Abstract
Bacterial spot (BST) is a highly impactful disease of open-field tomatoes produced in the U.S. Midwest. We combined BST survey data from 2010 to 2013 and 2017 to 2020 from midwestern states to characterize BST-causing Xanthomonas species associated with processing tomatoes. We identified 1009 Xanthomonas spp. strains and characterized their temporal/geographical distribution, bactericide resistance, and bacteriocin production. This work highlights a notable shift in species associated with BST in the study region from X. hortorum pv. gardneri to X. perforans over the course of the surveys. While the frequency of X. perforans associated with BST increased overall, some regions remained dominated by X. hortorum pv. gardneri, possibly due to the impact of seed or transplant source or local environment. No significant association was found between fruit maturity and Xanthomonas species isolated, suggesting no impact on the observed species shift. While in vitro screening demonstrated an increase in copper resistance for all Xanthomonads over time, and to a lesser degree streptomycin, no significant association with resistance and Xanthomonas species was identified, suggesting no association with the observed population shift. Finally, while nearly half of all X. perforans strains isolated produced bacteriocins, these antimicrobial compounds were found to have no impact on X. hortorum pv. gardneri strains and are not likely responsible for the observed species shift. However, the rise in bacteriocin-producing X. perforans strains may be linked to the disappearance of X. euvesicatoria in Midwest processing tomato production, although this will require further investigation.
Résumé
La tache bactérienne (TB) est une très grave maladie qui s’attaque aux tomates cultivées en champ dans le Midwest des États-Unis. Nous avons combiné les données d’études de 2010-2013 et de 2017-2020 provenant d’États du Midwest pour caractériser les espèces de Xanthomonas causant la TB chez la tomate de transformation. Nous avons identifié 1 009 souches de Xanthomonas spp. et caractérisé leur répartition temporelle et géographique, leur résistance aux bactéricides et leur production de bactériocines. Ces travaux mettent en lumière, au fil des études, une importante variation des espèces associées à la TB dans la région à l’étude, de X. hortorum pv. gardneri à X. perforans. Tandis que la fréquence de X. perforans associé à la TB s’est généralement accrue, certaines régions demeurent dominées par X. hortorum pv. gardneri, probablement à cause de la provenance des semences ou des plants à repiquer ou des conditions locales. Aucun lien majeur n’a été établi entre la maturité des fruits et les espèces de Xanthomonas isolées, ce qui suggère que cela n’a aucune répercussion sur la variation observée des espèces. Tandis que le criblage in vitro a affiché un accroissement de la résistance au cuivre chez toutes les xanthomonades au fil du temps, et à un degré moindre à la streptomycine, aucune association d’importance entre la résistance et les espèces de Xanthomonas n’a été établie, ce qui suggère que cela n’agit pas sur la variation de populations observée. Finalement, tandis que près de la moitié de toutes les souches de X. perforans isolées a produit des bactériocines, on a constaté que ces composés antimicrobiens n’exerçaient aucune influence sur les souches de X. hortorum pv. gardneri et qu’ils n’étaient vraisemblablement pas responsables de la variation des espèces observée. Toutefois, l’augmentation des souches de X. perforans produisant des bactériocines peut être liée à la disparition de X. euvesicatoria dans la production de tomates de transformation dans le Midwest, bien que cela nécessiterait des recherches supplémentaires pour le confirmer.
Introduction
Each year, bacterial spot of tomato (BST) adversely affects tomato production dramatically in the United States (U.S.). In 2010, BST outbreaks caused an estimated loss of $8 million to the processing tomato industry in the U.S. Midwest (USDA Citation2016). Bacterial spot outbreaks in different geographical regions have been associated predominantly with one or more of four Xanthomonas species: X. vesicatoria in Mexico and Italy (Bouzar et al. Citation1996; Zaccardelli et al. Citation2011), X. hortorum pv. gardneri in the United States (Pennsylvania), Canada, and Brazil (Quezado-Duval et al. Citation2004; Cuppels et al. Citation2006; Kim et al. Citation2010), and X. euvesicatoria in the Southwest Indian Ocean region (Hamza et al. Citation2010). Moreover, changes in Xanthomonas species predominance have been reported in Florida (Jones et al. Citation1998), where the most frequently isolated species progressed from X. euvesicatoria to X. perforans (Timilsina et al. Citation2019), and in Ohio, where X. vesicatoria was largely replaced by X. hortorum pv. gardneri and to a lesser extent X. perforans between the late 1990s and 2010 (Sahin Citation1997; Ma et al. Citation2011). More recently, surveys in North Carolina and Indiana suggest that X. perforans race T4 is now the dominant species followed by X. hortorum pv. gardneri and X. euvesicatoria (Egel et al. Citation2018; Adhikari et al. Citation2019). Shifts in species of Xanthomonas could be due to the production of bacteriocins. Bacteriocins are antimicrobial peptides with antagonistic activity against non-bacteriocin-producing members of the same or closely related species (Vidaver Citation1983). Bacteriocin-producing strains have been reported among different bacterial genera (James et al. Citation2002; Chuang et al. Citation2007; Hockett et al. Citation2015), including Xanthomonas spp. (Fett et al. Citation1987; Tudor-Nelson et al. Citation2003; Marutani-Hert et al. Citation2020). Tudor (Citation1999) and Tudor-Nelson et al. (Citation2003) attributed the displacement of X. euvesicatoria populations by X. perforans to the antagonistic activity of three bacteriocins. In 2020, Marutani-Hert et al. characterized the genetic determinants of these three bacteriocins and described one as belonging in the RHS family of toxins and the other two as proteases.
