Abstract
This study was conducted to identify and map stem rust resistance genes in Canada Western Red Spring (CWRS) wheat variety ‘AAC Prevail’ and elite line ‘BW961ʹ. A population of 227 doubled haploid lines from ‘AAC Prevail’/‘BW961ʹ and parents were evaluated for their response to stem rust (Puccinia graminis) at Njoro, Kenya, from 2016–2019 and genotyped using a targeted genotyping by sequencing SeqSNP assay. ‘AAC Prevail’ and ‘BW961ʹ were susceptible to race TTKSK in seedling tests in Canada. We identified stable QTL (significant in most environments tested) associated with field stem rust resistance on chromosome arms 2BS and 7AL, and minor QTL on 5AL, 6AS and 7BL. The QTL on 5AL, 7AL and 7BL were contributed by ‘AAC Prevail’, while those on 2BS and 6AS were contributed by ‘BW961ʹ. The QTL on 7AL was detected across all environments and explained 9.5% to 39% of the variation in disease severity (DS) and infection response (IR). The QTL on 2BS was associated with DS in all environments and in three of four environments for IR, and explained 4.8% to 12% of the variation in DS and IR. In two environments, the combination of 2BS and 7AL significantly enhanced field stem rust resistance compared with either QTL singly. Using SNP markers closely linked to the novel QTL on 2BS and 7AL, Kompetitive Allele Specific PCR markers were developed and validated in the population. These results provide breeders with new information and markers to utilize these sources of stem rust resistance.
Résumé
Cette étude a été menée pour identifier et cartographier les gènes de résistance à la rouille de la tige chez le cultivar ‘AAC Prevail’ et la lignée élite ‘BW961ʹ de blé roux de printemps de l’Ouest canadien. Une population de 227 lignées dihaploïdes de ‘AAC Prevail’/‘BW961ʹ et de parents ont été évalués en fonction de leur réaction à la rouille de la tige (Puccinia graminis) à Njoro, au Kenya, de 2016 à 2018, puis génotypées à l’aide d’un biotest SeqSNP de génotypage ciblé par séquençage. Chez les semis mis à l’essai au Canada, ‘AAC Prevail’ et ‘BW961ʹ étaient réceptifs à l’égard de la race TTKSK. Nous avons identifié des QTL stables (significatifs dans la majorité des environnements testés) associés à la résistance, au champ, à la rouille de la tige sur les bras 2BS et 7AL du chromosome et des QTL à effets mineurs sur les bras 5AL, 6AS et 7BL. Les QTL sur les bras 5AL, 7AL et 7BL étaient fournis par ‘AAC Prevail’, tandis que ceux sur 2BS et 6AS l’étaient par ‘BW961ʹ. Le QTL sur 7AL a été détecté dans tous les environnements et a expliqué de 9,5% à 39% de la variation de la gravité de la maladie (GM) et de la réaction à l’infection (RI). Le QTL sur 2BS était associé à la GM dans tous les environnements et à la RI dans trois environnements sur quatre, expliquant de 4,8% à 12% des variations de la GM et de la RI. Dans deux environnements, la combinaison de 2BS et 7AL a significativement amélioré la résistance à la rouille au champ, comparativement à l’un ou l’autre des QTL utilisé seul. Avec des marqueurs SNP étroitement liés au nouveau QTL situé sur les bras 2BS et 7AL, des marqueurs Kompetitive Allele Specific découlant de la PCR ont été développés, puis validés dans la population. Ces résultats fournissent aux sélectionneurs de nouvelles données et de nouveaux marqueurs pour qu’ils utilisent ces sources de résistance contre la rouille de la tige.
Introduction
Historically, stem rust, caused by Puccinia graminis f. sp. tritici Eriks. & E. Henn. (Pgt), was one of the most devastating diseases of wheat (Triticum aestivum L.). For North American wheat farmers, no disease was more pervasive and devastating in the late nineteenth and early twentieth centuries than stem rust (Peterson et al. Citation1948). For example, major epidemics of wheat stem rust occurred in the United States and Canada between 1900 and the 1950s (Peturson Citation1958; Kolmer et al. Citation2007; McCallum et al. Citation2007). To counteract the stem rust threat, wheat varieties were developed with stacks of genes conferring resistance (McIntosh et al. Citation1995; Singh et al. Citation2006; McCallum et al. Citation2007). In addition to the development of resistant cultivars, the eradication of the alternate host of Pgt (common barberry; Berberis vulgaris) from the major centres of wheat production in North America (Peterson Citation2018) has led to effective control of stem rust since the late 1950s. While development of varieties resistant to Pgt is a major achievement in wheat breeding, Pgt continues to be a major threat to wheat production with its prolific sporulation, efficient dissemination, pathogenic variability and ability to travel across continental regions (Roelfs Citation1989; Dean et al. Citation2012).
Global wheat production has been threatened by the emergence of the highly virulent race TTKSK (commonly known as Ug99) that was observed in Uganda in 1998 as the first race to defeat the stem rust resistance (Sr) gene Sr31 (Pretorius et al. Citation2000). Gene Sr31 has been used in agriculture on a large scale since the 1980s (Singh et al. Citation2015). Race TTKSK has continued to evolve and new Pgt races have emerged that overcome other widely deployed resistance genes including Sr21 (Singh et al. Citation2011), Sr24 (Jin et al. Citation2008), Sr36 (Jin et al. Citation2009), and SrTmp (Patpour et al. Citation2016), putting many wheat varieties at risk (Jin et al. Citation2008, Citation2009; Singh et al. Citation2015; Randhawa et al. Citation2018; Bhavani et al. Citation2019). The number of Ug99-type races has increased steadily, with thirteen variants being documented recently (Fetch et al. Citation2016; Bhavani et al. Citation2019; RustTracker Citation2021). The first variant of the Ug99 race group (TTKSF) was found in South Africa in 2000 and was avirulent on Sr31 (Visser et al. Citation2009; Singh et al. Citation2011). Another race from the Ug99 lineage (TTKST) with a combined virulence on Sr24 and Sr31 was identified in Kenya in 2006 (Jin et al. Citation2008). Other Ug99 lineage races identified in later years comprised TTKSP, PTKSK, PTKST, TTKSF+, TTTSK, TTKTT, TTHSK, PTKTK and TTHST, and TTKTK (Pretorius et al. Citation2012; Fetch et al. Citation2016; Patpour et al. Citation2016; Terefe et al. Citation2019; RustTracker Citation2021). Non-Ug99 related virulent races that caused epidemics and localized outbreaks in different countries have also been reported in recent years. One example is the emergence of TKTTF, a race that is genetically unrelated to Ug99 race group, which has overcome the most widely grown wheat cultivar Digalu in Ethiopia (Olivera et al. Citation2015). The second example is race TRTTF that was found in Yemen in 2006 and subsequently in Ethiopia and it has defeated resistant genes such as Sr9e, SrTmp, and SrIRAmigo (Olivera et al. Citation2012; Guerrero-Chavez et al. Citation2015). Nonetheless, Hiebert et al. (Citation2017) report gene Sr8a confers resistance to TRTTF in the Canadian wheat cultivar ‘Harvest’.
