ABSTRACT
Spinosad (SPN) and abamectin (ABM) are used in poultry premises to control external parasites including red mites (Dermanyssus gallinae). This study aimed to determine levels of SPN (spinosyn A + spinosyn D) and ABM residues in egg and edible tissues of laying hens following spray application. A total of 36 laying hens were divided into four groups of nine birds each, and they were kept in individual cages. Two different concentrations of SPN (2 and 4 g/l) and ABM (0.025 and 0.033 g/l) were applied in stocked and empty cages, respectively. Eggs were collected individually for 30 days. All hens were sacrificed at day 30 post–treatment, and tissue samples (liver, breast muscle, fat and skin) were collected. The residue levels in eggs and tissues were determined by high pressure liquid chromatography. ABM residues were not detectable in egg samples. SPN residues in eggs and residues of both ABM and SPN in liver, muscle and fat were under the maximum residue limits (MRLs) following low and high concentration applications. However, although the MRLs have not been established for SPN and ABM in skin tissue of chicken, residues in the skin detected at the low and high concentrations were greater than the MRLs for other edible tissues (except fat tissue) indicating that a withdrawal period would be necessary for the skin tissue after ABM and SPN use in laying hens.
Introduction
Abamectin (ABM) and spinosad (SPN; a mixture of two active compounds, spinosyn A and D) are naturally derived macrocyclic lactones produced by the soil-dwelling actinomycetes, Streptomyces avermitilis and Saccharopolyspora spinosa, respectively. Both chemicals are highly effective against many types of insects with very low mammalian toxicity and, therefore, have been used extensively to control ectoparasites in domestic animals (McKellar & Gokbulut, Citation2012; Schnitzler et al., Citation2012) and as insecticides for crop production (Dybas, Citation1989; Hertlein et al., Citation2011).
Dermanyssus gallinae, the poultry red mite (PRM), is one of the most economically important ectoparasites in laying hens in many countries worldwide (Tomley & Sparagano, Citation2018). It is a haematophagous ectoparasite and is capable of transmitting several infectious agents, some of which are zoonotic. PRM is also responsible for remarkable economic losses which affect the productivity of the egg industry (Flochlay-Sigognault et al., Citation2017). One of the widely applied control strategies for the PRM is the use of acaricides including chemical products. There is only a limited number of licensed products available in Europe, and various non-specifically approved (or banned) products are still widely used (Sparagano et al., Citation2014). However, a very recent case in several European countries has shown that the impact of such uncontrolled use of acaricides (“fipronil-crisis”) may have great consequences (European Commission Health and Food Safety Directorate, Citation2017).
Besides their antiparasitic activities, products used for the treatment of PRM must be in accordance with regulatory requirements for consumer health. With regard to this, EU legislation regulates the detection of acaricides in poultry tissues and eggs, and it identifies maximum residue limits (MRLs) for each substance (EFSA, Citation2011, Citation2014). In this manner, MRLs of several acaricides (e.g. propoxur, phoxim, carbaryl, permethrin) were investigated in eggs and/or tissues in previous studies (Hamscher et al., Citation2003, Citation2007; Marangi et al., Citation2012). SPN and ABM are also used against PRM and are available as formulations for spray application in some European countries. Regarding the residues of these products in poultry, there is only one feeding study with SPN in laying hens (Rutherford et al., Citation2000). However, there is no information in the literature on the residues of these two active ingredients in eggs and edible tissues of laying hens following spray application. Therefore, the objective of this research was to investigate the residue levels of SPN and ABM in eggs and edible tissues (liver, fat, muscle and skin) of laying hens following spray application.