The management of BST focuses on preventive exclusion of the pathogen utilizing certified pathogen-free seeds and transplants, good sanitation protocols and crop rotation. Some processing tomato transplant producers apply streptomycin to seedlings in the greenhouse (Kucharek Citation1994), alone or alternated with copper-based bactericides (Conover and Gerhold Citation1981). Copper-based bactericides are also widely used in the field, although their efficacy may be limited by an increasing number of copper-resistant populations within Xanthomonas species (Thayer and Stall Citation1962; Marco Citation1983; Abbasi and Weselowski Citation2015; Strayer-Scherer et al. Citation2019; Khanal et al. Citation2020).
We combined early survey data (2010–2013) (Ma Citation2015) with more extensive sampling carried out recently (2017–2020) to address the temporal-spatial distribution of Xanthomonas species associated with processing tomatoes since a major BST outbreak in 2010. We characterized 1009 Xanthomonas spp. strains. Specifically, the goals of this work were to evaluate: (1) the distribution of Xanthomonas spp. in processing tomatoes over time and across geographical regions, (2) the associations of Xanthomonas species among seed sources and production areas, (3) copper sulphate and streptomycin resistance among Xanthomonas species causing bacterial spot in processing tomatoes in the Midwest, and (4) bacteriocin production among Midwest X. perforans strains.
Materials and methods
Sample collection
The survey included 8 years (2010–2013 and 2017–2020) of sampling in processing tomato fields affected by bacterial leaf spot located in different counties in Ohio, Indiana and Michigan (). During 2010–2013 a total of 79 processing tomato fields were surveyed (2010 (n = 30), 2011 (n = 23), 2012 (n = 16), 2013 (n = 10)). Fields were sampled on a W-shaped transect and symptomatic leaves and fruits were collected from different disease foci. In 2010 one pooled sample each of symptomatic leaves and fruits was collected from different disease foci in each field, from which one random subsample of leaves and fruit was selected for bacterial isolation. From 2011 to 2013, one to three fruit samples were collected from each field based on field size and disease incidence. For the years 2017–2020 symptomatic fruit samples were collected from a total of 41 fields (2017 (n = 14), 2018 (n = 16), 2019 (n = 6), 2020 (n = 5)) at two different stages of maturity (unripe (green) and ripe (red)).As tomato fruits are most susceptible to infection by Xanthomonas spp. when immature (Bernal Citation2020), lesions on ripe and green fruits were likely the result of infection events separated by 5 to 7 weeks. Fields were sampled on a W-shaped transect and symptomatic fruits were collected from each field based on field size, the number of varieties present, and disease incidence. The hierarchical sampling scheme was partitioned over tomato varieties, growers, fields and counties. In addition, 22 Xanthomonas strains collected from processing tomato between 1994 and 1996 (Sahin Citation1997) and presumptively identified as tomato race 2 (X. vesicatoria) were included in this study.
Xanthomonas isolation and molecular identification
Bacteria were isolated from single lesions. Plant tissue was surface sterilized for 30 seconds in 70% ethanol (V/V), followed by two rinses in sterile deionized water. The tissue was minced with a razor blade and covered with 100 µL of sterile water, allowing the bacteria to ooze from the necrotic tissue for 30 seconds. The bacterial suspension was streaked on yeast dextrose calcium carbonate (YDC) agar (Vidaver Citation1989) medium and incubated in darkness at 28°C. Yellow mucoid Xanthomonas-like colonies were purified and subjected to oxidase, catalase, and potassium hydroxide (KOH 3%) tests (Schaad et al. Citation2001). A total of 167 isolates were collected from processing tomato fields in Citation2010–2013, while 842 isolates were collected from 2017 to 2020.
Xanthomonas isolates collected from Citation2010 to 2013 and 2017 to 2018, as well a sample of strains collected in 1994–1996 were identified to species using BOX-A1R PCR (Louws et al. Citation1994) by comparing banding profiles to those of known reference strains: X. euvesicatoria 110C (Sahin et al. Citation2003), X. vesicatoria 791 (Lewis Ivey et al. Citation2010), X. perforans 1220 (tomato race 3, Lewis Ivey et al. Citation2010), X. perforans 4B and X. hortorum pv. gardneri XcgA2 (both provided by Dr. J. B. Jones, University of Florida). PCR assay reactions were carried out in a final volume of 25 µL containing: 12.5 µL of GoTaq Green Master Mix (Promega, Madison, WI), 1.25 µL of 10 µM primer (5’-TCCGGCAAGGCGACGCTGAC-3’), 9.25 µL nuclease-free PCR water, and 2 µL of template. Templates consisted of one single colony suspended in 200 µL of sterile water and subjected to two cycles of five minutes each of freezing at −80°C and thawing at 95°C. The BOX-PCR cycle consisted of an initial denaturation step at 95°C for 7 min followed by 30 cycles of 94°C for 1 min, 53°C for 1 min and 65°C for 8 min, with a final step at 65°C for 16 min. PCR products were subjected to electrophoresis on a Tris-acetate-EDTA buffer (TAE) 1.7% agarose gel, stained in ethidium bromide, and exposed to ultraviolet light on a benchtop transilluminator (UVP, Cambridge, UK). The size of the amplicons was estimated by comparing the DNA bands with a 1 Kb DNA Plus ladder (Invitrogen, Thermo Fisher Scientific, Inc., Waltham, MA). Smeared or weak bands were not considered for species identification; bands that were consistent and unambiguous were used for the comparison with those of the reference strains listed above.
All isolates were also tested by species-specific qPCR (Strayer et al. Citation2016) to confirm the outcome of the BOX-PCR assay. BOX-PCR and qPCR analyses were each done once. Congruence of the two identification methods was confirmed by applying the Mantel test using the software Past 4.0 (Hammer et al. Citation2001) on the species matrices.
