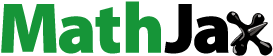
Abstract
Objective
The aim of this study was to use an alternative granulation technique, solventless roll compaction, and to investigate the effect of the roll compaction pressure on the properties of granules and high-drug-loaded (80%, w/w) immediate release piracetam tablets.
Significance
Piracetam is commonly manufactured as high drug-loaded tablets by wet granulation with an aqueous binder solution. Due to its high solubility in water, the wet granulation process is largely susceptible to processing methods and can induce the uncontrolled polymorphic transition of piracetam as well as convert it into mono- and di-hydrates.
Methods
The blends, comprising piracetam, Kollidon® 30, and Avicel® PH-101 were roll compacted at 4, 5 and 13 MPa hydraulic pressure and calibrated using an industrial roll compactor. The resultant granules milled and raw piracetam was investigated with DSC. The resultant granules are mixed with Ac-Di-Sol®, Aerosil® 200 Pharma, and magnesium stearate to prepare tablets using an industrial tablet press at the same compression force and 25, 65, and 100 rpm. The obtained tablets were film coated with an aqueous dispersion of Opadry® II using a pilot-scale solid-wall pan coater.
Results
Roll compaction pressure influenced the polymorphic composition of piracetam, the granule properties and tablet mixture in relation to morphology, particle size, flowability, bulk and tapped density, as well as tablet hardness, tablet friability, disintegration, and dissolution.
Conclusion
This study showed that roll compaction can be successfully used for the preparation of highly water-soluble, highly drug-loaded piracetam film-coated tablets avoiding wet granulation pitfalls.
Graphical Abstract
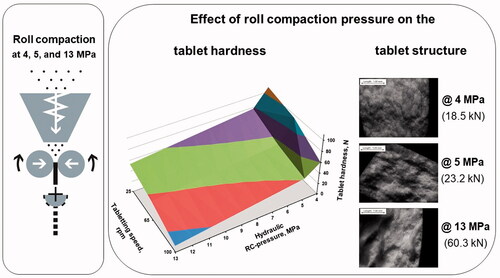
Introduction
Piracetam (2-oxo-1-pyrrolidine acetamide) is a cyclic derivative of gamma-aminobutyric acid, which is a weak base with a pKa of 13.6 [Citation1] and a high (479 mg/mL) aqueous solubility [Citation2,Citation3]. It is used clinically as a nootropic drug [Citation2,Citation4] and oral products of piracetam are usually in the form of coated high-drug-loaded immediate release tablets in the dose range of 200–1200 mg [Citation5].
Piracetam is prone to caking and clumping. To combat this [Citation6,Citation7] and avoid segregation during manufacturing steps while achieving the desired content uniformity [Citation8], piracetam tablets are typically manufactured by wet granulation. As piracetam is highly water-soluble, high piracetam concentrations are used in formulations, therefore the aqueous wet granulation process is very susceptible to process parameters and their variation [Citation3,Citation9]. Wet granulation can also induce the polymorphic transition of piracetam as well as convert it into mono- and di-hydrates [Citation10]. These factors complicate the process of development and scale-up, as well as production-scale batch-to-batch reproducibility. In this situation, dry granulation by roll compaction can become an alternative manufacturing method neutralizing the disadvantages linked to the wet granulation processing of piracetam.
Piracetam exists in a number of polymorphic forms. Polymorphic FIV and FV forms were reported to be only stable at high pressures [Citation11]. At atmospheric pressure, only three polymorphs (FI, FII, and FIII) of piracetam have been reported [Citation11,Citation12]. FII (triclinic) or FIII (monoclinic) polymorphic forms directly transform into FI upon heating and FI can be obtained after quench-cooling [Citation13]. During heating, FII transforms into FI at a slightly lower temperature compared to FIII, and the respective DSC-observed endotherms illustrate the process of FII-to-FI and FIII-to-FI transition, followed by the melting endotherm of FI [Citation14]. Nevertheless, obtained after quench-cooling FI is unstable and converts into FII within a few hours under ambient conditions [Citation13]. Under ambient conditions, the solid-state transformation from FII to FIII wasn’t found [Citation14], while the FIII form was recognized as more stable compared to FII [Citation15].
Typical processes such as milling/grinding and compression can cause conformational polymorph phase transitions because of localized increases in pressure and shear stress [Citation16]. Piracetam FIII-to-FII polymorphous transformations upon grinding were observed as well [Citation17]. Based on single crystal nanoindentation experiments, FIII has a higher hardness and elastic modulus compared to FII [Citation18]. Attempting to establish relationships between crystal structure and macroscopic physical properties of piracetam polymorphs, the tableting of FII was found as superior compared to FIII and explained with greater FII plasticity [Citation18].
Dry granulation by roll compaction is an alternative way of granulation and it is getting more popular for manufacturing pharmaceutical solid oral dosage forms [Citation19–22]. During the process, roll compaction pressure improves the contact area and powder particles are aggregated due to direct contact bonding forces between the solid surfaces [Citation22,Citation23]. Being a continuous method, roll compaction has less processing risk because of in-process adjustable parameters and less critical manufacturing steps, resulting in a less problematic scale-up process compared with batch granulation [Citation24]. Roll compactors have less physical size and footprint, decreasing cleaning time and contamination risk and capital investment than competing batch technologies. Roll compaction compared to wet granulation allows the process to avoid the addition of solvent or dissolved binder [Citation25], which is beneficial in the case of piracetam and allows for avoidance of uncontrolled polymorphic transitions and hydrates formation [Citation10,Citation26].
In accordance with the literature, roll compaction pressure is the most important process parameter affecting resulting ribbons and consequently granule properties. The effect of roll compaction pressure on the granule and tablet properties is highly pronounced and has been previously reported [Citation27–33], while in the context of piracetam, roll compaction pressure can additionally influence conformational polymorphism [Citation16,Citation17].
The aim of this study was to investigate the effect of roll compaction pressure on the piracetam polymorphism, the properties of granules, tablet-cores, and coated tablets during the preparation of high-drug-loaded (80%, w/w) immediate release piracetam tablets.