Materials and methods
Experimental animals
Thirty-six laying hens (White Commercial Laying hens – Nick Chick, Has Tavuk, Bursa, Turkey), 12 months old and weighing 1.67 kg (SD 0.16 kg) were used in this study. The hens were kept in individual cages (40 × 32 × 41 cm, height × width × depth) and were provided with ad libitum water and commercial layer feed (Eris Yem, Istanbul, Turkey) consisting of corn, wheat, soybean meal, limestone, sunflower meal, gluten, chicken flour, hydrolyzed soybean oil, dicalcium phosphate, salt, vitamin and mineral premix, lysine and methionine. The birds were observed for abnormal behaviours for two weeks before and throughout the study period. The hens were not fasted before acaricide applications. Animal procedures and management protocols were carried out in accordance with the Animal Welfare Policy of the Faculty of Veterinary Medicine, and approved by the Animal Ethic Committee of Uludag University, Bursa, Turkey (17.03.2015; No: 2015-04/02). The whole trial was carried out according to local and international regulations on animal welfare.
Treatments and sampling
The hens were allocated into four groups of nine birds such that the mean weight of birds in each group was similar. Two different concentrations of SPN (Group 1-low: 2 g/l, Group 2-high: 4 g/l) and ABM (Group 3-low: 0.025 g/l, Group 4-high: 0.033 g/l) were used according to appropriate literature and product information, respectively (Liebisch et al., Citation2011; GAT Omega®, GAT Microencapsulation AG, Ebenfurth, Austria). SPN (Elector®, 480 g/l, Elanco Animal Health, Bad Homburg, Germany) and ABM (Alopec EC®, 18 g/l, Astranova, Antalya, Turkey) were applied in stocked and empty cages, respectively, to the floor, side and ceiling wires, to all folds and connection points and to the egg canals of the cages as a coarse spray using a standard 1000 ml hand-operated atomizer. A total of 223 ml acaricide dilution (diluted in distilled water) per group (i.e. 24.8 ml per bird/cage) was used. In the ABM groups (Groups 3 and 4), the hens were placed in cages 30 min after the application. The eggs were collected daily for 30 days individually. The yolk and white (albumen) were separated and each yolk was placed in a plastic bag for analysis. All birds were euthanized at day 30 after spraying, and blood and tissue samples (liver, breast muscle, fat and skin) were collected from each hen. All samples were kept at −20°C until analysis.
Analytical procedure
Stock solutions (100 μg/ml) of analytic standard of SPN (Spinosyn A + D, purity 97.6%, PESTANAL, Sigma-Aldrich – CAS Number: 168316-95-8) and ABM (purity 98,6%, PESTANAL, Sigma-Aldrich – CAS Number: 71751-41-2) were prepared using acetonitrile (Sigma-Aldrich, St. Louis, MO, USA) as the solvent. These were diluted with acetonitrile to give solutions 0.1, 0.5, 1, 5, 10, 25 and 50 μg/ml for SPN and 1, 2.5, 5, 10, 100, 250 and 500 ng/ml for ABM for calibration of standard curves and to add to drug-free egg, tissue and plasma samples to determine the recoveries.
The egg, tissue and plasma concentrations of ABM were analyzed by High Performance Liquid Chromatography (HPLC) with fluorescence detector following solid phase extraction (SPE) procedure according to Cirak et al. (Citation2018). SPN (spinosyn A and D) were also analyzed by HPLC with photodiode array (PDA) detector following an SPE procedure similar to the method used to analyze ABM with small modifications, as described below. Previous studies indicated that macrocyclic lactones, including ivermectin, were not detected in egg white (Keukens et al., Citation2000; Moreno et al., Citation2015; Cirak et al., Citation2018) after analysis of egg yolk and white separately. For this reason, only egg yolks were used for HPLC analysis. The egg yolk and tissue samples were homogenized using a homogenizer (IKA, T18 Digital Ultra-Turrax, Staufen, Germany) before extraction. Briefly, 2 g homogenized drug-free yolk, tissue or 2 ml drug-free plasma samples were diluted with 2 ml distilled water, and then spiked with SPN standards or ABM standards to reach the following final concentrations: 0.0, 0.01, 0.05, 0.10, 0.5, 1.0, 5 μg/g or μg/ml and 0.0, 0.25, 0.5, 1, 10, 25 and 50 ng/g or ng/ml respectively. The spiked and experimental yolk, tissue and plasma samples (2 g or 2 ml) were combined with 50 μl of internal standard (moxidectin, 100 ng/ml) for ABM analysis, and then 7 ml acetonitrile/water (5:2 for ABM and 4:3 for SPN) was added to all samples. After mixing for 5 min, the samples were kept in an incubator (Incubator IN160, Memmert, Germany) at 70°C for 30 min and the solvent-sample mixtures were centrifuged (Rotina 380, Hettich, Newport Pagnell, England) at 5 000 rpm for 10 min following mixing for 5 min. The clear supernatant