Variants of Ug99 have been discovered in the Eastern African highlands, Southern African countries, Yemen, Egypt, and Iran, with a high chance of further spread into large wheat growing belts of Asia and beyond (Singh et al. Citation2015; Olivera et al. Citation2018). The spread of the Ug99 race group can be minimized by replacing susceptible varieties with new rust resistant varieties, which can be developed through pre-emptive resistance breeding. For example, the deployment of resistance gene combinations is expected to slow the evolution of Ug99 races and minimize potential crop losses (Randhawa et al. Citation2018) while increasing the longevity of genes in the field. Singh et al. (Citation2015) recently recommended deployment of new varieties with multiple race non-specific genes or adult plant resistance (APR) and reducing the cultivation of susceptible varieties as the best stem rust management strategy. There currently are 61 designated stem rust resistance genes (McIntosh et al. Citation2020) and several quantitative loci (McIntosh et al. Citation2014, Citation2020; Yu et al. Citation2014) have been documented. Most of the designated genes are race-specific and provide all-stage resistance. Some of these genes originate from the primary gene pool of wheat while others were transferred to wheat from more distant relatives. Resistance transferred from outside of the primary gene pool is often accompanied by deleterious traits (The et al. Citation1988), thus resistance from the primary gene pool is preferred. Genes Sr9h, Sr13, Sr14, Sr22, Sr28, Sr33, Sr35, Sr42 and Sr45 are examples of all-stage resistance genes from the primary gene pool of wheat that confer resistance to Ug99 (Jin et al. Citation2007; Hiebert et al. Citation2010), while genes Sr2, Sr55, Sr56, Sr57, and Sr58 confer resistance at the adult stage of plants (Singh et al. Citation2015). Yu et al. (Citation2014) provide a comprehensive list of stem rust resistance genes and quantitative trait loci (QTL) conferring resistance to Ug99.
Bhavani et al. (Citation2019) indicated the usefulness of combining APR genes in high-yielding backgrounds and discovery of new quantitative trait loci conferring stem rust resistance to enhance the durability of resistance. Field trials indicated that the CWRS wheat cultivar ‘AAC Prevail’ (Kumar et al. Citation2017) and elite line ‘BW961ʹ carry moderate resistance to Ug99 and other stem rust races in Kenya. The genes conferring field stem rust resistance in these two wheat lines have not been characterized. This study sought to map genomic regions associated with stem rust resistance of adult plants in a doubled haploid population of a cross between ‘AAC Prevail’ and ‘BW961ʹ.
Materials and methods
Plant materials
‘AAC Prevail’ (McKenzie/RL4933//Somerset) is a hard red spring wheat cultivar developed by the Cereal Research Centre (CRC), Agriculture and Agri-Food Canada (AAFC). ‘AAC Prevail’ is a high-yielding wheat variety adapted to the growing conditions in the Canadian Prairies and rated moderately resistant to stem rust in Canada (Kumar et al. Citation2017). ‘BW961ʹ (‘Alsen’/‘Waskada’) is an elite spring wheat line developed at Swift Current Research and Development Centre, AAFC and is resistant to stem rust pathotypes found in Canada (https://www.inspection.gc.ca/english/plaveg/pbrpov/cropreport/whe/app00009618e.shtml). Both lines displayed moderate field stem rust resistance in Kenya in 2012 (T. Fetch, unpublished data). ‘AAC Prevail’ was crossed with ‘BW961ʹ and the F1 plants of the cross were used to generate a doubled haploid population of 227 lines by the maize pollination method (Knox et al. Citation2000).
Stem rust disease evaluation
The response of ‘AAC Prevail’ and ‘BW961ʹ to the stem rust race TTKSK at the seedling stage was evaluated at the AAFC Morden Research and Development Centre, Canada, in a PPC Level 3 biocontainment facility following the procedures of Hiebert et al. (Citation2016). Seedlings were rated for their infection types (ITs) 14 days post-inoculation using the 0–4 scale described by Stakman et al. (Citation1962), with ITs of 0 to 2 classified as resistant and ITs of 3 to 4 classified as susceptible. For the field stem rust response study, the 227 DH lines of the cross ‘AAC Prevail’/‘BW961ʹ were grown in randomized unreplicated rows for four consecutive growing seasons (2016 to 2019) at Njoro, Kenya. The Njoro rust screening nursery is jointly managed by the Kenyan Agricultural and Livestock Research Organization (KALRO) and the International Centre for Maize and Wheat Improvement (CIMMYT). Parents and DH lines were randomized, with parental rows replicated three times in the 2018 and 2019 nurseries, and unreplicated in the 2016 and 2017 nurseries.
Seed of each line was planted in double rows of 70 cm length and 30 cm spacing. A bulked mix of urediniospores including the Ug99 lineage races TTKSK, TTKST, TTTSK, TTKTK, and TTKTT (Kosgey et al. Citation2021) were inoculated onto susceptible spreader rows to facilitate disease development and spread in the nursery from 2016 to 2019. Adult plant field rating of disease severity from 0% to 100% was assessed using the modified Cobb scale (Peterson et al. Citation1948). The infection response (IR) was rated as resistant (R), moderately resistant (MR), moderately susceptible (MS), or susceptible (S), or intermediate categories of RMR, MRMS, or MSS, modified from Roelfs et al. (Citation1992). Ratings at two different dates were made for each nursery between heading and plant maturity, with the second rating date of stem rust severity and infection response used in data analysis and reporting. For the QTL analysis, the IR ratings were converted to numbers as R = 1, RMR = 2, MR = 3, MRMS = 4, MS = 5, MSS = 6, and S = 7.
