecium NCIMB 10415, Cylactin®, DSM Nutritional Products, Heerlen, Netherlands); “Vaccine” (immunized with two doses of a live attenuated S. Enteritidis vaccine, Gallivac®, Merial, Lyon, France) and “Vaccine + probiotic”. Birds were vaccinated at 1 and 28 days of age, following the manufacturer’s protocol. Probiotic was administered continuously throughout the experiment. Birds were fed ad libitum with a mash diet designed to meet their nutritional requirements (Rostagno et al., Citation2005); the respective groups had probiotic added to the feed from day 1 at the concentration of 4 × 108 CFU/kg. Birds were housed in isolators with HEPA-filtered air flow; contact between the bird handlers and the birds was made exclusively from outside the isolator after housing, to avoid live vaccine or probiotic spread between the groups.
At 1, 35 and 48 days of age (−27, 7 and 20 days in relation to the booster vaccine), faecal samples were collected. For IgA measurements, 7–10 samples/group were collected on each date with a urinary catheter directly from the cloaca. For microbiome analysis, one sample/group was collected at day 1 and four pooled samples/group were collected at days 35 and 49. Faecal samples were frozen at −20°C until processed. For qualitative analysis of presence of Salmonella in the bedding, two swabs/group were collected from the “Control” and “Vaccine” groups at day 21. This was done to confirm the infection status of these groups.
Bird procedures were approved by the Animal Experimentation Ethics Committee of the Federal University of Paraná, process CEUA SCA UFPR 059/2016.
IgA analysis
This assay was performed as described previously (Dal Bérto et al., Citation2015; Salk et al., Citation2016). Briefly, faecal samples were diluted 1:5 in PBS + sodium azide. ELISA plates were coated with Salmonella Enteritidis lipopolysaccharide (LPS) (field isolate strain from Paraná, Brazil) at 150 ng/well. Plates were then washed thrice with 200 μl/well of PBS + 0.05% Tween 20 for 5 min/wash. Wells were blocked with 1% casein in PBS. Samples (100 μl) were tested in triplicate for 2 h at 37°C. Plates were washed and secondary antibody was added (HRP-conjugated anti-chicken IgA, AbD Serotec, Hercules, CA) diluted in 0.1% casein. After washing, the assay was developed with 3,3',5,5'-tetramethylbenzidine single solution (Life Technologies, Carlsbad, CA). Absorbance was read at 450 nm.
Microbiology
On day 21, the presence of Salmonella was assessed in the isolators of the “Control” and “Vaccine” groups. The bedding was swabbed and enriched in peptone water overnight. One millilitre was grown in both tetrathionate and Rappaport Vassiliadis broths and later spread on Brilliant Green Agar. The identity of the resulting characteristic colonies was confirmed biochemically and with serological testing of somatic (poly-O, O:4 and O:9) and flagellar (Hgm, Hgp, Hp and Hm) antigens (modified from Santos et al., Citation2005). Viability of the probiotic in the feed was confirmed at the end of the trial.
DNA extraction, sequencing and bioinformatics
DNA was extracted from faeces using the QIAamp DNA Stool Mini Kit (Qiagen, Hilden, Germany). DNA was quantified with a Nanodrop® spectrophotometer (Thermo Scientific, Carlsbad, CA). The variable V4 region of 16S rRNA was amplified using the universal primers 515F and 806R (Caporaso et al., Citation2011). PCR conditions were as follows: 94°C, 3 min; 18 cycles of 94°C, 45 s, 50°C, 30 s and 68°C, 60 s; followed by 72°C, 10 min. These amplicons were then sequenced (Illumina MiSeq, San Diego, CA). Sequencing reads were analysed with the Quantitative Insights Into Microbial Ecology platform (Caporaso et al., Citation2010, Citation2011). Sequences were classified into bacterial genera through the recognition of operational taxonomic units (OTUs) based on the homology of the sequences when compared to the latest update of the SILVA ribosomal sequence database [123 2016 release] (Yilmaz et al., Citation2013). Basic diversity analysis (OTU number and Unifrac-distances) was conducted using Quantitative Insights Into Microbial Ecology, and the OTU table exported to R for further analyses. Sequence data were submitted to the BioSample database under accession number SAMN08176750.