was transferred to a glass tube and the procedure repeated. The supernatant of each sample was transferred to an SPE cartridge (C18 ec f, 500 mg/6 ml, Chromobond, Macherey-Nagel, Düren, Germany), previously conditioned with 3 ml methanol and 3 ml deionized water, at a flow rate of approximately 6 ml/min. After sample application, the cartridge was washed with 3 ml of water/methanol (1:1 for ABM and 3:1 for SPN) and dried under vacuum for 30 min. The analytes were eluted with 3 ml of methanol and concentrated to dryness at 45°C in a sample concentrator (Maxi-dry plus, Heto Lab. Equipment, Denmark). For ABM samples, the reconstitution was made using 100 µl of a solution of N-methylimidazole in acetonitrile (1:1). Derivatisation was initiated by adding 150 µl trifluoroacetic anhydride solution in acetonitrile (1:2). After completion of the reaction, an aliquot (100 μl) of this solution was injected directly into the chromatograph. For SPN samples, the reconstitution was made using 250 µl of a mobile phase solution and an aliquot (100 μl) of this solution was injected directly into the chromatograph. Because of the photosensitivity of SPN, all preparative processes were conducted in covered containers or amber (brown) glass.
The samples were processed on a computerized gradient HPLC system (Agilent 1260 Series HPLC, Agilent, Waldron, Germany). For SPN analysis, the mobile phase consisted of 2% aqueous ammonium acetate (pH 5) and acetonitrile was delivered (1260 Series binary pump, Agilent, Waldron, Germany) at a flow rate of 1 ml/minute with a linear gradient fashion changing from 0:100 (acetonitrile-acetate buffer) to 100:0 for 15 min. This ratio was maintained for 3 min, then the ratio changed to the initial rate (0:100) and was maintained for 5 min for preparing the next injection. An analytical HPLC column (Agilent, Eclipse Plus C18, 5 µm, 250 mm × 4.6 mm, Agilent Technologies, USA) with nucleosil C18 guard column (Phenomenex, Macclesfield, Cheshire, UK) was used for analysis and kept at 30°C in the column oven. Photodiode array (PDA) detector (1260 Series, Agilent, Waldron, Germany) set at 250 nm for SPN (Spinosyn A and D).
For ABM analysis, the mobile phase consisted of acetonitrile and methanol (60:40, v/v) and was delivered (1260 Series binary pump, Agilent, Waldron, Germany) at a flow rate of 1.2 ml/min. An analytical HPLC column (Luna, 3 µm, 150 mm × 4.6 mm, Phenomenex, Macclesfield, Cheshire, UK) with nucleosil C18 guard column (Phenomenex, Macclesfield, Cheshire, UK) was used for analysis and kept at 40°C in the column oven. Fluorescence detection (1260 Series, Agilent, Waldron, Germany) was at an excitation wavelength of 365 nm and an emission wavelength of 475 nm.
The analytical methods used for SPN and ABM in egg, tissue and plasma samples were validated prior to the start of the experimental sample analysis. The analytes were identified with the retention times of pure reference standard. Recoveries of the molecules under study were measured by comparison of the peak areas from seven spiked egg, tissue and plasma samples and with the areas resulting from direct injections of standard solutions. The inter- and intra-assay precision of the extraction and chromatography procedures were evaluated by processing replicate aliquots of drug-free layer egg, tissue and plasma samples containing known amounts of the drugs on four different days. Calibration graphs for SPN (spinosyn A or D) and ABM were prepared (linear range 0.01–5 μg/g or μg/ml for SPN and 0.25–50 ng/g or ng/ml for ABM). The slope of the lines between peak areas and drug concentration was determined by least squares linear regression and correlation coefficient (r) and coefficient of variations (CV) calculated using Microsoft Excel (Office 365, Microsoft Corporation, Seattle, WA, USA). Linearity was established to determine the ABM or SPN concentration/detector response relationship. Precision and accuracy (intra- and inter-assay) of the method were determined by evaluation of replicates of drug-free samples (n = 7) fortified with SPN or ABM at different concentrations in egg, tissue and plasma samples. The detection limit of the SPN and ABM was established with HPLC analysis of blank egg, tissue and plasma fortified with the analytical standards, measuring the baseline noise at the retention time of the peak. The mean baseline noise at the peak retention time plus three standard deviations was defined as the limit of detection (LOD). The mean baseline noise plus six standard deviations was defined as the limit of quantification (LOQ). The selectivity of the method was determined by comparing the chromatograms of SPN- or ABM-free egg, tissue and plasma samples with those of each sample fortified with SPN or ABM analytical standards.