Genotyping and genetic mapping
A portion of primary leaves of the parents and progeny lines were excised for DNA extraction and purification using the oKtopure Automated DNA Extraction System and the Sbeadex Maxi Plant Kit (LGC Genomics, Beverly, MA) following manufacturer’s instructions. DNA samples of parents and progeny were genotyped using LGC’s customizable SeqSNP (LGC Citation2019) targeted genotyping by sequencing (tGBS) service. A subset of 5151 SNPs were chosen from the 81 587 wheat 90 K Infinium iSelect marker panel for the SeqSNP service based on high polymorphism information content (PIC) in Swift Current Research and Development Centre breeding material and even distribution across IWGSC RefSeq v1.0 (Alaux et al. Citation2018). The SeqSNP tGBS service entailed providing LGC SNP source sequences for Single Primer Enrichment Technology (SPET) probe library production (Nugen®, SPET, United States Patent 9 650 628), sequencing resulting libraries, and variant analysis to provide a final genotypic matrix of the markers for downstream analysis.
Genetic mapping and QTL analysis
Polymorphic markers obtained from the tGBS were used in linkage analysis. Linkage groups were constructed using the regression model in JoinMap v5 (https://www.kyazma.nl/index.php/JoinMap/). Recombination frequencies were translated to map distances in centimorgans (cM) using the Kosambi function (Kosambi Citation1943). Assignment of SNP linkage group markers to wheat chromosomes was based on Wang et al. (Citation2014). Quantitative trait loci (QTL) analysis was performed on the population using MapQTL.6 ® (Jansen et al. Citation1995; Van Ooijen Citation2009). The permutation test option of 1000 permutations was used to determine the significant threshold for the logarithm of the odds (LOD) ratios. The QTL identified were declared significant at the 5% level of significance based on the genome-wide (GW) threshold level. Co-factor markers used in multiple QTL Mapping (MQM), were identified by automatic backward marker elimination or manually. The linkage maps of QTL identified in the study were drawn in MapChart software (Voorrips Citation2002). Analysis of variance was performed in SAS (SAS institute, Cary, NC) to investigate the effect of the combination of the strongest QTL on stem rust resistance. Duncan’s Multiple Range test (DMRT) was used to determine differences between mean disease severity of groups of the lines classified based on QTL combinations. Linkage groups of consistent QTL were aligned with independently generated high-density consensus maps of common wheat (Wang et al. Citation2014; Bokore et al. Citation2020). Kompetitive Allele Specific PCR (KASP) markers were designed (Allen et al. Citation2011) for SNPs most closely associated with the most consistently expressed QTL. The developed KASP markers were validated in the ‘AAC Prevail’/‘BW961ʹ population.
Results
Disease evaluation
‘AAC Prevail’ and ‘BW961ʹ were both susceptible to TTKSK at the seedling stage and were rated with ITs of 33+ and 33− respectively. During field testing in Kenya, the 2016 and 2019 environments exhibited higher disease pressures than in 2017 and 2018 based on mean severity within the population (). Stem rust severity of ‘AAC Prevail’ was similar to ‘BW961ʹ across four years of testing. The infection response of both parents was MR in 2016, MRMS in 2018, and MS in 2019. In 2017, ‘AAC Prevail’ exhibited a lower MRMS infection response than the MSS response for ‘BW961ʹ. The DH lines had a wide range of stem rust severity and the infection response ranged from R to S. The distribution of disease severity of the population was generally continuous and skewed towards lower percent severity (). In separate tests of isolates obtained from 2019 Kenya nursery, race TKTTF was detected at trace (two samples) levels (Fetch, unpublished data).
Table 1. Mean and range of the stem rust severity and infection response scores for the ‘AAC Prevail’/‘BW961ʹ doubled haploid population and parents evaluated in field nursery near Njoro, Kenya for four years, 2016–2019
Fig. 1 Frequency distribution of stem rust severity in the ‘AAC Prevail’/’BW961ʹ doubled haploid population evaluated for the Ug99 and other stem rust races at Njoro experimental station, Kenya for each year from 2016 to 2019. Dark arrows indicate the stem rust response of the parents ‘AAC Prevail’ and ‘BW961ʹ
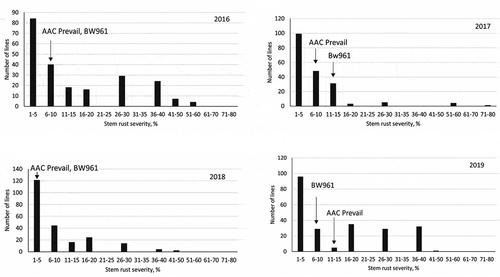
Genetic map
Of the 5151 SNPs genotyped, 963 were useful for linkage mapping and were assigned to 32 linkage groups (Supplemental Table 1). The 963 SNP markers covered a total length of 2898.8 cM distributed across 32 linkage groups covering all chromosomes of the hexaploid wheat genome except chromosome 4D, with an averaged interval of 3.5 cM between markers. The greatest number of SNPs were assigned to the B genome, followed by the A genome, with the fewest on the D genome.
Stem rust resistance QTL identified
Five QTL associated with resistance to Ug99 and other stem rust races were detected at Njoro, Kenya (). The QTL located on chromosomes 2B (designated as QSr-sparc-2B) and 7A (QSr-sparc-7A) were identified in multiple environments, including ones with lower disease intensity (2017 and 2018) (). The three QTL located on chromosomes 5A (QSr-sparc-5A), 6A (QSr-sparc-6A) and 7B (QSr-sparc-7B) were limited to single environments (Supplemental Fig. 2). The QSr-sparc-5A, QSr-sparc-7A, and QSr-sparc-7B QTL were contributed by ‘AAC Prevail’, and QSr-sparc-2B and QSr-sparc-6A QTL were contributed by ‘BW961ʹ (). The relative map positions of QSr-sparc-2B and QSr-sparc-7A QTL were compared with two high density hexaploid wheat consensus maps (Wang et al. Citation2014; Bokore et al. Citation2020) and showed good colinearity ().