Xanthomonas strain sensitivity to copper sulphate and streptomycin in in vitro assays
All strains collected were evaluated for copper sulphate and streptomycin sulphate sensitivity in in vitro assays. Single colonies selected from 2-day-old YDC plates were streaked on glucose nutrient agar (NA) (peptone 5 g L−1, beef extract 3 g L−1, agar 15 g L−1, 0.5% glucose) amended with copper sulphate (30 μg mL−1, 100 μg mL−1, or 200 μg mL−1) or streptomycin sulphate (20 μg mL−1 or 200 μg mL−1) (Ma Citation2015), and non-amended medium. Stock solutions of copper sulphate and streptomycin sulphate were prepared in sterile deionized water and passed through a 0.2 µm syringe filter (Merck KGaA, Darmstadt, Germany) and appropriate dilutions were added to the medium after autoclaving and cooling to 55°C. Bacterial growth was evaluated after 48 and 72 hours of incubation. For each strain isolated during the early survey (2010–2013), sensitivity was evaluated by comparing the growth of streaked cultures on amended and non-amended media. Strains for which bacterial growth was 50–100% of the growth on non-amended control medium were considered insensitive to the given chemical rate (Ma Citation2015). For each strain collected from 2017 to 2020, sensitivity was evaluated according to the method described in Wang et al. (Citation2017) with modifications. From each bacterial suspension (overnight ~2 x108 CFU mL−1, ODλ600 = 0.2) a working suspension of ~1 x 104 cells per mL was prepared and five separate droplets of 10 µL each were placed aseptically onto NA medium (Becton Dickson and Company, Maryland) amended with the fixed concentrations of copper sulphate and streptomycin reported above. Bacterial growth was evaluated after 48 hours of incubation at 28°C. The strains that exhibited transparent droplets (no growth) were considered sensitive for the assessed concentration. Assays were conducted twice.
Bacteriocin production
The production of bacteriocins by X. perforans strains and their inhibition of growth of X. euvesicatoria and X. hortorum pv. gardneri was assessed with deferred antagonism. The indicator kanamycin-resistant X. euvesicatoria strain (91–106 K) was kindly provided by Dr. Jeff Jones at the University of Florida. This strain was prepared via conjugation using the plasmid pRK2073 that served as a helper plasmid to introduce the target plasmid vector into the X. euvesicatoria rifampicin-resistant strain 91–106 R (Tudor-Nelson et al. Citation2003). The indicator X. hortorum pv. gardneri (SM775-12Xgb), both bioluminescent and kanamycin resistant, was obtained by transformation of X. hortorum pv. gardneri strain SM775-12 using the pUWGR4 plasmid and kanamycin-resistant gene (Rajashekara et al. Citation2005). The luxCDABE operon was introduced into the plasmid using an EZ::Tn5 system (Srivastava et al. Citation2021). This strain was utilized in this assay for its kanamycin resistance. The deferred-antagonism method was carried out following the methodology described in Klein et al. (Citation2020). Briefly, for each X. perforans strain, five microlitres of bacterial suspension (ODλ600 nm = 0.3) was spotted onto the centre of NA medium in 6 mm-diam Petri dishes then incubated for 48 hours at 28°C. Nutrient agar (0.6% w/V, 4 mL plate−1) was used as an overlay. Before overlaying, the top agar was amended with kanamycin (50 µg mL−1) and with 40 µL of bacterial suspension of one of the kanamycin-resistant indicator strains (ODλ600 nm = 0.3). Plates were incubated for 48 hours at 28°C, and then areas of bacterial growth inhibition were noted. Strains that produced an inhibition halo were scored as positive for bacteriocin production. The experiment was conducted twice.
Statistical analyses
The association between Xanthomonas species and variables of interest (geographical location, seed source, fruit maturity, and sensitivity to bactericidal products) was assessed through the Chi-Square statistic using the software JMP Pro (SAS Institute Inc., Cary, NC) and Minitab 18 (Minitab Inc., Chicago, IL). To evaluate changes in seed sources all the varieties sampled were grouped according to their origin (seed companies A trough G), then comparisons between and among varieties were carried out to evaluate the association with Xanthomonas species. The Chi-square test was also performed to investigate the relationship between fruit maturity and Xanthomonas strains. Similarly, the distribution of sensitivity to copper sulphate and streptomycin among the Xanthomonas species was evaluated using a Chi square test for association. Xanthomonas euvesicatoria was not included in the analyses due to the low number of strains recovered each year and for the year 2012 no analysis was performed because of the low number of isolations (fewer than five strains per species identified in this year).
Results
Xanthomonas species identification
A total of 1009 strains were collected during the surveys (). All strains were gram negative, catalase positive and oxidase negative. Colonies were yellow in colour and mucoid on YDC medium. Strains later identified in molecular tests as X. perforans were faster growing than those identified as X. hortorum pv. gardneri, and colonies were sticky in consistency after 48–72 hours of incubation at 28°C. The BOX-PCR assay produced reproducible banding patterns for each of the strains assessed (). The amplicons ranged in size from 150 bp to 2000 bp for a total of 14 polymorphic bands. The X. hortorum pv. gardneri banding profile consisted of six main amplicons with molecular weight ranging from 400 bp to 1800 bp. Xanthomonas perforans strains produced seven main amplicons (from 200 bp to 1900 bp), while the X. euvesicatoria profile consisted of five main amplicons (from 400 bp to 1400 bp). The profiles of 103 isolates with the X. perforans phenotype contained five main amplicons (from 400 bp to 1200 bp), four of which matched the X. perforans profile, while two (700 and 1400 bp) were shared with the X. hortorum pv. gardneri profile.
Table 1. Xanthomonas strains isolated from processing tomatoes in the U. S. Midwest, by species and geographical location, 2010–2020. Xanthomonas spp. identification is based on the qPCR outcome.
Fig. 2 BOX-PCR fingerprints of Xanthomonas spp. strains isolated from processing tomato in the U.S. Midwest. L) ladder 2 kb; 1 to 12) X. hortorum pv. gardneri; 13 and 14) putative X. perforans; 15 and 16) X. perforans; reference strains 17) X. hortorum pv. gardneri XcgA2; 18) X. euvesicatoria 110C; 19) X. perforans 1220.
