Abstract
Non-alcoholic beers have seen steady growth in recent years and are attracting customer interest. At the same time, consumer demand for non-alcoholic beers is rising. While wort-like flavors are often criticized in beer and also regarded as off-flavors, there is growing demand for beer flavor diversification. The latest research results prove that certain non-Saccharomyces yeast species can produce distinctive fruity flavor profiles during wort fermentation. In this study, 15 different yeast strains were evaluated for their suitability to produce novel flavor profiles during fermentation in wort from malt extract while being tested for their ability to produce less than 0.5% (v/v) ethanol. The yeast strains were therefore analyzed for their sugar utilization and were compared with the maltose-negative reference yeast strain Saccharomycodes ludwigii TUM SL17. Following the fermentation experiments, the beers were analyzed for secondary metabolites and ethanol content before being tasted by trained assessors. The results reveal that all 15 yeasts are suitable for the production of non-alcoholic beers based on their sugar utilization. Particularly promising flavors were produced by three yeast strains of the species Cyberlindnera saturnus, which were reminiscent of cool mint sweets, pear, and banana. Two further yeasts of the species Kluyveromyces marxianus and Saccharomycopsis fibuligera also produced a wide range of pleasant fruity flavors. Although the secondary metabolites were mostly below the odor thresholds based on regular alcoholic beers, this study reveals that the thresholds in non-alcoholic beers can be classified as significantly lower.
Supplemental data for this article is available online at https://doi.org/10.1080/03610470.2021.2012747 .
Introduction
The global market share for non-alcoholic beers has grown steadily in recent years and is expected to grow further in the years ahead. Alcohol is increasingly the subject of critical debate from both a health and social perspective, which is causing consumers to turn more and more to non-alcoholic beers.[Citation1,Citation2]
A simple technological tool of producing non-alcoholic beers is the use of low-fermentation yeasts that are maltose- and maltrotriose-negative, however, they also need sufficient hop tolerance. A study by Methner et al. revealed that most yeasts from food fermentations have a high tolerance to hops. Out of a total of 110 yeasts examined, without exception, all grew at iso-α-acids up to 50 IBU.[Citation3] Maltose and maltotriose account for over 80% of the total carbohydrates in brewer’s wort so there is a significant reduction in alcohol unless the yeasts are able to metabolize these two wort sugars.[Citation4] Maltose- and maltotriose-negative yeasts are therefore suitable for producing non-alcoholic beers. Whenever these two sugars are not metabolized by the yeasts to alcohol and carbon dioxide, they remain unmodified in the wort and provide residual sweetness as well as body.[Citation5] Due to the relatively small amount of the wort sugars fructose, glucose, and sucrose, the maltose-negative yeasts have only limited carbohydrate sources available for their metabolism. Once they are utilized, the yeast ceases its activity. For this reason, in this study, yeast strains were examined that had already been described in literature as maltose-negative or at least potentially maltose-negative during fermentation. The fact that maltose is not utilized by the yeasts is due to a lack of permease in the yeast cell membrane, which would transport maltose into the cell interior via active transport.[Citation6] This process technology for non-alcoholic beers is already known and was first practiced with the yeast Saccharomycodes ludwigii. As early as 1933, a patent by Haehn and Glaubitz described the production of low-alcohol beer using this yeast species,[Citation7] which was extended by an additional patent in 2009 by Kunz et al.[Citation8]
In the meantime, there is an increasing demand for flavor diversification with a focus on fruity beers without any wort flavor or other taste deficiencies as described by Blanco et al.[Citation9] Some studies have already investigated the flavor properties of various yeasts during the fermentation of wort to produce non-alcoholic beers. In 2016, Liu et al. investigated a Cyberlindnera (Williopsis) saturnus var. mrakii yeast strain for its suitability to produce a particularly fruity non-alcoholic beer and found that this resulted in desirable fruity and floral flavors, which were significantly higher in direct comparison to a beer produced with a conventional Saccharomyces cerevisiae.[Citation10] The same aim was pursued by Bellut et al. with several different Cyberlindnera yeast strains such as C. misumaiensis, C. fabianii, C. subsufficiens and C. mrakii. They classified a C. subsufficiens in particular as most suitable.[Citation11] A C. fabianii yeast strain turned out to be applicable for reducing alcohol content and increasing flavor complexity as a co-culture alongside a S. cerevisiae.[Citation12] In addition to the Cyberlindnera species, several studies have revealed Torulaspora delbrueckii to be a potential yeast strain for producing non-alcoholic beers with fruity aroma characteristics.[Citation13–16] Moreover, several studies proved that not only S. ludwigii, but also Pichia kluyveri and Kluyveromyces lactis enhanced desirable beer flavors by wort fermentations while being suitable for the production of non-alcoholic beers.[Citation15,Citation17,Citation18] Recent study results revealed that by using Schizosaccharomyces pombe, low alcohol beers can be produced despite the ability of this yeast to metabolize maltose.[Citation19] Kazachstania servazzii was also investigated for the fermentation of wort with the aim of producing a non-alcoholic beer, which was then analyzed for volatile components.[Citation20]
Based on the previous research results, 15 different non-Saccharomyces yeast strains were systematically investigated for their suitability in the production of non-alcoholic beers. Yeasts strains were considered from the genera Cyberlindnera, Kazachstania, Kluyveromyces, Pichia, Schizosaccharomyces, and Torulaspora, which have already been studied in connection with the production of non-alcoholic beers. Additionally, a yeast of the genera Lachancea as well as a yeast strain of the genera Saccharomycopsis were examined for the first time for their suitability in the production of non-alcoholic beers. To date, the yeast strain Saccharomycopsis fibuligera has been studied for the production of alcoholic beers and showed desirable aroma properties. Some strains showed weak fermentation activity in brewer’s wort so its use in the non-alcoholic sector could also be promising. The same study indicated that a Lachancea kluyveri yeast strain was maltose-negative and thus could have potential.[Citation3] Since Saccharomycodes ludwigii is well known for the production of non-alcoholic beers and has been investigated in numerous studies in this context by Meier-Dörnberg et al.,[Citation21] De Francesco et al.,[Citation22] Pahl,[Citation23] Parise et al.,[Citation24] Gutiérrez et al.,[Citation17] Callejo et al., [Citation25] and Vaštík et al.,[Citation19] this yeast was used as a reference in this study.
In addition to continuous fermentation control that included measuring the final ethanol contents as well as pH values of the beers, the parameters of sugar utilization and secondary metabolites were analyzed. The sugar compositions of the beers were determined by two different high-performance liquid chromatography (HPLC) methods, while additional growth tests were performed in selective media. Analysis of the fermentation by-products, various esters, organic acids, higher alcohols, ketones, acetaldehyde, dimethyl sulfide (DMS), and 4-vinylguaiacol (4-VG) was performed by gas chromatography/mass spectrometry (GC/MS). Moreover, sensory analyses were carried out that included profiling as well as testing for off-flavors.
The aim of this study was to find suitable non-Saccharomyces yeast strains to produce non-alcoholic beers with distinguished flavor properties but without wort flavor. Wort-like off-flavors [Citation20,Citation26,Citation27] were not analyzed by GC/MS, however, they were considered in the sensory evaluations of the beers. Yeasts were selected based on the fact that they did not produce phenolic off-flavors. The target alcohol content of the beers was a maximum of 0.5% (v/v) according to the German regulation for non-alcoholic beers.[Citation28]
Experimental
Yeast strains
The 16 yeast strains investigated in this study are listed in with corresponding abbreviations. Except for the yeast strain Cyberlindnera saturnus CSa1, which comes from a private collection, all other yeast strains can be purchased commercially. The collection site, from which the yeast strains were obtained, is indicated in the footnote of .
Table 1. Yeast species and strain numbers with corresponding abbreviations used in this study.
Yeast propagation and fermentation
The selected yeast strains from were inoculated from wort slope agars under sterile conditions into 500 mL flasks each containing 250 mL of unhopped wort (7.0°P, pH 5.5) that was made from malt extract (Weyermann®, Bamberg, Germany). Since the composition of malt extract is always the same, the results are highly comparable. The wort was analyzed (extract content, pH, and sugar composition) according to the methods in .
Table 2. Analytical methods of the wort and the non-alcoholic beers after MEBAKa and Donhauser et al.b
After 72 h of propagation at 20 °C on a WiseShake orbital shaker (Witeg Labortechnik GmbH, Wertheim, Germany) at 80 rpm, the yeast suspensions were transferred to sterile 2500 mL flasks filled with 1800 mL of comparable unhopped wort and propagated for an additional 72 h. After propagation, the cell counts were determined using the Cellometer® Vision (Nexcelom Bioscience LLC, Lawrence, MA, U.S.A.).