Table 2. Chromosomal position, associated markers, phenotype variation explained (PVE) and additive effects of stem rust resistance quantitative trait loci (QTL) detected in ‘AAC Prevail’/‘BW961ʹ population at Njoro, Kenya nursery evaluated for four years (2016–2019)
Fig. 2 Linkage map of stem rust resistance QTL detected on chromosomes 2B and 7A in the ‘BW961ʹ/‘AAC Prevail’ population in Njoro, Kenya from 2016 to 2019, aligned with previously published hexaploid wheat high density consensus maps (Wang et al. Citation2014; Bokore et al. Citation2020)
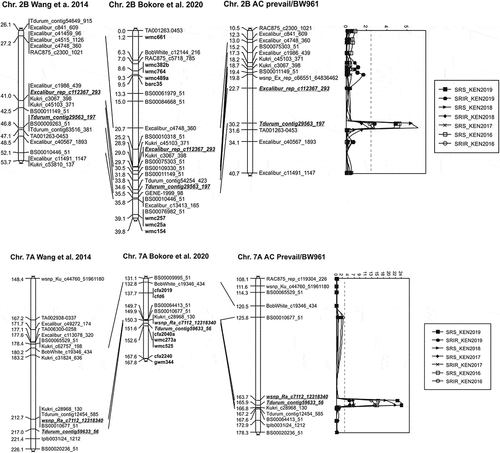
With the highest LOD scores, ranging from 5.0 to 24.2, the QSr-sparc-7A QTL was consistently detected across all environments for both stem rust severity and infection response. QSr-sparc-7A had a greater phenotypic effect (up to 39%), on stem rust severity than on infection response (up to 25%). The SNP markers associated with QSr-sparc-7A, Tdurum_contig59633_56 and wsnp_Ra_c7112_12318340, are located on the long arm of chromosome 7A (Wang et al. Citation2014). QSr-sparc-2B was the second strongest QTL and was associated with disease severity across all environments. This QTL accounted for the second largest proportion of phenotypic variation explained (PVE) after QSr-sparc-7A with up to 12% for stem rust severity. The effect of QSr-sparc-2B on infection response was marginal, with the LOD scores only slightly above the threshold of statistical significance. Markers closely associated with QSr-sparc-2B (Tdurum_contig29563_197 and TA001263-0453) are located on the short arm of chromosome 2B (Wang et al. Citation2014).
The QSr-sparc-2B QTL alone significantly reduced disease severity in each year, by an average of about 50% (). The QSr-sparc-7A QTL alone also significantly reduced severity in each year, by an average of about 70%, and was significantly greater than QSr-sparc-2B in reducing stem rust severity. The combination of QSr-sparc-2B with QSr-sparc-7A did not significantly reduce stem rust severity in 2016 or 2017 over QSr-sparc-7A alone, but did significantly reduce stem rust severity over each locus singly in 2018 and 2019.
Fig. 3 Individual and combined effects of stem rust resistance QTL located on chromosome 2B associated with the SNP marker Tdurum_contig29563_197 and 7A with Tdurum_contig59633_56 in Njoro, Kenya. Bars with the same letter within experiment (2016–2019) are not significantly different according to the Duncan’s Multiple Range test at P = 0.05.
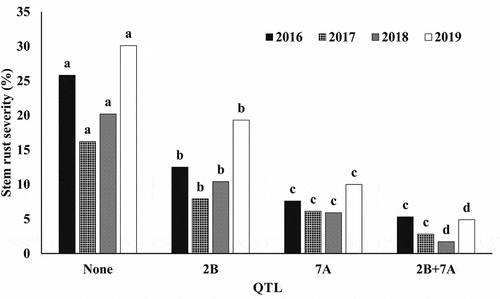
KASP markers were developed for SNPs closely associated with the stem rust resistance QTL QSr-sparc-2B and QSr-sparc-7A (). Three QSr-sparc-7A predictive KASPs, designated kwh810, kwh811 and kwh812 were developed. Additionally, a KASP marker, designated kwh808 was developed for QSr-sparc-2B. Marker validation based on the 227 lines and parents produced high quality clusters between alleles of the lines at each locus (Supplemental Fig. 2). Allele calls of each progeny genotype matched with alleles of parents ‘AAC Prevail’ or ‘BW961ʹ.
Table 3. List of KASP markers developed for stem rust resistance QSr-sparc-2B and QSr-sparc-7A and associated primer sequences
Discussion
There was a lack of seedling resistance to race TTKSK in both ‘AAC Prevail’ (IT = 33+) and ‘BW961ʹ (IT = 33−). Since the other four races (TTKST, TTTSK, TTKTK, and TTKTT) that were inoculated on spreader rows in the Njoro nursery (Kosgey et al. Citation2021) are more virulent than TTKSK, there would not be any major all-stage resistance genes in either parent effective to the Ug99 race lineage. The detection of race TKTTF may have influenced the IR in the 2019 nursery, as this was the only one where both parents had an MS response. However, significant QTL for both DS and IR were still observed on 2BS and 7AL in 2019, albeit at lower percent PVE than in previous years. The distribution observed in stem rust severity of the population indicates the resistance was conferred by multiple APR genes. The subsequent detection of two stable QTL for stem rust resistance is consistent with the nature of the phenotypic distribution. The field test results of ‘AAC Prevail’ and ‘BW961ʹ are in agreement with our preliminary observation that both wheat lines displayed an intermediate level of resistance to stem rust in Kenya. Furthermore, the absence of Ug99 seedling resistance in ‘AAC Prevail’ and ‘BW961ʹ is not surprising based on their pedigrees, which was also confirmed by seedling stem rust assays with Pgt race TTKSK.