Materials and methods
Materials
Piracetam substance (CAS No.: 7491-74-9; MW 142.16 g/mol; purity 99.9%) was purchased from Yangzhou Pharmaceutical Co Ltd. (Yangzhou, PR China). Povidone (Kollidon® 30) has been used as a dry binder and was kindly provided by BASF SE (Ludwigshafen, Germany). Microcrystalline cellulose (Avicel® PH-101) and croscarmellose sodium (Ac-Di-Sol®; FMC Corporation, Philadelphia, PA, USA) were used as tablet filler and disintegrant, respectively. Colloidal silicon dioxide (Aerosil® 200 Pharma) was provided by Evonik AG (Darmstadt, Germany) and magnesium stearate gifted by Sudeep Pharma Pvt. Ltd. (Nandesari, Gujarat, India) was used as glidant and lubricant, respectively. Opadry® II which is the premix of hypromellose, macrogol 4000, lactose, triacetin and iron oxide yellow was provided by Colorcon (Dartford, UK). Chemicals used for the preparation of dissolution media were of Pharmacopeia grade and used as received.
Differential scanning calorimetry (DSC)
To investigate the thermal properties of piracetam substance before and after processing a heat-flux DSC (DSC Q20; TA Instruments, New Castle, DE, USA) was conducted to characterize thermal behavior. For measurement, samples were weighed (5–8 mg) into aluminum DSC pans and heated from 30 °C to 200 °C at 50 °C/min with a continuous purge of nitrogen gas at 50 ml/min.
Roll compaction
Before the granulation, because of the large crystal size (D50%=196 µm), piracetam was milled with a hammer mill (Bohle Turbo Mill; L.B. Bohle Maschinen und Verfahren GmbH, Ennigerloh, Germany) at 4000 rpm. For the dry granulation by roll compaction, piracetam, Kollidon® 30 and Avicel® PH-101 in the required proportions (in the amount of 1 kg; ) were mixed in a Turbula mixer (model T2F; Willy A. Bachofen AG, Muttenz, Switzerland) for 10 min then sieved (mesh size 2 mm) to avoid unmixed lumps and mixed again for 10 min. To obtain the batch size of 10 kg, the procedure was repeated 10 times, the mixture was collected in a PE bag, sealed, and then mixed manually for 10 min.
Table 1. The composition of granules and tablet core.
Dry granulation was utilized with an industrial roll compactor (model RC 170 × 45; Powtec Maschinen und Engineering GmbH, Remscheid, Germany) comprised of conveying, feeding and roll compacting, and milling units () [Citation34]. The powder mixture was delivered with a drum-feeder and moved vertically downward on rolls by a forced screw feeder with (45 mm diameter). The powder mixture was then compacted into the ribbon by rolling between counter-rotating compaction rolls at a predetermined compaction pressure. The roll compactor was equipped with smooth surface rolls (170 mm diameter, 45 mm width). Being a function of the roll force, roll speed and screw speed, the gap distance between the rolls varied (due to the moving roll) keeping the roll compression force close to the set value. The obtained compaction ribbon after rolling was crushed at 125 rpm with a rotor sieving mill equipped with a 1.0 mm size square apertures sized screen.
Figure 1. The schematic illustration of the roll compactor and the main functional parts: (1) vertical forced screw feeder; (2) compaction rolls; (3) rotor sieving mill.
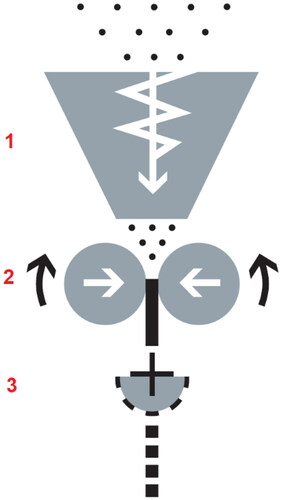
The described processing conditions (summarized in ) allowed the production of piracetam granules in a continuous mode. Prepared batches of granules were manufactured using the same processing conditions except for the applied hydraulic pressure. For three different batches, the applied hydraulic pressure was 4, 5 or 13 MPa (40, 50 or 130 bar) and proportional to the total roll compaction force of 18.5, 23.2 or 60.3 kN (calculated using the piston area of applied hydraulic pressure). The roll compaction productivity (kg/h) was measured by weighing granules collected every 2 min and presented as Av. (±SD) based on six measurements for every three batches. Resultant granules were collected, homogenized by mixing for 10 min in the Turbula mixer, tested to determine particle size distribution, bulk and tapped density, mass flow rate through the funnel, and used for the following tablets preparation.
Table 2. Summarized process conditions of granules manufacturing with industrial roll compactor.
Preparation of tablets
Before mixing, magnesium stearate and Aerosil® 200 Pharma were passed through a sieve with a 0.5 mm mesh. Roll compacted granules, Ac-Di-Sol® and Aerosil® 200 Pharma in required proportions (), were mixed in a Turbula mixer for 10 min. Then, magnesium stearate was added, and the premix was additionally mixed for 3 min. To obtain the batch size of 3 kg, the procedure was repeated 3 times, the mixture was collected in a PE bag, sealed, and then mixed manually for 3 min.
To obtain 200 mg piracetam dose biconvex tablets (diameter of 9 mm and with a radius of the hemisphere of 18 mm) with a weight of 250 mg (), the rotary tablet press with 12 punch stations and forced powder filler was utilized (model XL 100; Korsch AG, Berlin, Germany) at a constant force of 25 kN (half of the maximum recommended by iHolland Ltd. (Nottingham, UK) tableting force for this punch type) for tableting at 25, 65 and 100 rpm. Tableting parameters were the same except for tablet press rotor speed.
Weight uniformity
Twenty tablets were individually weighed, and the average (Av.) weight and weight relative standard deviation (R.SD) were calculated. Then, the individual tablet weights were compared to the mean and the difference (%) was calculated. In our case of 200-mg dose tablets (being in the range of 130–324 and 80–250 mg), to meet pharmacopeia requirements, not more than two of the individual weights deviated from the average weight by more than 7.5% and none deviate by more than 15%.
Film-coating of tablets
The premix of Opadry® II and deionized water were used for the preparation of coating suspensions with a 15% w/w concentration of solids [Citation35]. For the film coating experiment, three batches of piracetam tablet cores (2.8 kg of each batch) were coated in a laboratory solid-wall pan coater (model GS HT- 3 with a pan diameter of 450 mm [Citation36]; I.M.A. Industria Macchine Automatiche S.p.A., Bologna, Italy). The suspension was applied using one two-component 1-mm nozzle (model Schlick 931/7-1 S22; Schlick GmbH, Untersiemau, Germany). The distance between the nozzle and the flowing tablet bed was 120 mm. Utilizing specific coating process parameters (), 3% w/w film-coating of Opadry® II has been coated on the piracetam tablet cores.