The 16 different yeast strains listed in were tested in fermentation trials. For the fermentation experiments, a maltose-negative yeast strain known to produce non-alcoholic beers was included as a reference: Saccharomycodes ludwigii TUM SL17. In small-scale fermentations performed in triplicate, 1800 mL unhopped wort (7.0°P, pH 5.5) in 2000 mL sterile Duran glass bottles (Schott AG, Mainz, Germany) was pitched, each at 15 × 106 cells/mL (±σ = 1 × 106 cells/mL) and locked with glass fermentation locks on top. To produce the wort, malt extract (Weyermann®, Bamberg, Germany) was blended in a wort boiler in two batches to the defined original wort, boiled for 5 min and then cooled to the pitching temperature of 20 °C using a plate cooler. For each batch, 50 L wort was pumped into a cylindroconical tank before 1800 mL of each was filled into the 2000 mL bottles for the fermentation trials. Propagation yeasts were centrifuged (Roto Super 40, Andreas Hettich GmbH & Co. KG, Tuttlingen, Germany) in sterilized 500 mL PPCO centrifuge bottles (Nalgene, Thermo Fisher Scientific, Waltham, MA, U.S.A.) at 750 × g for 5 min and the supernatant was discarded before pitching. The samples were incubated at a 20 °C fermentation temperature. The fermentation time of 144 h was chosen based on previous experiments with the reference yeast S. lud SL17. For this yeast strain, based on well-founded preliminary trials, a recommendation exists for the production of non-alcoholic beers, which suggests an original wort of 7°P and a fermentation temperature of 15-20 °C in order to achieve an ethanol content < 0.5% (v/v) in the final beer.[Citation21,Citation30] Since it is known that the reference yeast strain completely converts glucose and fructose as well as sucrose after a fermentation period of 144 h at a temperature of 20 °C and an original wort of 7°P, this experimental design was transferred to the 15 investigated yeast strains. The main fermentation proceeded for 144 h and fermentation progress was checked every 24 h by recording the weight loss. Fermentation progress can be determined by weight loss, which is based on Balling’s assumption that during fermentation an average of 2.0665 g of extract is converted into 1 g of alcohol, 0.9565 g of carbon dioxide, and 0.11 g of yeast.[Citation31] Subsequently, the samples were then placed into cold storage at 2 °C for another 96 h before performing the analytical analyses shown in and the sensory evaluation.
Analytical methods
lists the analyses of the initial wort as well as of the non-alcoholic beers. All beers were prepared in triplicate with each sample analyzed by the methods listed in . Subsequent statistical analyses were performed using OriginPro 2020 statistical software.
The apparent attenuation is calculated using a formula for the degree of fermentation and the original wort of the sample, which is described in detail in Bleyer et al.[Citation32]
Sugar utilization test
In order to assess whether some of the investigated yeast strains were actually able to metabolize maltose or maltotriose, additional sugar utilization tests were performed based on the method of Nikulin et al.[Citation33] For this purpose, two synthetic YP-media were prepared from 1.0% yeast extract (Sigma-Aldrich, St. Louis, MO, U.S.A.), 2.0% peptone from casein, pancreatic digest (Sigma-Aldrich, St. Louis, MO, U.S.A.), either 1.0% D(+)-maltose monohydrate 95% (Merck, Darmstadt, Germany) or 1.0% D-maltotriose 98% (Thermo Fisher Scientific, Waltham, MA, U.S.A), and 96.7% double distilled water. The yeast strains were transferred from pure slope agar cultures into 1 mL sterile physiological sodium chloride solutions before they were counted using the Cellometer® Vision (Nexcelom Bioscience LLC, Lawrence, MA, U.S.A.). The required volumes for 0.1 × 106 cells/mL were calculated and inoculated under sterile conditions into 25 mL of YP-medium into 50 mL flasks each and were sealed with cotton plugs. The samples were incubated at 20 °C on a WiseShake orbital shaker (Witeg Labortechnik GmbH, Wertheim, Germany) at 80 rpm. Every 24 h, absorbances were measured in triplicate using an UV-Vis photometer (Evolution 300, Thermo Fisher Scientific, Madison, WI, U.S.A.), set to an optical density (OD) of 600 nm, until there was no further increase in OD. Although the coefficient of variation of the applied HPLC analysis is 13% for maltose and 15% for maltotriose, the limits for the tests in selective media were set significantly lower at 5% to achieve reliable results. In cases where the yeasts were able to utilize more than 5% of the maltose present in the beer by HPLC sugar analysis (cf ) based on the mean value of the triple determination, their growth was tested in YP-medium + 1% maltose. In addition, all yeasts that could metabolize more than 5% maltotriose based on the mean value of the triple determination were exposed to YP-medium + 1% maltotriose.
To check the measured values for maltose and maltotriose due to the relatively high coefficients of variation, glucose, maltose, and maltotriose of a selected beer were randomly checked using a further HPLC method. To be able to safely exclude measurement inaccuracies, the sugars were chemically modified in order to make them subsequently visible in the HPLC with a UV/Vis detector. Chemical modification for visualization in HPLC using UV/Vis detector proceeded as shown in .
Figure 1. Chemical modification of glucose for HPLC visualization using UV/Vis detector. (Endres, F.; Prowald, A.; Fittschen, U. E. A.; Hampel, S.; Oppermann, S.; Jacob, F.; Zarnkow, M.; Hutzler, M.; Methner, Y.; Laus, A. Vergleichendes isothermes Maischen bei 72 °C, BRAUWELT 2021, 9-10, 223-227.)
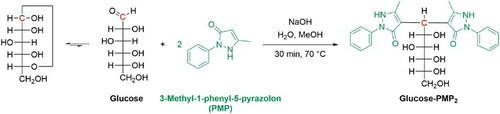
The reaction procedure followed Honda et al.[Citation34] Fructose was modified with only one molecule, while sucrose did not react with the chromophore. The modified sugars showed strong absorbance at 245 nm. The solutions were then analyzed using an RP18 column in combination with a mixture of 20% (v/v) acetonitrile and 80% (v/v) 0.15 M ammonium acetate-acetic acid buffer (pH = 5.0). The compound 1H-benzotriazole was added as an internal standard. For the measurements, the 7°P initial wort and the three beers of the selected yeast strain were determined in triplicate. A mean chromatogram was calculated from each of the three wort and the nine beer measurements.
Sensory evaluation
The beer samples, which were tempered to 12 °C, were profiled at 20 °C room temperature by a sensory panel of ten DLG (Deutsche Landwirtschafts-Gesellschaft e.V., Frankfurt, Germany)-certified assessors to determine the main flavor components of the individual beers. To exclude external interferences during the tastings, they were held in an appropriately neutral, white-colored room with individual tasting chambers. The trained panelists were asked to describe both the odor and the taste of the beers. As part of the evaluations, the specified odor and taste attributes were summarized. The first part of the evaluations recorded how many tasters described the respective beers as generally fruity. If significant fruitiness could be perceived in the beers, the individual fruit attributes could be broken down and statistically verified by percentage. The evaluation by percentage indicated how many tasters named the corresponding fruit attribute. The significance level was analyzed for the paired sensory testing of the attribute “fruity” according to MEBAK sensory analysis 3.1.1. and DIN EN ISO 5495:2007 with a significance level α = 0.05. Based on these sensory methods, a significant result was achieved in cases where at least nine of the ten tasters had matching results (significant results are marked with* in the results section). The result was classified as non-significant in the cases where eight or fewer tasters delivered a matching result. In addition, a sensory test was carried out according to the DLG evaluation scheme, which comprises a rating scale from zero to five. While zero is considered the lowest score and represents insufficient product quality, a score of five points fully meets the quality expectations of the product and matches the quality description very well.[Citation35] The odor, purity of taste, and body of the beers were evaluated, while the quality of bitterness as well as the carbonation were neglected. For the tasting, the samples were assigned randomized three-digit numbers and 50 mL of each sample was served in brown 200 mL tasting glasses.
To establish possible correlation between the specific volatile compounds measured analytically and the individual flavor characteristics described by the tasters, a principal component analysis (PCA) was performed using OriginPro 2020 statistical software.
Results and discussion
Fermentation
The weight loss of the individual samples was recorded every 24 h to monitor the extract degradation by the 16 investigated yeast strains during the fermentation process. shows the fermentation progress of the yeast strains with the corresponding standard deviations including the reference yeast strain S. ludwigii TUM SL17 (marked in red). The weight loss is plotted over a total fermentation period of 144 h at a fermentation temperature of 20 °C. The individual fermentation curves are available in Supplemental File 1.
Figure 2. Mean values (n = 3) with standard deviations of the fermentation samples’ total weight loss in grams of the investigated yeast strains during the fermentation process over a fermentation period of 144 h (6 days) at 20 °C.
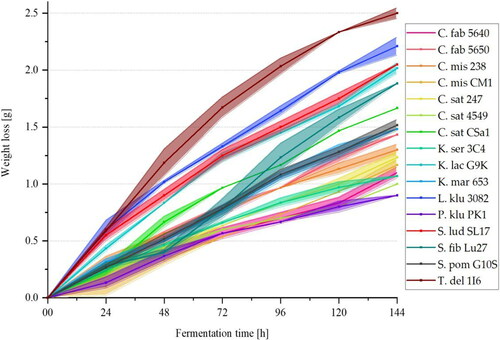
After the first 24 h of fermentation, all 16 yeasts showed a weak weight loss between 0.1 and 0.6 g. The reference yeast, S. lud SL17, exhibited a weight loss of about 0.5 g and thus fermented relatively strongly in comparison to the other yeast strains. Two yeast strains fermented slightly faster within the first 24 h: L. kluyveri 3082 as well as T. delbrueckii 1I6. The weakest fermentation activity with only approx. 0.1 g of weight loss was formed by the yeast strains P. kluyveri PK1 and C. misumaiensis CM1. The fermentation activity of the reference yeast decreased with increasing fermentation time and the other yeasts behaved similarly. The yeast strains L. klu 3082 and T. del 1I6 remained the strongest until fermentation was stopped by cooling down to 2 °C after 144 h. At 2.2 to 2.5 g, these strains lost slightly more weight than the reference yeast with about 2 g on day six. The Pichia yeast strain remained the weakest yeast within the six days of fermentation and only lost 0.9 g of weight, while the yeast strain C. mis CM1 lost slightly more weight with just over 1 g.
For each fermentation curve, a linear regression line with a corresponding coefficient of determination was generated for the mean values of weight losses. The regression equations and coefficients of determination are listed in . The coefficients of determination range between 94.8 and 99.8%, which indicates a low variation. The gradients of the regression lines can be related to the ethanol contents, which are presented in . The general trend is that the lower the gradient, the lower the ethanol content in the finished beer.
