Abstract
Fusarium tricinctum is among the pathogenic fungi that contaminate cereal crops, including barley. It produces toxic metabolites such as enniatins (ENNs) that affect the quality and safety of barley-based products such as malt and beer, thus representing a significant concern for the cereal industries. Therefore, it is critical to reduce Fusarium spp. development and enniatin contamination in cereal-based products. Fungicides are commonly used during agricultural practices, but they are facing growing fungal resistance and environmental and health concerns. Hence, there is need to define environmentally friendly control strategies. This study highlights the ability of a bacterial strain isolated from barley kernels, namely Erwinia gerundensis 6E4, to inhibit the growth of Fusarium tricinctum and to reduce its toxigenic potential. First, E. gerundensis 6E4 exhibited a significant fungistatic activity against pathogenic fungal strains during co-cultures, with fungal growth reduced by up to 100% on Petri dishes and by about 69% in liquid medium. The bacterial strain also led to a drastic reduction (74 to 100%) of ENNs production by F. tricinctum strains over 72 h co-cultures. Based on these results, our study supports the use of E. gerundensis 6E4 as a biocontrol agent in strategies aiming at reducing the presence of Fusarium tricinctum and enniatins in cereal-based products, or as a food and feed supplement for the bio-detoxification of enniatins.
Introduction
The microbial community of barley is made up of a wide range of microorganisms, including yeasts, bacteria, and filamentous fungi.[Citation1–3] The filamentous fungi include, among others, several pathogenic fungal species, such as Fusarium spp., that represent a generic problem for cereals, as they cause severe damage and considerable crop losses that account for a loss of about 10% of global food production.[Citation4] They can also cause several carcinogenic, genotoxic, hepatotoxic, mutagenic, nephrotoxic, and teratogenic diseases in humans and animals due to their natural production of toxic secondary metabolites known as mycotoxins.[Citation5,Citation6] The roles of mycotoxins have not yet been fully elucidated, but they are thought to protect the fungi against insects, plants, and other microorganisms. Due to their natural thermostability, mycotoxins can withstand thermal treatments during transformation processes, consequently representing a significant risk for many cereal industries including baking, malting, and brewing. As such, research efforts have been made over the past few years towards reducing the levels of mycotoxins in agricultural commodities.[Citation7–11]
Currently, there are approximately 400 compounds identified as mycotoxins, among which 30 have received a great deal of attention due to their threat to animal or human health.[Citation12] The most important compounds include aflatoxins and ochratoxins from Aspergillus, Fusarium mycotoxins (deoxynivalenol, nivalenol, T2 toxin and HT2 toxins, fumonisins, and zearalenone), and ochratoxins and patulin from Penicillium.[Citation6,Citation11,Citation13] The majority of the mycotoxins cited above are regulated in many countries, including EU countries under the regulation EC N°1881/2006 that sets their maximum authorized levels in foodstuffs.[Citation14,Citation15] However, studies conducted on mycotoxins over the last decade have highlighted the occurrence of a number of mycotoxins referred to as “emerging mycotoxins”.[Citation16–18] This group includes mycotoxins that are neither routinely determined nor legislatively regulated, such as beauvericin, monoliformin, fusaproliferin, and enniatins, the latter being the most prevalent of them.[Citation19] Enniatins can be found in a broad range of agricultural commodities including apple, coffee beans, sugar beet, and cereal grains, with cereals being the most commonly contaminated substrates,[Citation20] indicating that humans are continuously exposed to enniatins contamination through food. Scientists have recently drawn a map of enniatins occurrence in European cereal harvests and it clearly shows that there is a high frequency of enniatins contaminations, with high contamination levels exceeding 500,000 µg/kg for certain crops (wheat and maize).[Citation21]
Enniatins (ENNs) belong to the group of N-methylated cyclic hexadepsipeptides and consist of three residues of D-2-hydroxyisovaleric acid alternating with three N-methyl branched-chain L-amino acids, generally valine, leucine, and isoleucine.[Citation5,Citation22] They are biosynthesized through pathways that generate structurally related yet distinct compounds from each other by the nature of the N-methylamino acid residues. To date, about 29 ENNs have been characterized, among which, ENNs A, A1, B, and B1 are the most commonly detected in cereal grains.[Citation21] ENNs are produced by approximately 30 fungal species, including the toxigenic field fungi Fusarium tricinctum and Fusarium avenaceum that are reported as the major contributors to the contamination of cereal grains.[Citation21] Their production is catalyzed by enniatin synthetase (Esyn), a non-ribosomal multifunctional enzyme of 347-kDa polypeptide chain encoded by the esyn1 gene.[Citation23,Citation24] The enzyme synthesizes ENNs from their primary precursors (valine, leucine or isoleucine, D-2-hydroxyisovaleric acid, ATP, and S-adenosyl-methionine) through the thiotemplate mechanism that consists of a condensation of three dipeptidol groups units followed by cyclization, as shown in .[Citation21,Citation25] Many studies have been carried out regarding the occurrence of ENNs in various agricultural commodities and their cytotoxicity in different animal cell lines (porcine kidney cells, mouse macrophages), thus shedding light on their various effects such as cell death induced by apoptosis or necrosis, mitochondrial dysfunction, and reactive oxygen species production.[Citation17,Citation26–31]
Figure 1. Schematic model of the biosynthesis of ENNs A, A1, B, and B1; A1 and A2, adenylation domains; C1, C2, and C3, condensation domains; T1, T2a, and T2b, thiolation domains; and M, N-methyltransferase domain.[Citation21]
![Figure 1. Schematic model of the biosynthesis of ENNs A, A1, B, and B1; A1 and A2, adenylation domains; C1, C2, and C3, condensation domains; T1, T2a, and T2b, thiolation domains; and M, N-methyltransferase domain.[Citation21]](/cms/asset/c5422103-f236-4ff9-8135-4ef4b14c8565/ujbc_a_2041970_f0001_c.jpg)
Even though it is not possible to produce agricultural commodities that are completely free of all mycotoxin contaminations, it is important to reduce the presence of these contaminants in cereal harvests and to limit their development on cereal grains during subsequent transformation processes. For this purpose, there are several control methods that are currently applied, including agronomic control (crop residue management, choice of cultivar, crop rotation, appropriate sowing date and rate…), chemical control (use of fungicides), and control during storage.[Citation32] These methods are usually applied in combination in order to maximize their efficiency against fungal contaminations. However, it is important to point out that mycotoxin-producing Fusarium species are becoming increasingly resistant to some of these methods, thus rendering them more and more inefficient.[Citation33] Consumers also favor natural preservation methods, thus calling for the limitation of the use of fungicides.[Citation34] All of this has prompted research into alternative and complementary environmentally friendly methods of fungal contamination management. To date, several studies have focused on the use of antifungal microorganisms or natural compounds to inhibit fungal contamination in cereal-based products. One of the works in this context consists of using selected strains of the filamentous yeast Geotrichum candidum to inhibit the growth of pathogenic microorganisms such as Fusarium spp. during the malting process, where barley kernels (or other cereal grains) are germinated under controlled conditions to obtain the malt that is used to produce beer.[Citation35] The yeast is known to produce antimicrobial metabolites such as indoleacetic acid, phenylethyl alcohol, and phenyllactic acid,[Citation35] the latter having been recently proven to be an effective antifungal compound and a potential biocontrol agent in reducing T-2 toxin production.[Citation36] Even though the yeast has been successfully used in a number of malting plants across Europe, its efficiency remains variable. Many research works have also shown the capacity of plant phenolic compounds to inhibit fungal growth and to reduce the production of various Fusarium mycotoxins, such as deoxynivalenol,[Citation37–39] T2 and HT2 toxins,[Citation40,Citation41] and enniatins.[Citation11] Another increasingly studied and adapted way for the bio-preservation of foodstuffs against pathogenic fungi is the use of lactic acid bacteria (LAB) that produce antifungal metabolites and have a Generally Regarded as Safe (GRAS) status, thus representing a biotechnological advantage for the cereal industry. Some of the most frequently researched LAB strains for this purpose belong to the Lactobacillus genus.[Citation42–46] To date, several studies have demonstrated the capacity of LAB and other bacteria to efficiently inhibit fungal growth and the associated production of many Fusarium mycotoxins including deoxynivalenol, fumonisins, zearalenone, T2 and HT2 toxins.[Citation47–51] However, to the best of our knowledge, no study has investigated the effect of barley-associated bacteria on the growth of ENNs-producing Fusarium species and on their ENNs production.