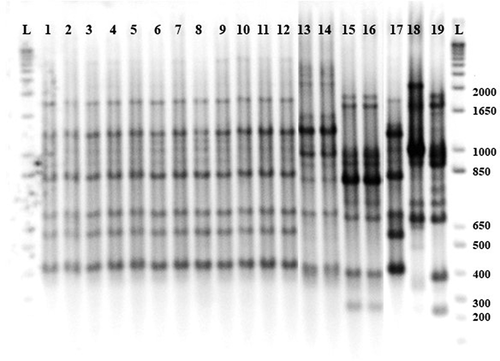
By comparison to known reference strains, the strains collected from 2010 to 2018 were genotyped as follows: X. euvesicatoria (n = 11), X. hortorum pv. gardneri (n = 339), X. perforans (n = 351), and putative X. perforans (n = 103). These 103 strains were confirmed as X. perforans by qPCR. The remaining strains (n = 906), as well as those collected in 2019 and in 2020, were identified by qPCR as follows: X. euvesicatoria (n = 13), X. hortorum pv. gardneri (n = 385), and X. perforans (n = 611) (). Species assignment based on the qPCR results did not significantly differ (Mantel Test, R = 0.999, p > 0.0001) from the BOX-PCR species identification. Final species assignment was based on qPCR results.
All four Xanthomonas species associated with the BST complex were identified among 22 putative race T2 strains (X. vesicatoria) collected from tomato in Ohio in 1994 and 1995 (11 strains each year) (Sahin Citation1997). Among the 22 strains, three were X. euvesicatoria, ten were X. vesicatoria, four were X. hortorum pv. gardneri, and two were X. perforans. The BOX-PCR profiles of the remaining three strains did not match those of any of the reference strains.
Temporal-spatial distribution of Xanthomonas species
A notable shift was observed in X species composition behind the outbreaks of BST in Northwest Ohio between 2010 and 2020 (, ). In 2010, 79% of isolates collected were characterized as X. hortorum pv. gardneri, 12% as X. euvesicatoria, and 11% as X. perforans. In 2017, 2018, and 2019 X. hortorum pv. gardneri comprised 51%, 29%, and 32% of the isolates relative to X. perforans, respectively. No isolates of X. euvesicatoria were recovered between 2017 and 2020. In 2020, the percentage of X. hortorum pv. gardneri isolates increased to 68%. Xanthomonas hortorum pv. gardneri strains were isolated from tomatoes only in Ottawa County (Ohio), which throughout the survey years yielded mostly X. hortorum pv. gardneri: 83% in 2010, 84% in 2017, and 73% in 2018. During the same time frame, recovery of X. perforans from Ottawa County increased from 6% in 2010 to 16% in 2017 and 27% in 2018. In Putnam County, the percentage of X. hortorum pv. gardneri strains recovered decreased from 71% in 2011 to 54% in 2012 and 0% in 2017–2019, while X. perforans isolations increased proportionally each year. Southeast Michigan was only sampled in 2010, with the majority (69%) of the 19 strains isolated identified as X. hortorum pv. gardneri and 21% and 11% identified as X. perforans and X. euvesicatoria, respectively. Central Indiana, first sampled in 2017, was dominated by X. perforans (99%).
Fig. 3 Xanthomonas species distribution (percent) in processing tomatoes in Ohio, Indiana and Michigan, 2010–2020. Data for years 2012 and 2013 are not reported in the figure because of their small sample size, and no sampling was done in 2014–2016.
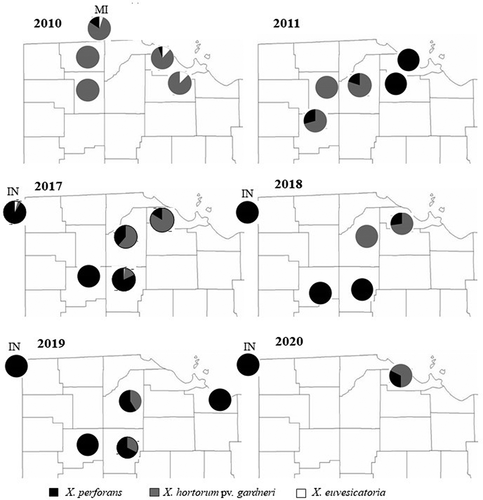
Xanthomonas spp. were isolated from fruits from plants across six different seed company sources, however, only fruits from seed company A (A) and seed company B (B) varieties were sampled every year of the survey. In 2010, X. hortorum pv. gardneri was significantly associated with B varieties (χ2 test of association, χ2 = 34.57, p < 0.0001) (). In 2011, only X. hortorum pv. gardneri was isolated from B varieties. Equal numbers of X. hortorum pv. gardneri and X. perforans strains were isolated from A varieties. There were no significant associations between Xanthomonas species isolated and seed source in 2012 and 2013. By 2017, the incidence of X. perforans was significantly greater than would be expected by chance in the B varieties (χ2 test of association, χ2 = 3.43, p = 0.03). Xanthomonas perforans was the only species isolated from A varieties. In 2018, both seed sources were dominated by X. perforans (χ2 test of association, χ2 = 17.8 and 67.9, p < 0.0001 and <0.0001 for A and B, respectively). In 2019 X. perforans was the only species found on B varieties. A similar number of X. perforans and X. hortorum pv. gardneri strains were isolated from A varieties. In 2020, only X. perforans was isolated from A varieties. Xanthomonas perforans and X. hortorum pv. gardneri were recovered from B varieties in similar numbers. Among other seed sources, company C varieties yielded very few Xanthomonas strains. In 2017, Seed company D varieties were dominated by X. hortorum pv. gardneri. However, in 2019 X. perforans was observed more frequently on varieties from the D source. Seed company E varieties, first sampled in 2011, were originally preferentially associated with X. hortorum pv. gardneri (χ2 test of association, χ 2 = 6.23, p = 0.013). The association shifted in 2017 to equal numbers of X. perforans and X. hortorum pv. gardneri strains. By 2018, the dominance shifted to X. perforans. A similar shift from X. hortorum pv. gardneri to X. perforans was seen between 2019 and 2020. Seed company F varieties had no significant association with either species when surveyed in 2017 (χ2 test of association, χ 2 = 0.86, p = 0.35), but in 2018 and 2020 X. perforans was the only species isolated from F varieties. Seed company G varieties were sampled only in 2018 and were associated with X. perforans (χ2 test of association, χ 2 = 12.5, p < 0.0001).