Table 3. Regression equations and corresponding coefficients of determination R2.
Table 4. Mean values and standard deviations (n = 3) of original wort (°P), apparent attenuation (%), ethanol content (% v/v) and pH value in the beers fermented by the 16 selected yeast strains.
In terms of ethanol content, it is noticeable in that the beers ranged between 0.26% and 0.55% (v/v). With an original wort of 7°P, a fermentation temperature of 20 °C, and a fermentation time of 144 h, all of the investigated yeasts are fundamentally suitable for producing non-alcoholic beers. The beers produced using the yeast strains L. kluyveri 3028 and T. delbrueckii 1I6 had values of 0.54 and 0.55% (v/v) making them slightly above the legally permissible ethanol content of 0.50% (v/v) according to the German regulation.[Citation28] They showed the strongest fermentation activity in the course of fermentation. By slightly decreasing the original wort, shortening the fermentation time, or even reducing the fermentation temperature, it could be possible to reduce the fermentation activity and the associated ethanol content of the two beers. The low apparent attenuations listed in result in relatively high amounts of residual sugars in the beers, which can potentially be metabolized by other yeasts or bacteria. Pasteurization is therefore necessary to ensure microbiological safety and at the same time to counteract a subsequent increase in alcohol content. Since the wort was produced in two batches, the original wort varies by around 0.1°P. While yeasts C. fab 5640, C. fab 5650, C. mis 238, C. mis CM1, C. sat 247, C. sat 4549, C. sat CSa1 and P. klu PK1 were pitched in the first wort batch, the second batch was used for the other yeasts.
The pH values of the beers in range between 4.60 and 5.08. Only the yeast strains S. fibuligera Lu27, K. marxianus 653, C. misumaiensis 238 and C. misumaiensis CM1 were able to induce a sufficient pH drop. Apart from these four yeast strains, the remaining ones stayed at pH values greater than 4.62, so that an additional drop in the pH to between 4.2 and 4.6 using sour wort would positively impact the taste and produce a safer product from a microbiological point of view.[Citation29]
Sugar utilization
The sugar composition of the main wort carbohydrates of fructose, glucose, sucrose, maltose, and maltotriose [Citation36] for the fermentation experiments are illustrated in , which gives the mean of the two wort batches.
Table 5. Sugar composition of the wort used for fermentation trials.
Both the wort sugars (cf ) and the sugar compositions of the beers were analyzed to draw conclusions about the sugar utilization of the individual yeast strains presented in . To minimize error when calculating the individual sugar utilizations, the average wort sugar composition of the respective wort batch was used rather than that from . Supplemental File 2 provides the sugar utilizations for each wort batch.
Figure 3. Mean values (n = 3) with standard deviations of wort sugar utilization in % of the 16 investigated yeast strains during fermentation from worts to non-alcoholic beers.
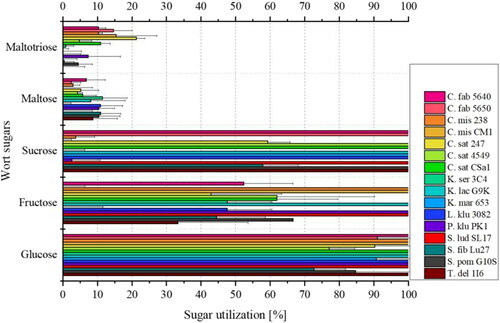
The reference yeast strain S. ludwigii SL17 (marked in red) as well as K. lactis G9K were the only yeast strains to metabolize 100% of the glucose, fructose, and sucrose present in the wort, while maltose and maltotriose were not utilized. This was to be expected for the reference yeast S. ludwigii.[Citation37] K. lactis is known to variably metabolize glucose, sucrose, and maltose.[Citation38] Accordingly, the specific yeast strain K. lactis G9K has the ability to utilize glucose and sucrose but presumably it has no permease activity.
Although T. delbrueckii 1I6 and L. kluyveri 3082 lost more weight in the fermentation control (cf ) and produced slightly higher amounts of ethanol, they seemed to only fully utilize glucose and sucrose, but not fructose. However, since the fructose concentration in wort is very low at 0.6 g/L (cf ) and the measurement inaccuracy with the applied HPLC method is relatively high for fructose with a coefficient of variation of about 20%, the fructose values should be considered only as an indication of whether fructose could generally be metabolized. Therefore, it is to be assumed that all yeasts examined have the ability to utilize fructose. In addition, the fructose content may initially appear higher when sucrose is hydrolyzed to its monosaccharides of glucose and fructose. Since S. cerevisiae is known for displaying a discrepancy in glucose and fructose utilization during fermentation,[Citation39] this behavior could be transferred to further yeast species leading to a relatively higher concentration of fructose. It is to be assumed that fructose would have been completely metabolized if the fermentation period was longer. Additionally, in a previous study by Methner et al., 8 of the 16 yeast strains were investigated in selective medium and completely metabolized fructose.[Citation3] These include yeast strains such as T. del 1I6, which according to metabolized only 33% of the fructose from the wort, K. ser 3C4 and L. klu 3082, which both utilized 48%, or C. Sat CSa1, which was at 60% utilization. This reinforces the assumption that fructose would have been fully utilized if the fermentation period was extended. The yeast strains that have not been investigated to date could also be tested in a fructose selective medium in future work in order to be able to make a reliable statement on fructose utilization. Similarly to T. delbrueckii 1I6 and L. kluyveri 3082, C. fabianii 5640 and C. saturnus CSa1 also metabolized 100% of the glucose and sucrose. Comparing the results of sugar utilization with existing literature, it can be confirmed that T. delbrueckii,[Citation40] L. kluyveri,[Citation41] C. fabianii, and C. saturnus [Citation42] metabolize glucose and sucrose. Although there are T. delbrueckii yeast strains that can variably utilize sucrose, the studied strain 1I6 could completely convert sucrose.
Although C. fabianii 5650, C. saturnus 4549, K. marxianus 653, and S. pombe had converted 100% of sucrose, only more than 75% of glucose was consumed. C. saturnus 247 was the only yeast strain showing weaker sugar utilization, as about 90% glucose and 60% sucrose were utilized. However, as fermentation was stopped after just six days, it is assumed that glucose and sucrose would also have been completely metabolized by the yeast strains given a slightly longer fermentation period. Another reason for the incomplete utilization of sucrose could be the pH value, whose optimum for the enzyme invertase is not clearly defined according to the literature.[Citation42–44] If wort acidification was applied, this could perhaps result in faster sucrose degradation. Additionally, the partially high standard deviations (relative standard deviations up to approx. 10%) need to be taken into account. According to the existing literature, C. saturnus,[Citation42] K. marxianus,[Citation38] and S. pombe [Citation46] are known for their ability to ferment glucose and sucrose.
As described in Kurtzman,[Citation45,Citation47,Citation48] the yeast strains K. servazzii 3C4, P. kluyveri PK1, C. misumaiensis 238, and CM1 were indeed unable to cleave sucrose into its monosaccharides, while they completely converted available glucose from the wort. Thus, a lack of extracellular invertase could be the reason why sucrose cannot be metabolized.[Citation49]
Based on the results (cf ), 75% of the yeasts investigated, namely C. saturnus,[Citation42] C. misumaiensis,[Citation47] K. servazzii,[Citation48] K. marxianus,[Citation38] L. kluyveri,[Citation41] P. kluyveri,[Citation45] and the reference S. ludwigii [Citation37] do not possess the property to utilize maltose, which is confirmed by the literature. The ability of K. lactis,[Citation38] S. pombe,[Citation46] and T. delbrueckii [Citation40] to metabolize maltose may vary. Only C. fabianii [Citation47] metabolizes maltose slowly and weakly, while S. fibuligera [Citation50] can metabolize maltose, albeit slowly. This can be attributed to an extracellular glucoamylase.[Citation50–53] Whether there is also a permease activity in addition to the extracellular glucoamylase activity would have to be investigated. However, the yeast strain has the potential for producing non-alcoholic beers. This is evident from a previous study, which showed that some S. fibuligera yeast strains fermented much more weakly at 27 °C and an original gravity of 12.5°P than a conventional brewing yeast such as Saccharomyces cerevisiae.[Citation3] In this study, reducing the temperature to 20 °C, and the original wort to 7°P, as well as the fermentation time to 144 h, resulted in an alcohol content < 0.5% (v/v) and thus a weak sugar utilization. Although S. fibuligera is the only yeast strain investigated besides C. fabianii, which is known to convert maltose as well as glucose and sucrose, S. fib Lu27 actually does not prove to be more fermentative. On average, only about 70% glucose, 60% sucrose, and 10% maltose were utilized (cf ). When comparing the maltose utilization with the other yeasts, some show a maltose utilization that is almost equal. Since the coefficient of variation for maltose is 13% by the applied HPLC method, measurement inaccuracies must also be taken into consideration here. Based on previous knowledge of the production of non-alcoholic beers using the reference yeast strain S. lud SL17, it is to be assumed for the investigated maltose-negative yeast strains that glucose and fructose, and optionally also sucrose, would have been utilized within the 144 h fermentation time at a temperature of 20 °C. The results show, however, that individual yeast strains certainly have different optima, which should be investigated within the scope of further research.
In general, the results of the HPLC analyses illustrate () that maltose and maltotriose can either not be metabolized at all or only to a small extent under the applied fermentation parameters in wort by the different yeast strains. Whether the yeasts from this study can actually metabolize maltose or maltotriose was investigated in further experiments in selective media. As described in the Experimental chapter, yeast strains utilizing, on average, more than 5% of maltose or maltotriose present in the beer were additionally incubated in YP-medium + 1% maltose or YP-medium + 1% maltotriose.
depicts the increases in the extinctions at an optical density of 600 nm over 168 h of ten different yeast strains in YP-media with 1% added maltose. The yeast strain S. ludwigii SL17 (marked in red) was additionally included as a maltose-negative reference yeast strain.