Among the barley-associated bacterial species, the most common and abundant species during growth belong to the Erwinia genus, which is also one of the most important microbial genera found in germinated barley (malt).[Citation52] Erwinia bacteria are primarily rod-shaped and gram-negative. An example of the bacterial species belonging to this genus is the novel Erwinia gerundensis, a motile, non-spore forming, and facultatively anaerobic bacterium originally isolated from pear tree leaves in Spain, during a study aimed at finding microbial strains against pome fruit diseases.[Citation53] Although the aforementioned bacterial strain was not reported as a potential biocontrol agent in the original study, it appears to have recently been the subject of a patent filed in South Korea, entitled “Erwinia gerundensis KUDC9201 strain having antifungal activity against pathogens and uses thereof”.[Citation54] The invention consists of using E. gerundensis as an environmentally friendly biological control agent against the soft rot of tobacco leaves and bacterial disease of pepper. Given this data and the fact that a strain of Erwinia gerundensis has recently been isolated from barley kernels in our laboratory, it was deemed important to look into its antifungal properties so as to investigate whether it could be considered as a potential biocontrol agent against barley pathogens and their mycotoxins.
Our study was therefore aimed at investigating the effect of the barley-associated E. gerundensis strain on the growth and ENNs production of Fusarium tricinctum, one of the major ENNs-producing Fusarium species.[Citation21] The originality of this approach lies in the use of an environmental bacterial strain that is naturally present in the microbiota of barley kernels originating from the Eastern region of France.
Experimental
Chemicals and reagents
The growth media and solvents used in this study were of analytical grade and were purchased from Sigma Aldrich (France). For LC-MS/MS analysis, solvents and standards were purchased from Carlo Erba (Val-de-Reuil, France).
Strains and culture conditions
F. tricinctum strains, growth media and culture conditions
Eight F. tricinctum strains (F. tricinctum 2319, F. tricinctum 2399, F. tricinctum 2447, F. tricinctum 2499, F. tricinctum 2500, F. tricinctum 2502, F. tricinctum 2503, and F. tricinctum 2504) were used in this study. In addition, a preliminary experiment was also carried out using three other fungal strains: F. avenaceum 2379, F. graminearum 2380, and F. langsethiae 2359. These strains were previously isolated and identified from contaminated barley kernels harvested over four years (2017 to 2020) from various regions in France, following the French Ministry of Agriculture and Food Official Method.[Citation55] The strains were identified and collected by the French Institute of Beverages, Brewing and Malting (IFBM), who provided them for this study.
Stock cultures of the fungal strains were maintained at 5 °C in mineral oil. When fresh cultures were required, the stock cultures were transferred to Petri dishes containing PDA medium (39 g/L) and incubated at 22 °C for 7 days. Cultures were then used for radial growth inhibition assays or for co-culture assays in liquid media.
Bacterial strain and culture conditions
The bacterial strain Erwinia gerundensis 6E4 used in this study was isolated from contaminated barley kernels harvested in the eastern region of France in 2018 and identified using a 16 S rRNA gene sequencing method; and was provided by the Technological Mixed Unit (UMT) OPTIMALT (Nancy, France). It was initially grown in Yeast Malt liquid medium (YM: 10 g/L glucose; 3 g/L malt extract; 5 g/L peptone; 3 g/L yeast extract) at 30 °C for 72 h. Then, the liquid culture was centrifuged (3000 x g for 5 min) and the pellet was recovered in fresh YM liquid medium with 10% glycerol and stored at −80 °C as a stock culture until required for further culture experiments.
Radial growth inhibition of the fungal strains
Four model fungal strains (F. avenaceum 2379, F. graminearum 2380, F. langsethiae 2359, and F. tricinctum 2399) were subjected to radial growth inhibition experiments on 90 mm diameter Petri dishes, following a modified form of the method proposed by Petatán-Sagahón et al.[Citation56] These are the major fungal strains found in barley and produce all together each type of mycotoxin: T2 and HT2 toxins, deoxynivalenol, enniatins, etc. A 0.5 cm2 fungal plug (from the fungi previously grown on a PDA plate) was cut and inoculated onto the center of a plate containing YM Agar medium, in such a way that the fungal mycelium was directly in contact with the solid medium; then, 80 µL of E. gerundensis 6E4 suspension (with a biomass concentration of 109 CFU/mL) was inoculated at four different places (4 × 20 µL) around the fungal mycelium, at an equidistance of 2.5 cm from the fungus. A fungal control was prepared by cutting and placing another 0.5 cm2 fungal plug of each fungal strain onto a plate containing the same medium, without the bacterial strain around it. The plates were incubated at 28 °C for five days. The percentage of inhibition of fungal growth (PIFG) was determined as: PIFG (%) = [(D1-D2)/D1]*100, where D1 represents the average diameter covered by the fungus on the control plates and D2 the average diameter covered by the fungus in the co-cultures. Experiments were conducted in duplicate.
Toxigenic potential of the Fusarium strains
The toxigenic potential of the eight F. tricinctum strains was evaluated in YM broth. Fungal plugs of each of the F. tricinctum strains were transferred to 250 mL Erlenmeyer flasks containing 100 mL of YM broth. The flasks were incubated at 28 °C in a rotary shaker operating at 210 rpm, for two different incubation times: 72 h and 168 h. Samples taken at the end of the culture time were filtered (pore size of 0.2 μm) and stored at −25 °C until required for enniatin analysis by the LC-MS/MS method.
Enniatin analysis by the LC-MS/MS method
The enniatins produced (during the cultures) throughout the study were determined by the method of liquid chromatography-mass spectrometry (LC-MS/MS), using a Famos-Switchos-Ultimate capillary LC system coupled to a triple-quadrupole mass spectrometer (ThermoFisher Scientific, USA). The samples were filtered using a 0.2 µm syringe filter before LC-MS/MS analysis. The quantification of A, A1, B and B1 enniatins was performed using a Gemini C18 column (150 mm x 4.6 mm, 3 µm) (Phenomenex, France). The chromatographic mobile phases consisted of Milli-Q water and methanol at a flow rate of 0.3 mL/min. The injection volume was 10 µL and the limit of detection of the method was 10 µg/kg.
Co-culture of the Fusarium strains and Erwinia gerundensis 6E4 in a liquid medium
Before the co-culture of the Fusarium strains and the bacterial strain, a microbial suspension of each microorganism was prepared.