Table 2. Association between Xanthomonas species isolated from processing tomatoes in the U.S. Midwest and tomato seed sources.
Among isolates recovered from Northwest Ohio and Central Indiana, there was no significant association of one Xanthomonas species with fruit stage in 2017; both species were distributed similarly among ripe and unripe fruits (). However, in 2018 and 2020, unripe fruits were associated with X. perforans (χ 2 test of association, χ 2 = 49.78 and 18.96, p < 0.0001 for 2018 and 2020, respectively) (). Xanthomonas perforans was also associated with ripe tomatoes in 2018 and 2019 (χ 2 test of association, χ 2 = 46.22 and 25.27, respectively, p < 0.0001).
Table 3. Association of Xanthomonas hortorum pv. gardneri and X. perforans with green and ripe processing tomato fruit, 2017–2020.
Xanthomonas spp. sensitivity to copper sulphate and streptomycin sulphate
All 1009 of the Xanthomonas spp. strains were resistant to 30 µg mL−1 copper sulphate, the lowest concentration tested (data not shown). Except for strains isolated in 2010–2012, all strains tested were also resistant to 100 µg mL−1 copper sulphate (). In 2010, 83% of X. euvesicatoria, 65% of X. hortorum pv. gardneri and 100% of X. perforans strains were resistant to 100 µg mL−1 copper sulphate. In 2011 and 2012, 39% and 87% of X. hortorum pv. gardneri and 93% and 87% of X. perforans strains, respectively, were resistant. The number of X. hortorum pv. gardneri strains resistant to 100 µg mL−1 copper sulphate in 2011 resulted in a significant association between species and resistance for that year (χ2 test of association, χ2 = 12.6, p = 0.004) (). In 2010 none of the 81 strains tested were resistant to 200 µg mL−1 copper sulphate, the highest concentration evaluated (). In 2017 and 2018, over 50% of all strains tested, regardless of species, were resistant to this concentration of copper sulphate. The proportion of Xanthomonas strains resistant to the highest dosage of copper sulphate continued to increase through 2020, when all tested strains exhibited resistance. In 2019 only 20% of X. hortorum pv. gardneri strains (N = 25) collected were resistant to 200 µg mL−1 copper sulphate, leading to a significant association among pathogen species and copper resistance. While there was a notable increase in copper resistance over time, there was no consistent association of higher resistance with one species or the other.
Table 4. Percentage of copper sulphate- and streptomycin sulphate-resistant Xanthomonas hortorum pv. gardneri and X. perforans strains isolated from processing tomatoes in the U.S. Midwest, 2010–2020.
Fig. 4 Mosaic charts showing the proportion of Xanthomonas spp. strains from processing tomatoes in the U.S. Midwest resistant to copper sulphate (200 µg mL−1) for each surveyed year. Resistance is indicated in black, sensitivity in white. The width of each column is proportional to the relative abundance of each species.
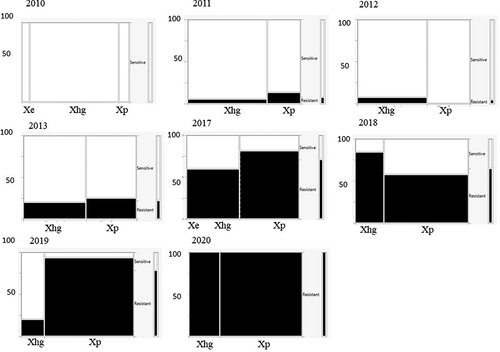
In 2010, 86% of X. hortorum pv. gardneri strains were resistant to both 20 and 200 µg mL−1 streptomycin sulphate, resulting in a significant association of resistance with this species (χ2 test of association, χ2 = 22.761 and 22.761, respectively, p < 0.0001) (). No significant association was observed in 2011, when less than 31% of the strains isolated were resistant to streptomycin. Resistance to 20 µg mL−1 streptomycin sulphate increased from 66% (X. hortorum pv. gardneri) and 80% (X. perforans) in 2017 to 100% of all strains in 2019 and 2020. For the highest concentration of streptomycin, the level of resistance and significant association of species with resistance varied among years (). In 2017 and 2018, a higher percentage of X. perforans strains were resistant to streptomycin than X. hortorum pv. gardneri strains (p < 0.0001), while in 2019 and 2020, a significantly higher percentage of X. hortorum pv. gardneri than X. perforans strains were resistant (p = 0.006 and p < 0.0001, respectively) ().
Fig. 5 Mosaic charts showing the proportion of Xanthomonas spp. strains from processing tomatoes in the U.S. Midwest resistant to streptomycin sulphate (200 µg mL−1) for each surveyed year. Resistance is indicated in black, sensitivity in white. The width of each column is proportional to the relative abundance of each species.