Statistical analysis
The residue levels were reported as mean ± SD. The differences between groups of SPN or ABM and different tissues were statistically compared by one-way analysis of variance (ANOVA). All statistical analyses were performed by using MINITAB for Windows (release 12.1, Minitab Inc., State College, PA, USA). Mean values were considered significantly different at P < 0.05.
Results
Clinically, no adverse reactions were observed in any of the laying hens treated with SPN or ABM. The analytical procedures and HPLC analysis of SPN and ABM were validated before the analysis of the experimental samples. The validation parameters for SPN (spinosyn A and D) and ABM in egg, tissue and plasma samples are summarized in . Examples of typical chromatograms of SPN in egg yolk and ABM in skin tissue, obtained under the HPLC conditions, are included in and , respectively. As the chromatograms for the other sample matrices were similar, those data are not shown.
Figure 1. Typical chromatograms from the determination of spinosad (SPN; spinosyn A and D) residues in egg yolk using HPLC with a photodiode array: (A) standards (1 μg/ml); (B) control egg yolk containing no detectable residue; (C) control egg yolk fortified with 0.5 μg/g of two analytes; (D) a study sample (10 days post-spraying) containing SPN (spinosyn A and D) residue.
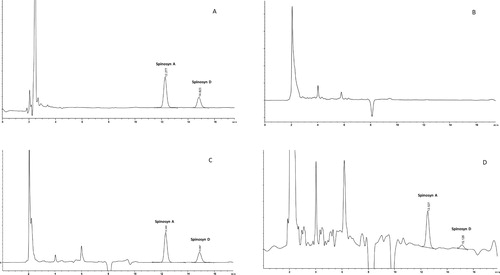
Figure 2. Typical chromatograms from the determination of abamectin (ABM) residues in skin tissue of laying hens using HPLC with a fluorescence detector: (A) standards (5 ng/ml); (B) control skin tissue containing internal standard (moxidectin) and no detectable residues of ABM; (C) control skin tissue fortified with 5 ng/g of ABM with IS; (D) a skin tissue from the study samples (30 days post-spraying) containing ABM residue (29.23 ng/g) with IS.
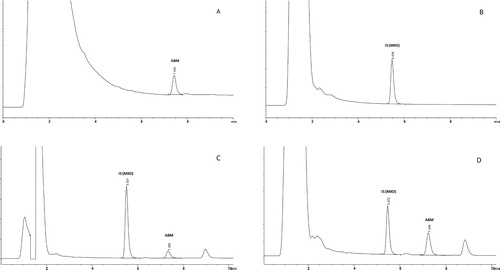
Table 1. Validation parameters of analytical method used for determination of levels of abamectin (ABM) and spinosad (SPN; spinosyn A and spinosyn D) residues in egg, tissue and plasma samples.
SPN residues in egg yolks were initially detected on the second day and were observed for 20 and 30 days after low and high concentration treatments, respectively (). SPN residues (spinosyn A + D) were higher than LOQ (0.01 μg/g) in all egg yolk () and tissue samples () except muscle samples following low concentration application. However, plasma SPN residues higher than LOQ were obtained only after high concentration treatment. Mean residue levels (µg/g) of spinosyn A and spinosyn D in egg yolk are shown in and , respectively. Spinosyn D was only detected following high concentration application. The highest SPN (spinosyn A + D) residues in the egg yolk were 0.09 ± 0.05 μg/g and 0.39 ± 0.09 μg/g observed 7 days post-treatment with SPN at low and high concentrations, respectively. In contrast to SPN, ABM residues were not detectable in eggs and plasma samples following application of both concentrations.