Statistical analysis
For statistical analysis of IgA data, the average of the technical replicates of each sample was used. Data were analysed by two-way ANOVA with Tukey post hoc test (P < 0.05). For genus analysis of the microbiome, the most frequent genera were selected. Phylum and diversity analysis were performed using the entire data set. Data were analysed with the R platform (The R Project). Data distribution was analysed by Shapiro–Wilk test, and groups were compared by ANOVA with a Tukey post hoc test assessing both mean treatment effects and differences in each time point. Graphs were constructed with GraphPad Prism 6 (GraphPad Software). Pairwise Pearson’s correlation was performed using R package, “psych” version.
Results
The effects of a probiotic on the immune responses induced by a live Salmonella vaccine were studied. For this, mucosal salmonella-specific IgA was measured. The faecal microbiome was assessed to identify possible factors that intermediated the actions of the probiotic on immune responses.
IgA
Group “Vaccine + probiotic” showed the highest level of salmonella-specific IgA at 7 and 20 days post-vaccination (dpv). This difference in relation to the “Vaccine” group was statistically significant at 20 days PV (). The interaction between probiotic and vaccination was significantly associated with the results seen at this date (). Unexpectedly, the “Control” group showed an increase in IgA levels at 7 dpv ().
Figure 1. Use of probiotic increased Salmonella-specific faecal IgA levels at 20 dpv. Results show all collection points. Statistically significant differences (two-way ANOVA, P < 0.05) comparing all groups at a given date are indicated by different letters with the colour of the respective group. Differences of mean treatment effects are shown in the table below the graph. Figure shows average ± SD.
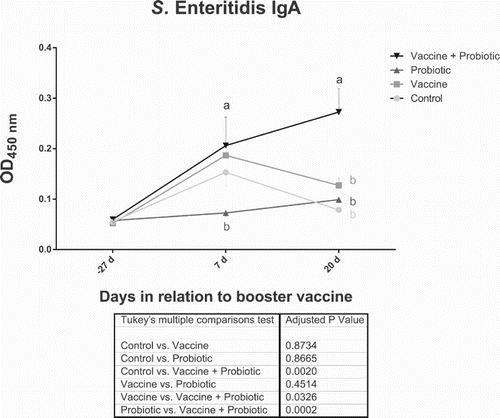
Microbiome
As shown above, the addition of probiotic affected mucosal immune responses against a Salmonella live vaccine at 20 dpv. The microbiota were analysed by next-generation sequencing with the aim of investigating its correlation to the immune findings. Over 400 bacterial genera were identified. Minimum and maximum read counts per sample were 4500 and 780,484. Analyses were performed with 4500 read counts for all samples, to avoid bias.
Principal Coordinate Analysis showed marked differences between the microbiota of 1-day-old chicks and of 35- (7 dpv) and 49-day-old (20 dpv) chicks. There was an interesting exception, as the “Control” group showed a microbial profile at 35 days that was very similar to that of 1-day-old chicks (). This reflected on the overall bacterial population diversity and phylum composition, which was significantly different between the negative control group and all others at 7 dpv ( and ). Probiotic, vaccine and the combination of these treatments altered the microbiota consistently, as can be seen by cluster analysis. The “Vaccine + probiotic” group had reduced variation from 7 to 21 dpv if considered in relation to the vaccination-only group, which is clustered in different tertiary branches at 7 and 21 dpv (). The only phylum difference between treatments was a trend of reduction of Proteobacteria (P = 0.0768 in the main treatment effect) in the “Vaccine + probiotic” group in relation to the “Vaccine” group.
