Abstract
The epidemiology and control of powdery mildew (Oidium neolycopersici) of tomato (Solanum lycopersicum L.) was studied in greenhouse, growth chamber, and field experiments at Harrow, Ontario. Greenhouse environmental conditions were more favourable for disease development, with higher disease progress rates than in the field. Symptoms were more severe on older, lower leaves where the air temperature was slightly lower and relative humidity higher than on young leaves at the top of the canopy. A temperature of 22°C with 16 or 24 h of leaf wetness was optimal for disease development in a growth chamber experiment. Early inoculation resulted in significantly greater final disease severity and area under the disease progress curve than later inoculations. Disease progress in greenhouse and growth chamber trials was best described by the Gompertz model, while the logistic model was best for disease progress in field studies and for sections of plants in greenhouse studies. No yield loss occurred in field studies. The best disease control was obtained with acibenzolar-S-methyl (Actigard®), trifloxystrobin (Flint®), JMS-Stylet Oil®, azoxystrobin (Quadris®) and sulphur applied as protectants. Some phytotoxicity occurred with Actigard in greenhouse studies. Serenade® (Bacillus subtilis) was less effective while Kaligreen, Sporodex® (Pseudozyma flocculosa), copper octanoate and fumed silica were ineffective. Quinoxyfen was phytotoxic at the rate applied. Several tobacco cultivars, jimsonweed, American and black nightshade were susceptible to infection by O. neolycopersici. Eggplant, hairy nightshade and bittersweet were less susceptible, while no visible symptoms developed on pepper, potato and tomato ‘Grace’, and only trace levels of sporulation occurred on cucumber.
Résumé
L’épidémiologie et la gestion du blanc (Oidium neolycopersici) de la tomate (Solanum lycopersicum L.) ont été étudiées au cours d’expériences menées en serre, en chambre de culture et en champ à Harrow, en Ontario. En serre, les conditions ambiantes étaient plus favorables au développement de la maladie, affichant des taux de progression plus élevés qu’en champ. Les symptômes étaient plus graves sur les vieilles feuilles du bas, où la température de l’air était légèrement plus basse et l’humidité relative, plus élevée, que sur les jeunes feuilles situées dans le haut du plant. En chambre de culture, une température de 22°C, avec une mouillure du feuillage de 16 ou 24 heures, était optimale en ce qui a trait au développement de la maladie. Les inoculations hâtives ont engendré une gravité finale de la maladie significativement plus intense et une plus grande aire sous la courbe de progression de la maladie que les inoculations tardives. Le modèle de Gompertz a décrit le mieux la progression de la maladie lors des essais menés en serre et en chambre de culture, tandis que le modèle logistique convenait davantage à la progression de la maladie lors des études sur le terrain et lors de celles relatives à des sections de plants en serre. Aucune perte de rendement n’a été subie durant les expériences en champ. La maladie a été plus efficacement gérée avec de l’acibenzolar-S-méthyl (Actigard®), de la trifloxystrobine (Flint®), JMS-Stylet Oil®, de l’azoxystrobine (Quadris®) et du soufre, appliqués comme phytoprotecteurs. Au cours des études en serre, nous avons relevé une certaine phytotoxicité avec Actigard. Serenade® (Bacillus subtilis) était moins efficace, tandis que Kaligreen, Sporodex® (Pseudozyma flocculosa), l’octanoate de cuivre et la silice sublimée étaient inefficace. Au taux appliqué, le quinoxyfen était phytotoxique. Certains cultivars de tabac, la stramoine commune, la morelle américaine et la morelle noire étaient vulnérables à l’infection causée par O. neolycopersici. L’aubergine, la morelle faux-sarracha et la morelle douce-amère étaient moins vulnérables, tandis qu’aucun symptôme visible ne s’est développé sur le poivron, la pomme de terre et la tomate ‘Grace’. Seulement quelques traces de sporulation sont apparues sur le concombre.
Introduction
Powdery mildew of tomato has been reported on greenhouse and field tomatoes in many countries (Whipps et al. Citation1998; Mieslerová & Lebeda Citation1999; Kiss et al. Citation2001; Park et al. Citation2010). A worldwide study, which included samples submitted from Ontario, identified the fungus causing tomato powdery mildew in Canada and all recent outbreaks from outside Australia as Oidium neolycopersici Kiss (Kiss et al. Citation2001, Citation2005). The disease was observed for the first time in Canada on greenhouse tomatoes in 1994 (Bélanger & Jarvis Citation1994). It has become more widespread in Ontario and BC greenhouses since then. Currently, it is a major concern to growers of greenhouse tomatoes in Ontario, the principal region of greenhouse tomato production in Canada, where 347 ha were grown in 2011, representing 64% of national production, and with a corresponding market value of $ 317.4 million (Agriculture & Agri-Food Canada, http://www.agr.gc.ca/pmc-cropprofiles). The disease was first observed on processing tomato varieties in the field at the Greenhouse and Processing Crops Research Centre (GPCRC), Harrow, ON in 1996–1998, where considerable foliar damage occurred, necessitating the application of microfine sulphur to prevent further disease development. The disease was also present at low levels in two commercial fields in 1997–98. Currently, powdery mildew poses a threat to yield, quality and production of greenhouse tomatoes in the region. This disease also has the potential to reduce yield of field crops, since all processing tomatoes in Essex and Kent counties are started in the greenhouse as transplants (Anonymous Citation2006). Damage to field-grown tomatoes by O. lycopersici has been reported in Europe (Mieslerová & Lebeda Citation1999), Florida (Pernezny & Sonoda Citation1998) and Connecticut (LaMondia et al. Citation1999). In south-western Ontario, where the majority of the processing tomato production in Canada occurs, the potential for the cycling of inoculum from the greenhouse in spring and summer seasons to the field and back to the greenhouse in the autumn season exists because of the aerial dispersal of inoculum and the proximity of commercial processing tomato fields to commercial greenhouse tomato operations in the Leamington, ON area.
Management options for control of powdery mildew of tomato in the greenhouse are limited and growers often depend on fungicide use. In Canada, there are several fungicides – microfine sulphur, myclobutanil and potassium bicarbonate – which are registered for use on greenhouse tomato. Sulphur is rarely applied to this crop because of phytotoxicity, particularly during warm weather, and some harmful effects on beneficial organisms (Koppert Biological Systems Citation2010); therefore growers depend upon a limited number of fungicides for disease control. Several greenhouse growers have reported decreased efficacy of myclobutanil for tomato powdery mildew control (Cerkauskas, unpublished), in agreement with previous reports of reduced efficacy of myclobutanil for control of powdery mildew of cucumber caused by Podosphaera (sect. Sphaerotheca) xanthii (Castagne) U. Braun & N. Shishkoff (syn. Sphaerotheca fusca (Fr.) S. Blumer and S. fuliginea (Schlechtend.:Fr.) Pollacci) in greenhouses (Cerkauskas & Ferguson Citation2014) and field production (McGrath et al. Citation1996). Alternative fungicides, biopesticides and other materials with a different chemistry and a different mode of action are required to enable growers to practice rotation as a resistance management strategy and to reduce pesticide levels on food crops.
The objectives of this study were to: (i) examine the potential for powdery mildew development on tomato in Ontario in greenhouse, field and growth chamber experiments under varying environmental conditions, (ii) to examine effects of disease on yield under Ontario field conditions, (iii) to determine host range on other solanaceous plants that commonly occur in south-western Ontario, and (iv) to investigate means of disease management. Preliminary accounts of this work have been reported (Cerkauskas et al. Citation1999, Citation2000).
Materials and methods
Greenhouse studies – disease development
‘Trust’ tomato plants were grown in rock wool slabs (7.6 × 7.2 × 6.5 cm, L × W × H) (Fibrgro Horticultural Products, Sarnia, ON) in a greenhouse with temperature controls set at 21–23/17–19°C for 14 and 10 h, respectively, and relative humidity of 80–85% maintained by venting and misting. Greenhouse temperature was controlled by a computer system (Priva Computers Inc., Vineland Station, ON) that was interfaced to a Priva® sensor, located in the centre of the greenhouse at mid-plant height, and to the heating and ventilation systems. Microclimate monitoring was conducted in the middle plant row at the top, middle and bottom third of the canopy using a temperature and relative humidity probe (model Survivor II, Hy Cal Engineering, El Monte, CA) at each level. The probes were outfitted with a radiation shield consisting of two concentric polyvinyl chloride pipes connected to an aspirating fan so that air was drawn through the centre pipe of each radiation shield to aspirate the temperature-humidity probes. A data-logger (model CR21X, Campbell Scientific, Inc., Edmonton, AB) scanned the sensors for in-canopy temperature and relative humidity measurements every 15 s and output 15-min average values.