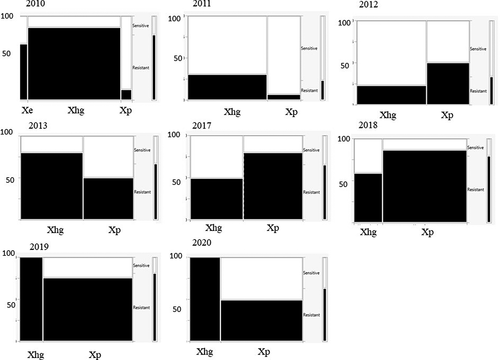
Bacteriocin production by Xanthomonas species
Inhibition of bacterial growth was only observed in dual cultures by X. perforans strains against the X. euvesicatoria indicator strain Xe 91–106. None of the X. perforans strains inhibited the growth of X. hortorum pv. gardneri SM775-12 Xgb. The percentage of bacteriocin-producing X. perforans strains collected between 2010 and 2020 that inhibited growth of X. euvesicatoria Xe 91–106 varied, ranging from 45% of 2017 strains to 66% of 2020 strains ().
Table 5. Percentage of bacteriocin-producing Xanthomonas perforans strains isolated from processing tomatoes in the U.S. Midwest, 2010–2020 that inhibited the growth of X. euvesicatoria 91–106 K.
Discussion
Our study aimed to characterize the Xanthomonas species distribution associated with bacterial spot in processing tomatoes in Ohio, Michigan and Indiana. In this study we compared data from a survey conducted from 2010 to 2013 (Ma Citation2015) to a more extensive survey performed from 2017 to 2020, as well as re-classified historical data from 1994 to 1996 (Sahin Citation1997). Two molecular methods were used to identify strains to species – qPCR and fingerprinting through BOX-PCR, which led to nearly identical results. This work demonstrates, in agreement with results reported for the Xanthomonas citri pathosystem (Fonseca et al. Citation2019), that different genetic approaches are suitable as diagnostic tools. Both BOX-PCR fingerprinting and species-specific qPCR were reliable for discriminating Xanthomonas species associated with tomato production. BOX-PCR is a more economical method for Xanthomonas species identification compared to the much quicker qPCR and has the advantage of visualizing strain diversity. We found 103 strains with a colony phenotype and qPCR identity of X. perforans, exhibiting a BOX-PCR profile containing elements of both X. perforans and X. hortorum pv. gardneri. Newberry et al. (Citation2019); (Citation2020)) highlighted the exchange of genetic material between X. hortorum pv. gardneri and X. perforans. The shared amplicons might represent the same plasmid identified in these previous studies (Newberry et al. Citation2019, Citation2020).
Surveying bacterial populations in different geographical locations is an important step in understanding how Xanthomonas species composition changes over time. Early surveys of BST in Ohio (1994–1996; Sahin Citation1997) identified 94 X. campestris pv. vesicatoria strains, of which 45% were tomato race 1, now associated with X. euvesicatoria; 49% were tomato race 2, associated with X. vesicatoria and X. hortorum pv. gardneri; and 6% were tomato race 3, now associated with X. perforans. In a sample of 22 putative race T2 strains from that survey that were re-characterized using the more specific molecular techniques utilized in this study, four strains were identified as X. hortorum pv. gardneri and two were X. perforans, indicating the presence of these species in Ohio by the mid-1990s. In our surveys in Northwest Ohio of processing tomatoes between 2010 and 2020, X. vesicatoria was never detected and X. euvesicatoria was rarely found, while X. perforans increased from a minor component of the BST Xanthomonas population in 2010 to predominating in some counties 7–10 years later. Surveys in two counties in Central Indiana between 2017 and 2020 also recovered nearly exclusively X. perforans. These results parallel observations in Ontario (Canada), Indiana and Illinois (Abbasi and Weselowski Citation2015; Egel et al. Citation2018; Khanal et al. Citation2020). Abbasi and Weselowski (Citation2015) documented a significant shift in Xanthomonas species from tomatoes in Ontario, from predominantly X. hortorum pv. gardneri in the late 1990s/early 2000s (Cuppels et al. Citation2006) to predominantly X. perforans in 2012. Among the 49 Xanthomonas strains collected in Indiana in 2016 and 2017, the majority (78%) were X. perforans (Egel et al. Citation2018). Ten strains were X. hortorum pv. gardneri, all recovered from the northern half of the state, north of interstate Highway I70. Both Indiana counties surveyed in this study were also north of I71. All counties surveyed in Northwest Ohio were north of I71, including Putnam County, from which only X. perforans was recovered from processing tomato fruit. The proportion of strains identified as X. hortorum pv. gardneri was generally higher than X. perforans in Ottawa and Wood counties, the two northernmost Ohio counties sampled between 2017 and 2020. Khanal et al. (Citation2020) surveyed fresh market tomatoes in Illinois between 2017 and 2019, recovering only X. hortorum pv. gardneri and X. perforans. The majority (86%) of the strains from the northern part of the state were X. hortorum pv. gardneri, while X. perforans strains predominated in the central (55%) and southern (73%) counties.
Xanthomonads surviving in transplant facilities could also explain the observed patterns of distribution. Processing tomato growers in different counties source seedlings from the same regional transplant houses (Jeff Unverferth, Hirzel Farms and Canning, personal communication). This species distribution could also be because X. hortorum pv. gardneri is more acclimated to cooler temperatures than other Xanthomonas species infecting tomatoes (Araujo et al. Citation2010; Potnis Citation2021), although other factors may also be relevant given the presence of both species in numerous locations, even within fields. The preference for cooler temperatures by X. hortorum pv. gardneri may be associated with specific effectors. Next generation whole genome sequencing has shown that X. hortorum pv. gardneri shares a suite of Type III effectors possessed by Pseudomonas syringae, another phytobacterium favoured by cool climates (Potnis et al. Citation2011). These effectors have not been observed in other Xanthomonas spp. causing BST (Potnis et al. Citation2015). Phylogenetic analyses based on whole genome and pathogenicity clusters showed that X. hortorum pv. gardneri is more closely related to X. campestris pv. campestris than to the other three Xanthomonas species associated with BST (Potnis et al. Citation2011). Xanthomonas hortorum pv. gardneri strains that are distributed globally are highly homogeneous regardless of the time and location of isolation (Timilsina et al. Citation2015). Khanal et al. (Citation2020) suggested endemic spread of both species after finding through Multilocus Sequence Analysis (MLSA) of six housekeeping genes that all Illinois X. hortorum pv. gardneri strains isolated in their surveys from fresh market tomatoes were identical to each other and to two reference strains. Strains of X. perforans fell into two geographically isolated groups identical to two Florida reference strains.