Figure 2. Time-induced changes in the overall composition of the intestinal microbiome. (A) Main coordinate analysis. Smaller dots indicate samples of 1-day-old birds. Light-grey dots indicate 7 dpv (the “Control” group at this date is indicated by the arrow). Darker dots indicate 20 dpv. (B) Cluster dendrogram. 7 dpv are shown in the darker colour, and 21 dpv in the lighter shade. Results of 1-day-old birds are not shown in the cluster dendrogram for clarity.
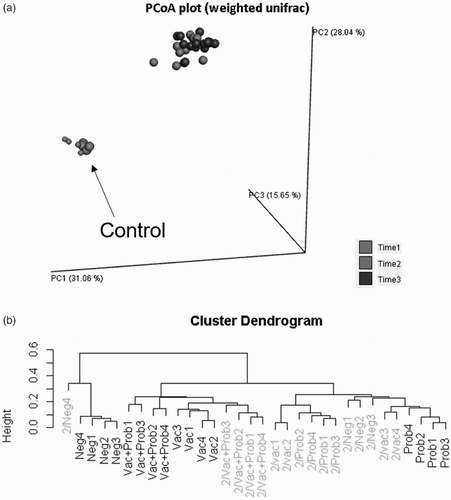
Figure 3. Bacterial population diversity. Results show collection points at 7 and 20 days post-booster vaccination. Statistically significant differences (two-way ANOVA, P < 0.05) comparing all groups at a given date are indicated by different letters. Differences of mean treatment effects are shown in the table below the graph. Results of 1-day-old birds are not shown, since these samples were comprised of a pool of all birds.
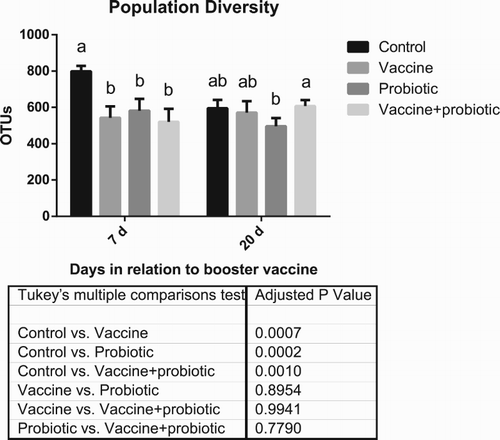
Figure 4. Distribution of the main bacterial phyla. Results show collection points at 7 and 20 days post-booster vaccination. Statistically significant differences (two-way ANOVA, P < 0.05) comparing all groups at a given date are indicated by different letters. Differences of mean treatment effects are shown in the table below the graph. Results of 1-day-old birds are not shown, since these samples were comprised of a pool of all birds.
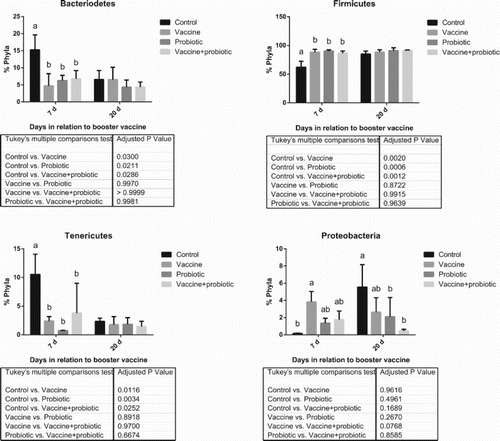
The most abundant genera were selected for individual analysis (). Genera that varied between the “Vaccine” and “Vaccine + probiotic” groups were Subdoligranulum (main treatment effect P = 0.0661; simple differences within a date are shown in ), Lactobacillus (P < 0.0001), Ruminococcaceae UCG-014 (no treatment effect difference), E. coli-Shigella (P = 0.0003), Anaerotruncus (P < 0.0001), Streptococcus (P < 0.0001) and Enterococcus (no treatment effect difference).