Figure 3. Mean spinosyn A residues (μg/g or mg/kg) in egg yolk following application of spinosad at two different concentrations (2 g/l or 4 g/l).
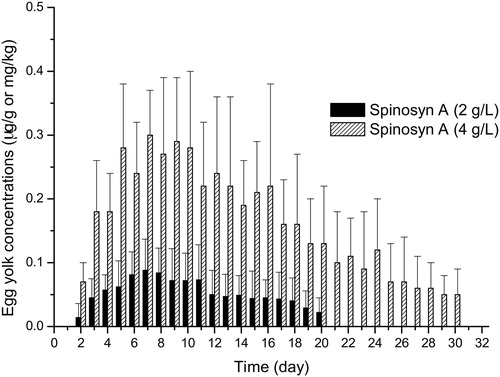
Figure 4. Mean spinosyn D residues (μg/g or mg/kg) in egg yolk following application of spinosad at the concentration of 4 g/l.
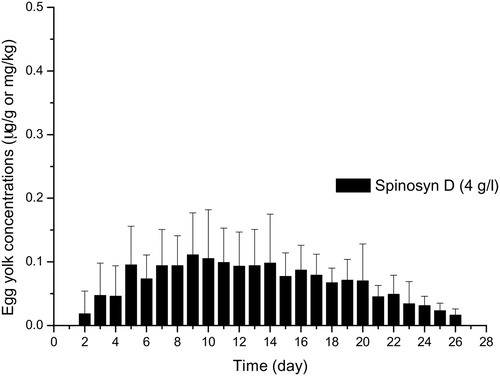
Table 2. Mean (± SD) tissue and plasma residues of spinosad (SPN) (μg/g or μg/ml) and abamectin (ABM) (ng/g or ng/ml) quantified on day 30 after spray application at two different concentrations to laying hens.
Mean residue levels of SPN (spinosyn A + D) (μg/g or mg/kg) and ABM (ng/g) in various tissues and plasma samples (μg/ml) are shown in . SPN residues in skin (0.15 and 0.45 μg/g) and fat (0.16 and 0.45 μg/g) were dramatically higher than those observed in the liver (0.02 and 0.04 μg/g) and muscle (not detected and 0.02 μg/g) following low and high concentration treatments, respectively. Similar to SPN, residues of ABM in skin samples (11.12 and 25.13 ng/g) observed after both treatment concentrations were also significantly higher when compared with other tissues (fat, liver and muscle) ().
Discussion
SPN and ABM are acaricides which have been labelled for use in poultry houses to control external parasites including red mites (Dermanyssus gallinae). However, there is a lack of data available in the literature on their residues in eggs and edible tissues in poultry. In the present study, SPN and ABM residues in eggs, edible tissues and plasma were reported in laying hens following spray application at two different concentrations.
Many drugs used in the treatment of laying hens accumulate preferentially in the white or yolk of eggs, depending on their physicochemical properties such as hydrophilicity or hydrophobicity, the binding capacity to plasma proteins, and the ability to move through different tissue types that affect the distribution of residues (Martinez, Citation1998). For example, due to the high lipophilicity of macrocyclic lactones, they can accumulate in fat, where they can persist for prolonged periods (McKellar & Gokbulut, Citation2012).
SPN consists of a naturally occurring mixture of two active components (spinosyn A and D). The octanol/water partition coefficient (log P) values of spinosyn A are 2.8 at pH 5.0 and 5.2 at pH 9.0; and the log P for spinosyn D ranges from 3.2 to 5.2 (Thompson et al., Citation1995). The log P of ABM is 4.4 at pH 7.0 (Solecki & Niemann, Citation2012). Because of these log P values, it was expected that ABM and SPN residues would tend to be highest in fat. The residues of ivermectin (IVM) and other macrocyclic lactones preferentially accumulate in the egg yolk following administration to laying hens (Keukens et al., Citation2000). Accordingly, it was reported that no IVM residues were found in egg white, which contains fat at trace levels, whereas significant IVM residues were measured in egg yolk, which is very rich in lipids, for 5 and 15 days following administration in water for 5 days (Moreno et al., Citation2015) and single oral administration (Cirak et al., Citation2018) of IVM, respectively, to laying hens.