The data from this study moderately supports seed transmission as the main driver in the distribution of Xanthomonas species on seed sources. There is strong evidence that bacterial pathogens can be transmitted and survive on seed for many months (Gardner and Kendrick Citation1921; Mtui et al. Citation2010). However, in our study the results varied from year to year. For example, in 2017 except for varieties from seed company B, there was no association between seed sources and the ratios of Xanthomonas species, but in 2018 all varieties yielded a greater number of X. perforans than X. hortorum pv. gardneri strains. We found anecdotal evidence of overwintering for maintaining residual populations of X. hortorum pv. gardneri in Northwest Ohio. Overwintering of Xanthomonas species that infect tomato has been reported (Gardner and Kendrick Citation1921; Peterson Citation1963; Jones et al. Citation1986). Volunteer tomato plants with bacterial spot symptoms caused by X. perforans were observed during the survey in 2020 (data not shown). Bacterial spot was present in the previous tomato crop, suggesting that the bacteria overwintered in debris or seed in the field (Gardner and Kendrick Citation1921). Ma (Citation2015) did not observe overwintering of X. hortorum pv. gardneri on infected tomato plant residue and seeds in Northwest Ohio after 6 or 12 months. However, bacterial survival in the soil has been reported in association with the living rhizosphere of host and nonhost plants (Diachun and Valleau Citation1946; Coyne and Schuster Citation1974; Leben Citation1981; Bashan et al. Citation1982). Soil temperatures have also been reported to play a key role in the survival of Xanthomonas species associated with BST (Jones et al. Citation1986).
There was no significant shift in Xanthomonas species recovered from green to ripe tomato fruit in any of the four years where fruit stage was tracked. In the years 2018, 2019 and 2020 X. perforans was significantly associated with infected fruits regardless of the maturity stage (green or ripe), suggesting the association had more to do with the overall shift in the prevalence of X. perforans (). Similarly, these results do not support a temporal effect on Xanthamonas species recovered from the fruits. Previous findings suggest that small fruits under 1.5 cm are most vulnerable to lesion development (Gardner and Kendrick Citation1923; Getz Citation1983). At the time of sample collection, when both green and ripe fruits were harvested at the same time, it is assumed that the lesions on the mature, ripe fruits would be older than those on the younger green fruits. Therefore, Xanthomonas species infecting early in the season would be responsible for lesions observed in ripe fruit at the time of harvest, while lesions on green fruit harvested at the same time would be the result of later infection events. It is unlikely that ripe fruit lesions would be caused by infection late in the season.
The analysis of bactericide resistance among isolated BST pathogens revealed a marked increase in resistance to copper and, to a lesser degree, streptomycin through the course of the survey, as well as a striking increase from 1994 to 1996, when only 11% and 13% of Xanthomonas strains from tomato were resistant to 100 µg mL−1 copper sulphate and streptomycin sulphate, respectively, and none were resistant to 150 µg mL−1 of either bactericide (Sahin Citation1997). However, a consistent association of resistance in one species over another was not observed. Therefore, bactericide resistance is likely not a significant driver in Xanthomonas species abundance in this system. It is likely then that bactericide resistance in Xanthomonas populations causing BST is related to other factors, such as a founder effect in individual locations. This would help to explain the inconsistent rise in streptomycin resistance compared to copper resistance, as application of streptomycin is limited to seedlings in greenhouses and relatively uncommon in the Midwest. If endemic Xanthomonas populations exist in the field they would not be subject to this selection pressure. On the other hand, copper-based bactericides are used commonly in both the greenhouse (seedlings) and field, applying selection pressure over time. In addition, the use of copper bactericides and streptomycin for BST management in processing tomato seed production, most of which occurs in Asia, may contribute to the introduction of bactericide-resistant Xanthomonas populations to the U.S. Midwest on seeds. Bacteriocin production was also hypothesized to be a factor underlying shifts in Xanthomonas species causing BST. The bacteriocins produced by X. perforans have been shown to give it a selective advantage over X. euvesicatoria in other geographic regions leading to a shift in species dominance (Hert et al. Citation2005). While approximately 50% of all X. perforans strains isolated during each year of this study produced bacteriocins in an assay versus a test strain of X. euvesicatoria, these same strains did not inhibit the growth of the test strain of X. hortorum pv. gardneri. Data from this study are insufficient to definitively link the nearly complete disappearance of X. euvesicatoria from Midwest processing tomatoes to the rise of X. perforans over the last decade. Interestingly, X. euvesicatoria remains the predominant species causing bacterial spot in Ohio peppers, even in the presence of minor populations of X. perforans (unpublished data). Additional factors likely contribute to the increase in X. perforans populations linked to BST relative to X. hortorum pv. gardneri populations observed over the past 25 years in Ohio. Turnover in BST Xanthomonas populations can occur at the level of race as well, despite the lack of BST-resistant varieties (Hert et al. Citation2005). The current study was limited to evaluating species changes over time in the surveyed regions, however, additional work characterizing the pathogens at the level of race may yield insights into the mechanisms underlying the shift in Xanthomonas species populations causing BST over time.
Acknowledgements
Authors thank Dr. J. Jones and Dr. J. Klein-Gordon, University of Florida, for sharing the X. euvesicatoria indicator strain used in the bacteriocin assay. The authors thank Dr. G. Rajashekara and Dr. L. Deblais from The Ohio State University for sharing the X. gardneri pv. hortorum indicator strain used in the bacteriocin assay.
Disclosure statement
No potential conflict of interest was reported by the author(s).