Table 1. Most abundant bacterial genera in the faecal microbiota. Results are shown as percentages of the total microbial composition. Different letters indicate statistical significance between the values at that collection date (rows) (Tukey P < 0.05). Asterisks indicate statistical significance between dates (columns). Results shown as mean ± SD.
E. faecium was the probiotic strain used in this test. This genus appeared in small concentrations in the faecal contents (). Nevertheless, the relative quantity of Enterococcus was highest in the “Probiotic” group at 7 and 20 dpv. In the analysis of the main treatment effects, only the “Probiotic” group differed from the negative control with regard to Enterococcus (P < 0.0001). Salmonella could not be identified in any treatment by 16S rRNA gene sequencing, despite the use of a live vaccine. The negative control and the vaccine-only group were screened by standard microbiological methods at 20 days of age (7 days before the booster vaccine) and Salmonella was only successfully isolated from the vaccinated group.
Discussion
Probiotics can have varying effects on vaccine-induced immune responses. The knowledge amounted thus far seems to indicate that the results of the association of probiotics with live vaccines are specific to each combination (Brisbin et al., Citation2011; Praharaj et al., Citation2015).
The combination of a salmonella vaccine and an E. faecium probiotic was tested in the present work. Interestingly, addition of the probiotic increased mucosal salmonella-specific IgA at 20 days post-booster vaccination. Several previous studies, mostly performed in species other than chickens, have also shown positive associations in immune responses with concomitant administration of live vaccines and probiotics (de Vrese et al., Citation2005; Zhang et al., Citation2008; Vintini et al., Citation2010; Naseem et al., Citation2012).
The bacterial genus used as probiotic in this study is active in pathways that directly modulate mucosal immunity (Lauková et al., Citation2015). Indeed, the same probiotic strain used in the present study has been shown elsewhere to affect the CD4 and CD8 cell dynamics in the intestines (Kuritza et al., Citation2013). This effect on lymphocytes could have influenced IgA production in the “Vaccine + probiotic” group, due to the auxiliary role of CD4 cells in intestinal immunoglobulin production (Cihak et al., Citation1991).
The microbiome was analysed to shed light on the possible role of the microbiota in the IgA results, and on how the probiotic might have acted indirectly on immunity. Genera and phyla found in the present study are, overall, in agreement with previous reports of the microbiota of chicken faeces (Oakley et al., Citation2014; Stanley et al., Citation2014). The probiotic genus, Enterococcus, was identifiable in the faecal contents by 16S rRNA gene sequencing, although it was undetectable in the “Vaccine + probiotic” group at 21 dpv. Detection inconsistencies could have played a role in this, as in all groups the relative abundance of the probiotic genus was very small. This genus is also commonly found in the intestines of chickens, and it is possible that the detected quantities are not correlated with the probiotic strain (Oakley et al., Citation2014). Although larger bacterial populations are intuitively more likely to have an impact on the host, less abundant bacteria are also of great relevance (Arumugam et al., Citation2011). Since probiotic viability does not seem to be a pre-requisite for its function (Adams, Citation2010; Lahtinen, Citation2012), it is possible that the bacteria in the product tested here do not replicate in the host, justifying its low abundance.
Principal Coordinate Analysis showed the expected age-related differences in the microbiota over time (Oakley et al., Citation2014). The delayed “maturation” of the microbiota of the control group at 35 days of age (corresponding to 7 dpv) was surprising. Birds were kept in strict isolation from 1 day of age. In contrast to the other three groups, external bacteria were introduced to the control group only involuntarily through feed, which was not sterile; restricted access to external bacteria may justify the similarities in microbiota of the control, at 35 days of age, and the 1-day-old birds. Treatments effectively altered the overall composition of the microbiome. Differences between “Vaccine” and “Vaccine + probiotic” increased with time, and these groups became markedly different at 21 dpv, indicating a possible role of the microbiota in the IgA response.