A hydroponic nutrient solution was used to fertilize plants according to standard commercial practice (Anonymous Citation1988). A planting density of 96 plants per greenhouse, with 8 rows of 12 plants each, was used with a spacing of 0.9 m between rows and a maximum plant height of 2.1 m.
Inoculum of O. neolycopersici was obtained from naturally infected ‘Trust’ tomato plants maintained in a separate greenhouse at the GPCRC. Experimental plants were inoculated in two separate experiments conducted at Harrow by shaking infected leaves over each healthy plant when there was an average of 24 and 12 nodes per plant. Foliar disease severity on three randomly selected plants in each row was rated 0, 7, 14, 21, 28, 35, 42, 52 days after inoculation for the first experiment, and 0, 7, 15, 22, 29, 36, 46 days after inoculation for the second experiment. The plants were rated for percentage leaf area with visible powdery mildew colonies using the Horsfall–Barratt rating scale (Horsfall & Barratt Citation1945), and the number of nodes on a representative of one of the three plants in each row was counted. To determine the location and extent of disease development, the top (T), middle (M) and bottom (B) third of each of these three plants were rated separately. The disease ratings were converted with the Elanco conversion tables (ELANCO Products Co., Indianapolis, IN, USA) to obtain percentage values for the midrange of each disease interval.
Field studies – disease development and yield
A field experiment was conducted at AAFC-GPCRC, Harrow, ON in a Fox sandy loam in 1997 and repeated in 1998 using tomato ‘Heinz 2478', a common processing cultivar. Treatments were arranged in a randomized complete block design with four replicates. The treatments consisted of four inoculation times with the pathogen, an unsprayed control, and a fungicide treatment. Each plot consisted of three rows, 3 m long, and 1.5 m apart, with 0.25 m spacing between plants. Transplants were set on 27 May in both years with a 2-row border of sweet corn (Zea mays var. rugosa Bonsf.) planted at the same time in a 1 m perimeter around each plot to reduce the potential for inter-plot interference. The middle row of tomatoes was inoculated with a spore suspension at four dates. Inoculum was prepared using greenhouse tomato leaves with severe powdery mildew development. These were placed in large beakers containing tap water, the mixture was swirled with a glass rod, strained through four layers of cheesecloth and adjusted to 104 spores mL−1 using a hemocytometer and diluted with tap water. To ensure uniform application for field inoculations, plants were sprayed to run-off immediately after inoculum preparation with a spore suspension containing 0.01% Tween 20 using a hand-operated sprayer (Solo Hanjet-455; Solo Kleinmotoren GMBH, Sindelfingen 6, Germany) with a flat spray nozzle at 138 kPa. In 1997, the tomato plants were inoculated 52, 76, 83 and 90 days after transplanting, and in 1998 at 47, 57, 71 and 85 days after transplanting. Fungicide check plots were protected both years with applications at weekly intervals of sulphur (92%, Micro-Fine; Chipman Chemical Co., Stoney Creek, ON) at 5.32 g product in 4.4 L water/subplot (3 × 3 m) applied using a Solo 425 backpack sprayer containing size 4074757 flat spray nozzle at 276–310 kPa. Inoculation treatments were protected with sulphur applications until 2 weeks before their inoculation date. Non-inoculated control plots provided an estimate of the incidence and severity of natural infection. Foliar disease severity in each plot was rated on four randomly selected plants in the middle row using the Horsfall–Barratt scale. Ratings were made at the time of inoculation and 7, 14, 21, 32 and 39 days after the first inoculation in 1997, and 7, 14, 22, 29, 37 and 43 days after the first inoculation in 1998. Yield of marketable fruit (red and green) was determined from two plants randomly sampled from the middle row of each plot on 8 and 15 September 1997 and 1 September 1998. In 1997, for each plot, two additional plants were cut at the base, the fruit separated, and both fruit and foliage dried at 40°C for 2 days. The dry weight served as a covariate for yield in each plot.
Growth chamber studies – disease development
Powdery mildew development was assessed on ‘Trust’ tomato plants grown in a Conviron (Controlled Environment Ltd., Winnipeg, MB) chamber set at 10, 15, 20, 25 or 30°C. Leaf wetness periods for each temperature were 4, 8, 12, 16 and 24 h. A 14-h photoperiod at 269 µE s−1 m−2 (PAR-photosynthetically active radiation) was maintained throughout the experiment using cool white VHO fluorescent tubes (Sylvania Co.) and incandescent bulbs. A relative humidity of 75% was maintained in the growth chamber following the leaf wetness period. The plants were pre-conditioned at the test temperature for 24 h prior to inoculation, and were inoculated at the 5- to 7-leaf stage (usually at 42 days after planting) using a hand-sprayer (Model 502 DeVilbiss, BlowTherm USA, Barrie, ON) at 138 kPa. Inoculum was prepared as described for the field studies above. For each leaf wetness period, four plants were used and plants sprayed with water served as a control for any pre-existing infection by the fungus. All plants were incubated in plastic bags for the leaf wetness duration period, and following this, they were placed in a randomized complete block arrangement in the growth chamber. Plants were fertilized with 20–20–20 (N-P-K) every week. While the plants were in the plastic bags, the temperature within was monitored using a thermocouple connected to a data-logger, as described earlier. The temperatures in the plastic bags were 17, 22, 26, 30 and 35°C for the respective growth chamber temperatures. Disease assessment was determined every 4–6 days for 3–4 weeks using the Horsfall–Barratt scale. The experiment was repeated once at each temperature for each leaf wetness period.
Disease management under greenhouse conditions
‘Trust’ tomato plants were grown in rock wool blocks (7.6 × 7.6 × 6.5 cm, L × W × H) in the greenhouse and fertilized as per nutrient film technique regime (Anonymous Citation1988). Plants were at the 5–6 node stage (about 7–8 weeks after seeding) when treatment applications were made. Test materials and rates used were based on manufacturer’s suggested rates (see below). Amounts were calculated using a tank mix formulation based on 1000 L of spray per hectare, and assuming 25 000 plants ha−1 for greenhouse tomato. The test materials with the formulations, product names and manufacturers were as follows: acibenzolar-S-methyl (Actigard®) and trifloxystrobin (Flint®) both at 50%, WG (Syngenta Crop Protection Inc., Greensboro, NC) 0.1 and 0.14 kg ha−1, respectively; copper octanoate soap (10%, Neu 1140 concentrate; Eco-Care Technologies Inc., Sidney, BC) 1:10 dilution of product; potassium bicarbonate (82%, SP, Kaligreen®; Monterey Chemical Co., Fresno, CA) 3.4 kg ha−1; myclobutanil (40%, W, Nova®; Dow AgroSciences Canada Inc., Calgary, AB) 0.34 kg ha−1; azoxystrobin (22.9%, SC, Quadris®; Syngenta Crop Protection Inc.) 0.044 kg ha−1; phenoxyquinoline (250 g L−1, SC, Quinoxyfen; Dow AgroSciences Canada, Inc., Calgary, AB) 0.18 kg ha−1; silica, fumed (Cabot Corp., Champaign, IL) 0.5 g plant−1; paraffinic oil (97.1%, oil, JMS-Stylet Oil®; JMS Flower Farms, Inc. Vero Beach, FL) rates – see below; and sulphur (92% Micro-Fine; United Agri Products, Dorchester, ON) 0.815 kg ha−1. Biological products evaluated were: Bacillus subtilis (Ehrenberg) Cohen (bacterial suspension, QRD 713.5AS; Serenade®; AgraQuest Inc., Parma, ID, 18.7 L ha−1); and Pseudozyma flocculosa (Traquair, L.A. Shaw & Jarvis) Boekhout & Traquair (powder, Sporodex®; Plant Products Co. Ltd., Brampton, ON, 10 kg ha−1). Each material was applied to five plants using a DeVilbiss Model 502 sprayer at 138 kPa. In separate trials, fumed silica was applied as a dust using a garden hand-duster bulb, and JMS Stylet Oil® was sprayed using a Rittenhouse Greenhouse sprayer (St. Catharines, ON) fitted with Albuz Lilac ATR hollow cone nozzles at 2068 kPa (McGrath & Shishkoff 2000) applied as per manufacturer’s recommendations. Rates of JMS Stylet Oil® were 12.5, 25, 50, 75, 125, 250 and 500 mL per 20 L water. One to three applications at 1–2 week intervals were evaluated. For all trials, plants sprayed with water served as the control check. Care was taken to treat both the adaxial and abaxial surface of the leaves, as well as the growing tip of the plant. After application of test materials, the plants were allowed to dry overnight in the greenhouse. After 24 h, a suspension of powdery mildew spores, prepared as described in the field trials study, was sprayed onto the plants (except for the untreated plants) using a DeVilbiss Model 502 sprayer at 138 kPa. The inoculated plants were placed in a mist chamber at 22°C for 24 h to ensure uniform infection. Subsequently, the plants were moved from the mist chamber to the greenhouse where they were placed on rock wool slabs in a completely randomized arrangement within the greenhouse, and plants were maintained under normal greenhouse conditions. Observations were made for disease development using the Horsfall–Barratt scale, and for symptoms of phytotoxicity at the time of inoculation and after 17 days, and again 14 days later. Since it was difficult to evaluate all materials in one trial, the testing was conducted over time in several trials ( & – 2000 to 2002; – 2008). There was a water control in each trial. All tests were repeated at least once.