European Food Safety Authority (EFSA) has set MRLs of ABM and SPN as 0.01 mg/kg (10 ng/g) (EFSA, Citation2014) and 0.2 mg/kg (0.2 μg/g) (EFSA, Citation2011) respectively, for eggs and edible tissues [(meat and liver) (MRL of SPN for kidney: 0.02 mg/kg and fat: 1.0 mg/kg) (EUR-Lex, Citation2015)] of chicken. In the present investigation, ABM residues were not detected in egg samples for 30 days following spray application at two different concentrations suggesting that ABM could be applied safely to layer houses without any withdrawal period for eggs. While the highest SPN (spinosyn A + D) residues in egg yolk never exceeded the MRL of 0.2 μg/g after application of low concentration (2 g/l), the highest level of SPN residues was 0.393 μg/g at 7 days of high dose application (4 g/l) and exceeded the MRL until day 18 post-treatment. However, as the yolk/white portion of an egg makes up about 1/3 of the liquid weight of the egg, the actual residue level of total SPN falls below this limit by diluting the egg white when considering a whole egg (yolk + white).
Rutherford et al. (Citation2000) investigated SPN residues in eggs and tissues of laying hens following per os administration for 42 days. They found that the residue levels in egg samples were usually below the MRL but reached a plateau at 0.227 μg/g after 13 days of dosing. SPN residues also occurred in various tissues such as liver, abdominal and subcutaneous fat, light and dark meat, with the highest amounts in fat samples (Rutherford et al., Citation2000). Our findings are generally in agreement with those obtained in the previous study, although the application method of SPN was different. In the current study, SPN residues occurred in almost all sample types but were highest (nearly ten times) in the skin and fat tissues and lowest in the muscle, liver and plasma at day 30 post-treatment at both concentrations. Thus, the residue levels of SPN in the skin tissue (0.45 μg/g), observed after application of high dose concentration, exceeded the MRL of SPN (0.2 μg/g) in respect of the MRL for other edible tissues in chicken except fat tissue (MRL: 1.0 mg/kg).
ABM residues in muscle, liver and fat, after both low and high concentration applications, were under the MRL of 10 ng/g. However, like SPN, significantly higher ABM residues (almost 50 times) were quantified in the skin (but not in the fat samples) than in the other tissues after low- and high- dose treatments. Although the MRLs have not been established for ABM and SPN in skin tissue of chicken, the residue levels in the skin quantified in the present study, at low and high concentrations of ABM and at high concentration of SPN, exceeded the MRLs of ABM and SPN in respect of the MRL values for other edible tissues except the fat tissue. The reasons for this accumulation pattern of SPN and ABM in the skin are not clear. In fact, it is also not clear how the uptake of the applied acaricides by the hens could take place in the present study. As described previously, ABM was applied to empty cages with no hens inside, whereas SPN was sprayed to cages in the presence of hens, as recommended by the manufacturer. As water and feed reservoirs were removed before the applications, an oral intake seems less likely for both products, which, however, doesn't exclude a possible uptake of the drugs by pecking the wires of the sprayed cages. In the case of SPN treatment, the transcutaneous and/or inhalation route could be a possible explanation for the uptake of this compound, even though the product has not been applied directly to the hens. Nevertheless, the birds inside the cages during spraying were apparently exposed to the active compound. Furthermore, during the post-treatment period, the direct contact of the abdominal regions of hens with the cage wires treated with the respective acaricide might have contributed to the skin residues since the skin samples were collected from the abdominal area of each animal. When mist-sprayed on hens, peak equivalents of permethrin, a pyrethroid insecticide, were found in the skin (Hunt et al., Citation1979). Accordingly, it was noted that pyrethroid residues in skin are more persistent than the residues in the egg yolk (Goetting et al., Citation2011). Marangi et al. (Citation2012) investigated various organs of 45 laying hens, originating from three farms in southern Italy, for carbaryl (organophosphate) and permethrin residues. On one farm, carbaryl residues were highest in muscle and especially in skin samples exceeding the MRL markedly. In this context, the skin of hens seems to be an appropriate target tissue for accumulating of insecticides. Therefore, it is likely that ABM and SPN have similar mechanisms of accumulation in the hen’s tissue to other insecticides. However, this needs more detailed investigation.