It is impossible to indicate, from the present study, the bacterial genera responsible for the promotion of salmonella IgA production in the “Vaccine + probiotic” group. However, the overall balance of microbial alterations induced by the combination of vaccine and probiotic represents a likely explanation for the improved outcome of vaccination. While analysis of the literature offers illustrative examples of positive and negative correlations between genera/phyla and vaccine responses, these seem to be very circumscribed to each specific situation. Mechanisms such as molecular mimicry between vaccine and microbiota will only be valid to a given immunogen, for instance (Nguyen et al., Citation2016). The effect of probiotics in inducing improved immune responses to live mucosal vaccination is not invariably positive, as it seems dependent on the vaccine itself, and on probiotic dosage, among several other variables (Valdez et al., Citation2014). Therefore, the present study helps in the delineation of the important parameters in the interaction between the E. faecium and S. Enteritidis.
Limitations of this study include the small number of samples, but especially the impossibility of making direct correlations between microbiome and immune responses, since faecal samples were pooled. Also, the negative control group showed an increase in anti-salmonella IgA at 7 dpv. Albeit this was not sustained at 20 dpv and it did not occur in the “Probiotic” group, this is relevant because it indicates cross-reactivity of IgA produced against other Proteobacteria to the LPS of salmonella, as described in the literature (Mirpuri et al., Citation2014). Finally, this study lacked a field strain challenge, which would have given definitive answers regarding protectivity and the importance of the probiotic.
The reduction of IgA in the “Vaccine” group at 20 dpv is interesting, but not entirely unexpected (Tran et al., Citation2010). Mucosal IgA memory is restricted in time by shifts in the microbiota, with the introduction of new immunodominant bacteria (Macpherson et al., Citation2012). The present study focused exclusively on the short-term immune effects of co-administration of probiotic and vaccine. It will be interesting to analyse if interaction with probiotics can also enhance long-term IgA production by the live Salmonella vaccine through stabilization of the microbiota.
Conclusion
In-feed E. faecium NCIMB 10415 probiotic increased the production of Salmonella-specific mucosal IgA following immunization with an attenuated S. Enteritidis vaccine. Modulation of the intestinal microbiome is a candidate mediator of this immune effect; the overall changes in the profile of the microbiome in the “Vaccine + probiotic” group are compatible with reported improvements in live vaccine immunogenicity. It has been suggested elsewhere that the results of the interaction between probiotics and live vaccines are very specific to each combination; the present study demonstrated that the specific interaction between an E. faecium probiotic and a S. Enteritidis live vaccine in layers is additive with regard to intestinal Salmonella-specific IgA production.
Disclosure statement
LCB and VBF are associated with DSM Nutritional Products, which produces the tested probiotic and sponsored the study. Imunova, LFC and DM performed the study in collaboration with DSM. There was no interference from DSM or its representatives in the selection of published data.