Table 3. Parameters of the coating process.
Determination of particle size distribution by sieving method
Powder material (50 g), sieves with the mesh size of 100, 250, 500, 700, 1000 and 2000 µm and vibratory sieve shaker (AS 200 Basic; Retsch GmbH, Haan, Germany) at 1 mm amplitude for 5 min were used for the determination of particle size distribution (n = 3). D10%, D50%, and D90% were graphically extracted from the cumulative weight fraction which was generated from the raw data. The Span was used as an indicator of particle size distribution and calculated using the following equation:
Bulk and tapped density testing
To investigate the bulk and tapped density of the powder mixture before and after the granulation, as well as the tableting mixture, a Tapped Density Tester (PT-TD300; Pharma Test Apparatebau AG, Hainburg, Germany) was used. The bulk and tapped density of samples were investigated using 100 g of powder material and a graduated volumetric cylinder. The volume of material was visually recorded before tapping (bulk densities, ρbulk), and after 10 and 500 taps (tapped densities, ρtapped). All measurements were made in triplicate (n = 3). Bulk and tapped densities as well as Hausner ratio (HR) were calculated using the following equations [Citation37,Citation38].
Mass flow rate determination with gravitational funnel method
A stainless-steel frustum cone funnel was fixed in a strictly vertical position (flowability tester; Pharma Test Apparatebau AG, Hainburg, Germany). 100 g samples were weighed and introduced carefully into the funnel with a 10 mm diameter orifice. During filling, the bottom opening of the funnel was closed. Once the funnel was opened the time taken for the 100 g of powder within the funnel to flow out was measured (n = 6). The mass flow rate was calculated as mass per time, expressed in g/s [Citation37].
Determination of tablet friability
The friability test was conducted using an automatic drum tablet friability instrument (PTF 20E; Pharma Test Apparatebau AG, Hainburg, Germany) at a fixed rotation speed and test duration (25 rpm for 4 min). To measure the friability, 20 tablets (n = 20) were dedusted, weighed and loaded into the testing drum (n = 3). After the friability test, tablets were dedusted, weighed and the friability (weight loss) of tablets was calculated and expressed as a percentage of the initial weight % (w/w) [Citation39].
Determination of tablets disintegration time
Disintegration time was determined with a disintegration tester (Pharma Test Apparatebau AG, Hainburg, Germany) according to the European Pharmacopeia monograph 2.9.1. The disintegration of tablets and capsules [Citation40], in 900 ml demineralized water at 37 ± 0.5 °C. Six tablets (n = 6) were measured [Citation41].
Testing the drug release from the coated piracetam tablets
Dissolution testing was conducted using USP Apparatus II with a paddle agitation speed of 75 rpm (VK 7000, VanKel Industries, NJ, USA) in a 900 ml dissolution medium (0.1 N solution of hydrochloric acid pH 1.2, acetic buffer solution pH 4.5 or phosphate buffer solution pH 6.8). During the dissolution test, samples were taken at predetermined time points (5, 10, 15, 20 and 30 min) and filtered through a 0.22 μm Durapore® membrane filter (Merck KGaA, Darmstadt, Germany). Based on the comparator product dissolution profile, tablets should release not less than 85% of piracetam in 15 min [Citation5].
The quantification of piracetam in dissolution samples was performed by HPLC with UV-detector. The HPLC-column (250 mm × 4.6 mm) is filled with 5 µm size C18 (RP18, ODS, Octadecyl) silica particles (YMC-Pack ODS-AQ; YMC Co., Ltd., Ishikawa, Japan). After the injection of 10 µL of dissolution sample, the mobile phase of acetonitrile and water solution (1 g/L) of dipotassium hydrogen phosphate (10:90) adjusted to pH 6.0 ± 0.05 by water solution of phosphoric acid was used at 1.0 ml/min flow rate. The signal was detected at 205 nm, and the concentration of piracetam was determined in accordance with the area under the piracetam peak in comparison with the standard solution. The released drug amount in percentage (presented as Av.±SD of twelve (n = 12) tested tablets) was plotted versus time.
Results
To improve the homogeneity of the formulation on the micro-level the raw piracetam substance was milled (). After milling, the particle size (D50%) of piracetam was reduced from 196 µm to 115 µm (). As a result of the milling process, along with particle size reduction, the span of particle size was increased from 1.6 to 2.6 (). The higher span of particle size for milled piracetam and wider particle size distribution was confirmed with light microscopy imaging (). For the following dry granulation using roll compaction, the milled piracetam substance (83.54%, w/w) was mixed with polymeric crystalline diluent Avicel® PH-101 (13.12%, w/w) and polymeric amorphous binder Kollidon® 30 (3.34%, w/w; ). To obtain granules, the resultant mixture was rolled compacted into ribbons and milled with a rotor sieving mill. The productivity of granule production was measured at the level of 39(±0.5) kg/h ().
Figure 3. Particle size distribution (A and C) and cumulative weight fractions (B and D) of piracetam substance before and after milling (A and B) and roll compacted granulates (C and D).
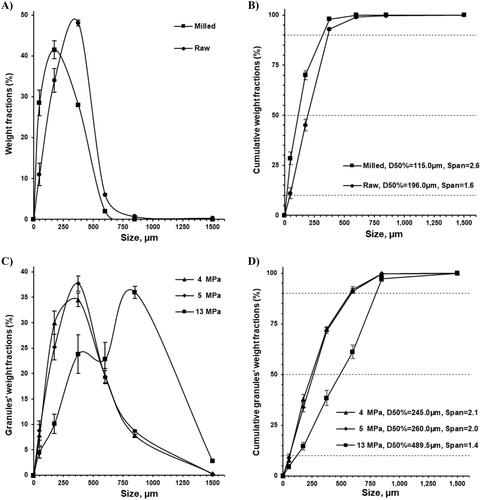
To investigate the polymorphic transformation from raw, to milled as well as to roll compacted and then milled piracetam, the DSC method has been used for the characterization of the respective samples. DSC curves of all samples showed two clear endothermic events. While the second endotherm with relatively the same values of the peak in the range between 157.8 and 159.9 °C appeared in the same temperature range, the first endotherm was different in terms of onset temperature and peak (, ). For the first endotherm, the onset (140.7, 126.0, and 116.4 °C) and peak temperature (143.7, 131.7 and 122.5 °C) decrease from raw, to milled as well as to roll compacted and then milled piracetam formulation.