In conclusion, in eggs, ABM residues were not detectable, and SPN residues were under the MRL following spray application of either compound. However, residues measured in the skin were greater than in the other edible tissues of hens, except fat tissue, indicating that a “withdrawal period” could be necessary for the skin tissue after ABM and SPN use in laying hens. Furthermore, it may be more useful to determine MRLs of residues in egg yolk and white separately because the chemicals can accumulate preferentially in the white or yolk of eggs, depending on their physicochemical properties.
Acknowledgements
The authors wish to thank EU COST for its support of the COREMI project (Action FA1404) (http://www.coremi.eu/home.html).
Disclosure statement
No potential conflict of interest was reported by the authors.
ORCID
Cengiz Gokbulut http://orcid.org/0000-0002-4912-7307
Veli Yilgor Cirak http://orcid.org/0000-0003-0570-2514
Additional information
Funding
References
- Cirak, V.Y., Aksit, D., Cihan, H. & Gokbulut, C. (2018). Plasma dispositions and concentrations of ivermectin in eggs following treatment of laying hens. New Zealand Veterinary Journal, 66, 121–125. doi: 10.1080/00480169.2018.1426504
- Dybas, R.A. (1989). Abamectin use in crop protection. In W.C. Campbell ( 1989), Ivermectin and Abamectin 1st edn (pp. 287–310). New York: Springer.
- EFSA. (2011). Modification of the existing MRLs for spinosad in various crops. EFSA Journal, 9, 2352.
- EFSA. (2014). Reasoned opinion on the review of the existing maximum residue levels (MRLs) for abamectin according to Article 12 of Regulation (EC) No 396/2005. EFSA Journal, 12, 3823.
- EUR-Lex. (2015). Commission Regulation (EU) 2015/603. Amending Annexes II, III and V to Regulation (EC) No 396/2005 of the European Parliament and of the Council as regards maximum residue levels for 2-naphthyloxyacetic acid, acetochlor, chloropicrin, diflufenican, flurprimidol, flutolanil and spinosad in or on certain products. Official Journal of the European Union.
- European Commission Health and Food Safety Directorate. (2017). Summary Report of the Standing Committee on Plants, Animals, Food and Feed.
- Flochlay, A., Thomas, E. & Sparagano, O. (2017). Poultry red mite (Dermanyssus gallinae) infestation: a broad impact parasitological disease that still remains a significant challenge for the egg-laying industry in Europe. Parasites & Vectors, 10, 457. doi: 10.1186/s13071-017-2390-3
- Goetting, V., Lee, K.A. & Tell, L.A. (2011). Pharmacokinetics of veterinary drugs in laying hens and residues in eggs: a review of the literature. Journal of Veterinary Pharmacology and Therapeutics, 34, 521–556. doi: 10.1111/j.1365-2885.2011.01287.x
- Hamscher, G., Priess, B., Hartung, J., Nogossek, M.I., Glünder, G. & Nau, H. (2003). Determination of propoxur residues in eggs by liquid chromatography–diode array detection after treatment of stocked housing facilities for the poultry red mite (Dermanyssus gallinae). Analytica Chimica Acta, 483, 19–26. doi: 10.1016/S0003-2670(02)01022-X
- Hamscher, G., Priess, B. & Nau, H. (2007). Determination of phoxim residues in eggs by using high-performance liquid chromatography diode array detection after treatment of stocked housing facilities for the poultry red mite (Dermanyssus gallinae). Analytica Chimica Acta, 586, 330–335. doi: 10.1016/j.aca.2006.09.041
- Hertlein, M.B., Thompson, G.D., Subramanyam, B. & Athanassiou, C.G. (2011). Spinosad: a new natural product for stored grain protection. Journal of Stored Products Research, 47, 131–146. doi: 10.1016/j.jspr.2011.01.004
- Hunt, L.M., Gilbert, B.N. & Lemeilleur, C.A. (1979). Distribution and depletion of radioactivity in hens treated dermally with C-14-labeled permethrin. Poultry Science, 58, 1197–1201. doi: 10.3382/ps.0581197
- Keukens, H.J., Kan, C.A., van Rhijn, J.A. & van Dijk, J. (2000). Ivermectin residues in eggs of laying hens and in muscle and liver of broilers after administration of feeds containing low levels of ivermectin. In L.A. van Ginkel & A. Ruiter (Eds.). Proceedings of the EuroResidue IV Conference (pp. 678–682). Veldhoven, the Netherlands.