Table 1. Mean development of Oidium neolycopersici in greenhouse-grown ‘Trust’ tomato at GPCRC-AAFC as determined by disease progress rates, intercepts and adjusted coefficients of determination (r2). Values were derived by fitting data obtained using the Horsfall–Barratt scale to two growth models, and by calculating mean final per cent foliar disease severity and mean area under the disease progress curve (AUDPC).
Table 2. Extent of development of Oidium neolycopersici in the top, middle and bottom third sections of greenhouse-grown ‘Trust’ tomato plants at GPCRC-AAFC as determined by disease progress rates, intercepts and adjusted coefficients of determination (r2). Values were derived by fitting data obtained using the Horsfall–Barratt scale to two growth models, and by calculating mean final per cent foliar disease severity and area under the disease progress curve (AUDPC).
Table 3. Disease progress rates, intercepts and adjusted coefficients of determination (r2) for powdery mildew of tomato. Values were derived by fitting data obtained using the Horsfall–Barratt scale to two growth models, and by calculating mean final per cent foliar disease severity, area under the disease progress curve (AUDPC), and yield of ‘Heinz 2478' tomato due to 12 epidemics in the field at GPCRC-AAFC in 1997–98.
Table 4. Effect of temperature and period of leaf wetness on development of Oidium neolycopersici on ‘Trust’ tomato in the growth chamber as determined by foliar disease progress rates using two growth models, area under the disease progress curve (AUDPC) and final foliar disease per cent.
Table 5. Efficacy of various products for control in the greenhouse of powdery mildew (Oidium neolycopersici) on tomato at GPCRC-AAFC.
Table 6. Efficacy of JMS-Stylet Oil® applied at various rates, and number of applications for control of powdery mildew (Oidium neolycopersici) on greenhouse tomato at GPCRC-AAFC in 2002–2003.
Table 7. Efficacy of post-infection application of various products for control of powdery mildew incited by Oidium neolycopersici in greenhouse ‘Trust’ tomato at GPCRC-AAFC.
Effect of cold temperature duration on pathogen survival
Two leaves were randomly selected from ‘Trust’ tomato plants grown in a greenhouse where extensive development of O. neolycopersici was present. For each treatment, three replicates were used. The leaves were kept in a plastic bag at temperatures of −10°, 4° or 10°C for 1, 2, 4 or 8 days. The two leaves from each replicate were placed in a sterile mortar, 20 mL of sterile distilled water was added, and the leaves were crushed with a mortar. Each suspension was filtered through four layers of cheesecloth and was applied with an air brush (Paasche Airbrush Co., IL) at 138 kPa onto one 6-week-old ‘Bonny Best’ tomato plant grown in a 10-cm-diameter clay pot in a soil mix (Fox sandy loam: peat moss, 3:2 v:v) under disease-free conditions. The plants were checked carefully for absence of symptoms before use. The pots were maintained in a growth room at 24°C, under ambient relative humidity and 16-h photoperiod (provided by Gro-Lux® fluorescent tubes (Sylvania Co.) and incandescent bulbs at 220 µE s−1 m−2 (PAR)). The plants were rated 12–13 days after inoculation using a 0 to 5 scale as follows: 0 = no disease, tr = trace (1–2 colonies/plant), 1 = some colonies on the lower third of the plant (>1 colony leaf−1), 2 = more than 10 colonies leaf−1 on lower third of the plant, 3 = lower third of the plant (>10 colonies leaf−1) to middle third of the plant (>1 colony leaf−1) of plant affected, 4 = both lower and middle third of the plant with >10 colonies leaf−1, 5 = entire plant with colonies. The experiment was repeated once.
Host range studies
All plants used in host range studies are listed in and were grown in 10-cm-diameter clay pots in a soil mix (Fox sandy loam:peat moss, 3:2, v:v), with one plant per pot, and maintained in a greenhouse free of powdery mildew prior to inoculation. A spore suspension was prepared as described in the field trials study using diseased tomato leaves from another greenhouse. Five-week-old randomly selected plants, 5 plants per treatment, were inoculated as described previously and maintained in a greenhouse supplemented with high pressure sodium lighting to extend the photoperiod to 16 h. Two or three randomly selected plants of each host species were sprayed with water and served as the control treatment. If any control plants showed powdery mildew colonies during the course of the experiment, the results were discarded and the experiment repeated. After 15 days, symptoms were rated using the 0 to 5 scale described previously. Tomato ‘Grace’ also included in the experiment was examined again for symptoms after 8 weeks. The experiment was repeated once. Both trials yielded similar results, so data were pooled for analysis.
Table 8. Effect of days of exposure to cold temperatures on survival of Oidium neolycopersici as determined by subsequent disease development of the fungus test sample on tomato.
Data analysis – foliar disease severity
The final disease severity values in the greenhouse disease development experiments were square-root transformed, since all final disease ratings were greater than 80% (Steel & Torrie Citation1960). For disease development studies in greenhouse, field, growth chamber, and disease management under greenhouse conditions, the area under the disease progress curve (AUDPC) was calculated by plotting the converted Horsfall–Barratt disease severity ratings over time and calculating area under the curve for each plant as described by Shaner & Finney (Citation1977). AUDPC was also calculated for each plant portion in the greenhouse disease development experiments by dividing each plant into top, middle and bottom-third portions. The mean disease rating per row in the greenhouse was also calculated. AUDPC and final per cent disease severity values for all trials were analysed using the GLM procedure of the Statistical Analysis System (SAS Institute Inc. Citation1989) and Fisher’s least significant difference (FLSD) (Steel & Torrie Citation1960). Bartlett’s test for homogeneity of variance (Steel & Torrie Citation1960) was used to determine if individual experiments could be combined for the greenhouse, field and growth chamber studies.
The logit and gompit transformations of disease severity in greenhouse, field and growth chamber studies were used, in which x = disease proportion in the range 0 < x < 1, and logit (x) = ln(x/(1−x)) (Vanderplank Citation1963), and gompit (x) = −ln(−ln(x)) (Berger Citation1981). A value of 0.001 was substituted for a disease severity value of 0, and 0.999 for a disease severity value of 1.00. Infection rates for each disease progress curve for 1997 and 1998, and for the combined growth chamber studies, were calculated by regressing logit and gompit transformed proportions of disease against time. The suitability of the transformations in linearizing the powdery mildew disease curves was tested based on adjusted coefficient of determination (r2) values, the F-test, and the residual distribution about the regression line.
Data analysis – yield
Covariance analysis was used with the 1997 yield data of the field studies–disease development experiment to adjust for effects on yield of non-random variation in soil compaction in some plots. Least-squares means (LSM) were used in the covariance analysis to obtain the set of estimates of the adjusted treatment (i.e. yield) means as follows: Yi.(adj.) = Yi. – gw(Xi. – X..) where Yi.(adj.) = the adjusted treatment mean, Yi. = unadjusted treatment mean, gw = average within-treatment slope, xi. = dry weight of the treatment sample, and X.. = the overall mean dry weight for all treatment samples. Mean separation techniques such as Fisher’s least significant difference cannot be readily applied to adjusted treatment means (LSM) since each mean has a different standard error (Freund & Littell Citation1981). Instead a maximum and an average standard error calculated by using the standard errors given by SAS for the least squares means were used. The 1998 yield data were analysed using the GLM procedure of the Statistical Analysis System (SAS Institute Inc. Citation1989) and Fisher’s least significant difference (FLSD) (Steel & Torrie Citation1960).