Bacterial suspension: 1 mL of the bacterial stock culture, previously stored at −80 °C, was thawed and inoculated into a 15 mL Falcon tube containing 10 mL of YM liquid medium (21 g/L). After 24 h of culture at 28 °C, the entire volume was transferred to a 250 mL Erlenmeyer flask containing 100 mL of YM liquid medium and the flask was incubated at 28 °C in a shaking incubator (210 rpm) for another 24 h. Finally, the bacterial biomass concentration was determined using the plate count technique and the suspension was ultimately used for co-culture assays in the liquid medium.
Fungal suspension: Fusarium spore suspensions were prepared in Spezieller Nährstoffärmer Agar medium (SNA: 1 g/L K2HPO4, 1 g/L KNO3, 0.5 g/L MgSO4.7H2O, 0.5 g/L KCl, 0.2 g/L glucose, 0.2 g/L saccharose, 20 g/L agar). First, at least 3 plugs of each Fusarium strain from a seven-day-old solid (PDA) pre-culture were inoculated onto Petri dishes (diameter 90 mm) containing SNA medium in such a way that the mycelium side was in direct contact with the solid medium and incubated at 22 °C for 7-10 days, in a climatic chamber with alternating light and dark. At the end of the incubation time, 3 mL of sterile water was added to each Petri dish to collect the fungal spores. Finally, the spores were counted on a Malassez cell counting chamber and ultimately used for co-culture assays in the liquid medium or conserved at 5 °C.
Co-culture in YM liquid medium: The fungal and bacterial strains were co-cultured in 250 mL Erlenmeyer flasks containing 100 mL of YM liquid medium. The bacterial strain was inoculated at 0.5% (0.5 mL in 100 mL YM), corresponding to a concentration of 3.5 × 107 CFU/mL. Then, each Fusarium strain was inoculated at a final concentration of 3 × 104 spores/mL (3 × 106 spores inoculated in 100 mL YM) in their corresponding flasks. For the control conditions, each microorganism was inoculated alone (separately) at the same concentrations. The cultures were then incubated at 28 °C in a rotary shaker operating at 210 rpm for 72 h. At the end, samples were taken and filtered using a syringe filter (pore size 0.2 µm) and stored in chromatography vials at −25 °C until required for enniatin analysis. For the kinetic study, additional sampling times (0 h, 24 h, 48 h, and 72 h) were included for the bacterial strain and the model fungal strain F. tricinctum 2399 and bacterial biomass, fungal biomass, and enniatin concentration were evaluated for each of the additional sampling times. Co-culture experiments were conducted in duplicate for the kinetic study with the model strain F. tricinctum 2399.
E. gerundensis and F. tricinctum 2399 biomass and metabolite evaluation
For kinetic purposes, samples were taken every 24 h for 3 days and the bacterial and fungal biomass concentrations were determined for each of the additional sampling times in the section above. The microbial biomass was determined by the plate count method in YM agar medium at 28 °C for 72 h for the bacterial strain and in Oxytetracycline-Glucose-Yeast Extract Agar (OGYE Agar) for 5 days for the fungus. Enniatin concentration was also determined by the LC-MS/MS method described above.
Presentation of results
Enniatin analysis results are presented as the mean ± percentage of uncertainty (15%) of the LC-MS/MS method. The other results are presented as the mean ± standard deviation (SD).
Results and discussion
Effect of Erwinia gerundensis 6E4 on the radial growth of Fusarium strains
E. gerundensis 6E4 was simultaneously co-cultured on solid medium at 28 °C for five days with four different fungal strains (F. avenaceum 2379, F. graminearum 2380, F. langsethiae 2359, and F. tricinctum 2399), considered as reference fungal species by the French Institute of Beverages, Brewing and Malting (IFBM), as they are among the main pathogenic fungi found on barley. The results show that the fungal growth was clearly affected by the presence of Erwinia gerundensis 6E4. The bacterial strain reduced the radial growth of all four reference fungal strains by at least 50% on the solid medium. The most affected fungi were F. langsethiae 2359 (∼100% inhibition), followed by F. tricinctum 2399 (∼ 84% inhibition), and F. graminearum 2380 (∼ 77% inhibition). F. avenaceum 2379 was the least affected (∼57% inhibition) ().
Figure 2. Visual effect of E. gerundensis 6E4 on the radial growth of Fusarium strains on solid medium.
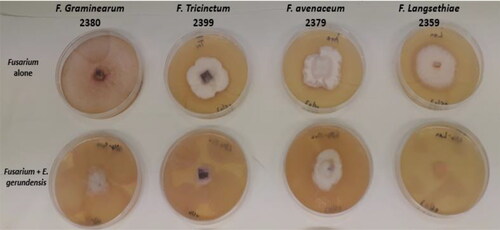
These results imply that E. gerundensis 6E4 has a significant fungistatic activity against a variety of Fusarium species and could potentially be used as a biological agent to reduce the fungal load in barley-derived products. Each of these fungal species produces a variety of mycotoxins (e.g., deoxynivalenol, nivalenol, enniatins, T2 & HT2 toxins), but as our study was focused on enniatins, the subsequent experiments were carried out on the enniatin-producing species Fusarium tricinctum. The focus on enniatins, and enniatin producers comes from the emerging nature of these toxins in the barley-malt-beer chain with associated health and technological concerns. Thus, a total of eight F. tricinctum strains were tested throughout the remaining part of the study, with F. tricinctum 2399 being considered as the reference fungal strain when necessary.
Toxigenic potential of F. tricinctum strains in liquid medium
The goal of this part of the study was to check the ability of the eight F. tricinctum strains to produce enniatins in liquid medium, prior to their co-culture with the bacterial strain. For this purpose, fungal plugs of each of the eight isolates were inoculated into YM liquid medium for two different incubation times (72 h and 168 h) at 28 °C, 210 rpm. For each incubation time, enniatin concentrations were analyzed by LC-MS/MS method.
The results show that all 8 isolates of F. tricinctum produce enniatins (sum of enniatins A, A1, B and B1) in high concentrations at both incubation times. In 72 h, the average total enniatin concentration produced by the fungal strains was 30753 μg/kg (±4613), with concentrations ranging from 1931 μg/kg (±290) for F. tricinctum 2447 to 51540 μg/kg (±7731) for F. tricinctum 2499. As for the 168 h incubation, the average total enniatin concentration detected was 50% lower than that detected at 72 h, with most of the isolates yielding lower enniatin concentrations ().
The range of total enniatin produced by these fungi (approximately 2000 µg/kg to 50000 µg/kg) is comparable to data observed in other studies where total enniatin produced by F. tricinctum strains were reported to vary from 3000 µg/kg to 1365 mg/L.[Citation26,Citation27,Citation57–59] The differences in total enniatin biosynthesis among the eight F. tricinctum strains might be due to the enniatin synthetase gene (Esyn1) divergence, as suggested in a previous study.[Citation59] In addition, the decrease in total enniatin concentration observed at 168 h could be explained by the fact that after 72 h of incubation, part of the enniatins released in the medium might have been used by the Fusarium strains as a carbon source for growth. It is known that some fungal species are able to use their mycotoxins as a carbon source during the last phase of growth.[Citation27] Therefore, the incubation time was limited to 72 h for the following experiments.
Effect of co-culture between Erwinia gerundensis 6E4 and F. tricinctum strains on enniatin production
Prior to the control culture and co-culture experiments, bacterial and fungal suspensions were prepared, and their respective microbial biomass was evaluated. The bacterial biomass obtained was 3.6 × 109 CFU/mL in 24 h of culture and the fungal biomass concentrations ranged from 6.7 × 105 spores/mL for F. tricinctum 2399 to 8 × 106 spores/mL for F. tricinctum 2447.