Table 4. Endotherm onset and peak temperatures for raw, milled and for roll compacted and then milled piracetam.
Granules prepared at roll compaction force of 4, 5 and 13 MPa had granules sizes (D50%) of 245, 260 and 489.5 µm, respectively (). D10%, D50%, and D90% increased along with roll compression pressure (). Comparing average particle size (D50%), granules prepared at the roll compaction force of 13 MPa were 229.5–244.5 µm bigger (489.5 µm vs. 260 and 245 µm), while D90% and D10% were 212.5–221.5 µm and 59–62 µm bigger, respectively (). Granules prepared at 4 and 5 MPa both having a particle distribution profile peak at 375 µm were very similar in terms of granule size and visual appearance ().
Figure 6. Light microscopy of roll compacted granules, cross-section (after determination of tablets hardness) and the surface of tablets and surface of coated tablets prepared at different roll compaction pressure and tableting at 25 kN.
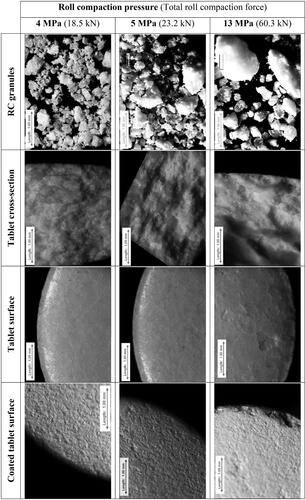
The bulk density (670 and 676 g/mL) and density after 10 taps (714 and 709 g/mL) were similar for roll compacted granules at 4 and 13 MP (). Also, the densification kinetics between 10 and 500 taps was faster for roll compacted granules at 4 MP compared to 13 MP (800 vs 769 g/mL, respectively). The bulk and tapped density at 10 taps for roll compacted granules at 5 MP was comparatively lower (633 and 690 g/mL) and the densification kinetics between 10 and 500 taps was comparable with roll compacted granules at 4 MP resulting in 769 g/mL tapped density equal to roll compacted granules at 13 MP. The bulk density and the density after 10 taps for the non-granulated powder mixture were the lowest (588 and 625 g/mL), while the densification kinetics between 10 and 500 taps was the fastest and resulted in 801 g/mL tapped density equal to the tapped density of roll compacted granules at 4 MP.
Figure 7. The effect of tapping on the density of roll compacted granules (RC granules) and the mixture which is intended for the tableting (tablet-mix).
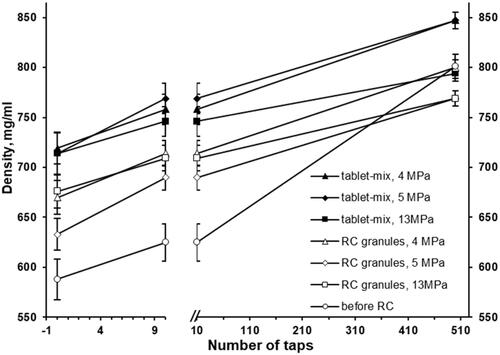
To prepare the tableting mixture, granules (95.76%, w/w) were mixed with disintegrant Ac-Di-Sol® (3.2%, w/w), glidant Aerosil® 200 Pharma (0.4%, w/w) and lubricant magnesium stearate (0.64%, w/w). The resultant concentration of piracetam in the obtained mixture reached 80,0%, w/w ().
The bulk density for all tablet mixtures with the roll compacted granules at 4, 5, and 13 MP was similar (719, 714, and 714 g/mL, respectively) and was higher than the bulk density of the non-granulated powder mixture and bulk density of roll compacted granules (). The values of density after 10 taps for tablet mixtures with the roll compacted granules at 4, 5, and 13 MP were very close to each other (758, 769, and 746 g/mL, respectively) and higher than the density of non-granulated powder mixture and bulk density of roll compacted granules. The densification kinetics between 10 and 500 taps of the tablet mixtures with roll compacted granules at 4 and 5 MP was almost the same and resulted in the same tapped density of 847 g/mL.
In general, the Hausner ratio of granules and tableting mixtures was decreased along with roll compaction pressure increase (). The Hausner ratio of granules was systematically higher than for tableting mixtures. The non-granulated powder mixture could not flow through the 10 mm orifice. While the flowability (mass flow rate) of granules and tableting mixtures was increased along with roll compaction pressure () and granule particle size (D50%) (). The flowability increase for tableting mixtures was more pronounced in comparison with roll compacted granules.
Figure 8. The effect of roll compaction pressure on the mass flow rate (FR) and Hausner ratio (HR) of roll compacted granules and the mixture which is intended for the tableting.
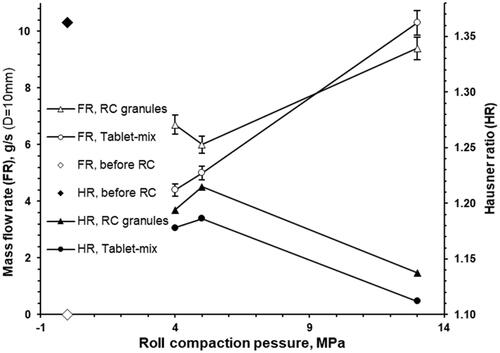
To prepare tablet cores (), tableting mixtures were compressed into tablets using a rotary tablet press Korsch XL-100. At the same tableting force (25 kN), the appearance of the tablet cross-section was different for tablets based on granules obtained at different roll compaction pressure. The roughness and fragmentation of the tablet cross-section increased with roll compaction pressure increase (). The surface of tablets prepared from tableting mixtures based on the roll compacted granules at 4 and 5 MPa was smooth and visually almost the same but different compared with tablets based on the roll compacted granules at 13 MPa (). The surface of the last was rough and fragmented.