Figure 4. Mean values (n = 3) with standard deviations of yeast growth at 20 °C of ten yeast strains metabolizing more than 5% maltose in the HPLC analysis according to (C. fabianii 5640, C. saturnus 247 and CSa1, K. servazzii 3C4, K. lactis G9K, L. kluyveri 3082, P. kluyveri PK1, S. fibuligera Lu27, S. pombe G10S, T. delbrueckii 1I6) measured at an extinction of 600 nm in YP-media with 1% maltose over 168 h (7 days). S. ludwigii SL17 was included as reference yeast strain.
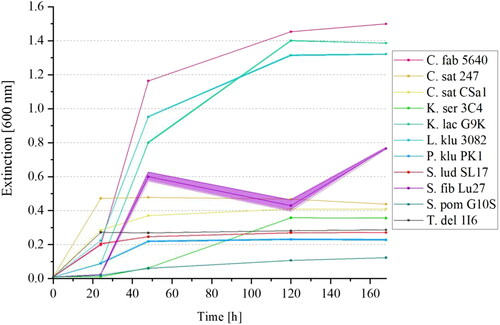
It can be observed that the extinctions increase most strongly within the first 24 to 48 h. Yeast cell growth mainly takes place during this period as the utilizable sugars from the medium are taken up by the yeast cells. In addition to 10 g/L maltose, traces of glucose, fructose, and sucrose were present in the YP-medium. These remained below concentrations of 0.1 g/L and thus below the detection limit of HPLC analysis. Nevertheless, the amounts were sufficient to ensure a slight growth of the maltose-negative yeasts and to trigger a slight increase in the absorbance. Therefore, at absorbances < 0.5, there is only slight growth due to the presence of glucose, fructose, and sucrose in the culture medium. Those yeasts that were selectively able to utilize maltose had absorbances > 0.5 within the medium due to their strong growth. shows that the yeast strains C. fabianii 5640, K. lactis G9K, and L. kluyveri 3082 reached extinctions above 1.0 after 72 h and were thus able to metabolize maltose. S. fibuligera Lu27 also metabolized maltose. Due to its unusual morphology in the selective medium, which manifested itself in a macroscopically spherical growth (small gelatinous yeast balls), the absorbance could only be measured to a limited extent by photometer. This led to measurement inaccuracies because of an inhomogeneous distribution of the yeast spheres. Even an addition of 10 mM EDTA could not break up these cell formations. HPLC sugar analysis of the selective media after 168 h showed that indeed 100% of the maltose present was metabolized by the four yeasts mentioned, while maltose was still fully detectable in the samples of the remaining six yeast strains (values not shown). The results confirm that C. fabianii and S. fibuligera are able to metabolize maltose, which is consistent with the literature.[Citation47,Citation50] K. lactis 3082 can also partially utilize maltose. This yeast species is known to be Kluyver effect positive on maltose.[Citation50,Citation54] Although Lachance et al. [Citation41] described that L. kluyveri is maltose negative during fermentation, the Kluyver effect needs to be considered as well since the samples were not incubated under completely anaerobic conditions.[Citation55] For sterile sampling every 24 h, the samples were opened so that oxygen could enter the flasks. However, further studies would be required to establish why C. fabianii and S. fibuligera metabolize maltose rapidly only when exposed to a selective medium containing maltose. It is known that these two yeast strains metabolize maltose during fermentation. However, it is not possible to provide a conclusive reason why they metabolize a similarly low level of maltose as actual maltose-negative yeast strains in a brewing wort at 7°P and a fermentation temperature of 20 °C within 144 h.
depicts the increased extinctions at an optical density of 600 nm over 168 h of seven different yeast strains in YP-media with 1% added maltotriose. Again, the maltrotriose-negative S. ludwigii SL17 (marked in red) was added as the reference yeast strain.
Figure 5. Mean values (n = 3) with standard deviations of yeast growth at 20 °C of seven strains (C. fabianii 5640 and 5650, C. misumaiensis 238 and CM1, C. saturnus 247 and CSa1, P. kluyveri PK1) metabolizing more than 5% maltotriose in the HPLC analysis according to and measured at an extinction of 600 nm in YP-media with 1% maltotriose for 168 h (7 days). S. ludwigii SL17 was included as a reference yeast strain.
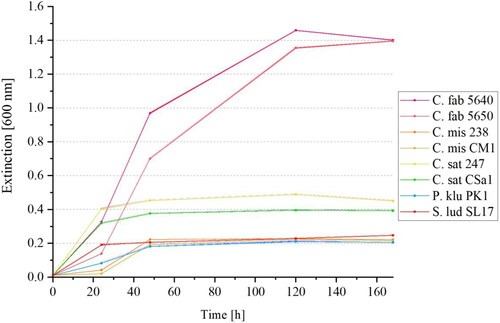
Analogous to the analysis in YP-medium + 1% maltose, the addition of 1% maltotriose at an absorbance < 0.5 indicates that the yeasts are maltotriose-negative. From the seven yeasts analyzed in total, the two C. fabianii yeast strains 5640 and 5650 were the only two to show significant growth after 168 h. They had an absorbance of around 1.4 measured at 600 nm in the photometer and are thus maltotriose-positive. Although the yeast strain C. fabianii 5650 was not examined in the selective medium with an additional 1% maltose, based on these findings it can be concluded that the yeast can metabolize both maltose and maltotriose. While the maltotriose utilization of yeast strain C. fab 5640 takes longer in direct comparison to the maltose utilization (cf ), sugar analysis of the samples by HPLC measurement after 168 h showed that the maltotriose was completely metabolized (values not shown). Here, too, further investigations are needed to clarify why maltotriose can be completely metabolized in the selective medium after around 120 h, whereas it is hardly utilized by the yeasts in brewer’s wort.
In general, the results show that the HPLC method for sugar analysis provides good indication values. However, additional analyses with greater accuracy are needed for accurate results. To safely exclude measurement inaccuracies, the sugars of a selected non-alcoholic beer produced by the yeast strain C. saturnus 247 were additionally chemically modified to make them visible in the HPLC with a UV/Vis detector. depicts the chromatogram with the modified sugars of the 7°P wort and the mean chromatogram of the three beers fermented with C. saturnus 247. The two chromatograms – wort (red) and beer (blue) – clearly show that maltose, maltotriose, and also maltotetraose remain unaffected. Glucose was utilized to 90.6%, which is consistent with the measured value from the previous HPLC analysis. Accordingly, the previous HPLC analyses of sugar utilization using the method according to are actually measurement inaccuracies. This can be applied to all other investigated yeast strains with a maltose utilization of up to 13% and maltotriose utilization of up to 15%.
Figure 6. Chromatograms (HPLC) of the modified sugars glucose, maltose, maltotriose and maltotetraose of the 7°P wort and beer fermented with the yeast strain C. saturnus 247. The sugar molecules contain one molecule of 3-methyl-1-phenyl-5-pyrazolone (fructose) and two molecules of 3-methyl-1-phenyl-5-pyrazolone (glucose, maltose, maltotriose and maltotetraose).
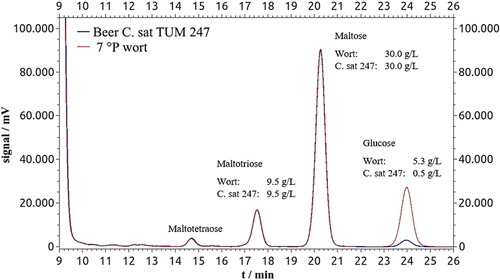
Above maltotetraose, higher sugars occurred only in traces, so that it can be assumed that the malt extract used was very well saccharified.