Additional information
Funding
References
- Abbasi PA, Weselowski B. 2015. Efficacy of Bacillus subtilis QST 713 formulations, copper hydroxide, and their tank mixes on bacterial spot of tomato. Crop Prot. 74:70–76. doi:10.1016/j.cropro.2015.04.009
- Adhikari P, Adhikari TB, Timilsina S, Meadows I, Jones JB, Panthee DR, Louws FJ. 2019. phenotypic and genetic diversity of Xanthomonas perforans populations from tomato in North Carolina. Phytopathology. 109(9):1533–1543. doi:10.1094/PHYTO-01-19-0019-R.
- Araújo ER, Pereira RC, Moita AW, Ferreira MASV, Café-Fiho AC, Quezado-Duval AM 2010. Effect of temperature on pathogenicity components of tomato bacterial spot and competition between Xanthomonas perforans and X. gardneri. III International Symposium on Tomato Diseases. Acta Hort. 914:39–42.
- Bashan Y, Diab S, Okon Y. 1982. Survival ofXanthomonas campestris pv.vesictoria in pepper seeds and roots in symptomless and dry leaves in non-host plants and in the soil. Plant Soil. 68(2):161–170. doi:10.1007/BF02373702.
- Bernal E, Liabeuf D, Francis DM 2020. Evaluating Quantitative Trait Locus.
- Bouzar H, Jones JB, Somodi GC, Stall RE, Daouzli N, Lambe RC, Gastelum RF, Correa RT. 1996. Diversity of Xanthomonas campestris pv. vesicatoria in tomato and pepper fields of Mexico. Can J Plant Pathol. 18(1):75–77. doi:10.1080/07060669609500659.
- Chuang D, Chien Y, Wu H-P. 2007. Cloning and Expression of the Erwinia carotovora subsp. carotovora Gene Encoding the Low-Molecular-Weight Bacteriocin Carocin S1. J Bacteriol. 189(2):620–626. doi:10.1128/jb.01090-06.
- Conover RA, Gerhold NR. 1981. Mixtures of copper and maneb or mancozeb for control of bacterial spot of tomato and their compatibility for control of fungus diseases .Vol 94. Florida State Horticultural Society, Proceedings; pp.154–156.
- Coyne DP, Schuster MLA. 1974. Morphological and physiological component genetic approach to breeding for tolerance to the bacterial pathogen’s, Pseudomonas phaseolicola and Xanthomonas phaseoli. Reports of Bean Improvement Cooperative and National Dry Bean Council Research Conference. pp. 23–24.
- Cuppels DA, Louws FJ, Ainsworth T. 2006. Development and evaluation of PCR-based diagnostic assays for the bacterial speck and bacterial spot pathogens of tomato. Plant Dis. 90(4):451–458. doi:10.1094/PD-90-0451.
- Diachun S, Valleau WD. 1946. Growth and overwintering of Xanthomonas vesicatoria in association with wheat roots. Phytopathology. 36:277–280.
- Egel DS, Jones JB, Minsavage GV, Creswell T, Ruhl G, Maynard E, Marchino C. 2018. Distribution and Characterization of Xanthomonas Strains Causing Bacterial Spot of Tomato in Indiana. Plant Health Progress. 19(4):319–321. doi:10.1094/PHP-07-18-0041-BR.
- Fett WF, Dunn MF, Maher GT, et al. 1987. Bacteriocins and temperate phage of Xanthomonas campestris pv. glycines Curr. Microbiol. 16:137–144. doi:10.1007/BF01568392
- Fonseca NP, Patané JSL, Varani AM, Felestrino ÉB, Caneschi WL, Sanchez AB, Cordeiro IF, de C LCG, de Ab AR, Garcia CCM, et al. 2019. Analyses of seven new genomes of Xanthomonas citri pv. aurantifolii strains, causative agents of citrus canker b and c, show a reduced repertoire of pathogenicity-related genes. Front Microbiol. 10:2361. doi:10.3389/fmicb.2019.02361
- Gardner MW, Kendrick JB. 1921. Bacterial spot of tomato. J Agric Res. 21:123–156.
- Gardner MW, Kendrick JB 1923. Bacterial spot of tomato and pepper. Phytopathology. 13:307–315.
- Getz S. 1983. Influence of Developmental Stage on Susceptibility of Tomato Fruit to Pseudomonas syringae pv. tomato. Tomato. Phytopathology. 73(1):36. doi:10.1094/Phyto-73-36.
- Hammer Ø, Harper DAT, Ryan PD. 2001. PAST: paleontological Statistics Software Package for Education and Data Analysis. Palaeontologia Electronica. 4(1):178. http://palaeo-electronica.org/2001_1/past/issue1_01.htm.
- Hamza AA, Robène-Soustrade I, Jouen E, Gagnevin L, Lefeuvre P, Chiroleu F, Pruvost O. 2010. Genetic and Pathological Diversity Among Xanthomonas Strains Responsible for Bacterial Spot on Tomato and Pepper in the Southwest Indian Ocean Region. Plant Dis. 94(8):993–999. doi:10.1094/PDIS-94-8-0993.
- Hert AP, Roberts PD, Momol MT, Minsavage GV, Tudor-Nelson SM, Jones JB. 2005. Relative Importance of Bacteriocin-Like Genes in Antagonism of Xanthomonas perforans Tomato Race 3 to Xanthomonas euvesicatoria Tomato Race 1 Strains. Appl Environ Microbiol. 71(7):3581–3588. doi:10.1128/AEM.71.7.3581-3588.2005.
- Hockett KL, Renner T, Baltrus DA, Lindow SE. 2015. Independent Co-Option of a Tailed Bacteriophage into a Killing Complex in Pseudomonas. mBio. 6(4):e00452. doi:10.1128/mBio.00452-15.
- James R, Penfold CN, Moore GR, Kleanthous C. 2002. Killing of E. coli cells by E group nuclease colicins. