Abstract
In order to understand the effect of a nitrogen gradient on the intraspecific and interspecific competition between plants, the two species Erodium cicutarium and Geranium pusillum were grown in a response-surface competion experiment at three different densities along a nitrogen gradient consisting of four different nitrogen levels. Seed set data were estimated from biomass measurements and fitted to a generalized hyperbolic competition model, and the probabilities of different ecological scenarios were calculated. The model predicted that E. cicutarium is competitively superior along most of the nitrogen gradient. This prediction was contrary to our prior expectations which were based on the occurance of the two species in natural habitats.
Introduction
Nitrogen is a constituent of fundamental plant cell components and it is often the resource which is limiting primary production in terrestrial ecosystems (Vitousek & Howarth Citation1991, Aerts & Chapin III Citation2000) and, consequently, it is a resource for which plants often compete. Examples of changes in the plant community composition as a consequence of a change in the nitrogen availability have been reported frequently (Ejrnæs et al. Citation2002, Rickey & Anderson Citation2004, Stevens et al. Citation2004, Tomassen et al. Citation2004).
A nitrogen gradient is often characterized by an increase in plant productivity from low to high nitrogen levels, thus a change in species composition from stress tolerant species to more competitive species is expected. Generally, stress-tolerant plants adapted to nutrient poor habitats have slow growth and invest in sclerophyll and persistent leaves with long life span and in a root system which is capable of obtaining the required nutrients even when these are in short supply. On the other hand, competitive plants adapted to nutrient rich habitats are fast growing and invest in above-ground biomass and photosynthetic tissue in order to compete efficiently for light (Lambers & Poorter Citation1992).
Nitrogen is considered a key factor for plant growth and generally, competition for soil nitrogen is assumed to be size-asymmetric among individuals (Hikosaka & Hirose Citation2001). In Citation1987, Berendse and Aerts defined the nitrogen use efficiency (NUE, g g−1 N) as the product of the nitrogen productivity (NP, g g−1 N yr−1) and the mean residence time (yr), and Poorter et al. (Citation1990) expressed the relative growth rate (RGR, mg g−1 day−1) as the product of the plant's nitrogen productivity and the plant's nitrogen concentration (PNC, mol g−1). Hikosaka and Hirose (Citation2001) showed that larger individuals had a longer mean retention time for nitrogen and a higher nitrogen productivity resulting in larger nitrogen use efficiency compared to smaller individuals. Hikosaka (Citation2004) calls attention to the fact that species with a high photosyntetic nitrogen efficiency (PNUE, the rate of photosynthesis per unit leaf nitrogen) tend to have a high growth rate and occur in disturbed or high productive habitats whereas those with a low PNUE occur in stressful or low productivity habitats. Thus, it is highly probable that different competitive strategies are based on differences in PNUE and consequently on differences in RGR.
In order to study the interaction between nitrogen and interspecific competive ability, a competition study between Geranium pusillum L. and Erodium cicutarium L. L'Hér. was performed. The two species were chosen as a model system because they belong to the same family and have similar growth forms but apperently differ in their N-requirements. According to the Ellenberg-N index (Ellenberg et al. Citation1992), G. pusillum prefers rather high nitrogen levels whereas, E. cicutarium is indifferent to nitrogen levels; furthermore, G. pusillum is more often found on nitrogen rich soils than E. cicutarium. Both species are annual plants, and they grow to approximately the same size.
The two model species were grown in monoculture and mixculture along a nitrogen gradient. Seed set data were fitted to the generalization of a hyperbolic competition model (Damgaard Citation2003) and the probabilities of different ecological scenarios were calculated. As G. pusillum has a high Ellenberg-N and grows on fertile soils compared to E. cicutarium, our prior expectation was that the competitive ability of G. pusillum relative to E. cicutarium would increase with the nitrogen concentration.
Materials and methods
Growth medium
The experiment was carried out from 17 February to 19 May 2004 in a greenhouse located at NERI Silkeborg, Denmark. In order to create a constant and controlled environment in which the only nitrogen available to the plants was the nitrogen applied with the water, vermiculite was chosen as growth medium (for details, see The Vermiculite Association. Information on vermiculite: http://www.vermiculite.org [accessed 17 December 2004]).