- Liebisch, G., Hack, R. & Smid, G. (2011). Efficacy of spinosad against the poultry red mite, Dermanyssus gallinae (Mesostigmata: Dermanyssidae), in laboratory and field trials. Zoosymposia, 6, 282–287.
- Marangi, M., Morelli, V., Pati, S., Camarda, A., Cafiero, M.A. & Giangaspero, A. (2012). Acaricide residues in laying hens naturally infested by red mite Dermanyssus gallinae. PLoS One, 7, e31795. doi: 10.1371/journal.pone.0031795
- Martinez, M.N. (1998). Use of pharmacokinetics in veterinary medicine – article III: physicochemical properties of pharmaceuticals. Journal of the American Veterinary Medical Association, 213, 1274–1277.
- McKellar, Q.A. & Gokbulut, C. (2012). Pharmacokinetic features of the antiparasitic macrocyclic lactones. Current Pharmaceutical Biotechnology, 13, 888–911. doi: 10.2174/138920112800399194
- Moreno, L., Dominguez, P., Farias, C., Canton, L., Virkel, G., Mate, L., Ceballos, L., Lanusse, C. & Alvarez, L. (2015). Integrated assessment of ivermectin kinetics, metabolism and tissue residues in laying hens. Journal of Veterinary Pharmacology and Therapeutics, 38, 100–101.
- Rutherford, B.S., Gardner, R.C., West, S.D., Robb, C.K. & Dolder, S.C. (2000). Residues of spinosad in meat, milk, and eggs. Journal of Agricultural and Food Chemistry, 48, 4428–4431. doi: 10.1021/jf0004457
- Schnitzler, B., Hayes, B., Wiseman, S. & Snyder, D.E. (2012). Confirmation of the efficacy of a combination tablet of spinosad and milbemycin oxime against naturally acquired infections of canine intestinal nematode parasites. Veterinary Parasitology, 184, 279–283. doi: 10.1016/j.vetpar.2011.11.012
- Solecki, R. & Niemann, L. (2012). Insecticides of natural origin, other than pyrethrum and nicotine. In T.C. Marrs ( 2012), Mammalian Toxicology of Insecticides (1st ed., pp. 254–287). Cambridge: RSC Publishing.
- Sparagano, O.A., George, D.R., Harrington, D.W. & Giangaspero, A. (2014). Significance and control of the poultry red mite, Dermanyssus gallinae. Annual Review of Entomology, 59, 447–466. doi: 10.1146/annurev-ento-011613-162101
- Thompson, G.D., Busacca, J.D., Jantz, O.K., Borth, P.W., Nolting, S.P., Winkle, J.R., Gantz, R.L., Huckaba, R.M., Nead, B.A., Peterson, L.G., Porteous, D.J. & Richardson, J.M. (1995). Field performance in cotton of spinosad: a new naturally derived insect control system. Proceedings of the Beltwide Cotton Conferences (pp 907–910). San Antonio, USA.
- Tomley, F.M. & Sparagano, O. (2018). Spotlight on avian pathology: red mite, a serious emergent problem in layer hens. Avian Pathology, 47, 533–535. doi: 10.1080/03079457.2018.1490493