The antifungal effect of Erwinia gerundensis 6E4 on the 8 fungal strains was investigated in liquid medium through co-culture experiments that consisted of simultaneously inoculating E. gerundensis 6E4 and the F. tricinctum strains into YM liquid medium at 28 °C and 210 rpm for 72 h. The bacterial inoculum used at the beginning of the co-cultures was 1.8 × 107 CFU/mL. The fungal inoculum used at the beginning of the co-cultures was adjusted to 106 spores in 100 mL of YM liquid medium, corresponding to 104 spores/mL. Control cultures were also carried out by inoculating each of the fungal strains and the bacterial strain alone into YM liquid medium. At the end of the incubation time (72 h), enniatin concentration was analyzed in the fungal control cultures and in the co-cultures.
During the experiments, it was observed that the fungus F. tricinctum has the particularity of producing specific secondary metabolites that change the color of the culture medium from yellowish orange to red. , panel A shows that in the control cultures, the color of the culture medium changed to red, indicating that the fungal strains were indeed in the secondary metabolism phase. The change in color could be due to a significant production of aurofusarin, a red pigment naturally produced as a secondary metabolite by a number of Fusarium species including Fusarium tricinctum.[Citation60] In contrast, there was no color change in the co-cultures (, panel B), suggesting that the bacterial strain may have visibly impacted the secondary metabolism of the fungal strains and possibly their enniatin production.
Figure 4. Visual impact of Erwinia gerundensis 6E4 on F. tricinctum strains after a 72 h co-culture. From left to right: example of strains F. tricinctum 2319, F. tricinctum 2399 and F. tricinctum 2504 in A) Control culture and in B) Co-culture with Erwinia gerundensis 6E4.
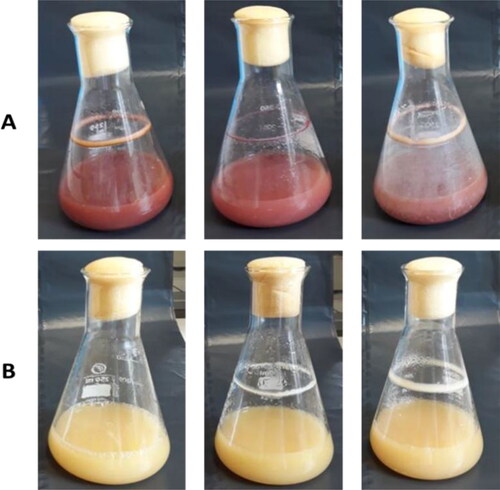
Enniatin analysis by LC-MS/MS method confirmed the toxigenic potential of all eight F. tricinctum strains in the experimental conditions. Among the four types of enniatins (ENN A, ENN A1, ENN B and ENN B1) produced by F. tricinctum strains in control cultures, enniatin B was produced in the highest concentration for most of the strains ().
Table 1. Concentration of enniatins A, A1, B and B1 produced by F. tricinctum strains in liquid medium: control cultures.
Total enniatin concentrations (sum of ENN A, A1, B and B1) detected in control cultures (F. tricinctum alone) ranged from 197 µg/kg (±30) to 31224 µg/kg (±4684), with the strain F. tricinctum 2502 being the highest enniatin producer. In co-cultures (F. tricinctum + E. gerundensis 6E4), the concentration of total enniatin produced by each of the eight fungal strains decreased radically by 74-100% as compared to those in control cultures ().
Figure 5. Total enniatin (ENN) concentration in control cultures and in co-cultures. ND: not detectable.
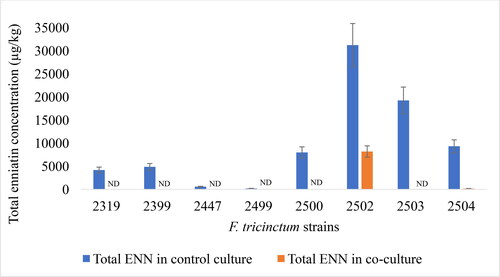
These results show that the presence of the bacterial strain Erwinia gerundensis 6E4 leads to a strong reduction in the concentration of enniatin produced by 8 different Fusarium tricinctum strains, thus suggesting that E. gerundensis 6E4 might be a strong inhibitor of enniatin production. They also confirm that the absence of a color change in the co-cultures () could indeed be related to a reduction in secondary metabolite production by the fungi. Hence, this phenomenon could be considered as a visual indicator of the impact of the bacterial strain Erwinia gerundensis on Fusarium tricinctum strains during laboratory experiments involving the simultaneous co-culture of these two microorganisms in liquid medium.
The reduction of enniatin might have occurred through a number of mechanisms. Firstly, E. gerundensis 6E4 might have the capacity to degrade enniatin, as in the case of several strains of Lactobacillus,[Citation61] Bacillus subtilis,[Citation62] and Bacillus licheniformis.[Citation59] Secondly, the bacterial strain could have prevented the Fusarium strains from producing enniatin by altering or inhibiting their metabolic pathways. Finally, the bacterial strain could have caused a reduction of enniatin by simply reducing the fungal growth. To investigate whether the reduction of enniatin production was related to the reduction of fungal growth, further experiments were carried out to assess the evolution of bacterial and fungal growth and enniatin production.
Evolution of bacterial growth, fungal growth and enniatin production during co-culture between F. tricinctum 2399 and Erwinia gerundensis 6E4
To better understand the impact of Erwinia gerundensis 6E4 on enniatin production by Fusarium tricinctum during co-culture, the microbial growth of the two types of microorganism as well as enniatin production were monitored for 72 h. For this purpose, only the reference fungal strain F. tricinctum 2399 was tested during a co-culture with the bacterial strain. Bacterial and fungal control cultures were also carried out and samples were taken and analyzed at four different incubation times: 0 h, 24 h, 48 h, and 72 h. The results are reported in .
Table 2. Growth characteristics of E. gerundensis 6E4 and F. tricinctum 2399 and enniatin production in the 72 h control culture and co-culture.
In the control cultures, the microbial biomass increased throughout the incubation, from 1.8 × 107 CFU/mL to a maximum of 1.3 × 1010 CFU/mL for the bacterial strain, and from 1.0 × 104 CFU/mL to 3.1 × 107 CFU/mL for the fungal strain in 72 h. The bacterial biomass determined in the 72 h co-culture was 1.5 × 1010 CFU/mL, quite similar to that obtained in the bacterial control culture. On the other hand, fungal growth experienced a slower start at the beginning of the co-culture, with a lag phase of about 48 h, before increasing to a maximum of 9.7 × 106 CFU/mL at 72 h. As per the growth parameters, the average growth rate (µav) of the bacterial strain was similar under the two conditions: 0.093 h−1 in the control culture and 0.096 h−1 in the co-culture, with a generation time (G) of about 7 h in both cases. Therefore, the co-culture did not affect the bacterial growth. As far as the fungal strain is concerned, its average growth rate (µav) in co-culture (0.085 h−1) was lower than that in control culture (0.115 h−1), with the generation time (G) being logically longer in co-culture (8 h) as compared to that in control culture (6 h), suggesting that the co-culture had an impact on the fungal growth. More precisely, the fungal biomass was reduced by 69% within 72 h of co-culture, as compared to that in the fungal control culture. Thus, the presence of the bacterial strain E. gerundensis 6E4 led to a significant reduction in the growth of the reference fungal strain F. tricinctum 2399. As for enniatin analysis, results reported in show that in the fungal control culture, the total enniatin produced by the fungus was 1355 µg/kg (±203) over the 72 h incubation, while in contrast, no enniatin was detected throughout the co-culture, meaning that the percentage of enniatin reduction was 100%.