Fertilizer and water
During germination, the plants were watered with fertilizer solution 1: Pioner NPK Makro 14−3−23+Mg 100 g l−1 and phosphoric acid 25 ml l−1. During the competition experiment, the plants were watered with fertilizer solution 2: 12 mg l−1 P; 54 mg l−1 K; 46 mg l−1 Ca; 9 mg l−1 Mg; 36 mg l−1 S; 0.6 mg l−1 Fe; 0.2 mg l−1 Mn; 0.06 mg l−1 Cu; 0.07 mg l−1 Zn; 0.09 mg l−1 B and 0.01 mg l−1 Mo, and varying nitrogen concentrations.
Nitrogen was applied as NH4NO3 and added to fertilizer solution 2 in different concentrations resulting in four dilutions with different nitrogen concentrations: 1, 4, 20 and 60 mg total N l−1. As ammoniumnitrate has previously been applied successfully when a nitrogen gradient was to be established (Wilson & Tilman Citation1991, Davis et al. Citation1999, Lowe et al. Citation2003) and has proven to generate optimal growth in many plant species (Kirkby Citation1981, Marschner Citation1995), this was chosen as the nitrogen source.
The four nitrogen concentrations represent a gradient ranging from nitrogen poor to nitrogen rich environment (Gundersen & Rasmussen Citation1995, Marschner Citation1995, Callesen et al. Citation1999).
In order to verify the nitrogen gradient, 2×20 g growth medium were taken from all nitrogen levels two days after the last fertilizer application. Plant available ammonium (), nitrate (
), nitrite (
) and orthophosphate (
) were extracted from the growth medium samples in 100 ml 1M KCL. The level of the different ions in the filtered extract were then measured on a spectrophotometer after having mixed 5 ml of the filtered extract with the relevant reagents listed below:
: 0.5 ml salicylate/citrate-reagent +0.5 ml DIC-reagent, measured at 690 nm after 1 hour;
: 250 µl 4M H2SO4, measured at 220 and 270 nm, the two results were subtracted;
: 0.1 ml sulphanilamide solution +0.1 ml N-(1-naphthyl)-ethylendiamine-dihydrochloride-solution, measured at 545 nm after 20 min;
: 1 ml Ap-reagent, measured at 880 nm after 10 min (Schierup & Jensen Citation1981, Brix & Schierup Citation2003).
Plants
Seeds of both species were purchased from Herbiseed (Wokingham, UK), sown in vermiculite filled polystyrene boxes, and placed in a greenhouse cell with 16 hours day (contribution from artificial growth light, OSRAM VIALOX NAV T approximately 900 µmol m−2 s−1) and day/night temperatures of 18°C/15°C. Light and temperature were controlled automatically (DGT-Volmatic climate computer). During germination, the plants were watered six times a day in a 5-min period (ebb/flood watering system) with fertilizer solution 1. Conductivity and pH in the water were recorded and manually adjusted.
Competition experiment
Two-week-old seedlings of the two species were transplanted and grown in a response surface design (Inouye Citation2001) at three different densities and in two culture-types (mono, mix). The 12 combinations of species, culture-type, and density were replicated at four nitrogen levels resulting in a total of 48 treatments, and 100 plants were assigned to each treatment. This design results in 36 plots as each mix-plot contains two treatments. In order to cover the span from ample to crowded condition, three densities (200, 400 and 1000 plants m−2) were chosen on the basis of preparatory experiments and literature studies (Harmon & Stamp Citation2002).
The observed variation in biomass and fecundity may arise from three different sources: Among plants within a plot, among plots, and among treatments. However, our experience from observation of individually competing plants tells us that a skewed size-distribution due to size-asymmetric growth and a sizeable border effect are frequently observed. As there were logistic constraints on the number of experimental plants, the number of plants within a plot was augmented in the experimental design at the price of being able to measure a possible among-plot effect, i.e., the among-plot variation was a priori assumed to be negligible compared to the two other sources of variation. This assumption was validated by subjective assessments of the plots during the course of the experiment. Furthermore, as the mean treatment effects were analysed in a regression-based response surface competition model in which the primary goal is to determine the shape of the response surface, a possible among-plot effect among adjoining treatment points will partly be “averaged out” (Inouye Citation2005). In order to minimize spatial covariance (Damgaard Citation2004a) the seedlings were transplanted to random positions in the 36 experimental plots.