Results
Greenhouse studies – disease development
The mean temperatures for the top, middle and lower plant sections for the first experiment were 22° (range 19–27.5°), 20° (range 17–27°), and 21°C (range 14–27.5°), while for the second experiment, they were 23° (range 19.5–33°), 20° (range 17.5–28°) and 22°C (range 18.5–31.5°), respectively. Corresponding top, middle and lower plant section’s mean relative humidity readings for the first experiment were 80% (range 60–99%), 89% (range 72–99.5%) and 97% (range 78–100%), and for the second experiment, 88% (range 70–100%), 89% (range 73–100%) and 91% (range 75–100%). The highest temperatures and lowest relative humidity were consistently at the top portion of the plant, and coincided with the least development of powdery mildew. The lower temperature and higher relative humidity readings, at the bottom portion of the plant, corresponded with the earliest and greatest powdery mildew development.
Powdery mildew colonies appeared 8–10 days after inoculation and disease began to increase rapidly about 22 days after inoculation in both experiments (), corresponding to when plants had 29 stem nodes for experiment 1, and 20 in the second experiment. This is evident in the change in slope of the disease progress curves (). The high and significant (P = 0.01, ) disease progress rates for the logistic model of 0.28 for the first experiment and 0.26 for the second, and a corresponding value for the Gompertz model of 0.09 for experiment one and 0.07 for experiment two, indicate rapid disease development. As well, the adjusted coefficients of determination (r2) of 0.93 and 0.87 for the logistic model for experiments one and two, and 0.91 and 0.98 for the Gompertz model for the two experiments, were highly significant ().
Fig. 1 Development of powdery mildew of tomato in the greenhouse at AAFC-GPCRC in 2001–2002. The per cent disease severity represents the mean values per experiment based on 8 rows with 3 plants rated in each row in the greenhouse. Disease severity ratings were determined using the Horsfall–Barratt scheme. Ratings were converted with the Elanco conversion tables to obtain per cent values for the mid-range of each disease interval. Number of plant nodes for corresponding rating periods are: nd, nd, 28, 29, 31, 34, 35, nd, and nd, 14, 17, 20, 24, 28, 31 for experiments 1 (denoted by ♦) and 2 (denoted by ★), respectively, where nd = not determined.
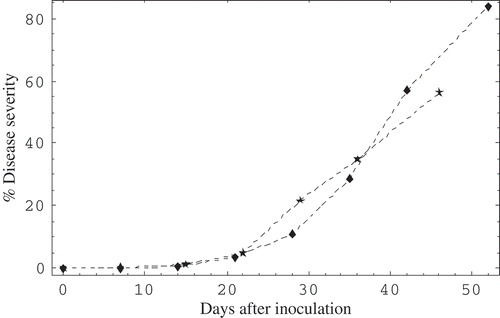
There was no difference in final disease severity among the rows in the greenhouse, except for the row nearest the outside greenhouse wall, which had significantly more disease, although all disease progress rates were similar with best fit of data generally obtained using the logistic model (data not shown). There was a significant (P = 0.05) difference in AUDPC and final disease severity among the top, middle and bottom plant portions (). Differences between bottom- and middle-, and bottom- and top-third of the plants were usually significant. Greatest disease development usually occurred in the bottom-third (0.7 m) of the plant ().
Field studies – disease development and yield
The logistic model with higher adjusted coefficients of determination described disease progress more accurately than the Gompertz model for the field data (). All rates and coefficients of determination were significant at P ≤ 0.0001. The highest disease progress rates and greatest final disease severity and AUDPC were at earliest times of inoculation for both years. Rate of powdery mildew development as well as AUDPC and final foliar disease severity was greater in 1997 than in 1998 at the earliest time of inoculation (). All intercept values with the logistic and Gompertz models were similar. Differences were only 0.96 for the logistic and 0.36 for the Gompertz model in 1997, while in 1998 these were 0.66 for the logistic and 0.20 for the Gompertz model. Fungicide-treated plants showed significantly lower disease severity than untreated plants exposed to inoculum early in the season in 1998 but not in 1997. There were no significant differences in yield among the treatments in either year (). Other foliar diseases, such as septoria blight (Septoria lycopersici Speg.) and alternaria leaf spots (Alternaria solani (Ell. & Mart.) L.R. Jones & Grout, A. alternata (Fr.:Fr.) Keissl. f. sp. lycopersici Grogan et al.) were low in incidence and severity and they were not observed in the field plots until late in the season.
Growth chamber studies – disease development
The Gompertz model with higher adjusted r2 described disease progress better than the logistic model (). AUDPC, final foliar disease percentage and rate of disease progress were all greatest at 22°C (temperature inside plastic bag; 15°C in growth chamber outside the plastic bag) and least at 30°C. At 35°C (data not shown), there was trace sporulation with less than one colony per plant and chlorosis was evident on the lower foliage; at 26°C, there was an intermediate level of disease. There was no effect of leaf wetness duration period on AUDPC or final disease severity at 17, 26 and 30°C; however, at 22°C, the greatest AUDPC and final disease severity occurred after 16 and 24 h of leaf wetness duration ().
Disease management under greenhouse conditions
Significant control of the pathogen, in order of increasing efficacy for both disease parameters, was achieved with Quadris (azoxystrobin), Actigard (acibenzolar-S-methyl), Quinoxyfen (phenoxyquinoline), sulphur and Flint (trifloxystrobin) in experiment two and with Flint and Actigard for AUDPC in experiment one (). There was considerable phytotoxicity consisting of foliar chlorosis, some necrosis and plant stunting associated with the rate of Quinoxyfen (180 g product ha−1) that was used. B. subtilis (Serenade®) significantly reduced final powdery mildew severity in the second experiment only but not the AUDPC in either experiment in comparison to the control treatment. Potassium bicarbonate (Kaligreen®) and P. flocculosa (Sporodex®) were not effective in reducing powdery mildew (, Study A). Actigard at 50 g a.i. ha−1 caused phytotoxicity symptoms, consisting of some foliar chlorosis and/or necrosis, mottling, misshapen leaves, curling of leaf margins and plant stunting. Cu-soap (copper octanoate) and fumed silica did not control powdery mildew in the greenhouse in either experiment (, Study B), whereas JMS-Stylet Oil® was effective at all rates tested (, Study A–C). The highest rates of JMS-Stylet Oil® reduced final disease severity more than lowest rates, whereas rate effects were not evident in the AUDPC (, Study C). Rates of 25–75 mL JMS-Stylet Oil® per 20 L water were effective for disease control. There were no significant differences among these treatments (, Study A). Multiple applications of JMS-Stylet Oil® at 12.5 mL per 20 L water or 25 mL per 20 L water reduced disease severity and were similarly effective (, Study B). Ten days after Stylet Oil application, there was some foliar phytotoxicity with marginal burn, restricted growth and cupping of foliage at rates of 375 and 500 mLper 20 L water with phytotoxicity ratings of 0.17 and 0.2, respectively, while lower levels of foliar phytotoxicity at 0.07 were observed at 125 mL per 20 L water on 50% of plants sprayed.
In experiments to determine the efficacy of post-infection application of treatments, powdery mildew severity at first rating varied from 1.5% (Flint, Quadris) to 9.1% (Cu-soap) in trial 1, and from 1.9% (check-inoculated, water) to 7.4% (Cu-soap) in trial 2 (). The proportion of foliage covered by powdery mildew declined, 21 and 27 days after the first disease rating in trials 1 and 2, respectively, indicating some degree of control, as demonstrated by negative difference values (, B–A) for most treatments. Greatest powdery mildew control, as demonstrated by negative difference values and smallest AUDPC, occurred with Stylet Oil, Serenade, Actigard, Flint, Kaligreen and Quadris, with no significant differences among these treatments in both trials except for Actigard in trial 2 ().
Effect of cold temperature duration on pathogen survival
The fungus survived all temperature and duration intervals tested as determined by disease development on all inoculated tomato plants ().