Tableting mixtures based on roll compacted granules at 4, 5 and 13 MPa were compressed into tablets at the same tableting force of 25 kN at 25, 65 and 100 rpm, and their hardness was tested and plotted versus roll compaction pressure and tableting speed (). The increasing roll compaction pressure resulted in a non-linear tablet hardness decrease at every tableting speed (). The increases in tableting speed from 25 to 65 and 100 rpm resulted in an almost linear tablet hardness decrease for every roll compaction pressure (). The tablet hardness increased along with tableting speed and roll compaction pressure decreased (). It should be mentioned, that for all preparations and tableting speed, the uniformity of tablet weight met pharmacopeia requirements, with not more than 2.5% R.SD value.
Figure 9. The tablet hardness is a function of (A) roll compaction pressure; (B) tableting speed and; (C) roll compaction pressure and tableting speed.
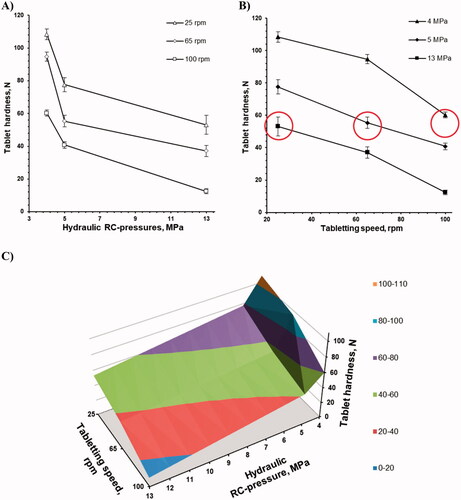
The friability of tablets is related to the microstructure and hardness of the tablet and from a practical point of view, the hardness of tablets should be high enough to allow tablets to fit the friability test. To compare the effect of the roll compaction pressure on the friability, coating process and drug dissolution profile, tablets with approximately equal hardness (circled in ) were used. Being tested using the pharmacopeial friability test, all tablets demonstrated good mechanical resistance supported with weight loss values lower than 0.15% for tablets based on roll compacted granules at 4, 5 and 13 MPa.
Tablets were coated with a hypromellose-based coating Opadry II using pilot-scale solid-pan-coater at a batch size of 3 kg. Despite the good results of the pharmacopeia friability test, tablets based on the roll compacted granules at 13 MPa demonstrated unsatisfactory friability during the coating process in relation to the chipped edge of tablets () and stuck chipped particles at the surface of other tablets (not more than approx. 5% of tablets had coating defects). Tablets based on the roll compacted granules at 4 and 5 MPa demonstrated good results in comparison due to no defects on the tablet edge and the visually smooth and homogeneous coated surface of tablets (). Resulted tablets demonstrated 8-, 7- and 5-min disintegration time for tablets based on the roll compacted granules 4, 5 and 13 MPa, respectively.
The composition of dissolution media did not have a significant effect on the drug release from coated tablets. Tablets based on the roll compacted granules at 4 MPa showed almost the same immediate release profile at pH 1.2, 4.5 and 6.8, specifically, in every media more than 85% of piracetam was released in 10 min and 100% in 20 min (). The increase of roll compaction pressure from 4 to 5 MPa did not have a significant effect on the drug release from coated tablets (no data provided), while tablets based on granules prepared at 13 MPa (only tablets without coating defects were used) demonstrated faster drug release ().
Discussion
In order to increase the hardness of tablets, the raw piracetam substance was milled [Citation42]. High MCC content is commonly used as plastically deforming materials and is frequently used in roll compaction granulation [Citation23,Citation25,Citation43,Citation44]. Also, compared with wet granulation, the choices of binders for roller compaction are limited and one of the commonly used binders is povidone [Citation23,Citation45,Citation46]. In this work, only 3.34% and 13.12% (w/w) of plastically deforming under compression Kollidon® 30 and Avicel® PH-101, respectively, were used for the preparation of roll compacted granules (). Thus, the resulting concentration of piracetam comprised 83.54% (w/w) observing the high input of brittle piracetam substance into the properties of the processed formulation.
As far as Kollidon® 30 is amorphous and used in the relatively small amount (3.34% w/w) and melting of microcrystalline cellulose Avicel® PH-101 can be observed upon thermal degradation between 360 and 385 °C, for the processed piracetam formulation, the thermal events such as endotherms in the range of 35–200 °C can be attributed to piracetam only. The melting endotherms with respective melting peaks (Peak2) in the range between 157.8 and 159.2 °C on the DSC-profiles of raw, milled, as well as roll, compacted and then milled piracetam (, ) illustrated the occurrence of FI form because of FII or FIII form transition upon heating [Citation10,Citation14,Citation15]. The onset and peak temperature decrease of the first endotherm from raw, to milled as well as to roll compacted and then milled piracetam formulation showed the FIII-to-FII polymorph transition as a result of milling and followed roll compaction technological steps. Thus, the increase of the FII polymorph content along with the increase of roll compaction pressure can be concluded and the highest FII content was concluded at the highest roll compaction pressure.
The particle size of granules increased along with the increase of the roll compaction pressure (, ). Apart from that, the roll compacted granules at 4, 5 and 13 MPa (proportional to total roll compaction force of 18.5, 23.2 or 60.3 kN; “Roll compaction” method description) have shown different densification () and Hausner ratios (). The mixing of the roll compacted granules with 3.2% (w/w) of Ac-Di-Sol®, 0.4% (w/w) of Aerosil® 200 Pharma, and magnesium stearate improved the packing of particles and increased the bulk density of tablet mixtures. Compared to the decrease in bulk density of granules along with the increase of the roll compaction pressure and particle size, the mixing with additional excipients made the bulk density of tablet mixtures similar to the roll compacted granules at 4, 5 and 13 MPa. It was likely to be due to the decrease in interparticle friction because of the lubrication and glidant properties arising from the magnesium stearate and Aerosil® 200 Pharma properties [Citation37].
The densification kinetics during the determination of bulk density reflects the behavior of granulated powders at low compression which can occur during tablet manufacturing. For example, ten taps can reflect a short influence of vibration and five hundred taps, a comparatively long influence of vibration or properties of powder experienced the pressure of the same powder on top of it. Additionally, a comparison of bulk and tapped density (Hausner ratio) between different batches can give an understanding of the flowability of powder and internal cohesion/possibility of particle rearrangement, sliding, and restacking without particle deformation.
Hausner ratio for powders of 1.0–1.1 is classified as free-flowing, 1.1–1.25 as medium flowing, 1.25–1.4 as difficult flowing, and >1.4 as very difficult flowing [Citation47]. Additionally, the relationship between the Hausner ratio and flowability (defined as the ratio of the principal consolidation stress and the unconfined yield strength, which gives the flow function coefficient) is known from the literature [Citation48,Citation49]. Hausner ratio, which is an indirect measure of the flow character, changed from medium flowing for the roll compacted granules at 4 and 5 MPa to the higher level of the medium flowing for the roll compacted granules at 13 MPa. The same trend was observed for the respective tablet mixtures. The increase of powder flow properties along with the increase of roll compaction pressure from 4 to 13 MPa was additionally confirmed with the direct measurement of powder flowability (mass flow rate; ) and agrees with the literature [Citation50]. Additionally, it’s in the agreement with current understanding as to the flowability increase along with particle size increase because the flowability is dependent on the specific surface area [Citation51]. Thus, no “rat holing” or “bridging” was expected in the tablet press hopper and no negative effect of tablet mixture flowability on the weight uniformity of tablets was expected because of obtained values of Hausner ratio and high flowability [Citation52]. No powder flow problems occurred at Korsch XL 100 hopper loading with 3 kg of tablet mixture composed of the 4, 5, and 13 MPa roll compressed granules.
The lower tapped density of tablet mixture composed of the 13 MPa vs. 4 and 5 MPa roll compressed granules witnessing looser tapped structure, lower ability of particles rearrangement and/or less efficient filling the structure voids with fine particles. Thus, the tablet mixture composed of the 13 MPa was comparatively less susceptible to low pressure which is reflecting the initial part of tableting without particle fragmentation. This behavior of tablet mixture composed of the 13 MPa roll compressed granules can be explained by a larger particle size (D50% and D90%) and a smaller fraction and a larger size of fines (D10%) [Citation50].
Despite the recently reported superior tableting behavior of FII vs. FIII [Citation18], the tablet hardness of the 250-mg weight 200-mg dose 9-mm diameter biconvex tablets was decreased along with the increase of roll compaction pressure (with the increase of FII content) and tableting speed (). It is well known that particle size and press speed can influence the deformation mechanism [Citation53–56] as well as plastic and brittle materials are affected differently on the tablet hardness as a function of particle size and press speed [Citation57–61]. For the formulation with roll compacted granules, the loss of compatibility, especially at higher roll compaction forces has been previously reported, and the two main hypotheses as granule hardening and granule size enlargement were proposed [Citation31,Citation32,Citation61].
The looser tapped density of roll compacted granules at 13 MPa has already been mentioned above. For formulations with lower granule size (D50% and D90%) and lower size of fines (D10%) the specific surface area of granules is relatively higher than for formulations with a large granule size and higher size and a lower fraction of fines (4 and 5 MPa vs. 13 MPa, respectively). The higher specific surface area resulted in higher contact area during tableting and consequently in greater tablet hardness [Citation49].
At lower roll compaction pressure (4 and 5 MPa), roll compacted granules are more porous with relatively low solid fraction, consequently, they should be more susceptible to the tableting compression force which is resulting in the fragmentation and increase of specific surface area [Citation56]. The higher roll compaction pressure (13 MPa) resulted in a harder structure (the higher solid fraction and the lower porosity) of granules which allowed granules' structure to survive after compression into the tablet [Citation31,Citation62]. Interestingly, the signs of preserved granules can be observed on the microscopy of tablet surface and cross-section resulting after tablet hardness testing and they were increasing along with the increase of roll compaction pressure (). Thus, the roll compaction force should be sufficiently high to produce a granule with desired flow properties but as low as possible to avoid the loss in tablet hardness () [Citation63].
Roll compacted granules are porous, viscoelastic, nonhomogeneous, Mohr bodies [Citation27] and the hardness of tablets made from viscoelastic materials is substantially affected by tableting speed () [Citation60,Citation64].
The higher friability of tablets based on the roll compacted granules at 13 MPa during film-coating can be explained by tablet structure including survived granules [Citation65]. Thus, we can observe the chipped edge of tablets after the detachment of granules () because the bonds inside the granule are much stronger than between granules.
Microstructure plays a decisive role in the disintegration and drug release from the tablet [Citation65]. The particle size of the roll compacted granules and the deformation of granules during tableting is influencing the water ingress into the tablet [Citation66]. From another side, it’s logical to assume that the distribution of disintegrant in the tablet, which was produced from roll compacted granules at 4, 5 and 13 MPa was different. In the tablet mixture based on the roll compacted granules at 4 and 5 MPa vs. 13 MPa, the disintegrant should be distributed more evenly because of the smaller granule size. At the same time, in the tablet mixture based on the roll compacted granules at 13 MPa vs 4 and 5 MPa, the disintegrant clusters of disintegrant should be greater. Thus, considering the high solubility of piracetam, the faster disintegration time and drug release () for tablets based on the roll compacted granules at 13 MPa vs 4 and 5 MPa, can be explained by the microstructure of tablets, higher disintegration force of disintegrant clusters, and faster water ingression into the tablet.
Conclusion
This study showed that roll compaction can be successfully used for the preparation of highly water-soluble, highly drug-loaded piracetam film-coated tablets. In comparison with wet granulation, roll compaction allows to avoid water susceptible granulation process variations as well as the formation of hydrates and uncontrolled piracetam polymorphic transition.
Being a continuous process with controlling, monitoring, and in-process automated adjustment possibilities of roll compaction pressure, where every discrete portion of material undergoes very similar mechanical treatment, the roll compaction allows the production of piracetam granules in a reproducible way with roll compaction pressure-dependent properties.
The roll compaction pressure as a critical manufacturing factor, influenced the polymorphic composition of piracetam, the properties of granules including the morphology, particle size, flowability, bulk and tapped density (inc. Hausner ratio), as well as tablet hardness, disintegration, and dissolution of piracetam from tablets.
The investigation of roll compaction pressure on the properties of granules and tablets helps to find the tendency in the effect of roll compaction pressure increase on the mentioned properties and to make data-driven decisions regarding project-required process settings.