Taken together, the data indicates that the bacterial strain E. gerundensis 6E4 was not only efficient in reducing fungal development in solid and liquid media but also significantly reduced the production of ENNs in the liquid medium. This could be of interest for fungal contamination management strategies, especially in the Barley-Malt-Beer industry, where fungal and mycotoxin contaminations can lead to disastrous economic, health, and technological consequences. The malting process (transformation of barley into malt) provides favorable conditions (temperature of 18-22 °C and high humidity) for the development of Fusarium species and their mycotoxin production.[Citation63,Citation64] Also, the other microorganisms that are naturally present on barley kernels, such as E. gerundensis 6E4, can grow well under these conditions, as most of them are mesophilic. Therefore, it could be interesting to develop a process where Erwinia gerundensis 6E4 can be used as a biocontrol agent during the malting process in order to reduce the consequences of fungal contamination in the barley-beer chain. The mycoparasitic fungus Pythium oligandrum has recently been shown to exhibit antifungal properties against Fusarium culmorum (contamination reduced by 20%) and its mycotoxin production (mycotoxin content reduced by 17-21%) during the steeping step of wheat malting.[Citation65] Erwinia gerundensis 6E4 could also be inoculated during the steeping step (hydration and oxygenation of barley kernels at 18-22 °C) of the malting process, where it would compete with pathogenic fungal species naturally present on the barley kernels, and it could possibly reduce their proliferation and their mycotoxin production. The biocontrol agent could consequently be used in a competitive solid-state fermentation process, and then the bacterial strain could be completely eliminated by the thermal treatments (up to 110 °C for a few hours) applied during the kilning step of the malting process, as it does not produce spores. However, before any technological use, an important factor that needs to be assessed is the inoculation rate of the bacterial strain. In this study, Erwinia gerundensis 6E4 proved to be highly efficient with an initial inoculation rate of 1.8 × 107 CFU/mL at the beginning of the co-cultures with F. tricinctum strains. Thus, for economic and technological reasons, it is important to determine the optimal bacterial inoculum rate, hence the minimum inhibitory concentration that can be used to maintain a high efficiency. In addition, it would be interesting to evaluate the antifungal property of Erwinia gerundensis 6E4 on several other fungal species and in mixed fungal cultures in order to assess its efficiency toward a variety of mycotoxins, thus broadening its application possibilities.
It was shown that E. gerundensis 6E4 is highly efficient in reducing fungal growth and enniatin production, however, the mechanisms underlying its antifungal activity were not assessed in our current study. Therefore, it would be interesting to look into how E. gerundensis 6E4 expresses its antagonistic properties against Fusarium tricinctum strains. One way to do this, would be to investigate the effect of cell-free culture supernatants derived from the broth culture of E. gerundensis 6E4 on the growth and metabolism of F. tricinctum strains in order to shed light on the bacterial metabolites that may be involved in the inhibition of the growth of F. tricinctum and the reduction of its enniatin production. In the literature, it was recently reported that a strain of E. gerundensis isolated from almond inner leaf tissues exhibits the ability of producing enterobactin, gluconic acid, and polyamines, which play important roles in living organisms.[Citation66] For example, enterobactin is one of the most important siderophores generally produced by gram-negative bacteria.[Citation67] Siderophores produced by Pseudomonas aeruginosa have been reported to have antagonistic activity against phytopathogenic fungi such as Fusarium oxysporum, Fusarium udum, and Aspergillus niger.[Citation68] Polyamines are organic compounds that are essential for cell growth and development;[Citation69] they are also involved in defense responses against plant pathogens.[Citation70,Citation71] Gluconic acid has been reported to be an important antifungal agent in the control of take-all disease in cereal grains.[Citation72] These studies suggest that enterobactin, gluconic acid, and polyamines might be among the metabolites responsible for the antifungal activity of E. gerundensis 6E4 that was evidenced in our study.
Conclusion
This study investigated the antifungal activity of a barley-associated bacterial strain against Fusarium strains. The results are extremely important as they highlight for the first time the high efficiency of a bacterial strain belonging to the Erwinia genus to reduce the proliferation of the pathogenic fungus Fusarium tricinctum and its enniatin production. Based on these results, the study supports the use of Erwinia gerundensis as a biocontrol agent in strategies aimed at reducing the presence of pathogenic fungi and enniatins in cereal-based products, or as a food and feed supplement for the bio-detoxification of enniatins. However, more research efforts are required to fully understand the mechanisms underlying the effect of the bacterium on fungal growth and enniatin biosynthesis.
Acknowledgments
The authors would like to thank Léa-Jehanne Robert for her valuable technical support.
Disclosure statement
No potential conflict of interest was reported by the authors.
Additional information
Funding
Literature cited
- Laitila, A.; Kotaviita, E.; Peltola, P.; Home, S.; Wilhelmson, A. Indigenous Microbial Community of Barley Greatly Influences Grain Germination and Malt Quality. J. Inst. Brew. 2007, 113, 9–20. DOI: 10.1002/j.2050-0416.2007.tb00250.x.
- Laitila, A.; Manninen, J.; Priha, O.; Smart, K.; Tsitko, I.; James, S. Characterisation of Barley-Associated Bacteria and Their Impact on Wort Separation Performance. J. Inst. Brew. 2018, 124, 314–324. DOI: 10.1002/jib.509.
- Bianco, A.; Fancello, F.; Balmas, V.; Zara, G.; Dettori, M.; Budroni, M. The Microbiome of Sardinian Barley and Malt. J. Inst. Brew. 2018, 124, 344–351. DOI: 10.1002/jib.522.
- Pitt, J. I.; Hocking, A. D. The Ecology of Fungal Food Spoilage. In Fungi and Food Spoilage; Pitt, J. I., Hocking, A. D., Eds.; Springer US: Boston, MA, 2009; pp 3–9.
- Mamur, S.; Yuzbasioglu, D.; Yılmaz, S.; Erikel, E.; Unal, F. Assessment of Cytotoxic and Genotoxic Effects of Enniatin-A in Vitro. Food Addit Contam Part A Chem Anal Control Expo Risk Assess 2018, 35, 1633–1644. DOI: 10.1080/19440049.2018.1486513.
- Agriopoulou, S.; Stamatelopoulou, E.; Varzakas, T. Advances in Occurrence, Importance, and Mycotoxin Control Strategies: Prevention and Detoxification in Foods. Foods Basel Switz 2020, 9, 137. DOI: 10.3390/foods9020137.
- Yang, J.; Li, J.; Jiang, Y.; Duan, X.; Qu, H.; Yang, B.; Chen, F.; Sivakumar, D. Natural Occurrence, Analysis, and Prevention of Mycotoxins in Fruits and Their Processed Products. Crit. Rev. Food Sci. Nutr. 2014, 54, 64–83. DOI: 10.1080/10408398.2011.569860.
- Pitt, J. I.; Miller, J. D. A Concise History of Mycotoxin Research. J. Agric. Food Chem. 2017, 65, 7021–7033. DOI: 10.1021/acs.jafc.6b04494.
- Ben Taheur, F.; Kouidhi, B.; Al Qurashi, Y. M. A.; Ben Salah-Abbès, J.; Chaieb, K. Review: Biotechnology of Mycotoxins Detoxification Using Microorganisms and Enzymes. Toxicon 2019, 160, 12–22. DOI: 10.1016/j.toxicon.2019.02.001.
- Torres, A. m.; Palacios, S. a.; Yerkovich, N.; Palazzini, J. m.; Battilani, P.; Leslie, J. f.; Logrieco, A. f.; Chulze, S. n. Fusarium Head Blight and Mycotoxins in Wheat: Prevention and Control Strategies across the Food Chain. World Mycotoxin J. 2019, 12, 333–355. DOI: 10.3920/WMJ2019.2438.
- Gautier, C.; Pinson-Gadais, L.; Verdal-Bonnin, M.-N.; Ducos, C.; Tremblay, J.; Chéreau, S.; Atanasova, V.; Richard-Forget, F. Investigating the Efficiency of Hydroxycinnamic Acids to Inhibit the Production of Enniatins by Fusarium avenaceum and Modulate the Expression of Enniatins Biosynthetic Genes. Toxins 2020, 12, 735. DOI: 10.3390/toxins12120735.
- Alassane-Kpembi, I.; Schatzmayr, G.; Taranu, I.; Marin, D.; Puel, O.; Oswald, I. P. Mycotoxins Co-Contamination: Methodological Aspects and Biological Relevance of Combined Toxicity Studies. Crit. Rev. Food Sci. Nutr. 2017, 57, 3489–3507. DOI: 10.1080/10408398.2016.1140632.
- Winter, G.; Pereg, L. A Review on the Relation between Soil and Mycotoxins: Effect of Aflatoxin on Field, Food and Finance. Eur. J. Soil Sci. 2019, 70, 882–897. DOI: 10.1111/ejss.12813.
- van Egmond, H. P.; Schothorst, R. C.; Jonker, M. A. Regulations Relating to Mycotoxins in food: Perspectives in a global and European context. Anal. Bioanal. Chem. 2007, 389, 147–157. DOI: 10.1007/s00216-007-1317-9.
- European Commission. Setting Maximum Levels for Certain Contaminants in Foodstuffs; 2006; Vol. Official Journal of the European Union, L364, pp 5−24.
- Vaclavikova, M.; Malachova, A.; Veprikova, Z.; Dzuman, Z.; Zachariasova, M.; Hajslova, J. ‘Emerging’ Mycotoxins in Cereals Processing Chains: Changes of Enniatins during Beer and Bread Making. Food Chem. 2013, 136, 750–757. DOI: 10.1016/j.foodchem.2012.08.031.
- Fraeyman, S.; Croubels, S.; Devreese, M.; Antonissen, G. Emerging Fusarium and Alternaria Mycotoxins: Occurrence, Toxicity and Toxicokinetics. Toxins 2017, 9, 228. DOI: 10.3390/toxins9070228.
- Orlando, B.; Grignon, G.; Vitry, C.; Kashefifard, K.; Valade, R. Fusarium Species and Enniatin Mycotoxins in Wheat, Durum Wheat, Triticale and Barley Harvested in France. Mycotoxin Res. 2019, 35, 369–380. DOI: 10.1007/s12550-019-00363-x.
- Jestoi, M. Emerging Fusarium-Mycotoxins Fusaproliferin, Beauvericin, Enniatins, and Moniliformin: A Review. Crit. Rev. Food Sci. Nutr. 2008, 48, 21–49. DOI: 10.1080/10408390601062021.
- Lee, H. J.; Ryu, D. Worldwide Occurrence of Mycotoxins in Cereals and Cereal-Derived Food Products: Public Health Perspectives of Their Co-Occurrence. J. Agric. Food Chem. 2017, 65, 7034–7051. DOI: 10.1021/acs.jafc.6b04847.
- Gautier, C.; Pinson-Gadais, L.; Richard-Forget, F. Fusarium Mycotoxins Enniatins: An Updated Review of Their Occurrence, the Producing Fusarium Species, and the Abiotic Determinants of Their Accumulation in Crop Harvests. J. Agric. Food Chem. 2020, 68, 4788–4798. DOI: 10.1021/acs.jafc.0c00411.
- Jestoi, M.; Rokka, M.; Järvenpää, E.; Peltonen, K. Determination of Fusarium Mycotoxins Beauvericin and Enniatins (A, A1, B, B1) in Eggs of Laying Hens Using Liquid Chromatography–Tandem Mass Spectrometry (LC–MS/MS). Food Chem. 2009, 115, 1120–1127. DOI: 10.1016/j.foodchem.2008.12.105.
- Zocher, R.; Keller, U.; Kleinkauf, H. Enniatin Synthetase, a Novel Type of Multifunctional Enzyme Catalyzing Depsipeptide Synthesis in Fusarium oxysporum. Biochemistry 1982, 21, 43–48. DOI: 10.1021/bi00530a008.
- Haese, A.; Schubert, M.; Herrmann, M.; Zocher, R. Molecular Characterization of the Enniatin Synthetase Gene Encoding a Multifunctional Enzyme Catalysing N-Methyldepsipeptide Formation in Fusarium scirpi. Mol. Microbiol. 1993, 7, 905–914. DOI: 10.1111/j.1365-2958.1993.tb01181.x.
- Fanelli, F.; Ferracane, R.; Ritieni, A.; Logrieco, A. F.; Mulè, G. Transcriptional Regulation of Enniatins Production by Fusarium avenaceum. J. Appl. Microbiol. 2014, 116, 390–399. DOI: 10.1111/jam.12371.
- Wätjen, W.; Debbab, A.; Hohlfeld, A.; Chovolou, Y.; Kampkötter, A.; Edrada, R. A.; Ebel, R.; Hakiki, A.; Mosaddak, M.; Totzke, F.; et al. Enniatins A1, B and B1 from an Endophytic Strain of Fusarium tricinctum Induce Apoptotic Cell Death in H4IIE Hepatoma Cells Accompanied by Inhibition of ERK Phosphorylation. Mol. Nutr. Food Res. 2009, 53, 431–440. DOI: 10.1002/mnfr.200700428.
- Meca, G.; Mañes, J.; Font, G.; Ruiz, M. J. Study of the Potential Toxicity of Enniatins A, A(1), B, B(1) by Evaluation of Duodenal and Colonic Bioavailability Applying an in Vitro Method by Caco-2 Cells. Toxicon 2012, 59, 1–11. DOI: 10.1016/j.toxicon.2011.10.004.
- Rodríguez-Carrasco, Y.; Heilos, D.; Richter, L.; Süssmuth, R. D.; Heffeter, P.; Sulyok, M.; Kenner, L.; Berger, W.; Dornetshuber-Fleiss, R. Dornetshuber-Fleiss, R. Mouse Tissue Distribution and Persistence of the Food-Born Fusariotoxins Enniatin B and Beauvericin. Toxicol. Lett. 2016, 247, 35–44. DOI: 10.1016/j.toxlet.2016.02.008.
- Prosperini, A.; Berrada, H.; Ruiz, M. J.; Caloni, F.; Coccini, T.; Spicer, L. J.; Perego, M. C.; Lafranconi, A. A Review of the Mycotoxin Enniatin B. Front. Public Health. 2017, 5, 304. DOI: 10.3389/fpubh.2017.00304.
- Maranghi, F.; Tassinari, R.; Narciso, L.; Tait, S.; Rocca, C. L.; Felice, G. D.; Butteroni, C.; Corinti, S.; Barletta, B.; Cordelli, E. In Vivo Toxicity and Genotoxicity of Beauvericin and Enniatins. Combined Approach to Study in Vivo Toxicity and Genotoxicity of Mycotoxins Beauvericin (BEA) and Enniatin B (ENNB). EFSA Support. Publ. 2018, 15, 1406E. DOI: 10.2903/sp.efsa.2018.EN-1406.