Host range studies
Extensive development of powdery mildew occurred on the susceptible tomato ‘Trust’, but not on the resistant ‘Grace’ () even when the latter was maintained in the greenhouse adjacent to severely affected ‘Trust’ plants for 8 weeks. Occasional small flecks, less than 1 mm in diameter, were visible on the foliage. No symptoms were visible on pepper ‘Early California Wonder’, Indian yellow-berried nightshade, smooth or red root pigweed, velvetleaf, spreading atriplex, Queen Anne’s lace, peppergrass, smooth groundcherry, sulphur cinquefoil, wild buckwheat, chickweed or potato 15 days after inoculation (). One small colony was observed on ‘Corona’ cucumber 7 days after inoculation. A few colonies with very sparse sporulation occurred on the adaxial surface of lower leaves of eggplant (‘Black Beauty’ and ‘Long Tom’), hairy nightshade and Indian yellow-berried nightshade. The tobacco lines had greater numbers of small mildew colonies on the lower leaves, often accompanied by indistinct chlorotic margins (). Sporulation was greatest on the lower leaves of jimsonweed, American and black nightshade, with a few colonies evident in the mid-sections of these plants ().
Table 9. Host range of different plant species tested against Ontario isolates of Oidium neolycopersici, 15 days after inoculation.
Discussion
Greenhouse environmental conditions are more likely to be favourable for tomato powdery mildew development than conditions in the field in Ontario, where greater fluctuations in temperature, relative humidity (RH) and leaf wetness generally occur. However, damage by powdery mildew infection on field-grown tomatoes has been correlated with the occurrence of high RH in late-season crops (Mieslerová & Lebeda Citation1999). The higher rates of disease progress noted in the greenhouse studies support this observation, with more severe symptoms on the older, lower foliage where the air temperature is somewhat lower and RH greater under shade. This agrees with the observations of Jacob et al. (Citation2008) and Chelal & Hau (Citation2015). A similar relationship was observed between RH and temperature and location with respect to plant canopy for powdery mildew development on greenhouse cucumber in Ontario (Zhang et al. Citation2002). Control measures applied early in powdery mildew development and involving complete spray coverage in lower plant portions could be beneficial in limiting development of this disease on greenhouse tomato.
The growth chamber results indicate an optimal temperature of 22°C for disease development. This is in close agreement with Abiko (Citation1978), who reported an optimum of 23°C with RH varying from 45 to 100%, while Mieslerová & Lebeda (Citation2010) and Jacob et al. (Citation2008) reported 25°C to be the optimum temperature for O. neolycopersici development. In greenhouse studies, Whipps & Budge (Citation2000) observed greatest disease development at 80% RH and progressively less disease with increasing RH to 95%. In that study, however, spore germination occurred between 65% and 100% RH, with an optimum at 95% RH or greater. In our experiments, specific leaf wetness periods were maintained by covering plants with plastic bags and temperature was maintained in the growth chambers, although temperatures during the leaf wetness periods were higher due to the plastic bags. In contrast, in greenhouse studies, the temperature may not always be controlled and thus the vapour pressure deficit and leaf wetness can vary widely. Such observations may partly explain differences in the influence of RH and leaf wetness on disease development in this study, and as noted by Jacob et al. (Citation2008). Mieslerová & Lebeda (Citation2010) and Jacob et al. (Citation2008) noted that tomato powdery mildew development was negatively correlated with temperatures in the low (5–15°C) and high range (35–40°C) similar to some of the growth chamber observations in this study, while Elad et al. (Citation2009) reported the same results as described in this study but where RH levels of 40% and below, or 90–100% RH occurred.
Jacob et al. (Citation2008) and Chelal & Hau (Citation2015) reported that tomato powdery mildew was first noticed on the lower leaves at 10 and 11 days after inoculation (DAI) in their respective greenhouse studies, similar to observations in the present study; however, in this study, disease development became rapid at 22 DAI. Jacob et al. (Citation2008) noted 60% and 55% disease severity in one and two greenhouses 44 DAI while in the present study a similar disease severity level, under different environmental conditions was noted 42 and 46 DAI, respectively, in two experiments ().
The Gompertz model described disease progress more accurately in greenhouse and growth chamber studies than the logistic model while the latter was more accurate in field studies and in greenhouse studies where plant sections were rated. Such differences between epidemic progress in greenhouse compartments and large fields (Plaut & Berger Citation1981) or even between cultivars (Campbell et al. Citation1984) have been noted.
In our field experiments, no yield loss was observed at 42% final foliar disease severity at harvest. Dik (Citation1999) also reported no loss in greenhouse tomato production where 20% of leaf area was affected by powdery mildew. In the present study, the earliest inoculations were done 52 days after transplanting in 1997 and 47 days after transplanting in 1998. However, symptomless infected tomato plants or those with one or two colonies per plant, if transplanted into the field soon after infection in the greenhouse by O. neolycopersici, could develop considerably more disease than in the present study. The subsequent foliar damage results in the defoliation of diseased leaves (Chelal & Hau Citation2015) and would necessitate fungicide applications. This occurred in the nearby processing and experimental tomato lines in 1996–1998 at GPCRC, Harrow, ON where transplants with trace levels of powdery mildew symptoms were set into the field (author, unpublished observations).
Strobilurin fungicides, such as Quadris and Flint, were effective in controlling powdery mildew on greenhouse tomato in the present study. In other preliminary trials, another strobilurin fungicide, Sovran® (kresoxim-methyl, BASF Canada Inc.) was also very effective at 40.6 g of product per 100 L of water (R. Cerkauskas, unpublished) in controlling tomato powdery mildew in the greenhouse. Similarly, pyraclostrobin, another strobilurin fungicide, was effective in controlling powdery mildew under low disease pressure on field tomato (Shishkoff & McGrath Citation2002). However, resistance to several strobilurins has been observed in the cucumber powdery mildew pathogen in greenhouses in Japan (Ishii et al. Citation2001), and partial cross-sensitivity between the strobilurins and demethylation-inhibiting fungicides, such as Nova (myclobutanil) (Wong & Wilcox Citation2002), has been reported in grapevine powdery mildew caused by Uncinula necator (Schwein.) Burrill. The extensive use of Nova for control of powdery mildew on tomato in greenhouses in Ontario suggests there is potential for very rapid loss of efficacy of the strobilurins for control of this disease. Resistance in pepper powdery mildew to Nova and resistance in cucumber powdery mildew to Nova and Pristine has already been reported to be a problem in greenhouse vegetable production in Ontario (Cerkauskas et al. Citation2011; Cerkauskas & Ferguson Citation2014).
Quinoxyfen, belonging to a new class of fungicides (phenoxyquinolines), was effective in controlling tomato powdery mildew in the current study and in other greenhouse trials (Baysal-Gurel & Miller Citation2015) and in trials to control cucumber powdery mildew (Cerkauskas & Ferguson Citation2014) and pepper powdery mildew (Cerkauskas et al. Citation2011). Quinoxyfen previously showed specific activity against powdery mildews and provided exceptional and reliable control of disease, irrespective of disease pressure and environmental conditions (Green et al. Citation1998). Extensive symptoms of phytotoxicity occurred in our trials, consisting of foliar chlorosis with some necrosis on the lower half of the plant, at a rate of 180 g ha−1. This was accompanied by stunting and occasional wilting of the plants. Baysal-Gurel & Miller (Citation2015) also reported phytotoxicity at a higher rate. No decrease in sensitivity to this fungicide was observed in powdery mildew of wheat in a 5-year resistance-monitoring program conducted in Europe (Bernhard et al. Citation2002). Quinoxyfen has no known cross-resistance to current fungicides and could be valuable as a resistance management tool at lower rates to avoid phytotoxicity on greenhouse tomato.
Actigard, a plant resistance activator in tomato (Cohen et al. Citation1994; Benhamou & Bélanger Citation1998; Louws et al. Citation2001), provided effective control at 50 g a.i. ha−1, but was phytotoxic. Similarly, Louws et al. (Citation2001) observed reduced growth of tomato in the greenhouse at 35 g a.i. ha−1. More studies at different rates and timing of application need to be done.
Sporodex (Pseudozyma flocculosa) was previously registered for control of powdery mildew on greenhouse cucumber under conditions of high relative humidity, moderate temperature, and cloud cover (Jarvis et al. Citation1989). However, in our studies, the product was not very effective for control of O. neolycopersici under less environmentally favourable conditions, in contrast to the results presented by Jones et al. (Citation2001). Requirements for specific environmental conditions at the time of application and during the subsequent overnight period (Jarvis et al. Citation1989) can limit the efficacy and ease of application of Sporodex.