Secondary metabolites
As part of the analysis of secondary metabolites by GC/MS measurement of the 16 non-alcoholic beers, the following common volatiles in beer were quantified: esters, organic acids, higher alcohols, ketones, acetaldehyde, DMS, and 4-VG. In the heat map in , the volatile compounds are shown as relative values. The 100% value represents the odor threshold of the respective substance in beer based on literature sources from Meilgaard, Sannino et al., and Schieberle.[Citation56–58] If the values are above 100%, the volatile compounds should have a perceptible influence on the beer flavor. The complete table with values from triplicates can be found in Supplemental File 3. It should be noted that the flavor threshold values refer to regular alcoholic beers, so they should be considered as indicator values only. It is to be expected that some threshold values of non-alcoholic beers are fundamentally lower for selected volatiles, as both ethanol and certain volatile compounds have masking effects.[Citation27,Citation59]
Figure 7. Heat map of volatile compounds in final non-alcoholic beers produced by the 16 investigated yeast strains during fermentation of brewer’s wort at 20 °C for 144 h and detected by headspace gas chromatography measurement. The 100% value represents the threshold of volatile compounds in beer, which is based on relative values from Meilgaard, Sannino et al., and Schieberle.[Citation56–58]
![Figure 7. Heat map of volatile compounds in final non-alcoholic beers produced by the 16 investigated yeast strains during fermentation of brewer’s wort at 20 °C for 144 h and detected by headspace gas chromatography measurement. The 100% value represents the threshold of volatile compounds in beer, which is based on relative values from Meilgaard, Sannino et al., and Schieberle.[Citation56–58]](/cms/asset/95346aed-d484-456f-a35a-f85af0e3ec9b/ujbc_a_2012747_f0007_c.jpg)
By taking a closer look at the fermentation by-products, it is evident that only a few volatiles were above the odor threshold. The brighter the fields in the heat map, the more the odor threshold was exceeded. The levels of isoamyl acetate in the non-alcoholic beers produced using C. saturnus yeast strains were strikingly high between 3.1 and 7.5 mg/L. In particular, the isoamyl acetate value of the yeast strain C. sat CSa1 stood out with a concentration of 7.5 mg/L, which is 6.25 times the threshold value of 1.2 mg/L. The P. kluyveri yeast strain PK1 was also capable of producing approx. 3.5 mg/L isoamyl acetate. This substance leads to desirable fruity flavors reminiscent of banana, pear, and apple, although the flavor can also be solvent-like.[Citation56,Citation60]
A less desirable impact on the beer flavor is described for volatiles such as acetaldehyde, diacetyl, and isovaleric acid. Aldehydes are known to cause worty off-flavors in non-alcoholic beers. The specific aldehydes for unpleasant worty off-flavors in non-alcoholic beers such as methional, 2-methylbutanal, and 3-methylbutanal were not analyzed in this study.[Citation9,Citation61] However, acetaldehyde in particular is known for its fruity, green apple, grassy flavor at a threshold above 10 mg/L. Therefore, it does not necessarily lead to an undesirable flavor in beer. The same is true for diacetyl, which causes buttery flavors at thresholds above 0.10 to 0.15 mg/L.[Citation56,Citation59] Isovaleric acid, on the other hand, is associated with negative flavor characteristics, as it is responsible for sweaty-cheesy to rancid flavors when exceeding the threshold of 1.5 mg/L.[Citation56,Citation61–64] Isovaleric acid was found in a single beer from the C. saturnus strain 4549 slightly above the odor threshold at a concentration of 1.77 mg/L. Diacetyl was above the threshold of 0.15 mg/L in four non-alcoholic beers. The values of the two beers from the C. fabianii yeast strains were significantly high at 0.48 mg/L (C. fab 5640) and 0.25 mg/L (C. fab 5650). The K. marxianus and S. fibuligera yeast strains also produced diacetyl quantities above the odor threshold at values of 0.26 mg/L (K. mar 653) and 0.21 mg/L (S. fib Lu27). Acetaldehyde was detected above the threshold in six beers. The highest value, which was more than 2.5 times the threshold value, was reached by K. lactis G9K. With values ranging from about 10.0 to 14.5 mg/L, beers produced using C. fabianii 5640 and 5650, C. misumaiensis CM1, and K. servazzii 3C4 also exceeded the acetaldehyde threshold. The T. delbrueckii yeast strain 1I6 even produced about 19.5 mg/L acetaldehyde. Saccharomyces yeasts are known to degrade both diacetyl and acetaldehyde during the maturation phase.[Citation65] Whether this also applies to the non-Saccharomyces yeast strains in this study would have to be verified, provided that a stronger diacetyl and acetaldehyde degradation is targeted. Since the cold storage of the non-alcoholic beers lasted for only 72 h after the six-day fermentation, the maturation phase was very short.
Although no further secondary metabolites were above the literature-based flavor thresholds, it is important to note that these thresholds refer to regular beers with alcohol. As shown in the heat map (cf ), the higher alcohols were well below the thresholds without exception, as expected for non-alcoholic beers. The organic acids hardly had any influence. Therefore, it can be assumed that the total esters already have a perceptible influence on the overall flavor of the respective beers at lower concentrations. According to this assumption, 2-phenylethyl acetate seems to have a noticeable influence on the overall flavor although the substance was measured only in smaller quantities below the odor threshold. In particular, the yeast K. servazzii 3C4 produced about 2.2 mg/L of 2-phenylethyl acetate, approaching the known threshold of 3.8 mg/L.[Citation56] The three investigated C. saturnus yeast strains, as well as the K. lactis G9K, the P. kluyveri PK1, and the K. marxianus 653, were also able to produce between 0.35 and 1.30 mg/L of the fruity ester, which is linked to floral, honey, and apple flavor impressions.[Citation66,Citation67] The following sensory evaluations were to investigate this assumption in more detail.
The compound 4-VG, with its clove-like flavor,[Citation68] was either not produced by any of the yeasts or only in concentrations below the limit of detection, and DMS was only produced at low concentrations below the odor threshold.
Sensory evaluation
First, all beers were evaluated to determine whether they were identified as fruity by the tasters. For this purpose, the paired test method according to MEBAK sensory analysis 3.1.1. and DIN EN ISO 5495:2007 was applied with a significance level α = 0.05. To achieve a significantly fruity result, nine of the ten tasters had to identify the beer as generally fruity. The results are depicted in .
Table 6. Number of sensory assessors (n = 10) identifying the beers produced with the 16 different non-Saccharomyces yeast strains as “fruity”.
From the total of 16 beers, twelve beers were identified by the tasters as significantly fruity. These included, without exception, all seven beers from the Cyberlindnera yeast strains, the two beers produced with the Kluyveromyces yeast strains, and the three beers from L. klu 3082, S. fib Lu27, and S. pom G10S. Although four beers were classified as non-significantly fruity using the paired test method, 80% of the tasters nevertheless identified a fruity flavor in three beers (K. ser 3C4, P. klu PK1, S. lud SL17), while only one beer, namely the beer produced from T. del 1I6, was described as fruity by just 70% of the tasters. The results reveal that the four beers that were evaluated as non-significantly fruity had a more weakly pronounced fruit character. The individual attributes for all beers are shown in the spiderwebs in and .
Figure 8. Aroma profiles of the beers fermented with the seven different Cyberlindnera yeast strains C. saturnus CSa1, 247, 4549, C. misumaiensis CM1, 238 and C. fabianii 5650, 5640.
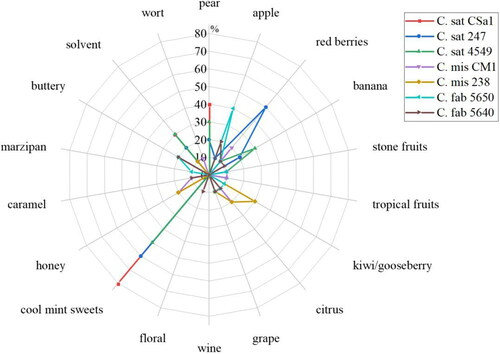
Due to the large number of yeast strains studied, the tastings of the beers were carried out on two different days. First, all beers produced with the seven Cyberlindnera yeast strains were evaluated. The flavor profiles are depicted as spiderwebs in . The individual spiderwebs can be found in Supplemental File 4.
The dominant cool mint sweets flavor is striking and was perceived by 50-80% of the tasters for all three C. saturnus yeast strains. Between 20 and 40% of the tasters additionally detected pear and banana flavors, as well as a solvent-like aroma. The tasters noted a clear difference between the three beers in 247, as 50% of the tasters perceived a red berry flavor, which was detected by only 10% of the tasters in 4549 and by none in CSa1. With the exception of the red berry flavor, the other attributes can be traced back to the high concentrations of isoamyl acetate, which were well above the odor threshold in the beers (cf ). To establish which secondary metabolites cause the red berry flavor would require an analysis of all volatile components, which was not conducted as part of these investigations.
No distinct aromas could be identified in the two beers of the C. misumaiensis yeast strains. The beer produced with C. mis 238 was most strongly reminiscent of kiwi and gooseberries with slight hints of citrus and honey, whereas the C. mis CM1 beer was perceived as non-specifically fruity with a slight honey-caramel character. Although acetaldehyde was above the threshold of 10 mg/L in the case of CM1, the assessors did not mention any green or apple-like impression. The fact that non-specific fruity flavors were perceived could be explained by the low concentrations of fruit esters. The conceivably lower threshold values could result in the total esters contributing to a slightly fruity character. Accordingly, these two yeast strains would be suitable for producing more neutral non-alcoholic beers with subtle fruity flavors.
The two C. fabianii yeast strains appeared to produce similar flavors. Apple flavors and buttery aromas dominated, which can be attributed to acetaldehyde and diacetyl (cf ). Nevertheless, these two beers were also perceived as non-specifically fruity, which would suggest that here, too, the sum of the esters has a fruity influence on the overall flavor character of the beers.
In , the flavor profiles of the further nine beers are depicted. The individual spiderwebs can be found in Supplemental File 4.
Figure 9. Aroma profiles of the beers fermented with the reference yeast strain S. ludwigii SL17 and the investigated yeast strains K. servazzii 3C4, K. lactis G9K, K. marxianus 653, L. kluyveri 3082, P. kluyveri PK1, S. fibuligera Lu27, S. pombe G10S, and T. delbrueckii 1I6.
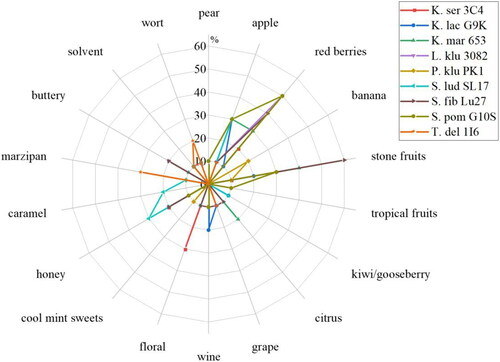
While the beer from K. servazzii 3C4 was perceived as floral and honey-like, possibly due to a lower threshold of 2-phenylethyl acetate, red berries were additionally detected by 20% of the tasters. Although acetaldehyde was analyzed in concentrations above the threshold, none of the assessors detected an apple-like flavor. It should be noted here that only 80% of tasters identified the beer as generally fruity, so it is classified as non-significant (cf ). In contrast, an apple flavor was perceptible in the beer from K. lactis G9K, which could be due to acetaldehyde as well as 2-phenylethyl acetate. Otherwise, the beer was rated as rather non-specifically but significantly fruity (cf ).