‘AAC Prevail’ carries QTL QSr-sparc-7A that was effective against the Pgt races in Njoro across four years. Two stem rust resistance genes, Sr15 and Sr22, have been located to chromosome arm 7AL (Watson and Luig Citation1966; Gerechter-Amitai et al. Citation1971; The Citation1973; Olson et al. Citation2010; Yu et al. Citation2014). A marker for QSr-sparc-7A, Tdurum_contig59633_56, is situated proximal to cfa2019, a marker linked with Sr22 (Khan et al. Citation2005; Liu et al. Citation2014) (). Since Sr22 confers all-stage resistance and is effective at the seedling stage (Olson et al. Citation2010), the QSr-sparc-7A QTL detected in this paper cannot be Sr22. Gene Sr15 is linked to SSR markers Xgwm344 and Xcfa2240 (Jayatilake et al. Citation2013), which mapped 16.2 cM distal to Tdurum_contig59633_56 on the consensus map of Bokore et al. (Citation2020). The proximity on the map cannot rule out that Sr15 is responsible for the effect of QSr-sparc-7A. Gene Sr15 is temperature sensitive, and the seedling response is effective against race TTKSK under low incubation temperatures (15–18°C) but not at higher temperatures (22–25°C) (Gao et al. Citation2019). However, the reaction of Sr15 (RL1888, Prelude*4/Norka) under field conditions in Njoro from 2016 to 2019 was always susceptible (Fetch, unpublished data), while the QSr-sparc-7A QTL was effective in all the years. Bhavani et al. (Citation2011) reported a chromosome 7A QTL associated with DArT marker XwPt-8670. This marker is about 35 Mbp from the AC Prevail derived QTL SNP marker wsnp_Ra_c7112_12318340 and 37 Mbp from Tdurum_contig59633_56 on the RefSeq.V1.0 wheat physical map, suggesting two different genes. Given that Sr15 and Sr22 can be excluded as the underlying gene of QSr-sparc-7A and the physical separation from XwPt-8670, the data suggests that QSr-sparc-7A could be the location of a novel gene.
Elite wheat line ‘BW961ʹ carries the stable QTL QSr-sparc-2B located on the short arm of chromosome 2B (). Genes Sr36, Sr39, and Sr40 are also on chromosome 2BS (Tsilo et al. Citation2008; Mago et al. Citation2009; Niu et al. Citation2011; Bernardo et al. Citation2013; Yu et al. Citation2014; Chemayek et al. Citation2017) and effective to most races in the Ug99 lineage. Comparing the map position of QSr-sparc-2B with Sr36, Sr39, and Sr40 shows that markers linked to Sr39 are near QSr-sparc-2B while markers linked to Sr36 and Sr40 are distant to QSr-sparc-2B (Bariana et al. Citation2001; Tsilo et al. Citation2008; Mago et al. Citation2009; Wu et al. Citation2009; Niu et al. Citation2011). Since Sr36, Sr39, and Sr40 all confer seedling resistance to TTKSK (Jin et al. Citation2009) and ‘BW961ʹ is susceptible to TTKSK at the seedling stage, none of these genes can be the QSr-sparc-2B QTL. Gene Sr23 is also on 2BS, but is not effective at the seedling stage to race TTKSK (Jin et al. Citation2007). Since Lr16, which is tightly linked or pleitropic to Sr23 (McIntosh and Luig Citation1973), is common in Canadian wheat, this gene may be present in the population. Genotyping ‘AAC Prevail’ and ‘BW961ʹ with two KASP markers that co-segregate with Lr16 and are predictive of the gene (Kassa et al. Citation2017) showed that both parents appear to be carriers of Lr16/Sr23 (data not shown). Thus, the resistance conferred by QSr-sparc-2B must be conditioned by a different gene. There have been other QTL for stem rust resistance detected on 2BS as summarized by Kosgey et al. (Citation2021), Bajgain et al. (Citation2015) and others.
‘AAC Prevail’ has two additional QTL located on chromosome arms 5AL and 7BL that have minor effects on the stem rust. Similarly, a minor locus on chromosome arm 6AS was contributed by ‘BW961ʹ. The detection of the QTL on 5AL and 7BL in 2016 and the 6AS QTL in 2018 and their absence in the other three years indicates they are sensitive to environmental influence.
The enhanced resistance conditioned by co-occurrence of QSr-sparc-2B and QSr-sparc-7A indicates the usefulness of stacking these genes. Stacking QSr-sparc-2B and QSr-sparc-7A with other Ug99 resistance could improve the durability of resistance. KASP markers that can readily be deployed in wheat breeding programmes for MAS were designed for SNPs associated with the 2B and 7A stem rust resistance QTL.
Supplemental Material
Download MS Word (1 MB)Acknowledgements
We thank Mandeep Randhawa from the International Centre for Maize and Wheat Improvement (CIMMYT) and Godwin Macharia from the Kenyan Agricultural and Livestock Research Organization (KALRO) for managing field stem rust nurseries at Njoro and technical support.
Disclosure statement
No potential conflict of interest was reported by the author(s).
Supplemental material
Supplemental data for this article can be accessed online here: https://doi.org/10.1080/07060661.2021.1966651.
Additional information
Funding
References
- Alaux M, Rogers J, Letellier T, Flores R, Alfama F, Pommier C, Mohellibi N, Durand S, Kimmel E, Michotey C, et al. 2018. Linking the international wheat genome sequencing consortium bread wheat reference genome sequence to wheat genetic and phenomic data. Genome Biol. 19(1):111. doi:https://doi.org/10.1186/s13059-018-1491-4
- Allen AM, Barker GL, Berry ST, Coghill JA, Gwilliam R, Kirby S, Robinson P, Brenchley RC, D’Amore R, McKenzie N, et al. 2011. Transcript-specific, single-nucleotide polymorphism discovery and linkage analysis in hexaploid bread wheat (Triticum aestivum L.). Plant Biotechnol J. 9(9):1086–274. doi:https://doi.org/10.1111/j.1467-7652.2011.00628.x
- Bajgain P, Rouse M, Bhavani S, Anderson J. 2015. QTL mapping of adult plant resistance to Ug99 stem rust in the spring wheat population RB07/MN06113-8. Mol Breeding. 35(8):1–15. doi:https://doi.org/10.1007/s11032-015-0362-x
- Bariana HS, Hayden MJ, Ahmed NU, Bell JA, Sharp PJ, McIntosh RA. 2001. Mapping of durable adult plant and seedling resistances to stripe rust and stem rust diseases in wheat. Aust J Agr Res. 52(11–12):1247–1255. doi:https://doi.org/10.1071/AR01040
- Bernardo AN, Bowden RL, Rouse MN, Newcomb MS, Marshall DS, Bai G. 2013. Validation of molecular markers for new stem rust resistance genes in U.S. hard winter wheat. Crop Sci. 53(3):755–764. doi:https://doi.org/10.2135/cropsci2012.07.0446
- Bhavani S, Hodson DP, Huerta-Espino J, Randhawa MS, Singh RP. 2019. Progress in breeding for resistance to Ug99 and other races of the stem rust fungus in CIMMYT wheat germplasm. Front Agr Sci Eng. 6(3):210–224. doi:https://doi.org/10.15302/J-FASE-2019268
- Bhavani S, Singh RP, Argillier O, Huerta-Espino J, Singh S, Njau P. 2011. Mapping of durable adult plant stem rust resistance in six CIMMYT wheats to Ug99 group of races. In: McIntosh R, editor. Proceedings of the borlaug global rust initiative 2011 technical workshop. Saint Paul (Minnesota, Ithaca): Borlaug Global Rust Initiative; p. 43–53, June 13–16.
- Bokore FE, Knox RE, Cuthbert RD, Pozniak CJ, McCallum BD, N’Diaye A, DePauw RM, Campbell HL, Munro C, Singh A, et al. 2020. Mapping quantitative trait loci associated with leaf rust resistance in five spring wheat populations using single nucleotide polymorphism markers. PLoS ONE. 15(4):e0230855. doi:https://doi.org/10.1371/journal.pone.0230855
- Chemayek B, Bansal UK, Qureshi N, Zhang P, Wagoire WW, Bariana HS. 2017. Tight repulsion linkage between Sr36 and Sr39 was revealed by genetic, cytogenetic and molecular analyses. Theor Appl Genet. 130(3):587–595. doi:https://doi.org/10.1007/s00122-016-2837-5
- Dean R, Van Kan JAL, Pretorius ZA, Hammond-Kosack KE, Di Pietro A, Spanu PD, Rudd JJ, Dickman M, Kahmann R, Ellis J, et al. 2012. The top 10 fungal pathogens in molecular plant pathology. Mol Plant Path. 13(4):414–430. doi:https://doi.org/10.1111/j.1364-3703.2011.00783.x
- Fetch T, Zegeye T, Park RF, Hodson D, Wanyera R. 2016. Detection of wheat stem rust races TTHSK and PTKTK in the Ug99 race group in Kenya in 2014. Plant Dis. 100:1495. doi:https://doi.org/10.1094/PDIS-11-15-1356-PDN.
- Gao L, Babiker EM, Nava IC, Nirmala J, Bedo Z, Lang L, Chao S, Gale S, Jin Y, Anderson JA, et al. 2019. Temperature-sensitive wheat stem rust resistance gene Sr15 is effective against Puccinia graminis f. sp. tritici race TTKSK. Plant Path. 68(1):143–151. doi:https://doi.org/10.1111/ppa.12928
- Gerechter-Amitai ZK, Wahl I, Vardi A, Zohary D. 1971. Transfer of stem rust seedling resistance from wild diploid einkorn to tetraploid durum wheat by means of a triploid hybrid bridge. Euphytica. 20(2):281–285. doi:https://doi.org/10.1007/BF00056088
- Guerrero-Chavez R, Glover KD, Rouse MN, Gonzalez-Hernandez JL. 2015. Mapping of two loci conferring resistance to wheat stem rust pathogen races TTKSK (Ug99) and TRTTF in the elite hard red spring wheat line SD4279. Mol Breed. 35(1):8. doi:https://doi.org/10.1007/s11032-015-0198-4
- Hiebert CW, Fetch TG Jr, Zegeye T. 2010. Genetics and mapping of stem rust resistance to Ug99 in the wheat cultivar Webster. Theor Appl Genet. 121(1):65–69. doi:https://doi.org/10.1007/s00122-010-1291-z
- Hiebert CW, Kassa MT, McCartney CA, You FM, Rouse MN, Fobert P, Fetch TG. 2016. Genetics and mapping of seedling resistance to Ug99 stem rust in winter wheat cultivar Triumph 64 and differentiation of SrTmp, SrCad, and Sr42. Theor Appl Genet. 129(11):2171–2177. doi:https://doi.org/10.1007/s00122-016-2765-4
- Hiebert CW, Rouse MN, Nirmala J, Fetch T. 2017. Genetic mapping of stem rust resistance to Puccinia graminis f. Sp. tritici race TRTTF in the Canadian wheat cultivar harvest. Phytopathology. 107(2):192–197. doi:https://doi.org/10.1094/PHYTO-05-16-0186-R
- Jansen RC, Van Ooijen JW, Stam P, Lister C, Dean C. 1995. Genotype-by-environment interaction in genetic mapping of multiple quantitative trait loci. Theor Appl Genet. 91(1):33–37. doi:https://doi.org/10.1007/BF00220855
- Jayatilake DV, Tucker EJ, Bariana H, Kuchel H, Edwards J, McKay AC, Chalmers K, Mather DE. 2013. Genetic mapping and marker development for resistance of wheat against the root lesion nematode Pratylenchus neglectus. BMC Plant Biol. 13:230. doi:https://doi.org/10.1186/1471-2229-13-230.
- Jin Y, Singh RP, Ward RW, Wanyera R, Kinyua M, Njau P, Fetch T, Pretorius ZA, Yahyaoui A. 2007. Characterization of seedling infection types and adult plant infection responses of monogenic Sr gene lines to race TTKS of Puccinia graminis f. sp. tritici. Plant Dis. 91(9):1096–1099. doi:https://doi.org/10.1094/PDIS-91-9-1096
- Jin Y, Szabo LJ, Pretorius ZA, Singh RP, Ward R, Fetch T Jr. 2008. Detection of virulence to resistance gene Sr24 within race TTKS of Puccinia graminis f. sp. tritici. Plant Dis. 92(6):923–926. doi:https://doi.org/10.1094/PDIS-92-6-0923
- Jin Y, Szabo LJ, Rouse MN, Fetch T, Pretorius ZA, Wanyera R, Njau P. 2009. Detection of virulence to resistance gene Sr36 within the TTKS race lineage of Puccinia graminis f. sp. tritici. Plant Dis. 93(4):367–370. doi:https://doi.org/10.1094/PDIS-93-4-0367
- Kassa MT, You FM, Hiebert CW, Pozniak CJ, Fobert PR, Sharpe AG, Menzies JG, Humphreys G, Rezac Harrison N, Fellers JP, et al. 2017. Highly predictive SNP markers for efficient selection of the wheat leaf rust resistance gene Lr16. BMC Biol. 17(1):45.