- Altomare, C.; Logrieco, A. F.; Gallo, A. Mycotoxins and Mycotoxigenic Fungi: Risk and Management. A Challenge for Future Global Food Safety and Security. In Encyclopedia of Mycology; Zaragoza, Ó., Casadevall, A., Eds.; Elsevier: Oxford, 2021; pp 64–93.
- Mielniczuk, E.; Skwaryło-Bednarz, B. Fusarium Head Blight, Mycotoxins and Strategies for Their Reduction. Agronomy 2020, 10, 509. DOI: 10.3390/agronomy10040509.
- Price, C. L.; Parker, J. E.; Warrilow, A. G. S.; Kelly, D. E.; Kelly, S. L. Azole Fungicides - Understanding Resistance Mechanisms in Agricultural Fungal Pathogens. Pest Manage. Sci. 2015, 71, 1054–1058. DOI: 10.1002/ps.4029.
- Reis, J. A.; Paula, A. T.; Casarotti, S.; Penna, A. Lactic Acid Bacteria Antimicrobial Compounds: Characteristics and Applications. Food Eng. Rev. 2012, 4, 124–140. DOI: 10.1007/s12393-012-9051-2.
- Dieuleveux, V.; Van Der Pyl, D.; Chataud, J.; Gueguen, M. Purification and Characterization of anti-Listeria Compounds Produced by Geotrichum candidum. Appl. Environ. Microbiol. 1998, 64, 800–803.
- Kawtharani, H.; Snini, S. P.; Heang, S.; Bouajila, J.; Taillandier, P.; Mathieu, F.; Beaufort, S. Phenyllactic Acid Produced by Geotrichum candidum Reduces Fusarium sporotrichioides and F. langsethiae Growth and T-2 Toxin Concentration. Toxins 2020, 12, 209. DOI: 10.3390/toxins12040209.
- Boutigny, A.-L.; Barreau, C.; Atanasova-Penichon, V.; Verdal-Bonnin, M.-N.; Pinson-Gadais, L.; Richard-Forget, F. Ferulic Acid, an Efficient Inhibitor of Type B Trichothecene Biosynthesis and Tri Gene Expression in Fusarium Liquid Cultures. Mycol. Res. 2009, 113, 746–753. DOI: 10.1016/j.mycres.2009.02.010.
- Gauthier, L.; Bonnin-Verdal, M.-N.; Marchegay, G.; Pinson-Gadais, L.; Ducos, C.; Richard-Forget, F.; Atanasova-Penichon, V. Atanasova-Penichon, V. Fungal Biotransformation of Chlorogenic and Caffeic Acids by Fusarium graminearum: New Insights in the Contribution of Phenolic Acids to Resistance to Deoxynivalenol Accumulation in Cereals. Int. J. Food Microbiol. 2016, 221, 61–68. DOI: 10.1016/j.ijfoodmicro.2016.01.005.
- Kulik, T.; Stuper-Szablewska, K.; Bilska, K.; Buśko, M.; Ostrowska-Kołodziejczak, A.; Załuski, D.; Perkowski, J. Sinapic Acid Affects Phenolic and Trichothecene Profiles of F. Culmorum and F. graminearum Sensu Stricto. Toxins 2017, 9, 264. DOI: 10.3390/toxins9090264.
- Ferruz, E.; Atanasova-Pénichon, V.; Bonnin-Verdal, M.-N.; Marchegay, G.; Pinson-Gadais, L.; Ducos, C.; Lorán, S.; Ariño, A.; Barreau, C.; Richard-Forget, F. Effects of Phenolic Acids on the Growth and Production of T-2 and HT-2 Toxins by Fusarium langsethiae and F. sporotrichioides. Molecules 2016, 21, 449. DOI: 10.3390/molecules21040449.
- Schöneberg, T.; Kibler, K.; Sulyok, M.; Musa, T.; Bucheli, T. D.; Mascher, F.; Bertossa, M.; Voegele, R. T.; Vogelgsang, S. Can Plant Phenolic Compounds Reduce Fusarium Growth and Mycotoxin Production in Cereals? Food Addit. Contam. Part A 2018, 35, 2455–2470. DOI: 10.1080/19440049.2018.1538570.
- Laitila, A.; Sweins, H.; Vilpola, A.; Kotaviita, E.; Olkku, J.; Home, S.; Haikara, A. Lactobacillus plantarum and Pediococcus pentosaceus Starter Cultures as a Tool for Microflora Management in Malting and for Enhancement of Malt Processability. J. Agric. Food Chem. 2006, 54, 3840–3851. DOI: 10.1021/jf052979j.
- Crowley, S.; Mahony, J.; van Sinderen, D. Current Perspectives on Antifungal Lactic Acid Bacteria as Natural Bio-Preservatives. Trends Food Sci. Technol. 2013, 33, 93–109. DOI: 10.1016/j.tifs.2013.07.004.
- Oliveira, P. M.; Zannini, E.; Arendt, E. K. Cereal Fungal Infection, Mycotoxins, and Lactic Acid Bacteria Mediated Bioprotection: From Crop Farming to Cereal Products. Food Microbiol. 2014, 37, 78–95. DOI: 10.1016/j.fm.2013.06.003.
- Peyer, L. C.; De Kruijf, M.; O’Mahony, J.; De Colli, L.; Danaher, M.; Zarnkow, M.; Jacob, F.; Arendt, E. K. Lactobacillus brevis R2Δ as Starter Culture to Improve Biological and Technological Qualities of Barley Malt. Eur. Food Res. Technol. 2017, 243, 1363–1374. DOI: 10.1007/s00217-017-2847-9.
- Schmidt, M.; Lynch, K. M.; Zannini, E.; Arendt, E. K. Fundamental Study on the Improvement of the Antifungal Activity of Lactobacillus reuteri R29 through Increased Production of Phenyllactic Acid and Reuterin. Food Control. 2018, 88, 139–148. DOI: 10.1016/j.foodcont.2017.11.041.
- Franco, T. S.; Garcia, S.; Hirooka, E. Y.; Ono, Y. S.; Santos, J. S. Dos Lactic Acid Bacteria in the Inhibition of Fusarium graminearum and Deoxynivalenol Detoxification. J. Appl. Microbiol. 2011, 111, 739–748. DOI: 10.1111/j.1365-2672.2011.05074.x.
- Pan, D.; Mionetto, A.; Tiscornia, S.; Bettucci, L. Endophytic Bacteria from Wheat Grain as Biocontrol Agents of Fusarium graminearum and Deoxynivalenol Production in Wheat. Mycotoxin Res. 2015, 31, 137–143. DOI: 10.1007/s12550-015-0224-8.
- Oliveira, P.; Brosnan, B.; Jacob, F.; Furey, A.; Coffey, A.; Zannini, E.; Arendt, E. K. Lactic Acid Bacteria Bioprotection Applied to the Malting Process. Part II: Substrate Impact and Mycotoxin Reduction. Food Control 2015, 51, 444–452. DOI: 10.1016/j.foodcont.2014.11.011.
- Perczak, A.; Goliński, P.; Bryła, M.; Waśkiewicz, A. The Efficiency of Lactic Acid Bacteria against Pathogenic Fungi and Mycotoxins. Arhiv Za Higijenu Rada I Toksikologiju 2018, 69, 32–45. DOI: 10.2478/aiht-2018-69-3051.