The above-ground biomass was harvested when the majority of the plants had finished flowering. Numbers of fruits from ten randomly selected plants from each treatment were counted. The plants were dried for 24 h at 80°C and weighed individually afterwards.
Relationship between biomass and fecundity
Effects of biomass, nitrogen, and density on the seed set in the two species were analysed by use of the ANOVA procedure (SAS Citation2001) with number of seeds as the dependent variable, and a linear regression model was made. The following equations were used to estimate number of seeds for all plants:
Competition model
The effect of the nitrogen treatment on the fecundity of the two competing species was modelled by a generalization of a discrete hyperbolic competition model (Damgaard Citation2003). We assume that within the limited domain of the conducted competition experiment, nitrogen affects the competitive interactions and the fecundity of the susceptible plant species linearly, i.e.,
The model was fitted to the average fecundity measurements after that both the data and the competition model had been Box-Cox-transformed with λ=-1 and λ=1. In order to avoid negative parameter values the parameters a i , b i , d i, and f i were re-parameterized with the exponential function during the fitting procedure.
The joint Bayesian posterior distribution of the parameters in the competition model was sampled using the Metropolis-Hastings algorithm with a multinomial candidate distribution (100.000 iterations with a burn-in period of 1000) assuming uniform prior distributions of the location parameters. The sampling procedure was checked by visual inspections of the sampling chains besides which also the computing, the autocorrelation, and acceptance ratio were checked (Carlin & Louis Citation1996).
Equilibrium properties of the competition model
Knowledge on the equilibrium properties may provide valuable information in predicting the future states of the plant community. Assuming a constant and density-independent probability of seed germination and seedling establishment (p i ), the recursive equations for the density of the two annual plant species in the year t+1 are:
Results
The presence of a nitrogen gradient was confirmed by analysing the vermiculite medium in the laboratory at the end of the experiment: The content of plant available nitrogen increased along the gradient.
There was a significant effect of nitrogen, density and culture-type on the biomass of both species (). Individuals of both species grew larger at higher nitrogen levels and when grown at low density (200 plants m−2), the plants displayed saturated growth and mean individual biomass seemed to increase asymptotically towards a maximum (a–d).
Figure 1. The relationship between nitrogen applied to the plants grown in the greenhouse and the mean individual plant biomass at density 200 plants m−2, 400 plants m−2 and 1000 plants m−2. (a) G. pusillum grown in mix with E. cicutarium; (b) G. pusillum grown in monoculture; (c) E. cicutarium grown in mix with G. pusillum; and (d) E. cicutarium grown in monoculture. Variation is shown as standard error.
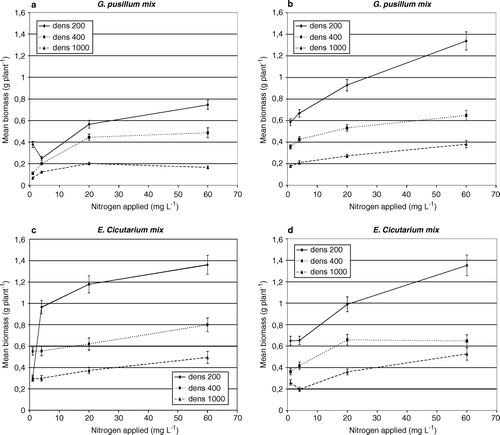
Table I Analysis of variance on the mean biomass of each treatment in the greenhouse.
However, when the density was increased, the response to nitrogen became less conspicuous and rather linear than asymptotic (a–d). The two species responded differently to culture-type (): E. cicutarium obtained the highest mean individual biomass and responded more markedly to increased nitrogen when grown in mixculture (c,d) whereas G. pusillum performed best and responded most to nitrogen when grown in monoculture (a,b).