References
- Adams, C.A. (2010). The probiotic paradox: live and dead cells are biological response modifiers. Nutrition Research Reviews, 23, 37–46. doi: 10.1017/S0954422410000090
- Arumugam, M., Raes, J., Pelletier, E., Le Paslier, D., Yamada, T., Mende, D.R., Fernandes, G.R., Tap, J., Bruls, T., Batto, J.-M., Bertalan, M., Borruel, N., Casellas, F., Fernandez, L., Gautier, L., Hansen, T., Hattori, M., Hayashi, T., Kleerebezem, M., Kurokawa, K., Leclerc, M., Levenez, F., Manichanh, C., Nielsen, H.B., Nielsen, T., Pons, N., Poulain, J., Qin, J., Sicheritz-Ponten, T., Tims, S., Torrents, D., Ugarte, E., Zoetendal, E.G. Wang, J., Guarner, F., Pedersen, O., de Vos, W.M., Brunak, S., Doré, J., Antolín, M., Artiguenave, F., Blottiere, H.M., Almeida, M., Brechot, C., Cara, C., Chervaux, C., Cultrone, A., Delorme, C., Denariaz, G., Dervyn, R., Foerstner, K.U., Friss, C., van de Guchte, M., Guedon, E., Haimet, F., Huber, W., van Hylckama-Vlieg, J., Jamet, A., Juste, C., Kaci, G., Knol, J., Lakhdari, O., Layec, S., Le Roux, K., Maguin, E., Mérieux, A., Melo Minardi, R., M’rini, C., Muller, J., Oozeer, R., Parkhill, J., Renault, P., Rescigno, M., Sanchez, N., Sunagawa, S., Torrejon, A., Turner, K., Vandemeulebrouck, G., Varela, E., Winogradsky, Y., Zeller, G., Weissenbach, J., Ehrlich, S.D. & Bork, P. (2011). Enterotypes of the human gut microbiome. Nature, 473, 174–180. doi: 10.1038/nature09944
- Brisbin, J.T., Gong, J., Orouji, S., Esufali, J., Mallick, A.I., Parvizi, P., Shewen, P.E. & Sharif, S. (2011). Oral treatment of chickens with lactobacilli influences elicitation of immune responses. Clinical and Vaccine Immunology, 18, 1447–1455. doi: 10.1128/CVI.05100-11
- Caporaso, J.G., Kuczynski, J., Stombaugh, J., Bittinger, K., Bushman, F.D., Costello, E.K., Fierer, N., Peña, A.G., Goodrich, J.K., Gordon, J.I., Huttley, G.A., Kelley, S.T., Knights, D., Koenig, J.E., Ley, R.E., Lozupone, C.A., McDonald, D., Muegge, B.D., Pirrung, M., Reeder, J., Sevinsky, J.R., Turnbaugh, P.J., Walters, W.A., Widmann, J., Yatsunenko, T., Zaneveld, J. & Knight, R. (2010). QIIME allows analysis of high-throughput community sequencing data. Nature Methods, 7, 335–336. doi: 10.1038/nmeth.f.303
- Caporaso, J.G., Lauber, C.L., Walters, W.A., Berg-Lyons, D., Lozupone, C.A., Turnbaugh, P.J., Fierer, N. & Knight, R. (2011). Global patterns of 16S rRNA diversity at a depth of millions of sequences per sample. Proceedings of the National Academy of Sciences, 108, 4516–4522. doi: 10.1073/pnas.1000080107
- Capozzo, A.V.E., Cuberos, L., Levine, M.M. & Pasetti, M.F. (2004). Mucosally delivered Salmonella live vector vaccines elicit potent immune responses against a foreign antigen in neonatal mice born to naive and immune mothers. Infection and Immunity, 72, 4637–4646. doi: 10.1128/IAI.72.8.4637-4646.2004
- Carvajal, B.G., Methner, U., Pieper, J. & Berndt, A. (2008). Effects of Salmonella Enterica serovar Enteritidis on cellular recruitment and cytokine gene expression in caecum of vaccinated chickens. Vaccine, 26, 5423–5433. doi: 10.1016/j.vaccine.2008.07.088
- Cihak, J., Hoffmann-Fezer, G., Ziegler-Heibrock, H.W., Stein, H., Kaspers, B., Chen, C.H., Cooper, M.D. & Losch, U. (1991). T cells expressing the V beta 1 T-cell receptor are required for IgA production in the chicken. Proceedings of the National Academy of Sciences, 88, 10951–10955.