Acknowledgments
I would like to express my acknowledgment to Powtec Maschinen und Engineering GmbH (Remscheid, Germany) represented by Mr. Felix Tietzsch (B. Eng., project engineer) for the help during the preparation of this article (incl. ). And finally, I want to thank Mr. Nathan Scott (School of Biomedical Sciences, Ulster University, UK) for the improvement of the written English of this work.
Disclosure statement
No potential competing interest was reported by the authors.
References
- Subirats X, Redón L, Rosés M. Lipophilicity determination of acidic compounds: MEEKC as a reliable high-throughput methodology. Admet Dmpk. 2018;6(2):153–161.
- Wishart DS, Feunang YD, Guo AC, et al. DrugBank 5.0: a major update to the DrugBank database for 2018. Nucleic Acids Res. 2018;46(D1):D1074–D1082.
- Kuhs M, Moore J, Kollamaram G, et al. Predicting optimal wet granulation parameters for extrusion-spheronisation of pharmaceutical pellets using a mixer torque rheometer. Int J Pharm. 2017;517(1–2):19–24.
- Winblad B. Piracetam: a review of pharmacological properties and clinical uses. CNS Drug Rev. 2005;11(2):169–182.
- Saevels J, Braekeleer D, Corthout K. J. Piracetam preparations on the belgian market: a comparative study. J Pharm Belg. 2005;60(3):92–96.
- Briel T, Guebeli R, Bhattarai R, et al. Addressing handling challenges of chemicals by dry granulation. Darmstadt, Germany: Merck KGaA; 2020.
- Brockbank K, Armstrong B, Clayton J. Measurement and quantification of caking in excipients and food products with emphasis on the non-homogeneous interaction with ambient moisture. Particuology. 2021;56:75–83.
- Jakubowska E, Ciepluch N. Blend segregation in tablets manufacturing and its effect on drug content uniformity—a review. Pharmaceutics. 2021;13(11):1909.
- Fábregas JL, Cucala J. New approach to aqueous granulation of highly hydrosoluble drugs. Drug Dev Ind Pharm. 1987;13(7):1217–1227.
- Potter CB, Kollamaram G, Zeglinski J, et al. Investigation of polymorphic transitions of piracetam induced during wet granulation. Eur J Pharm Biopharm. 2017;119:36–46.
- Fabbiani FPA, Allan DR, Parsons S, et al. An exploration of the polymorphism of piracetam using high pressure. CrystEngComm. 2005;7(29):179.
- Fabbiani FPA, Allan DR, David WIF, et al. High-Pressure studies of pharmaceuticals: an exploration of the behavior of piracetam. Crystal Growth & Design. 2007;7(6):1115–1124.
- Céolin R, Agafonov V, Louër D, et al. Phenomenology of polymorphism, III:p,TDiagram and stability of piracetam polymorphs. J Solid State Chem. 1996;122(1):186–194.
- Maher A, Seaton CC, Hudson S, et al. Investigation of the solid-state polymorphic transformations of piracetam. Crystal Growth Design. 2012;12(12):6223–6233.
- Picciochi R, Diogo HP, da Piedade ME. Thermodynamic characterization of three polymorphic forms of piracetam. J Pharm Sci. 2011;100(2):594–603.
- Boldyrev VV. Mechanochemical modification and synthesis of drugs. J Mater Sci. 2004;39(16/17):5117–5120.
- Maher A. Crystal transformations and crystallisation methodologies: Polymorphic transformations of piracetam [Doctoral Thesis]. Limerick, Ireland: University of Limerick; 2013.
- Upadhyay PP, Mishra MK, Ramamurty U, et al. Structure–property correlations in piracetam polytypes. CrystEngComm. 2021;23(5):1226–1233.
- Kleinebudde P. Roll compaction/dry granulation: pharmaceutical applications. Eur J Pharm Biopharm. 2004;58(2):317–326.
- Dehont FR, Hervieu PM, Jerome E, et al. Briquetting and granulation by compaction new granulator-compactor for the pharmaceutical industry. Drug Dev Ind Pharm. 1989;15(14–16):2245–2263.
- Fayed ME, Otten L. Pressure agglomeration methods. Handbook of powder science and technology. New York, USA: Chapman & Hall; 1997.
- Miller RW. Roller compaction technology. In: Handbook of pharmaceutical granulation technology. Boca Raton (FL): Taylor & Francis Group, LLC; 2005. p. 187–218.
- Teng Y, Qiu Z, Wen H. Systematical approach of formulation and process development using roller compaction. Eur J Pharm Biopharm. 2009;73(2):219–229.
- Leuenberger H, Betz G. Granulation process control-production of pharmaceutical granules" the classical batch concept and the problem of scale-up. In: Salman AD, Hounslow MJ, Seville JPK, editors. Elsevier B.V.; 2007, p. 705–733.
- Taipale-Kovalainen K, Ketolainen J, Korhonen O, et al. Converting a batch based high-shear granulation process to a continuous dry granulation process; a demonstration with ketoprofen tablets. Eur J Pharm Sci. 2020;151:105381.
- Rehder S, Christensen NP, Rantanen J, et al. High-shear granulation as a manufacturing method for cocrystal granules. Eur J Pharm Biopharm. 2013;85(3 Pt B):1019–1030.
- Hiestand EN. Mechanical properties of compacts and particles that control tableting success. J Pharm Sci. 1997;86(9):985–990.
- Zinchuk AV, Mullarney MP, Hancock BC. Simulation of roller compaction using a laboratory scale compaction simulator. Int J Pharm. 2004;269(2):403–415.
- Farber L, Hapgood KP, Michaels JN, et al. Unified compaction curve model for tensile strength of tablets made by roller compaction and direct compression. Int J Pharm. 2008;346(1–2):17–24.
- Iyer RM, Hegde S, Dinunzio J, et al. The impact of roller compaction and tablet compression on physicomechanical properties of pharmaceutical excipients. Pharm Dev Technol. 2014;19(5):583–592.
- Mosig J, Kleinebudde P. Critical evaluation of root causes of the reduced compactability after roll compaction/dry granulation. J Pharm Sci. 2015;104(3):1108–1118.
- Sun CC, Kleinebudde P. Mini review: mechanisms to the loss of tabletability by dry granulation. Eur J Pharm Biopharm. 2016;106:9–14.