- Morimura, H.; Ito, M.; Yoshida, S.; Koitabashi, M.; Tsushima, S.; Camagna, M.; Chiba, S.; Takemoto, D.; Kawakita, K.; Sato, I. In Vitro Assessment of Biocontrol Effects on Fusarium Head Blight and Deoxynivalenol (DON) Accumulation by DON-Degrading Bacteria. Toxins 2020, 12, 399. DOI: 10.3390/toxins12060399.
- Felšöciová, S.; Kowalczewski, P. Ł.; Krajčovič, T.; Dráb, Š.; Kačániová, M. Quantitative and Qualitative Composition of Bacterial Communities of Malting Barley Grain and Malt during Long-Term Storage. Agronomy 2020, 10, 1301. DOI: 10.3390/agronomy10091301.
- Rezzonico, F.; Smits, T. H. M.; Born, Y.; Blom, J.; Frey, J. E.; Goesmann, A.; Cleenwerck, I.; de Vos, P.; Bonaterra, A.; Duffy, B.; Montesinos, E. Erwinia gerundensis Sp. Nov., a Cosmopolitan Epiphyte Originally Isolated from Pome Fruit Trees. Int. J. Syst. Evol. Microbiol. 2016, 66, 1583–1592. DOI: 10.1099/ijsem.0.000920.
- Ghim, S.-Y.; Jin-Soo, S.; Seung-Kun, K. Erwinia gerundensis KUDC9201 Strain Having Antifungal Activity against Pathogens and Uses Thereof. Patent Summary 2018 and Available at Erwinia gerundensis KUDC9201 Strain Having Antifungal Activity against Pathogens, and Uses Thereof. Patent KR-20190086947-A - PubChem (nih.gov)
- French Ministry of Agriculture and Food. Detection and Identification of Fusarium spp. and Microdochium nivale Species on Cereal Grains by Semi-Selective Mycological Isolation and Microbiological Study. Ref. MH.03-16: Version B); Malzéville, France, 2008.
- Petatán-Sagahón, I.; Anducho-Reyes, M. A.; Silva-Rojas, H. V.; Arana-Cuenca, A.; Tellez-Jurado, A.; Cárdenas-Álvarez, I. O.; Mercado-Flores, Y. Isolation of Bacteria with Antifungal Activity against the Phytopathogenic Fungi Stenocarpella maydis and Stenocarpella macrospora. Int. J. Mol. Sci. 2011, 12, 5522–5537. DOI: 10.3390/ijms12095522.
- Burmeister, H. R.; Plattner, R. D. Enniatin Production by Fusarium tricinctum and Its Effect on Germinating Wheat Seeds. Phytopathology 1987, 77, 1483–1487. DOI: 10.1094/Phyto-77-1483.
- Wang, J.-P.; Debbab, A.; Hemphill, C. F. P.; Proksch, P. Optimization of Enniatin Production by Solid-Phase Fermentation of Fusarium tricinctum. Z. Naturforschung C 2013, 68, 223–230. DOI: 10.1515/znc-2013-5-608.
- Suchfort, R. G. Biological Detoxification of Enniatins. Doctoral thesis, University of Göttingen, Göttingen, Germany, 2016.
- Lagashetti, A. C.; Dufossé, L.; Singh, S. K.; Singh, P. N. Fungal Pigments and Their Prospects in Different Industries. Microorganisms 2019, 7, 604. DOI: 10.3390/microorganisms7120604.
- Roig, M.; Meca, G.; Ferrer, E.; Mañes, J. Reduction of the Enniatins A, A1, B, B1 by an in Vitro Degradation Employing Different Strains of Probiotic Bacteria: Identification of Degradation Products by LC-MS-LIT. Toxicon 2013, 70, 44–53. DOI: 10.1016/j.toxicon.2013.04.001.
- Meca, G.; Roig, M.; Ferrer, E.; Mañes, J. Degradation of the Bioactive Compounds Enniatins A, A1, B, B1 Employing Different Strains of Bacillus subtilis. J. Food Process. Technol. 2014, 5, 334. DOI: 10.4172/2157-7110.1000334.
- Strub, C.; Pocaznoi, D.; Lebrihi, A.; Fournier, R.; Mathieu, F. Influence of Barley Malting Operating Parameters on T-2 and HT-2 Toxinogenesis of Fusarium langsethiae, a Worrying Contaminant of Malting Barley in Europe. Food Addit. Contam. Part A 2010, 27, 1247–1252. DOI: 10.1080/19440049.2010.487498.
- Hu, L.; Gastl, M.; Linkmeyer, A.; Hess, M.; Rychlik, M. Fate of Enniatins and Beauvericin during the Malting and Brewing Process Determined by Stable Isotope Dilution Assays. LWT - Food Sci. Technol. 2014, 56, 469–477. DOI: 10.1016/j.lwt.2013.11.004.
- Ng, C. A.; Pernica, M.; Yap, J.; Belakova, S.; Vaculova, K.; Branyik, T. Biocontrol Effect of Pythium oligandrum on Artificial Fusarium Culmorum Infection during Malting of Wheat. J. Cereal Sci. 2021, 100, 103258. DOI: 10.1016/j.jcs.2021.103258.
- Saldierna Guzmán, J. P.; Reyes-Prieto, M.; Hart, S. C. Characterization of Erwinia gerundensis A4, an Almond-Derived Plant Growth-Promoting Endophyte. Front. Microbiol. 2021, 12, 687971. DOI: 10.3389/fmicb.2021.687971.
- Moynié, L.; Milenkovic, S.; Mislin, G. L. A.; Gasser, V.; Malloci, G.; Baco, E.; McCaughan, R. P.; Page, M. G. P.; Schalk, I. J.; Ceccarelli, M.; Naismith, J. H. The Complex of Ferric-Enterobactin with Its Transporter from Pseudomonas aeruginosa Suggests a Two-Site Model. Nat. Commun. 2019, 10, 3673 DOI: 10.1038/s41467-019-11508-y.
- Sulochana, M. B.; Jayachandra, S. Y.; Kumar, S. K. A.; Dayanand, A. Antifungal Attributes of Siderophore Produced by the Pseudomonas aeruginosa JAS-25. J. Basic Microbiol. 2014, 54, 418–424. DOI: 10.1002/jobm.201200770.
- Kusano, T.; Berberich, T.; Tateda, C.; Takahashi, Y. Polyamines: Essential Factors for Growth and Survival. Planta 2008, 228, 367–381. DOI: 10.1007/s00425-008-0772-7.
- Fu, X.-Z.; Chen, C.-W.; Wang, Y.; Liu, J.-H.; Moriguchi, T. Ectopic Expression of MdSPDS1 in Sweet Orange (Citrus sinensis Osbeck) Reduces Canker Susceptibility: Involvement of H₂O₂ production and transcriptional alteration. BMC Plant Biol. 2011, 11, 55 DOI: 10.1186/1471-2229-11-55.
- Moselhy, S. S.; Asami, T.; Abualnaja, K. O.; Al-Malki, A. L.; Yamano, H.; Akiyama, T.; Wada, R.; Yamagishi, T.; Hikosaka, M.; Iwakawa, J.; et al. Spermidine, a Polyamine, Confers Resistance to Rice Blast. J. Pestic. Sci. 2016, 41, 79–82. DOI: 10.1584/jpestics.D16-008.
- Kaur, R.; Macleod, J.; Foley, W.; Nayudu, M. Gluconic Acid: An Antifungal Agent Produced by Pseudomonas Species in Biological Control of Take-All. Phytochemistry 2006, 67, 595–604. DOI: 10.1016/j.phytochem.2005.12.011.