The beers made from K. marxianus 653 as well as S. fibuligera Lu27 were both described as slightly buttery, which can be attributed to the diacetyl value being above the odor threshold. Both beers were reminiscent of red berry, honey, stone fruit, and apple flavors. However, the apple flavor was more evident with K. marxianus 653 (possibly due to the presence of 2-phenylethyl acetate), while Lu27 had more red berry and especially stone fruit flavors. The stone fruit aroma showed a clear expression of plum (data not shown).
Although no flavor components above the odor thresholds were measured in the beers from S. pombe G10S, L. kluyveri 3082 or in the reference yeast strain S. ludwigii SL17 in the GC/MS analysis (cf ), the three beers exhibited distinct flavor characteristics in the sensory evaluation. Consistent with descriptions from the literature, the beer S. lud SL17 was described as having honey, apple, and also caramel-like flavors.[Citation69,Citation70] Even though the threshold value of 2-phenylethanol was not exceeded, only the beer produced from the S. ludwigii showed this flavor substance with honey and floral notes at a concentration of 6.6 mg/L. This could explain why the beer was perceived as less fruity than most of the other evaluated beers. The profiling of the beers S. pom G10S and L. klu 3082 showed strong similarities. Half of the tasters described red berry flavors for both beers, and 30% of the tasters additionally identified stone fruit flavors. An apple flavor was additionally described, although it was more prominent in the S. pom G10S beer. One difference between the two beers was in the honey-buttery flavors exhibited by the beer made from L. kluyveri 3082. The diacetyl value was measured at 0.08 mg/L and was thus below the threshold value of 0.1 mg/L. However, due to the generally low concentration of secondary metabolites, a buttery flavor could possibly be perceived by tasters who were sensitive to diacetyl.
The two beers from P. kluyveri PK1 and T. delbrueckii 1I6 were rated as relatively neutral. This explains why the two beers were not perceived as significantly fruity. Only a few tasters recognized the isoamyl acetate analyzed in the P. klu PK1 beer (3.43 mg/L) as banana, cool mint sweets, and solvent. The beer T. del 1I6 was also described as neutral, however, the tasters detected marzipan and wort-like flavors. These are probably due to aldehydes present in the malt extract or wort, which were enhanced by boiling. Many yeasts are capable of degrading the aldehydes during fermentation, which T. delbrueckii 1I6 obviously did not. Although acetaldehyde was analyzed at twice the threshold level, only 10% of the tasters indicated an apple-like aroma. Accordingly, the wort-like flavors seemed to predominate.
Comparable to the beer fermented with the C. saturnus yeast strain 247, L. kluyveri 3082, S. pombe G10S, and S. fibuligera Lu27 in particular showed red berry flavors. As mentioned above, further volatile components would have to be analyzed to detect the substances responsible for this kind of flavor. Additionally, there may also be a synergistic effect from different flavor compounds. Furthermore, some beers exhibited a honey flavor that could not be clearly attributed to the analyzed secondary metabolites. It is possible that the honey aroma was caused by phenylacetaldehyde, which was not quantified in this study.[Citation71]
Looking at the biplot in with the principal components PC 1 and PC 2 as a result of principal component analysis, it can be seen that only 55% of the variation in the data is captured by the first two principal components. Therefore, only slightly more than half of the available information is included in the biplot. Accordingly, PC 3 and PC 4 were also included in the analysis to account for over three-quarters (77.09%) of the data. The full data can be found in Supplemental File 5.
Figure 10. Principal Component Analysis (PCA) biplot with two principal components PC 1 and PC 2 showing the correlation of the beers produced with the 16 investigated yeast strains and the specific volatile compounds measured analytically as well as the individual flavor characteristics described by the tasters.
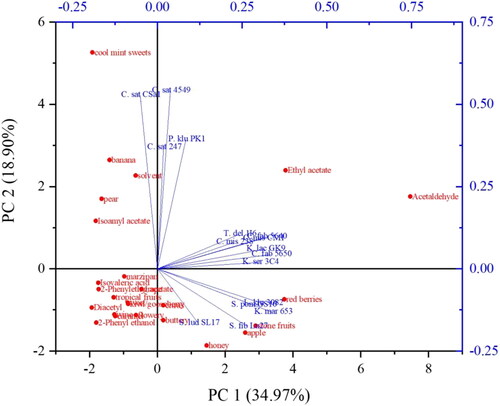
Considering the loading plot in , the three beers produced with the Cyberlindnera saturnus yeast strains as well as the P. klu PK1 have a positive correlation with each other. They are directly correlated with PC 2, which results from the flavor impressions cool mint sweets, banana, pear, and solvent perceived by the tasters. While isoamyl acetate correlates with the aforementioned flavor impressions, the correlation with ethyl acetate, which has a solvent-like aroma, is lower according to the PCA plot. Based on PC 1, the PCA plot shows that the beers produced with the yeast strains K. mar 653, L. klu 3082, S. pom GS10, and S. fib Lu27 exhibit red berry, stone fruit, and apple flavors, while the beer from the reference yeast strain S. lud SL17 shows next to apple-like more a honey-like flavor. When PC 1 and PC 3 are correlated with each other in the principal component analysis (cf Supplemental File 5), this correlation can be confirmed. However, the loading plot of PC 1 and PC 3 shows that there is only a positive correlation between K. mar 653, L. klu 3082, S. pom GS10, and S. fib Lu27, but not with S. lud SL17. By depicting PC 4, a correlation can be seen between the beers from the yeast strains C. mis 238 and C. mis CM1, with the flavor impressions kiwi/gooseberry as well as citrus. Apart from the distinct correlation of isoamyl acetate with the flavor impressions of cool mint sweets, banana, and pear, no further correlations between analyzed volatile components and tasted flavors can be statistically verified. This illustrates that further volatile components need to be analyzed in order to be able to explain the flavor characteristics perceived by the tasters and that even synergistic effects of certain volatiles may occur.
shows the total DLG score according to the DLG evaluation scheme.[Citation17] Since the beers were unhopped and fermentation was unpressurized, the quality of bitterness and carbonation ratings were excluded from the evaluation. The DLG evaluation scheme is primarily intended to detect sensory product faults. A score of 4.0 designates the product as good, with 5.0 being the top rating.
Table 7. Total DLG score according to the DLG evaluation scheme excluding the quality of bitterness as well as carbonation of the 15 investigated yeast strains and the reference yeast strain S. ludwigii SL17.
All beers were rated between 4.0 and 5.0, indicating a consistently good product quality. In a comparison of the 16 beers, those fermented with the C. saturnus yeast strains C. sat 247 and C. sat 4549 stood out with the best ratings at 4.54 each, closely followed by C. sat CSa1. Although the threshold value of isovaleric acid was slightly exceeded in the 4549 beer, no negative sensory influence was described. The high concentration of isoamyl acetate (5.23 mg/L), which is known to cause a masking effect, was most probably responsible for masking the sensory effect of isovaleric acid.[Citation59] The reference yeast S. lud SL17 with a rating of 4.16 was to the lower end of the middle range. While the buttery flavor from diacetyl and the apple-like flavor from acetaldehyde were rated as positive in the beers produced with the yeast strains C. fabianii 5650, K. marxianus 653, and S. fibuligera Lu27, other beers, such as the L. klu 3082, were devalued by it. Nevertheless, it is known from previous studies that diacetyl in particular does not necessarily lead to negative flavor impressions.[Citation25] This illustrates that the threshold values of individual flavor components can be evaluated, however, the overall flavor profile determines how harmoniously the individual flavors interact.
Conclusion
In summary, the 15 yeast strains investigated are suitable for producing non-alcoholic beers. Although not all yeast strains are maltose-negative during fermentation, as S. fibuligera Lu27 and the two C. fabianii yeast strains 5640 and 5650 possess the ability to fundamentally metabolize maltose, the activity at 20 °C is low. Therefore, the ethanol contents remain below 0.5% (v/v) and stay within the German regulation for non-alcoholic beers during a six-day fermentation period at an original wort of 7°P. For the two yeast strains L. kluyveri 3082 and T. delbrueckii 1I6, the original wort should be adjusted in a reducing manner to remain safely within the alcohol-free range.
The aim of producing non-alcoholic beers with desirable flavor characteristics but without wort and phenolic off-flavors during fermentation was achieved, as a wide range of novel aromas were detected during sensory evaluation. The non-alcoholic beers produced with the three C. saturnus yeast strains (C. sat 247, C. sat 4549, C. sat CSa1) were particularly impressive with cool mint sweets, pear, and in some cases red berry flavors. There were hardly any sensory flaws in the beers fermented with the yeasts K. marxianus 653 and S. fibuligera Lu27, which displayed a broad flavor diversity of red berry, honey, stone fruit, and apple. Only one yeast stood out with less desirable wort flavors, namely T. delbrueckii 1I6. Many of the secondary metabolites analyzed were below the flavor threshold values known for beer from the literature despite the sensory perception of high flavor diversity. However, since the thresholds relate to regular alcoholic beers, masking effects due to ethanol or certain secondary metabolites can be assumed to be the reason. In addition, GC/MS analysis would need to be extended to include further volatile flavor compounds to obtain a complete picture of which secondary metabolites are responsible for the overall flavor of the analyzed non-alcoholic beers.
Supplemental Material
Download MS Word (6 MB)Disclosure statement
No potential conflict of interest was reported by the authors.
Additional information
Funding
Literature cited
- Ahuja, K.; Rawat, A. Market Size of Non-Alcoholic Beer Worldwide from 2016 to 2024 (in Billion U.S. Dollars). 2020. https://www.gminsights.com/industry-analysis/non-alcoholic-beer-market. (accessed Jun 17, 2021).
- Bellut, K.; Arendt, E. K. Chance and Challenge: Non-Saccharomyces Yeasts in Nonalcoholic and Low Alcohol Beer Brewing – A Review. J. Am. Soc. Brew. Chem. 2019, 77, 77–91. DOI: 10.1080/03610470.2019.1569452.
- Methner, Y.; Hutzler, M.; Matoulková, D.; Jacob, F.; Michel, M. Screening for the Brewing Ability of Different Non-Saccharomyces Yeasts. Fermentation 2019, 5, 101. DOI: 10.3390/fermentation5040101.
- Stewart, G. G. The Horace Brown Medal Lecture: Forty Years of Brewing Research. J. Inst. Brew. 2009, 115, 3–29. DOI: 10.1002/j.2050-0416.2009.tb00340.x.
- Gotsick, J. T.; Benson, R. F. HPLC Analysis of Carbohydrates Important to Beer Brewing Using an Aminopropyl Stationary Phase. J. Liq. Chromatogr. 1991, 14, 1887–1901.DOI: 10.1080/01483919108049660.
- Harris, G.; Thompson, C. C. The Uptake of Nutrients by Yeasts III. The Maltose Permease of a Brewing Yeast. Biochim. Biophys. Acta 1961, 52, 176–183. DOI: 10.1016/0006-3002(61)90915-5.
- Haehn, H.; Glaubitz, M. Beer Manufacture: Patent US1898047 A. 1933.
- Kunz, T.; Methner, F.-J. Verfahren Zur Herstellung Eines Getränkes. (EP2382303A1). 2009.
- Blanco, C. A.; Andrés-Iglesias, C.; Montero, O. Low-Alcohol Beers: Flavor Compounds, Defects, and Improvement Strategies. Crit. Rev. Food Sci. Nutr. 2016, 56, 1379–1388. DOI: 10.1080/10408398.2012.733979.
- Liu, S.-Q.; Quek, A. Y. H. Evaluation of Beer Fermentation with a Novel Yeast Williopsis saturnus. Food Technol. Biotechnol. 2016, 54, 403–412. DOI: 10.17113/ftb.54.04.16.4440.
- Bellut, K.; Michel, M.; Zarnkow, M.; Hutzler, M.; Jacob, F.; Atzler, J. J.; Hoehnel, A.; Lynch, K. M.; Arendt, E. K. Screening and Application of Cyberlindnera Yeasts to Produce a Fruity, Non-Alcoholic Beer. Fermentation 2019, 5, 103. DOI: 10.3390/fermentation5040103.
- van Rijswijck, I. M. H.; van Mastrigt, O.; Pijffers, G.; Wolkers-Rooijackers, J. C. M.; Abee, T.; Zwietering, M. H.; Smid, E. J. Dynamic Modelling of Brewers’ Yeast and Cyberlindnera fabianii Co-Culture Behaviour for Steering Fermentation Performance. Food Microbiol. 2019, 83, 113–121. DOI: 10.1016/j.fm.2019.04.010.
- Bellut, K.; Michel, M.; Zarnkow, M.; Hutzler, M.; Jacob, F.; Schutter, D.; de; Daenen, L.; Lynch, K.; Zannini, E.; Arendt, E. Application of Non-Saccharomyces Yeasts Isolated from Kombucha in the Production of Alcohol-Free Beer. Fermentation 2018, 4, 66. DOI: 10.3390/fermentation4030066.
- Capece, A.; Fusco, D d.; Pietrafesa, R.; Siesto, G.; Romano, P. Performance of Wild Non-Conventional Yeasts in Fermentation of Wort Based on Different Malt Extracts to Select Novel Starters for Low-Alcohol Beers. Appl. Sci. 2021, 11, 801. DOI: 10.3390/app11020801.
- Holt, S.; Mukherjee, V.; Lievens, B.; Verstrepen, K. J.; Thevelein, J. M. Bioflavoring by Non-Conventional Yeasts in Sequential Beer Fermentations. Food Microbiol. 2018, 72, 55–66. DOI: 10.1016/j.fm.2017.11.008.
- Michel, M.; Kopecká, J.; Meier-Dörnberg, T.; Zarnkow, M.; Jacob, F.; Hutzler, M. Screening for New Brewing Yeasts in the Non-Saccharomyces Sector with Torulaspora delbrueckii as Model. Yeast (Chichester, England) 2016, 33, 129–144. DOI: 10.1002/yea.3146.
- Gutiérrez, A.; Boekhout, T.; Gojkovic, Z.; Katz, M. Evaluation of Non‐Saccharomyces Yeasts in the Fermentation of Wine, Beer and Cider for the Development of New Beverages. J. Inst. Brew. 2018, 124, 389–402. DOI: 10.1002/jib.512.
- Saerens, S.; Swiegers, J. H. Production of Low-Alcohol or Alcohol-Free Beer with pichia kluyveri Yeast Strains. Google Patents, 2016.
- Vaštík, P.; Šmogrovičová, D.; Kafková, V.; Sulo, P.; Furdíková, K.; Špánik, I. Production and Characterisation of Non-Alcoholic Beer Using Special Yeast. Kvasný Průmysl/Ferment. Ind. 2020, 66, 336–344. DOI: 10.18832/kp2019.66.336.,
- Johansson, L.; Nikulin, J.; Juvonen, R.; Krogerus, K.; Magalhães, F.; Mikkelson, A.; Nuppunen-Puputti, M.; Sohlberg, E.; de Francesco, G.; Perretti, G.; Gibson, B. Sourdough Cultures as Reservoirs of Maltose-Negative Yeasts for Low-Alcohol Beer Brewing. Food Microbiol. 2021, 94, 103629. DOI: 10.1016/j.fm.2020.103629.
- Meier-Dörnberg, T.; Schneiderbanger, H.; Jacob, F.; Hutzler, M. Alcohol-Free Wheat Beer with Maltose Negative Yeast Strain Saccharomycodes ludwigii. Young Scientists Symposium on Malting, Brewing and Distilling. 2014. DOI: 10.13140/RG.2.2.26169.36968.
- De Francesco, G.; Turchetti, B.; Sileoni, V.; Marconi, O.; Perretti, G. Screening of New Strains of Saccharomycodes ludwigii and Zygosaccharomyces rouxii to Produce Low-Alcohol Beer. J. Inst. Brew. 2015, 121, 113–121. DOI: 10.1002/jib.185.,
- Pahl, R. Modern Production of Alcohol Free Beers for Varying Breweries and Beer Styles. 12th Trends in Brewing. Ghent, April 3-7, 2016.
- Parise, A.; Kovaleva, E. G.; Glukhareva, T. V.; Shatunova, S. A. Biotechnological Production of Non-Traditional Beer. In AIP Conference Proceedings (Online). 2017 DOI: 10.1063/1.5002995.
- Callejo, M. J.; García Navas, J. J.; Alba, R.; Escott, C.; Loira, I.; González, M. C.; Morata, A. Wort Fermentation and Beer Conditioning with Selected Non-Saccharomyces Yeasts in Craft Beers. Eur. Food Res. Technol. 2019, 245, 1229–1238. DOI: 10.1007/s00217-019-03244-w.
- Perpète, P.; Collin, S. Contribution of 3-Methylthiopropionaldehyde to the Worty Flavor of Alcohol-Free Beers. J. Agric. Food Chem. 1999, 47, 2374–2378. DOI: 10.1021/jf9811323.
- Perpète, P.; Collin, S. Influence of Beer Ethanol Content on the Wort Flavour Perception. Food Chem. 2000, 71, 379–385. DOI: 10.1016/s0308-8146(00)00179-5.
- Müller, M.; Bellut, K.; Tippmann, J.; Becker, T. Physical Methods for Dealcoholization of Beverage Matrices and Their Impact on Quality Attributes. ChemBioEng Rev. 2017, 4, 310–326. DOI: 10.1002/cben.201700010.
- Back, W.; Bohak, I. Ausgewählte Kapitel Der Brauereitechnologie, 2. aktualisierte Aufl.; Carl: Nürnberg, 2008.
- Hutzler, M.; Methner, Y.; Jacob, F. Spezialhefen für alkoholfreie und alkoholarme Biere. 2021, 25–26, 634–637.
- Esslinger, H. M. Handbook of Brewing: Processes, Technology, Markets; John Wiley & Sons: Weinheim, Germany, 2009.
- Bleyer, B.; Bames, E.; Büttner, G.; Diemair, W.; Holthöfer, H.; Reichard, O.; Vogt, E.; Bömer, A.; Juckenack, A.; Tillmans, B. Alkoholische Genussmittel; Springer: Berlin Heidelberg, 2013.
- Nikulin, J.; Eerikäinen, R.; Hutzler, M.; Gibson, B. Brewing Characteristics of the Maltotriose-Positive Yeast Zygotorulaspora Florentina Isolated from Oak. Beverages 2020, 6, 58. DOI: 10.3390/beverages6040058.
- Honda, S.; Akao, E.; Suzuki, S.; Okuda, M.; Kakehi, K.; Nakamura, J. High-Performance Liquid Chromatography of Reducing Carbohydrates as Strongly Ultraviolet-Absorbing and Electrochemically Sensitive 1-Phenyl-3-Methyl5-Pyrazolone Derivatives. Anal. Biochem. 1989, 180, 351–357. DOI: 10.1016/0003-2697(89)90444-2.
- Hildebrandt, G.; Schneider-Haeder, B. Sensorische Analyse: Methodenüberblick und Einsatzbereiche – DLG-Sensorik, 2009. www.dlg.org.
- Narziß, L.; Back, W.; Miedaner, H.; Lustig, S. Untersuchung Zur Beeinflussung Der Geschmacksstabilität Durch Variation Technologischer Parameter Bei Der Bierherstellung. Monatsschrift Für Brauwissenschaft 1999, 1999, 192–206.
- Boundy-Mills, K.; Stratford, M.; Miller, M. W. Chapter 62 - Saccharomycodes E.C. Hansen (1904). In The Yeasts: A Taxonomic Study, 5th ed.; Kurtzman, C. P., Ed.; Elsevier: Amsterdam, 2011; pp 747–750.