- Khan RR, Bariana HS, Dholakia BB, Naik SV, Lagu MD, Rathjen AJ, Bhavani S, Gupta VS. 2005. Molecular mapping of stem and leaf rust resistance in wheat. Theor Appl Genet. 111(5):846–850. doi:https://doi.org/10.1007/s00122-005-0005-4
- Knox RE, Clarke JM, DePauw RM. 2000. Dicamba and growth condition effects on doubled haploid production in durum wheat crossed with maize. Plant Breed. 119:289–298. doi:https://doi.org/10.1046/j.1439-0523.2000.00498.x.
- Kolmer JA, Jin Y, Long DL. 2007. Wheat leaf and stem rust in the United States. Aust J Agr Res. 58:631–638. doi:https://doi.org/10.1071/AR07057.
- Kosambi DD. 1943. The estimation of map distances from recombination values. Ann Eugen. 12:172–175. doi:https://doi.org/10.1111/j.1469-1809.1943.tb02321.x.
- Kosgey ZC, Edae EA, Dill-Macky R, Jin Y, Bulbula WD, Gemechu A, Macharia G, Bhavani S, Randhawa MS, Rouse MN. 2021. Mapping and validation of stem rust resistance loci in spring wheat line CI 14275. Front Plant Sci. 11:609659. doi:https://doi.org/10.3389/fpls.2020.609659.
- Kumar S, Fox SL, Humphreys DG, Mitchell Fetch J, Green D, Fetch T, McCallum B, Menzies J. 2017. AAC prevail Canada western red spring wheat. Can J Plant Sci. 98(2):475–482.
- LGC. 2019. Biosearch technologies. SeqSNP targeted GBS as alternative for array genotyping in routine breeding programs [White Paper]. [accessed 2019 July]. https://biosearch-cdn.azureedge.net/assetsv6/seqsnp-tgbs-alternative-genotyping-routine-breeding-programs.pdf.
- Liu S, Rudd JC, Bai G, Haley SD, Ibrahim AMH, Xue Q, Hays DB, Graybosch RA, Devkota RN, St. Amand P. 2014. Molecular markers linked to important genes in hard winter wheat. Crop Sci. 54(4):1304–1321. doi:https://doi.org/10.2135/cropsci2013.08.0564
- Mago R, Zhang P, Bariana HS, Verlin DC, Bansal UK, Ellis JG, Dundas IS. 2009. Development of wheat lines carrying stem rust resistance gene Sr39 with reduced Aegilops speltoides chromatin and simple PCR markers for marker-assisted selection. Theor Appl Genet. 119(8):1441–1450. doi:https://doi.org/10.1007/s00122-009-1146-7
- McCallum BD, Fetch T, Chong J. 2007. Cereal rust control in Canada. Aust J Agr Res. 58(6):639–647. doi:https://doi.org/10.1071/AR06145
- McIntosh R, Wellings C, Park R. 1995. Wheat rusts: an atlas of resistance genes. East Melbourne, Australia: CSIRO Publishing.
- McIntosh RA, Dubcovsky J, Rogers WJ, Morris CF, Appels R, Xia CX 2014. Catalogue of gene symbols for wheat: 2013-14 supplement. [accessed 2021 June]. https://shigen.nig.ac.jp/wheat/komugi/genes/macgene/supplement2013.pdf.
- McIntosh RA, Dubcovsky J, Rogers WJ, Xia CX, Raupp WJ 2020. Catalogue of gene symbols for wheat: 2020 supplement. [accessed 2021 June]. https://wheat.pw.usda.gov/GG3/sites/default/files/Catalogue%20of%20Gene%20Symbols%20for%20Wheat%20-%20supplement2020.pdf.
- McIntosh RA, Luig NH. 1973. Linkage of genes for reaction to Puccinia graminis f. sp. tritici and P. recondita in Selkirk wheat and related cultivars. Aust J BioI Sci. 26:1145–1152. doi:https://doi.org/10.1071/BI9731145.
- Niu Z, Klindworth DL, Friesen TL, Chao S, Jin Y, Cai X, Xu SS. 2011. Targeted introgression of a wheat stem rust resistance gene by DNA marker-assisted chromosome engineering. Genetics. 187(4):1011–1021. doi:https://doi.org/10.1534/genetics.110.123588
- Olivera P, Newcomb M, Szabo LJ, Rouse M, Johnson J, Gale S, Luster DG, Hodson D, Cox JA, Burgin L, et al. 2015. Phenotypic and genotypic characterization of race TKTTF of Puccinia graminis f. sp. tritici that caused a wheat stem rust epidemic in Southern Ethiopia in 2013–14. Phytopathology. 105(7):917–928. doi:https://doi.org/10.1094/PHYTO-11-14-0302-FI
- Olivera PD, Jin Y, Rouse M, Badebo A, Fetch T Jr, Singh RP, Yahyaoui A. 2012. Races of Puccinia graminis f. sp. tritici with combined virulence to Sr13 and Sr9e in a field stem rust screening nursery in Ethiopia. Plant Dis. 96(5):623–628. doi:https://doi.org/10.1094/PDIS-09-11-0793
- Olivera PD, Rouse MN, Jin Y. 2018. Identification of new sources of resistance to wheat stem rust in Aegilops spp. in the tertiary genepool of wheat. Front Plant Sci. 9:1719. doi:https://doi.org/10.3389/fpls.2018.01719.
- Olson EL, Brown-Guedira G, Marshall D, Stack E, Bowden RL, Jin Y, Rouse M, Pumphrey MO. 2010. Development of wheat lines having a small introgressed segment carrying stem rust resistance gene Sr22. Crop Sci. 50(5):1823–1830. doi:https://doi.org/10.2135/cropsci2009.11.0652
- Patpour M, Hovmøller MS, Justesen AF, Newcomb M, Olivera P, Jin Y, Szabo LJ, Hodson D, Shahin AA, Wanyera R, et al. 2016. Emergence of virulence to SrTmp in the Ug99 race group of wheat tritici, in Africa. Plant Dis. 100(2):522. doi:https://doi.org/10.1094/PDIS-06-15-0668-PDN
- Peterson PD. 2018. The barberry eradication program in Minnesota for stem rust control: a case study. Annu Rev Phytopathol. 56(1):203–223. doi:https://doi.org/10.1146/annurev-phyto-080417-050133
- Peterson R, Campbell A, Hannah A. 1948. A diagramatic scale for estimating rust intensity of leaves and stem of cereals. Can J Res. 26:496–500. doi:https://doi.org/10.1139/cjr48c-033.
- Peturson B. 1958. Wheat rust epidemics in western Canada in 1953, 1954 and 1955. Can J Plant Sci. 38:16–38. doi:https://doi.org/10.4141/cjps58-004.
- Pretorius ZA, Singh RP, Wagoire WW, Payne TS. 2000. Detection of virulence to wheat stem rust resistance gene Sr31 in Puccinia graminis. f. sp. tritici in Uganda. Plant Dis. 84(2):203. doi:https://doi.org/10.1094/PDIS.2000.84.2.203B
- Pretorius ZA, Szabo LJ, Boshoff WHP, Herselman L, Visser B. 2012. First report of a new ttksf race of wheat stem rust (Puccinia graminis f. sp. tritici) in South Africa and Zimbabwe. Plant Dis. 96(4):590. doi:https://doi.org/10.1094/PDIS-12-11-1027-PDN
- Randhawa MS, Singh RP, Dreisigacker S, Bhavani S, Huerta-Espino J, Rouse MN, Nirmala J, Sandoval-Sanchez M. 2018. Identification and validation of a common stem rust resistance locus in two bi-parental populations. Front Plant Sci. 9:1788. doi:https://doi.org/10.3389/fpls.2018.01788.
- Roelfs AP. 1989. Epidemiology of the cereal rusts in north america. Can J Plant Pathol. 11(1):1–90. doi:https://doi.org/10.1080/07060668909501153
- Roelfs AP, Singh RP, Saari EE. 1992. Rust diseases of wheat: concepts and methods of disease management. Mexico: CIMMYT.
- RustTracker. 2021. RustTracker.org: a global wheat rust monitoring system. [accessed 2021 June]. https://rusttracker.cimmyt.org/.
- Singh RP, Hodson DP, Huerta-Espino J, Jin Y, Bhavani S, Njau P, Herrera-Foessel S, Singh PK, Singh S, Govindan V. 2011. The emergence of Ug99 races of the stem rust fungus is a threat to world wheat production. Annu Rev Phytopathol. 49:465–481. doi:https://doi.org/10.1146/annurev-phyto-072910-095423.
- Singh RP, Hodson DP, Jin Y, Huerta-Espino J, Kinyua MG, Wanyera R, Njau P, Ward RW. 2006. Current status, likely migration and strategies to mitigate the threat to wheat production from race Ug99 (TTKS) of stem rust pathogen. CAB Reviews. [accesed 2021 April]. https://www.cabi.org/cabreviews/review/20073043169.
- Singh RP, Hodson DP, Jin Y, Lagudah ES, Ayliffe MA, Bhavani S, Rouse MN, Pretorius ZA, Szabo LJ, Huerta-Espino J, et al. 2015. Emergence and spread of new races of wheat stem rust fungus: continued threat to food security and prospects of genetic control. Phytopathology. 105(7):872–884. doi:https://doi.org/10.1094/PHYTO-01-15-0030-FI
- Stakman E, Stewart D, Loegering W. 1962. Identification of physiologic races of Puccinia graminis var. tritici. Washington (DC): USDA Agricultural Research Service E617; p. 53.
- Terefe T, Pretorius ZA, Visser B, Boshoff WHP. 2019. First report of Puccinia graminis f. sp. tritici race PTKSK, a variant of wheat stem rust race Ug99, in South Africa. Plant Dis. 103(6):1421. doi:https://doi.org/10.1094/PDIS-11-18-1911-PDN
- The T, Latter B, McIntosh R, Ellison F, Brennan P, Fisher J, Hollamby G, Rathjen A, Wilson R. 1988. Grain yields of near isogenic lines with added genes for stem rust resistance. In: Miller TE, Koebner RMD, editors. Proceedings of the seventh international wheat genetics symposium. Cambridge (UK): Institute of Plant Science Research; p. 901–906.
- The TT. 1973. Chromosome location of genes conditioning stem rust resistance transferred from diploid to hexaploid wheat. Nat New Biol. 241(112):256. doi:https://doi.org/10.1038/newbio241256a0
- Tsilo TJ, Jin Y, Anderson JA. 2008. Diagnostic microsatellite markers for the detection of stem rust resistance gene Sr36 in diverse genetic backgrounds of wheat. Crop Sci. 48(1):253–261. doi:https://doi.org/10.2135/cropsci2007.04.0204
- Van Ooijen J 2009. MapQTL® 6: software for the mapping of quantitative trait loci in experimental populations of diploid species. Kyazma BV. Wageningen (The Netherlands); p. 59. [accessed 2020 August 2020]. https://www.kyazma.nl/docs/MQ6Manual.pdf.
- Visser B, Herselman L, Pretorius ZA. 2009. Genetic comparison of Ug99 with selected South African races of Puccinia graminis f.sp. tritici. Mol Plant Pathol. 10(2):213–222. doi:https://doi.org/10.1111/j.1364-3703.2008.00525.x
- Voorrips RE. 2002. MapChart: software for the graphical presentation of linkage maps and QTLs. J Hered. 93:77–78. doi:https://doi.org/10.1093/jhered/93.1.77.
- Wang S, Wong D, Forrest K, Allen A, Chao S, Huang BE, Maccaferri M, Salvi S, Milner SG, Cattivelli L, et al. 2014. Characterization of polyploid wheat genomic diversity using a high-density 90 000 single nucleotide polymorphism array. Plant Biotechnol J. 12(6):787–796. doi:https://doi.org/10.1111/pbi.12183
- Watson IA, Luig NH. 1966. SR 15 - A new gene for use in the classification of Puccinia graminis var. tritici. Euphytica. 15(2):239–247. doi:https://doi.org/10.1007/BF00022329
- Wu S, Pumphrey M, Bai G. 2009. Molecular mapping of stem-rust-resistance gene Sr40 in wheat. Crop Sci. 49(5):1681–1686. doi:https://doi.org/10.2135/cropsci2008.11.0666
- Yu LX, Barbier H, Rouse MN, Singh S, Singh RP, Bhavani S, Huerta-Espino J, Sorrells ME. 2014. A consensus map for Ug99 stem rust resistance loci in wheat. Theor Appl Genet. 127(7):1561–1581. doi:https://doi.org/10.1007/s00122-014-2326-7