Another biological control organism, B. subtilis (Serenade), when applied as a protectant, was not effective in control of O. neolycopersici in the present study, similar to a report by Baysal-Gurel & Miller (Citation2015). This result contrasts with a report by Bettiol et al. (Citation1997) in which a wettable powder formula of B. subtilis was used for control of powdery mildew of cucumber. Other rates, different strains of the organism and a shorter interval between spray application and inoculation need to be tested for control of O. neolycopersici.
Kaligreen (potassium bicarbonate) applied as a protectant was ineffective in controlling powdery mildew on tomato in this study and it was less effective than conventional fungicides in control of cucumber and pepper powdery mildews (Cerkauskas et al. Citation2011; Cerkauskas & Ferguson Citation2014) while Koller (Citation2011) observed efficacy in control of tomato powdery mildew that was comparable to sulphur. Similarly, Smith et al. (Citation2002) and Ferrandino et al. (Citation2002a, Citation2002b) reported that baking soda treatments were consistently less effective than milk for control of powdery mildew on tomato and powdery mildew on muskmelon and pumpkin (both incited by S. fuliginea), and that lactose without surfactant was effective (Smith et al. Citation2003). Cerkauskas et al. (2014) reported enhanced efficacy using Lactosan, a fermented dairy product, with a surfactant to control cucumber powdery mildew but not tomato powdery mildew (R. Cerkauskas, unpublished). Ziv & Zitter (Citation1992) reported that potassium and sodium bicarbonate at 0.5% and 1% (w/v) were not effective as preventative treatments for powdery mildew on cucumber, although curative properties by direct contact with the fungus were observed, while Koller (Citation2011) observed reduced efficacy with post-infection applications. Bicarbonate-plus-oil treatments were more effective when applied prior to inoculation on cucumber. In contrast, Ko et al. (Citation2003) found sodium bicarbonate to be less effective than sunflower oil for control of powdery mildew of tomato. Ehret et al. (Citation2002), using foliar applications of fertilizer salts such as CaCl2, Ca(NO3)2 and K2HPO4, reported reductions in powdery mildew of greenhouse tomato.
Soluble silicon applied as a foliar spray of potassium silicate (1000 ppm) or as an amendment in hydroponic culture (100 ppm) has been reported to be effective in control of powdery mildew of cucumber (Menzies et al. Citation1992; Bélanger et al. Citation1995), but inconclusive results were obtained in control of powdery mildew of greenhouse tomato (Bélanger et al. Citation1995). Garibaldi et al. (Citation2011) demonstrated that inclusion of potassium silicate (100 ppm) in the drip irrigation system significantly reduced tomato powdery mildew severity at final evaluation from 47 to 3%, and from 35 to 1% when delivered via the sub-irrigation in one trial. In a second trial, disease was reduced from 46 to 2% when delivered in the drip irrigation system, and from 48 to 11% in the sub-irrigation system. In both trials, the addition of NaCl at 0.87 g L−1 significantly reduced powdery mildew incidence and severity in all the assessments (Garibaldi et al. Citation2011). A foliar application of potassium silicate at 1 g L−1 was as effective in control of another powdery mildew, Leveillula taurica (Lév.) G. Arnaud, on field tomato as fungicides sulphur + triadimenol (Yanar et al. Citation2011). In our studies, silicon was ineffective when a non-soluble product, fumed silica, was used as a protective dust on the foliage.
In this study JMS Stylet-Oil®, a food-grade paraffinic oil, provided effective control of tomato powdery mildew, similar to other observations for control of pepper and cucumber powdery mildew in the greenhouse (Cerkauskas et al. Citation2011; Cerkauskas & Ferguson Citation2014). In contrast, Baysal-Gurel & Miller (Citation2015) reported reduced severity of powdery mildew at 39 and 62% in two experiments but with significant phytotoxicity at the much higher rate of 300 mL per 20 L water. Based on our observations, it is possible that JMS Stylet-Oil®could be used effectively at low rates within an IPM program in greenhouse vegetable production. Northover & Schneider (Citation1996) demonstrated protective action with pre-inoculation sprays of JMS-Stylet Oil® and observed an ‘antisporulative’ effect in studies with powdery mildew of grape. Smith (Citation1996) reported JMS-Stylet Oil® to be equally effective in control of powdery mildew of bell pepper as conventional fungicides such as sulphur. Ko et al. (Citation2003) observed that sunflower oil was more effective in control of O. neolycopersici on tomato than corn, sesame or peanut oils. In the present study, JMS-Stylet Oil® was effective in control of powdery mildew of tomato whereas potassium bicarbonate was not effective. McGrath & Shishkoff (Citation1999) reported similar observations using these two products for control of cucumber powdery mildew in the field. Their 4-day spray program with oil, beginning 5 days after inoculation, suppressed powdery mildew development, although the oil did not reduce fungal spore viability. As noted in other studies involving cucumber and pepper powdery mildew (Cerkauskas et al. Citation2011; Cerkauskas & Ferguson Citation2014), additional trials involving different rates, spray intervals, better spray coverage on both tomato leaf surfaces and/or the use of conventional fungicides when powdery mildew disease pressure is high, or until disease resistance is incorporated into commercially acceptable cultivars, may be necessary to achieve enhanced efficacy. These alternative materials and novel fungicides (Gullino et al. Citation2000; Ehret et al. Citation2001) need to be incorporated into integrated control programmes for all diseases of greenhouse tomato (Bélanger et al. Citation1998; Bardin et al. Citation2008).
Survival of O. neolycopersici on tomato foliage for 8 days at −10°C was observed in this study while Bardin et al. (Citation2007) reported survival of conidia of some isolates of the fungus in liquid nitrogen (−196°C) after 10 days.
Differential pathogenicity of O. neolycopersici has been demonstrated on some weeds and other economically important Solanaceous plants in the current and previous studies (Fletcher et al. Citation1988; Whipps et al. Citation1998; LaMondia et al. Citation1999; Huang et al. Citation2000; Utkehede et al. Citation2001; Kashimoto et al. Citation2003a). For example, tobacco had very limited powdery mildew development in some studies (Utkehede et al. Citation2001) but not in others (; LaMondia et al. Citation1999; Huang et al. Citation2000; Kashimoto et al. Citation2003a) Eggplant was a host in several cases (Whipps et al. Citation1998; LaMondia et al. Citation1999; Huang et al. Citation2000; Utkehede et al. Citation2001; Kashimoto et al. Citation2003a) but had limited disease development in the present study. Potato was observed as a host in some studies (LaMondia et al. Citation1999; Huang et al. Citation2000; Kashimoto et al. Citation2003a) but not in this study or by Utkehede et al. (Citation2001). Whipps et al. (Citation1998) observed variation in the degree of infection and symptom development among 45 potato cultivars that were tested. With differential pathogenicity observed in O. neolycopersici isolates from different parts of Canada (Cerkauskas – Ontario; Utkhede – British Columbia), control of the several potential alternative hosts located near greenhouse tomato production in different parts of Canada may be important.
Lebeda & Mieslerová (Citation2002) reported that an English isolate of O. neolycopersici exhibited considerable pathogenicity on Lycopersicon species compared with isolates from the Czech Republic, Germany and the Netherlands. The existence of pathotypes (formae speciales) of O. neolycopersici has been suggested, based principally on infection differences on an extensive range of cucurbitaceous and solanaceous hosts (Mieslerová & Lebeda Citation1999). LaMondia et al. (Citation1999) reported that most commercial tomato field cultivars were severely affected by powdery mildew. During the summer of 1997, natural epidemics of powdery mildew were observed in some of the fresh market and interspecific tomato breeding plots at Harrow, ON (Cerkauskas, unpublished). Utkehede et al. (Citation2001) in tests with 26 tomato cultivars found only ‘DRW4409F1' (from De Ruiter Seeds Inc.) to be resistant while He et al. (Citation2010), using Ontario isolates of O. neolycopersici, showed that resistance in this cultivar was controlled by a single dominant gene. ‘Grace’ was resistant under greenhouse conditions in the present study, and under field conditions, as observed by Smith & LaMondia (Citation2001), whereas Kashimoto et al. (Citation2003b) reported that ‘Grace’ was severely infected by a common Japanese isolate, KTP-01. Lebeda et al. (Citation2014) described the interaction between wild Solanum spp., tomato and the powdery mildew fungus as race-specific and governed by different race-specific genes.