- Lachance, M.-A. Chapter 35 - Kluyveromyces Van Der Walt (1971). In The Yeasts: A Taxonomic Study, 5th ed.; Kurtzman, C. P., Ed.; Elsevier: Amsterdam, 2011; pp 471–481.
- Berthels, N. J.; Cordero Otero, R. R.; Bauer, F. F.; Thevelein, J. M.; Pretorius, I. S. Discrepancy in Glucose and Fructose Utilisation during Fermentation by Saccharomyces cerevisiae Wine Yeast Strains. FEMS Yeast Res. 2004, 4, 683–689. DOI: 10.1016/j.femsyr.2004.02.005.
- Kurtzman, C. P. Chapter 75 - Torulaspora Lindner (1904). In The Yeasts: A Taxonomic Study, 5th ed.; Kurtzman, C. P., Ed.; Elsevier: Amsterdam, 2011; pp 867–874
- Lachance, M.-A.; Kurtzman, C. P. Chapter 41 - Lachancea Kurtzman (2003): Lachancea Kluyveri (Phaff, M.W. Miller & Shifrine) Kurtzmann (2003). In The Yeasts: A Taxonomic Study, 5th ed.; Kurtzman, C. P., Ed.; Elsevier: Amsterdam, 2011; pp 514–515.
- Kurtzman, C. P. Chapter 42 - Lindnera Kurtzman, Robnett & Basehoar-Powers (2008). In: The Yeasts: A taxonomic study, 5th ed. Kurtzman, C. P., Ed.; Elsevier: Amsterdam, 2011; pp 521–543.
- Kulshrestha, S.; Tyagi, P.; Sindhi, V.; Yadavilli, K. S. Invertase and Its Applications – A Brief Review. J. Pharm. Res. 2013, 7, 792–797. DOI: 10.1016/j.jopr.2013.07.014.
- Suzuki, H.; Ozawa, Y.; Maeda, H. Studies on the Water-Insoluble Enzyme. Agric. Biol. Chem. 1966, 30, 807–812. DOI: 10.1080/00021369.1966.10858683.
- Kurtzman, C. P. Chapter 57 - Pichia E.C. Hansen (1904): Pichia Kluyveri Bedford Ex Kudryavtsev (1960). In The Yeasts: A Taxonomic Study, 5th ed.; Kurtzman, C. P., Ed.; Elsevier: Amsterdam, 2011; pp 695–696.
- Vaughan-Martini, A.; Martini, A. Chapter 66 - Schizosaccharomyces Lindner (1893): Schizosaccharomyces pombe Lindner (1893). In The Yeasts: A Taxonomic Study, 5th ed. Kurtzman, C. P., Ed.; Elsevier: Amsterdam, 2011; pp 782–784.
- Kurtzman, C. P. Chapter 42 - Lindnera Kurtzman, Robnett & Basehoar-Powers (2008): Lindnera misumaiensis (Sasaki & Yoshida Ex Kurtzman, Robnett & Basehoar-Powers). In The Yeasts: A Taxonomic Study, 5th ed.; Kurtzman, C. P., Ed.; Elsevier: Amsterdam, 2011; pp 534–535.
- Vaughan-Martini, A.; Lachance, M.-A.; Kurtzman, C. P. Chapter 34 - Kazachstania Zubkova (1971): Kazachstania servazzii (Capriotti) Kurtzman (2003). In The Yeasts: A Taxonomic Study, 5th ed. Kurtzman, C. P., Ed.; Elsevier: Amsterdam, 2011; pp 439–470.
- Wickerham, L. J. Evidence of the production of extracellular invertase by certain strains of yeasts. Archives of Biochemistry and Biophysics 1958, 76, 439-448. DOI: 10.1016/0003-9861(58)90169-3.
- Kurtzman, C. P.; Smith, M. T. Chapter 63 - Saccharomycopsis Schiönning (1903): Saccharomycopsis fibuligera (Lindner) Klöcker (1924). In The Yeasts: A Taxonomic Study, 5th ed.; Kurtzman, C. P., Ed.; Elsevier: Amsterdam, 2011; pp 755–756.
- Chen, L.; Chi, Z.-M.; Chi, Z.; Li, M. Amylase Production by Saccharomycopsis fibuligera A11 in Solid-State Fermentation for Hydrolysis of Cassava Starch. Appl. Biochem. Biotechnol. 2010, 162, 252–263. DOI: 10.1007/s12010-009-8744-3.
- Futatsugi, M.; Ogawa, T.; Fukuda, H. Purification and Properties of Two Forms of Glucoamylase From Saccharomycopsis fibuligera. J. Ferment. Bioeng. 1993, 76, 521–523. DOI: 10.1016/0922-338X(93)90252-4.
- Hostinová, E. Amylolytic Enzymes Produced by the Yeast Saccharomycopsis fibuligera. BIOLOGIA-BRATISLAVA 2002, 57, 247–252.
- Fukuhara, H. The Kluyver Effect Revisited. FEMS Yeast Res. 2003, 3, 327–331. DOI: 10.1016/S1567-1356(03)00112-0.,
- Kluyver, A. J.; Custers, M. T. J. The Suitability of Disaccharides as Respiration and Assimilation Substrates for Yeasts Which Do Not Ferment These Sugars. Antonie Van Leeuwenhoek 1939, 6, 121–162. DOI: 10.1007/BF02146183.
- Meilgaard, M. C. Flavor Chemistry of Beer. II. Flavor and Threshold of 239 Aroma Volatiles. Tech. Quart. Master. Brew. Assoc. Am. 1975, 12, 151–168.
- Sannino, C.; Mezzasoma, A.; Buzzini, P.; Turchetti, B. Non-Conventional Yeasts for Producing Alternative Beers. In Non-Conventional Yeasts: From Basic Research to Application; Sibirny, A., Ed.; Springer International Publishing: Cham, 2019; pp 361–388.
- Schieberle, P. Primary Odorants of Pale Lager Beer. Z Lebensm. Unters. Forch. 1991, 193, 558–565. DOI: 10.1007/BF01190873.
- Saison, D.; Schutter, D. P.; de; Uyttenhove, B.; Delvaux, F.; Delvaux, F. R. Contribution of Staling Compounds to the Aged Flavour of Lager Beer by Studying Their Flavour Thresholds. Food Chem. 2009, 114, 1206–1215. DOI: 10.1016/j.foodchem.2008.10.078.
- Collin, S.; Derdelinckx, G.; Dufour, J.-P. Relationships between the Chemical Composition and Sensory Evaluation of Lager Beers. Food Qual. Preference 1994, 5, 145–149. DOI: 10.1016/0950-3293(94)90021-3.
- Gernat, D. C.; Brouwer, E.; Ottens, M. Aldehydes as Wort off-Flavours in Alcohol-Free Beers—Origin and Control. Food Bioprocess Technol. 2020, 13, 195–216. DOI: 10.1007/s11947-019-02374-z.
- Kishimoto, T.; Wanikawa, A.; Kono, K.; Shibata, K. Comparison of the Odor-Active Compounds in Unhopped Beer and Beers Hopped with Different Hop Varieties. J. Agric. Food Chem. 2006, 54, 8855–8861. DOI: 10.1021/jf061342c.
- Olšovská, J.; Vrzal, T.; Štěrba, K.; Slabý, M.; Kubizniaková, P.; Čejka, P. The Chemical Profiling of Fatty Acids during the Brewing Process. J. Sci. Food Agric. 2019, 99, 1772–1779. DOI: 10.1002/jsfa.9369.
- Witrick, K. Comparison of the Flavor and Aroma Compounds Present in Aging Lambic Beer; ASBC Meeting, Fort Myers, Florida, 2017. https://www.asbcnet.org/events/archives/2017ASBCMeeting/proceedings/2017Proceedings/10_Witrick.pdf.
- Narziss, L.; Back, W.; Gastl, M.; Zarnkow, M. Abriss Der Bierbrauerei, 8th ed.; John Wiley & Sons Incorporated: Newark, 2017.
- Fritsch, H. T.; Schieberle, P. Identification Based on Quantitative Measurements and Aroma Recombination of the Character Impact Odorants in a Bavarian Pilsner-Type Beer. J. Agric. Food Chem. 2005, 53, 7544–7551. DOI: 10.1021/jf051167k.
- Kobayashi, M.; Shimizu, H.; Shioya, S. Beer Volatile Compounds and Their Application to Low-Malt Beer Fermentation. J. Biosci. Bioeng. 2008, 106, 317–323. DOI: 10.1263/jbb.106.317.
- Schwarz, K. J.; Stübner, R.; Methner, F.-J. Formation of Styrene Dependent on Fermentation Management during Wheat Beer Production. Food Chem. 2012, 134, 2121–2125. DOI: 10.1016/j.foodchem.2012.04.012.
- Hutzler, M.; Zarnkow, M.; Hans, S.; Stretz, D.; Meier-Dörnberg, T.; Methner, Y.; Schneiderbanger, H.; Jacob, F. New Yeasts - New Beers. Brewing and Beverage Industry International, 2020, pp 16–23.
- Romano, P.; Marchese, R.; Laurita, C.; Saleano, G.; Turbanti, L. Biotechnological Suitability of Saccharomycodes ludwigii for Fermented Beverages. World Journal of Microbiology and Biotechnology 1999, 15, 451–454. DOI: 10.1023/A:1008948623024.
- Schieberle, P.; Komarek, D. Changes in Key Aroma Compounds during Natural Beer Aging. In Freshness and Shelf Life of Foods; Cadwallader, K. R., Weenen, H., Eds.; American Chemical Society: Washington, DC, 2003; pp 70–79.