Based on the joint posterior distributions of the parameters, the interspecific competitive abilities of the two species as functions of the nitrogen concentrations were calculated as c i +γ i h. By calculating the frequency of incidences in the MCMC simulation where c E +γ E h was larger than c G +γ G h at a specific nitrogen concentration, it was found that E. cicutarium had a higher competitive impact on G. pusillum than opposite at most nitrogen levels (). However, the interspecific competitive ability of the two species varied with the nitrogen level thus showing that the probability of an E. cicutarium having a higher competitive impact on G. pusillum increased strongly to a maximum at nitrogen level 10 mg l−1 and subsequently decreased, but never reached probabilities less than 0.95 afterwards ().
Figure 2. The relationship between nitrogen applied to the plants and the probability that c E >c G .
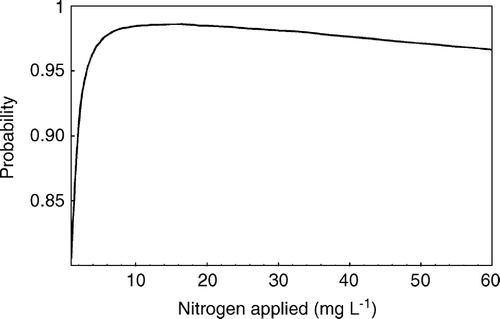
The equilibrium properties of the fitted model were investigated with varying probability of germination and establishment within a biologically realistic range and, if the probability of germination and establishment of both species were assumed to be identical, the overall conclusion was always the same: The model predicted that E. cicutarium would outcompete G. pusillum at high nitrogen levels, that the most abundant species would outcompete the other at intermediary nitrogen levels thus leaving invasion impossible, and that G. pusillum would only be competitive superior at low nitrogen levels. The probability of co-existence is very low (a).
Figure 3. The probability of different outcomes of the competition between E. cicutarium and G. pusillum along the nitrogen gradient at ecological equilibrium. (a) Probability of germination and establishment: E. cicutarium=0.1 and G. pusillum=0.1; (b) Probability of germination and establishment: E. cicutarium=0.05 and G. pusillum=0.1.
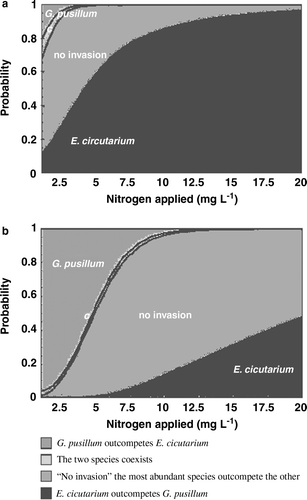
Naturally, when the probability of germination and establishment of E. cicutarium was assumed to be relatively low compared to G. pusillum, G. pusillum was able to invade at low nitrogen concentrations (b), but the overall outcome of the interaction between nitrogen and competitive ability of the two species remained the same.
Discussion
The plant species G. pusillum and E. cicutarium belong to the family Geraniaceae. They are therophytes reaching vegetative and reproductive stage in one season. Both species flower from April to August, they have small flowers, and they are partly self-pollinating (Hansen Citation2004). Furthermore, they have schizocarp fruits containing five seeds (Judd et al. Citation1999), and they obtain similar size. There are examples of the two species occurring naturally in the same area (Strandberg Citation1999, Ejrnæs personal communication 2004), but normally, E. cicutarium is found on sandy, infertile soils whereas G. pusillum prefers arable areas (Hansen Citation2004).
The Ellenberg-N for G. pusillum is 7 whereas the Ellenberg-N for E. cicutarium is X which means that E. cicutarium is indifferent to nitrogen level. Ejrnæs (personal communication 2004) has estimated the Ellenberg-N in abandoned Danish fields. He observed that the mean Ellenberg-N for habitats containing G. pusillum was 5.6 whereas the mean Ellenberg-N for habitats containing E. cicutarium was 4.5. This means that generally, G. pusillum is observed to co-exist with more nitrogen demanding plants than E. cicutarium.
We therefore expected that the nitrogen availability had a significant impact on the interspecific competition.
Nitrogen had a significant effect on the biomass of both species, and the response to nitrogen varied significantly with density. Plants which were grown at low density increased their biomass relatively more and faster in response to nitrogen compared to plants grown at higher density. When density was low, the growth limiting factor was nitrogen, and when density was high, competition for light became more important. According to the Ellenberg Index, both species used in this experiment are light demanding (Ellenberg-L: E. cicutarium=8, G. pusillum=7) (Ellenberg et al. Citation1992) and therefore possibly inhibited by the decreased light availability which is the result of the shading by neighbour plants at high plant densities.
Nitrogen had a significant effect on the fecundity of both species and had a significant impact on the competitive ability of the two species. E. cicutarium was generally more competitive superior when grown together with G. pusillum. The difference in mean individual biomass between the two species in mixculture can be explained by an effect of interspecific competition as G. pusillum obtained mean individual biomass equal to E. cicutarium when grown in monoculture. Kimball and Schiffman (Citation2003) found that E. cicutarium grew best without intraspecific competition in nature, but were not noticeably limited by interspecific competition; this fact supports the observations from our greenhouse experiment. At the lowest nitrogen level, the interspecific difference in mean individual biomass is insignificant and additionally, the competition is more symmetric. When extrapolating to ecological equilibrium, E. cicutarium is generally competitively superior and the probability of E. cicutarium outcompeting G. pusillum increases with the level of nitrogen.
Plants are generally highly plastic organisms, and high density of neighbour plants can influence on the biomass allocation and stem elongation (Callaway et al. Citation2003). Changes in the red:far-red ratio may trigger a process of stem elongation which may enable the individual plant to capture more light (Dudley & Schmitt Citation1995 Citation1996, Harley & Bertness Citation1996, Schmitt et al. Citation1999). In our case, it was observed visually that E. cicutarium and G. pusillum both grew taller and more slender when density increased; apart from making the plant more competitive for light, this might also have the consequence that the plants experience less conflict with neighbour plants and thus extra biomass can be gained without increasing mortality (Weller Citation1987). Individuals from both species displayed plasticity, but E. cicutarium developed very long stems twisting their way through the plots whereas G. pusillum tended to have less exploitative growth. The more plastic growth form of E. cicutarium has probably made it possible for individuals of this species to grow larger, compete more efficiently for light, and thus produce more seeds. Brooks (Citation2003) demonstrated that soil nitrogen addition can increase the dominance of alien annuals in the Mojave Dessert – one of them was E. cicutarium. This observation is in accordance with the observations in our experiment; namely that E. cicutarium increased its biomass proportionally more than G. pusillum with increased nitrogen in mixculture and thus increased its competitive ability with increased nitrogen.
Both species are naturally associated with open, ruderate habitats (Lid & Lid Citation1994, Mossberg et al. Citation1999, Hansen Citation2004) and this might indicate that the species establishment and growth depend on a colonization window rather than competition for nutrients. Ejrnæs (personal communication 2004) has recorded the occurrence of plant species in abandoned fields and suggests that Ellenberg-N is only marginally significant for the occurrence of G. pusillum in nature and not at all significant for the occurrence of E. cicutarium in nature; the two species respond more to the habitat age, pH, and soil moisture. The reason why G. pusillum is often observed in nutrient rich habitat might be that these habitats are also early successional and open habitats (Ejrnæs, personal communication 2004). Lauringson and Talgre (Citation2003) reported that abandoned fields were characterized by constantly changing plant communities: Species disappeared while others became prevalent year by year indicating large stochasticity, and the less competitive G. pusillum could benefit from such upheavals. In nature, the two species may really diverge along a disturbance gradient or successional gradient rather than of an underlying nitrogen gradient, and this could explain that the results of the competition experiment were not in accordence with the expected outcome.
Nevertheless, the experiments described in this paper demonstrate that there is an effect of a nitrogen gradient on the competition, and this fact stresses the need for further investigations into the effect of gradients on plant competition. Our results show that even a slight increase in nitrogen level can cause large changes of the outcome of competition between species and thus potentially result in changes in competitive exclusion and plant community composition.
John-Geert Rytter, Trine Guldager S⊘rensen, Jens Krebs, The staff of Department of Plant Biology Aarhus University, Andreas Isager, Johannes Irminger, and Eva Christensen provided practical help in the field, in the laboratory, and during the writing process. Rasmus Ejrnæs and Morten Tune Strandberg delivered information on the two species and gave us access to their own data. Preben Blæsild helped with the statistics. Marie Louise Udesen Theut, Kristian Danielsen and Gitte Hansen reviewed early versions of this manuscript. Eskil Irminger provided linguistic assistance.
References
- Aerts , R and Chapin , III FS . 2000 . The mineral nutrition of wild plants revisited: A re-evaluation of processes and patterns . Adv Ecologic Res , 30 : 1 – 67 .
- Berendse , F and Aerts , R . 1987 . Nitrogen-use-efficiency: a biologically meaningful definition? . Functional Ecol , 1 : 293 – 296 .
- Brix , H and Schierup , H . 2003 . Analyseforskrifter 2003 , Afdeling for Botanisk Økologi : Aarhus Universitet .
- Brooks , ML . 2003 . Effects of increased soil nitrogen on the dominance of alien annual plants in the Mojave Desert . J Appl Ecol , 40 : 344 – 353 .
- Callaway , RM , Pennings , SC and Richards , CL . 2003 . Phenotypic plasticity and interactions among plants . Ecology , 84 : 1115 – 1128 .
- Callesen , I , Raulund-Rasmussen , K , Gundersen , P and Stryhn , H . 1999 . Nitrate concentrations in soil solutions below Danish forests . Forest Ecol Manage , 114 : 71 – 82 .
- Carlin , BP and Louis , ThA . 1996 . Bayes and Empirical Bayes Methods for Data Analysis , London : Chapman and Hall .
- Damgaard , C . 1998 . Plant competition experiments: Testing hypotheses and estimating the probability of coexistence . Ecology , 79 : 1760 – 1767 .
- Damgaard , C . 2003 . Modeling plant competition along an environmental gradient . Ecological Modelling , 170 : 45 – 53 .
- Damgaard , C . 2004a . Inference from plants competition experiments: The effect of spatial covariance . Oikos , 107 : 225 – 230 .
- Damgaard , C . 2004b . Evolutionary ecology of plant-plant interactions – an empirical modelling approach , Aarhus : Aarhus University Press .
- Davis , MA , Keith , JW , Reich , PB , Tjoelker , MG , Schaeffer , T and Muermann , C . 1999 . Survival, growth, and photosynthesis of tree seedlings competing with herbaceous vegetation along a water-light-nitrogen gradient . Plant Ecol , 145 : 341 – 350 .
- Dudley , SA and Schmitt , J . 1995 . Genetic differentiation in morphological responses to simulated foliage shade between populations of Impatiens capensis from open and woodland sites . Functional Ecol , 9 : 655 – 666 .
- Dudley , SA and Schmitt , J . 1996 . Testing the adaptive plasticity hypothesis: Density-dependent selection on manipulated stem length in Impatiens capensis . Am Naturalist , 147 : 445 – 465 .
- Ejrnæs , R , Hansen , DN and Aude , E . 2002 . Changing course of secondary succesion in abandoned sandy fields . Biolog Conserv , 109 : 343 – 350 .
- Ellenberg , H , Weber , HE , Düll , R , Wirth , V , Werner , W and Paulissen , D . 1992 . Zeigerwerte von Pflanzen in Mitteleuropa . Scripta Geobotanica , 18 : 1 – 258 .
- Gundersen , P and Rasmussen , L . 1995 . Nitrogen mobility in a nitrogen limited forest at Klosterhede, Denmark, examined by NH4NO3 addition . Forest Ecol Manage , 71 : 75 – 88 .
- Hansen K , editor . 2004 . Dansk feltflora. 1. udgave, 11. oplag, Gyldendal, Viborg .
- Harley , CDG and Bertness , MD . 1996 . Structural interdependence: An ecological consequence of morphological responses to crowding in marsh plants . Functional Ecol , 10 : 654 – 661 .
- Harmon , GD and Stamp , NE . 2002 . Relative size early in population development determines reproductive status of individual Erodium cicutarium plants . Am Midland Naturalist , 147 : 32 – 43 .
- Hikosaka , K . 2004 . Interspecific differences in the photosynthesis-nitrogen relationship: Patterns, physiological causes, and ecological importance . J Plant Res , 117 : 481 – 494 .
- Hikosaka , K and Hirose , T . 2001 . Nitrogen uptake and use by competing individuals in a Xanthium canadense stand . Oecologia , 126 : 174 – 181 .
- Inouye , BD . 2001 . Response surface experimental designs for investigating interspecific competition . Ecology , 82 : 2696 – 2706 .
- Inouye , BD . 2005 . Scaling up from local competition to regional coexsistence across to scales of spatial heterogeneity: insect larvea in the fruits of Apaiba membranacea . Oecologia , 145 ( 2 ) : 188 – 196 .
- Judd , WS , Cambell , CS , Kellogg , EA and Stevens , PF . 1999 . Plant systematics: A phylogenetic approach , Sunderland, , USA : Sinnauer Associates, Inc .
- Kimball , S and Schiffman , PM . 2003 . Differing effects of cattle grazing on native and alien plants . Conserv Biol , 17 : 1681 – 1693 .
- Kirkby , EA . 1981 . “ Plant growth in relation to nitrogen supply ” . In Terrestrial nitrogen cycles , Edited by: Clark , FE and Rosswall , T . Stockholm : Ecological Bulletin .
- Lambers , H and Poorter , H . 1992 . Inherent variation in growth rate between higher plants: A search for physiological causes and ecological consequences . Adv Ecological Res , 23 : 187 – 261 .
- Lauringson , E and Talgre , L . 2003 . Problems of abandoned fields . Agron Res , 1 : 63 – 67 .
- Lid , J and Lid , DT . 1994 . Norsk flora. Det Norske Samlaget , A.s. Norway : Gj⊘vik Trykkeri .
- Lowe , PN , Laurenroth , WK and Burke , IC . 2003 . Effects of nitrogen availability on competition between Bromus tectorum and Bouteloua gracilis . Plant Ecol , 167 : 247 – 254 .
- Marschner , H . 1995 . Mineral nutrition of higher plants , London : Academic Press .
- Mossberg , B , Stenberg , L and Ericsson , S . 1999 . Den store nordiske flora , Gads forlag, K⊘benhavn .
- Poorter , H , Remkes , C and Lambers , H . 1990 . Carbon and nitrogen economy of 24 wild-species differing in relative growth-rate . Plant Physiol , 94 ( 2 ) : 621 – 627 .
- Rickey , MA and Anderson , RC . 2004 . Effects of nitrogen addition on the invasive grass Phragmites australis and a native competitor Spartina pectinata . J Appl Ecol , 41 : 888 – 896 .
- SAS. 2001 . SAS/STAT version 8.02 , Cary, NC, USA : SAS Institute Inc .
- Schierup H , Jensen A . 1981 . Vejledning i kemisk og fysisk analyse af jordpr⊘ver og plantemateriale . Botanisk Institut, Aarhus Universitet .
- Schmitt , J , Dudley , SA and Pigliucci , M . 1999 . Manipulative approaches to testing adaptive plasticity: Phytochrome-mediated shade-avoidance responses in plants . Am Naturalist , 154 : S43 – S54 Suppl .
- Sprungel , DG . 1983 . Correcting for bias in log-transformed allometric equations . Ecology , 64 : 209 – 210 .
- Stevens , CJ , Disse , NB , Mountford , JO and Gowing , DJ . 2004 . Impact of nitrogen deposition on the species richness of grassland . Science , 303 : 1876 – 1878 .
- Strandberg M , 1999 . St⊘rrelse og sammensætning af fr⊘banken på fire midtjyske græslandslokaliter, Danmarks Milj⊘unders⊘gelser .
- Tomassen , HBM , Smolders , AJP , Limpens , J , Lamers , LPM and Roelofs , JGM . 2004 . Expansion of invasive species on ombotrophic bogs: Desiccation or high N deposition? . J Appl Ecol , 2004 : 139 – 150 .
- Vitousek , PM and Howarth , RW . 1991 . Nitrogen limitation on land and sea: How can it occur? . Biogeochemistry , 13 : 87 – 115 .
- Weller , DE . 1987 . Self-thinning exponent correlated with allometric measures of plant geometry . Ecology , 68 : 813 – 821 .
- Wilson , SD and Tilman , D . 1991 . Components of plant competition along an experimental gradient of nitrogen availability . Ecology , 72 : 1050 – 1065 .