In our host range tests, there was very limited development of O. neolycopersici on cucumber. The possible existence of, or the selection for, virulent pathotypes of O. neolycopersici, particularly those capable of infecting both greenhouse tomatoes and cucumbers, is a remote possibility. In view of the extensive sporulation of this pathogen on cucumber reported by Whipps et al. (Citation1998), and the relatively confined 9 km radius of Leamington, ON where production of both greenhouse crops occurs, there could be inoculum that is rapidly and easily dispersed between neighbouring greenhouses or infected alternate hosts outside the greenhouses. In related studies, Leveillula taurica, a powdery mildew affecting greenhouse pepper in Ontario, was observed to infect greenhouse tomato (Cerkauskas et al. Citation2011).
There is also potential for the spread of powdery mildew inoculum from greenhouse tomato to the field and back to the greenhouse in Ontario. Environmental factors and dispersal of inoculum from adjacent greenhouse tomato crops in Leamington, ON and from transplants, which are initially symptomless, may play a key role in establishment of powdery mildew in commercial fields of processing tomato and on solanaceous weeds in southwestern Ontario. An integrated approach to control this disease in Ontario is needed.
Acknowledgements
The support of the Ontario Greenhouse Vegetable Producers Marketing Board and the financial assistance of the Matching Investment Initiative Program of Agriculture and Agri-Food Canada (Project No. 2158), and the Ontario Ministry of Agriculture, Food and Rural Affairs (OMAFRA) Food Systems 2002 - Pest Management Program (Project SR9059) is gratefully acknowledged. Appreciation is gratefully expressed to S. Weaver, GPCRC, for providing weed seeds and T. Welacky for tobacco seeds. The assistance of K. Vollans, M. Ferrar, J. Zheng and L. Leopold is gratefully acknowledged.
Additional information
Funding
References
- Abiko K. 1978. Influence of temperature and humidity on development of tomato powdery mildew. Proc Kansai Plant Prot Soc. 20:49–52.
- Anonymous. 1988. Growing greenhouse vegetables. Publication 526, Ontario Ministry of Agriculture, Food & Rural Affairs. Toronto (ON): Queen’s Printer for Ontario.
- Anonymous. 2006. Vegetable production recommendations 2006-2007. Publication 363, Ontario Ministry of Agriculture, Food & Rural Affairs. Toronto (ON): Queen’s Printer for Ontario.
- Bardin M, Fargues J, Nicot PC. 2008. Compatibility between biopesticides used to control grey mold, powdery mildew and whitefly on tomato. Biol Cont. 46:476–483.
- Bardin M, Suliman ME, Sage-Palloix A-M, Mohamed YF, Nicot PC. 2007. Inoculum production and long-term conservation methods for cucurbits and tomato powdery mildew. Mycol Res. 111:740–747.
- Baysal-Gurel F, Miller SA. 2015. Management of powdery mildew in greenhouse tomato production with biorational products and fungicides. Acta Hort. 1069:179–183.
- Bélanger RR, Bowen PA, Ehret DL, Menzies JG. 1995. Soluble silicon: its role in crop and disease management of greenhouse crops. Plant Dis. 79:329–336.
- Bélanger RR, Dik AJ, Menzies JG. 1998. Powdery mildews - recent advances toward integrated control. In: Boland GJ, Kuykendall LD, editors. Plant - microbe interactions and biological control. New York (NY): Marcel Dekker; p. 89–109.
- Bélanger RR, Jarvis WR. 1994. Occurrence of powdery mildew (Erysiphe sp.) on greenhouse tomatoes in Canada. Plant Dis. 78:640.
- Benhamou N, Bélanger RR. 1998. Benzothiadiazole-mediated induced resistance to Fusarium oxysporum f.sp. radicis-lycopersici in tomato. Plant Physiol. 118:1203–1212.
- Berger RD. 1981. Comparison of the Gompertz and logistic equations to describe plant disease progress. Phytopathology. 71:716–719.
- Bernhard U, Leader A, Longhurst C, Felsenstein FG. 2002. Quinoxyfen-resistance management and sensitivity in wheat: 1995-2000. Pest Manag Sci. 58:972–974.
- Bettiol W, Garibaldi A, Mighel Q. 1997. Bacillus subtilis for the control of powdery mildew on cucumber and zucchini. Bragantia. 56:281–287.
- Campbell CL, Jacobi WR, Powell NT, Main CE. 1984. Analysis of disease progression and the randomness of occurrence of infected plants during tobacco black shank epidemics. Phytopathology. 74:230–235.
- Cerkauskas RF, Brown J, Zheng J. 1999. Tomato powdery mildew development under greenhouse, growth chamber and field conditions. Phytopathology. 89:S12–S13. (abstr.).
- Cerkauskas RF, Ferguson G. 2014. Management of powdery mildew (Podosphaera xanthii) on greenhouse cucumber in Ontario. Can. J Plant Pathol. 36:22–37.
- Cerkauskas RF, Ferguson G, Banik M. 2011. Powdery mildew (Leveillula taurica) on greenhouse and field peppers in Ontario – host range, cultivar response and disease management strategies. Can. J Plant Pathol. 33:485–498.
- Cerkauskas RF, Leopold L, Ferguson G. 2000. Control of powdery mildew in greenhouse cucumbers, peppers, and tomatoes. Phytopathology. 90:S12. (abstr.).
- Chelal J, Hau B. 2015. Modelling the interaction between powdery mildew epidemics and host dynamics of tomato. Eur J Plant Pathol. 142:461–479.
- Cohen Y, Niderman T, Mosinger E, Fluhr R. 1994. Beta-aminobutyric acid induces the accumulation of pathogenesis-related proteins in tomato (Lycopersicon esculentum L.) plants and resistance to late blight infection caused by Phytophthora infestans. Plant Physiol. 104:59–66.
- Dik AJ. 1999. Damage relationships for powdery mildews in greenhouse vegetables. In: Bélanger RR, Bushnell WR, Carver TLW, Dik AJ, Kunoh H, Nicot P, Schmitt A, organizing committee, editors. The first international powdery mildew symposium. Avignon, France; p. 53–54.
- Ehret DL, Koch C, Menzies J, Sholberg P, Garland T. 2001. Foliar sprays of clay reduce the severity of powdery mildew on long English cucumber and wine grapes. HortScience. 36:934–936.
- Ehret DL, Menzies JG, Bogdanoff C, Utkhede RS, Frey B. 2002. Foliar applications of fertilizer salts inhibit powdery mildew of tomato. Can J Plant Pathol. 24:437–444.
- Elad Y, Jacob D, David DR, Burshtein M, Sztjenberg A, Yehezkel H, Ganot L, Shmuel D, Matan E, Messika Y. 2009. Development of climate control methods for integrated management of powdery mildew of tomato caused by Oidium neolycopersici. Acta Hort. 807:727–732.
- Ferrandino FJ, Smith VL, Cecarelli R. 2002a. Evaluation of milk as a foliar spray for control of powdery mildew on muskmelon, 2001. Biol Cult Tests. 17:V12.
- Ferrandino FJ, Smith VL, Cecarelli R. 2002b. Evaluation of milk as a foliar spray for control of powdery mildew on pumpkin, 2001. Biol Cult Tests. 17:V19.
- Fletcher JT, Smewin BJ, Cook RTA. 1988. Tomato powdery mildew. Plant Pathol. 37:594–598.
- Freund RJ, Littell RC. 1981. SAS for linear models - a guide to the ANOVA and GLM procedures. Cary (NC): SAS Institute Inc.
- Garibaldi A, Gilardi G, Gullino ML. 2011. Effect of potassium silicate and electrical conductivity in reducing powdery mildew of hydroponically grown tomato. Phytopathol Mediterr. 50:192–202.
- Green EA, Bernhard U, Bacci L. 1998. Control of powdery mildews with quinoxyfen in horticultural crops. Br Crop Prot Conf-Pests & Dis. 3:857–862.
- Gullino ML, Leroux P, Smith CM. 2000. Uses and challenges of novel compounds for plant disease control. Crop Prot. 19:1–11.
- He C, Poysa V, Yu K, Shi C. 2010. Inheritance of resistance to powdery mildew (Oidium neolycopersici) and its linkage to an SSR marker in tomato hybrid DRW4409. Can J Plant Sci. 90:803–807.