- Collard, J.M., Bertrand, S., Dierick, K., Godard, C., Wildemauwe, C., Vermeersch, K., Duculot, J., Van Immerseel, F., Pasmans, F., Imberechts, H. & Quinet, C. (2008). Drastic decrease of Salmonella Enteritidis isolated from humans in Belgium in 2005, shift in phage types and influence on foodborne outbreaks. Epidemiology and Infection, 136, 771–781. doi: 10.1017/S095026880700920X
- Dal Bérto, L., Beirão, B.C.B., Fernandes Filho, T., Ingberman, M., Fávaro Jr, C., Tavella, R., de Mesquita Silva, R.B. & Caron, L.F. (2015). Live and inactivated Salmonella Enteritidis vaccines: immune mechanisms in broiler breeders. World Journal of Vaccines, 5, 155–164. doi: 10.4236/wjv.2015.54018
- de Vrese, M., Rautenberg, P., Laue, C., Koopmans, M., Herremans, T. & Schrezenmeir, J. (2005). Probiotic bacteria stimulate virus–specific neutralizing antibodies following a booster polio vaccination. European Journal of Nutrition, 44, 406–413. doi: 10.1007/s00394-004-0541-8
- Dórea, F.C., Cole, D.J., Hofacre, C., Zamperini, K., Mathis, D., Doyle, M.P., Lee, M.D. & Maurer, J.J. (2010). Effect of salmonella vaccination of breeder chickens on contamination of broiler chicken carcasses in integrated poultry operations. Applied and Environmental Microbiology, 76, 7820–7825. doi: 10.1128/AEM.01320-10
- Jawale, C.V & Lee, J.H. (2014). An immunogenic Salmonella ghost confers protection against internal organ colonization and egg contamination. Veterinary Immunology and Immunopathology, 162, 41–50. doi: 10.1016/j.vetimm.2014.08.015
- Kabir, S.M. (2009). The role of probiotics in the poultry industry. International Journal of Molecular Sciences, 10, 3531–3546. doi: 10.3390/ijms10083531
- Kuritza, L.N., Lourenço, M.C., Miglino, L., Pickler, L., Kraieski, A.L. & Santin, E. (2013). Effects of Enterococcus faecium on diet in the dynamics of CD4+ and CD8+ cell infiltration in the intestinal mucosa of broilers challenged with Salmonella Minnesota. International Journal of Poultry Science, 12, 523–528. doi: 10.3923/ijps.2013.523.528
- Lahtinen, S.J. (2012). Probiotic viability – does it matter? Microbial Ecology in Health and Disease, 23, 18567. doi: 10.3402/mehd.v23i0.18567
- Lauková, A., Kandričáková, A. & Ščerbová, J. (2015). Use of bacteriocin-producing, probiotic strain Enterococcus faecium AL41 to control intestinal microbiota in farm ostriches. Letters in Applied Microbiology, 60, 531–535. doi: 10.1111/lam.12409
- Liu, W., Yang, Y., Chung, N. & Kwang, J. (2001). Induction of humoral immune response and protective immunity in chickens against Salmonella Enteritidis after a single dose of killed bacterium-loaded microspheres. Avian Diseases, 45, 797–806. doi: 10.2307/1592859
- Macpherson, A.J., Geuking, M.B., Slack, E., Hapfelmeier, S. & McCoy, K.D. (2012). The habitat, double life, citizenship, and forgetfulness of IgA. Immunological Reviews, 245, 132–146. doi: 10.1111/j.1600-065X.2011.01072.x
- Mirpuri, J., Raetz, M., Sturge, C.R., Wilhelm, C.L., Benson, A., Savani, R.C., Hooper, L.V & Yarovinsky, F. (2014). Proteobacteria-specific IgA regulates maturation of the intestinal microbiota. Gut Microbes, 5, 28–39. doi: 10.4161/gmic.26489
- Naseem, S., Rahman, S.U., Shafee, M., Sheikh, A.A. & Khan, A. (2012). Immunomodulatory and growth-promoting effect of a probiotic supplemented in the feed of broiler chicks vaccinated against infectious bursal disease. Revista Brasileira de Ciência Avícola, 14, 109–113. doi: 10.1590/S1516-635X2012000200004