- Yu J, Xu B, Zhang K, et al. Using a material library to understand the impacts of raw material properties on ribbon quality in roll compaction. Pharmaceutics. 2019;11(12):662.
- Powtec. Roller compactors for dry granulation. POWTEC Maschinen und Engineering GmbH; 2005.
- Colorcon. Product Information – Opadry® II: Reconstitution. USA: Colorcon, Inc.; 2015.
- IMA. Solid-wall pan coater – Coating System GS HT-3 (IMA S.p.A.) Italy: I.M.A. Industria Macchine Automatiche S.p.A.; 2021. [cited 6 Apr 2021]. Available from https://ima.it/pharma/machine/gs-ht-he-hp/.
- Mohylyuk V, Styliari ID, Novykov D, et al. Assessment of the effect of cellets’ particle size on the flow in a Wurster fluid-bed coater via powder rheology. J D Deliv Sci Tec. 2019;54:101320.
- Grey RO, Beddow JK. On the hausner ratio and its relationship to some properties of metal powders. Powder Technol. 1969;2(6):323–326.
- Osei-Yeboah F, Sun CC. Validation and applications of an expedited tablet friability method. Int J Pharm. 2015;484(1–2):146–155.
- Eur.Ph. European pharmacopeia. 9th edn. ed. Strasbourg: Council of Europe; 2017.
- Berkenkemper S, Keizer HL, Lindenberg M, et al. Functionality of disintegrants with different mechanisms after roll compaction. Int J Pharm. 2020;584:119434.
- McKenna A, McCafferty DF. Effect on particle size on the compaction mechanism and tensile strength of tablets. J Pharm Pharmacol. 1982;34(6):347–351.
- Brudy J, Farrenkopf J, Bultmann J. Influence of roll compaction force on pore structure and surface structure of MCC ribbons and tablets AAPS Annual Meeting and Exposition. Nashville, Tennessee, USA. 2005.
- Soh JL, Wang F, Boersen N, et al. Utility of multivariate analysis in modeling the effects of raw material properties and operating parameters on granule and ribbon properties prepared in roller compaction. Drug Dev Ind Pharm. 2008;34(10):1022–1035.
- Herting MG, Klose K, Kleinebudde P. Benchmark of different dry binders for roll compaction/dry granulation. Excipients Actives Pharma. 2008;20:6–7.
- Mangal H, Kirsolak M, Kleinebudde P. Roll compaction/dry granulation: suitability of different binders. Int J Pharm. 2016;503(1–2):213–219.
- Ortega-Rivas E. Unit operations of particulate solids: theory and practice. Boca Raton, FL, USA: CRC Press/Taylor & Francis Group; 2012.
- Saw HY, Davies CE, Paterson AHJ, et al. Correlation between powder flow properties measured by shear testing and hausner ratio. Procedia Eng. 2015;102:218–225.
- Hildebrandt C, Gopireddy SR, Fritsch AK, et al. Evaluation and prediction of powder flowability in pharmaceutical tableting. Pharm Dev Technol. 2019;24(1):35–47.
- Freeman T, Vom Bey H, Hanish M, et al. The influence of roller compaction processing variables on the rheological properties of granules. Asian J Pharm Sci. 2016;11(4):516–527.
- Hou H, Sun CC. Quantifying effects of particulate properties on powder flow properties using a ring shear tester. J Pharm Sci. 2008;97(9):4030–4039.
- Zettler A, Hilden J, Koenig M, et al. Evaluation of small-scale powder flow characterization tests in the prediction of large-scale process failures. J Pharm Innov. 2016;11(3):189–199.
- Fell JT, Newton JM. Effect of particle size and speed of compaction on density changes in tablets of crystalline and spray-dried lactose. J Pharm Sci. 1971;60(12):1866–1869.
- Ishino R, Yoshino H, Hirakawa Y, et al. Influence of tabletting speed on compactibility and compressibility of two direct compressible powders under high speed compression. Chem Pharm Bull. 1990;38(7):1987–1992.
- Rees JE, Rue PJ. Time-dependent deformation of some direct compression excipients. J Pharm Pharmacol. 1978;30(10):601–607.
- Skelbæk-Pedersen AL, Vilhelmsen TK, Wallaert V, et al. Investigation of the effects of particle size on fragmentation during tableting. Int J Pharm. 2020;576:118985.
- Rowlings CE, Wurster DE, Ramsey PJ. Calorimetric analysis of powder compression: II. The relationship between energy terms measured with a compression calorimeter and tableting behavior. Int J Pharm. 1995;116(2):191–200.
- Coffin-Beach DP, Gary Hollenbeck R. Determination of the energy of tablet formation during compression of selected pharmaceutical powders. Int J Pharm. 1983;17(2–3):313–324.
- Wurster DE, Rowlings CE, Creekmore JR. Calorimetric analysis of powder compression: I. Design and development of a compression calorimeter. Int J Pharm. 1995;116(2):179–189.
- Tye CK, Sun CC, Amidon GE. Evaluation of the effects of tableting speed on the relationships between compaction pressure, tablet tensile strength, and tablet solid fraction. J Pharm Sci. 2005;94(3):465–472.
- Skelbæk-Pedersen AL, Vilhelmsen TK, Rantanen J, et al. The relevance of granule fragmentation on reduced tabletability of granules from ductile or brittle materials produced by roll compaction/dry granulation. Int J Pharm. 2021;592:120035.
- Mitra B, Chang J, Hilden J, et al. Deformation potential and tensile strength of tablets of a dry granulated formulation. J Pharm Sci. 2021;111(3):710–716.
- Hakanen A, Laine E. Acoustic characterization of a microcrystalline cellulose powder during and after its compression. Drug Dev Ind Pharm. 1995;21(13):1573–1582.
- Chandler HW, Sands CM, Song JH, et al. A plasticity model for powder compaction processes incorporating particle deformation and rearrangement. Int J Solids Struct. 2008;45(7–8):2056–2076.
- Sun CC. Microstructure of tablet-pharmaceutical significance, assessment, and engineering. Pharm Res. 2017;34(5):918–928.
- Skelbæk-Pedersen AL, Al-Sharabi M, Vilhelmsen TK, et al. Effect of particle size and deformation behaviour on water ingress into tablets. Int J Pharm. 2020;587:119645.