- Horsfall JG, Barratt RW. 1945. An improved grading system for measuring plant diseases. Phytopathology. 35:655. (abstr.).
- Huang -C-C, Biesheuvel J, Lindhout P, Niks RF. 2000. Host range of Oidium lycopersici occurring in the Netherlands. Eur J Plant Pathol. 106:465–473.
- Ishii H, Fraaije BA, Sugiyama T, Noguchi K, Nishimura K, Takeda T, Amano T, Hollomon DW. 2001. Occurrence and molecular characterization of strobilurin resistance in cucumber powdery mildew and downy mildew. Phytopathology. 91:1166–1171.
- Jacob D, Rav David D, Sztjenberg A, Elad Y. 2008. Conditions for development of powdery mildew of tomato caused by Oidium neolycopersici. Phytopathology. 98:270–281.
- Jarvis WR, Shaw LA, Traquair JA. 1989. Factors affecting antagonism of cucumber powdery mildew by Stephanoascus flocculosus and S. rugulosus. Mycol Res. 92:162–165.
- Jones HE, Whipps JM, Gurr SJ. 2001. The tomato powdery mildew fungus Oidium neolycopersici. Mol Plant Pathol. 2:303–309.
- Kashimoto K, Matsuda Y, Matsutani K, Sameshima T, Kakutani K, Nonomura T, Okada K, Kusakari S, Nakata K, Takamatsu S, Toyoda H. 2003a. Morphological and molecular characterization of a Japanese isolate of tomato powdery mildew Oidium neolycopersici and its host range. J Gen Plant Pathol. 69:176–185.
- Kashimoto K, Sameshima T, Matsuda Y, Nonomura T, Oichi W, Kakutani K, Nakata K, Kusakari S, Toyoda H. 2003b. Infectivity of a Japanese isolate of Oidium neolycopersici KTP-01 to a European tomato cultivar resistant to O. lycopersici. J Gen Plant Pathol. 69:406–408.
- Kiss L, Cook RTA, Saenz GS, Cunnington JH, Takamatsu S, Pascoe I, Bardin M, Nicot PC, Sato Y, Rossman AY. 2001. Identification of two powdery mildew fungi, Oidium neolycopersici sp. nov. and O. lycopersici, infecting tomato in different parts of the world. Mycol Res. 105:684–697.
- Kiss L, Takamatsu S, Cunnington JH. 2005. Molecular identification of Oidium neolycopersici as the causal agent of the recent tomato powdery mildew epidemics in North America. Plant Dis. 89:491–496.
- Ko WH, Wang SY, Hsieh TF, Ann PJ. 2003. Effects of sunflower oil on tomato powdery mildew caused by Oidium neolycopersici. J Phytopathol. 151:144–148.
- Koller M. 2011. Potassium bicarbonate as a potential sulphur substitute in protected organic cropping. Acta Hort. 915:157–163.
- Koppert Biological Systems. 2010. Side effects of pesticides on beneficials. [cited 2014 Dec 19]. Available from: http://side-effects.koppert.nl/
- LaMondia JA, Smith VL, Douglas SM. 1999. Host range of Oidium lycopersicum on selected solanaceous species in Connecticut. Plant Dis. 83:341–344.
- Lebeda A, Mieslerová B. 2002. Variability in pathogenicity of Oidium neolycopersici on Lycopersicon species. Z Pflanzenkr Pflanzensch. 109:129–141.
- Lebeda A, Mieslerová B, Petrivalský M, Luhová L, Špundová M, Sedlápová M, Nožková-Hlavácková V, Pink D. 2014. Resistance mechanisms of wild tomato germplasm to infection of Oidium neolycopersici. Eur J Plant Pathol. 138:569–596.
- Louws FJ, Wilson M, Campbell HL, Cuppels DA, Jones JB, Shoemaker PB, Sahin F, Miller SA. 2001. Field control of bacterial spot and bacterial speck of tomato using a plant activator. Plant Dis. 85:481–488.
- McGrath MT, Shishkoff N. 1999. Evaluation of biocompatible products for managing cucurbit powdery mildew. Crop Prot. 18:471–478.
- McGrath MT, Shishkoff N. 2000. Control of cucurbit powdery mildew with JMS Stylet-Oil. Plant Dis. 84:989–993.
- McGrath MT, Staniszewska H, Shishkoff N, Casella G. 1996. Fungicide sensitivity of Sphaerotheca fuliginea populations in the United States. Plant Dis. 80:697–703.
- Menzies J, Bowen P, Ehret D, Glass ADM. 1992. Foliar applications of potassium silicate reduce severity of powdery mildew on cucumber, muskmelon, and zucchini squash. J Am Soc Hort Sci. 11:902–905.
- Mieslerová B, Lebeda A. 1999. Review: taxonomy, distribution and biology of the tomato powdery mildew (Oidium lycopersici). Z Pflanzenkr Pflanzensch. 106:140–157.
- Mieslerová B, Lebeda A. 2010. Influence of temperature and light conditions on germination, growth and conidiation of Oidium neolycopersici. J Phytopathol. 158:616–627.
- Northover J, Schneider KE. 1996. Physical modes of action of petroleum and plant oils on powdery and downy mildews of grapevines. Plant Dis. 80:544–550.
- Park MJ, Jee HJ, Kim JH, Shin HD. 2010. First confirmed report of tomato powdery mildew caused by Oidium neolycopersici in Korea. Plant Pathol. 59:409.
- Pernezny K, Sonoda RM. 1998. Powdery mildew of field-grown tomato in Florida. Plant Dis. 82:262.
- Plaut JL, Berger RD. 1981. Infection rates in three pathosystem epidemics initiated with reduced disease severities. Phytopathology. 71:917–921.
- SAS Institute Inc. 1989. SAS/STAT© user’s guide, version 6 [computer program]. Cary (NC): SAS Institute Inc.
- Shaner G, Finney RE. 1977. The effect of nitrogen fertilization on the expression of slow-mildewing resistance in Knox wheat. Phytopathology. 67:1051–1056.
- Shishkoff N, McGrath MT. 2002. Evaluation of fungicides for powdery mildew in staked tomatoes, 2001. Fungic Nemat Tests. 57:V119.
- Smith R. 1996. Evaluation of materials for control of bell pepper powdery mildew, 1995. Fungic Nemat Tests. 51:120.
- Smith VL, Ferrandino FJ, Cecarelli R. 2002. Evaluation of milk as a foliar spray for control of powdery mildew on tomato, 2001. Biol Cult Tests. 17:PT16.
- Smith VL, Ferrandino FJ, Cecarelli R. 2003. Evaluation of milk and lactose as foliar sprays for control of powdery mildew on tomato, 2002. Biol Cult Tests. 18:PT15.
- Smith VL, LaMondia JA. 2001. Tomato cultivar response to powdery mildew, 2000. Biol Cult Tests. 16:PT78.
- Steel RGD, Torrie JH. 1960. Principles and procedures of statistics. New York (NY): McGraw-Hill.
- Utkehede RS, Koch CA, Menzies JG, Ehret DL. 2001. Host range of a powdery mildew (Erysiphe orontii) on tomato. Can J Plant Sci. 81:179–182.
- Vanderplank JE. 1963. Plant diseases: epidemics and control. New York (NY): Academic Press.
- Whipps JM, Budge SP. 2000. Effect of humidity on development of tomato powdery mildew (Oidium lycopersici) in the glasshouse. Eur J Plant Pathol. 106:395–397.
- Whipps JM, Budge SP, Fenlon JS. 1998. Characteristics and host range of tomato powdery mildew. Plant Pathol. 47:36–48.
- Wong FP, Wilcox WF. 2002. Sensitivity to azoxystrobin among isolates of Uncinula necator: Baseline distribution and relationship to myclobutanil sensitivity. Plant Dis. 86:394–404.
- Yanar Y, Yanar D, Gebologlu N. 2011. Control of powdery mildew (Leveillula taurica) on tomato by foliar sprays of liquid potassium silicate (K2SO3). Afr J Biotech. 10:3121–3123.
- Zhang Y, Jewett TJ, Shipp JL. 2002. A dynamic model to estimate in-canopy and leaf-surface microclimate of greenhouse cucumber crops. Trans ASAE. 45:179–192.
- Ziv O, Zitter TA. 1992. Effects of bicarbonates and film-forming polymers on cucurbit foliar diseases. Plant Dis. 76:513–517.