- Nguyen, Q.N., Himes, J.E., Martinez, D.R., Permar, S.R. & Hogan, D.A. (2016). The impact of the gut microbiota on humoral immunity to pathogens and vaccination in early infancy. PLoS Pathogens, 12, e1005997. doi: 10.1371/journal.ppat.1005997
- Oakley, B.B., Lillehoj, H.S., Kogut, M.H., Kim, W.K., Maurer, J.J., Pedroso, A., Lee, M.D., Collett, S.R., Johnson, T.J. & Cox, N.A. (2014). The chicken gastrointestinal microbiome. FEMS Microbiology Letters, 360, 100–112. doi: 10.1111/1574-6968.12608
- Phillips, I., Casewell, M., Cox, T., De Groot, B., Friis, C., Jones, R., Nightingale, C., Preston, R. & Waddell, J. (2004). Does the use of antibiotics in food animals pose a risk to human health? A critical review of published data. Journal of Antimicrobial Chemotherapy, 53, 28–52. doi: 10.1093/jac/dkg483
- Praharaj, I., John, S.M., Bandyopadhyay, R. & Kang, G. (2015). Probiotics, antibiotics and the immune responses to vaccines. Philosophical Transactions of the Royal Society B: Biological Sciences, 370, 20140144. doi: 10.1098/rstb.2014.0144
- Rostagno, H.S., Albino, L.F.T., Donzele, J.L., Gomes, P.C., de Oliveira, R.F., Lopes, D.C., Ferreira, A.S. & de Toledo Barreto, S.L. (2005). Tabelas brasileiras para aves e suínos: composição de alimentos e exigências nutricionais. H.S. Rostagno (Ed.), 2nd ed. Viçosa: UFV.
- Salk, H.M., Simon, W.L., Lambert, N.D., Kennedy, R.B., Grill, D.E., Kabat, B.F., Poland, G.A. & Huber, V.C. (2016). Taxa of the nasal microbiome are associated with influenza-specific IgA response to live attenuated influenza vaccine. PloS One, 11, e0162803. doi: 10.1371/journal.pone.0162803
- Santos, F.B.O., Li, X., Payne, J.B. & Sheldon, B.W. (2005). Estimation of most probable number Salmonella populations on commercial North Carolina turkey farms. The Journal of Applied Poultry Research, 14, 700–708. doi: 10.1093/japr/14.4.700
- Stanley, D., Hughes, R.J. & Moore, R.J. (2014). Microbiota of the chicken gastrointestinal tract: influence on health, productivity and disease. Applied Microbiology and Biotechnology, 98, 4301–4310. doi: 10.1007/s00253-014-5646-2
- Tran, T.Q.L., Quessy, S., Letellier, A., Desrosiers, A. & Boulianne, M. (2010). Immune response following vaccination against Salmonella Enteritidis using 2 commercial bacterins in laying hens. Canadian Journal of Veterinary Research, 74, 185–192.
- Valdez, Y., Brown, E.M. & Finlay, B.B. (2014). Influence of the microbiota on vaccine effectiveness. Trends in Immunology, 35, 526–537. doi: 10.1016/j.it.2014.07.003
- Vintini, E., Villena, J., Alvarez, S. & Medina, M. (2010). Administration of a probiotic associated with nasal vaccination with inactivated Lactococcus lactis-PppA induces effective protection against pneumoccocal infection in young mice. Clinical & Experimental Immunology, 159, 351–362. doi: 10.1111/j.1365-2249.2009.04056.x
- Yilmaz, P., Parfrey, L.W., Yarza, P., Gerken, J., Pruesse, E., Quast, C., Schweer, T., Peplies, J., Ludwig, W. & Glöckner, F.O. (2013). The SILVA and “all-species living tree project (LTP)” taxonomic frameworks. Nucleic Acids Research, 42, D643–D648.
- Zhang, W., Azevedo, M.S.P., Wen, K., Gonzalez, A., Saif, L.J., Li, G., Yousef, A.E. & Yuan, L. (2008). Probiotic Lactobacillus acidophilus enhances the immunogenicity of an oral rotavirus vaccine in gnotobiotic pigs. Vaccine, 26, 3655–3661. doi: 10.1016/j.vaccine.2008.04.070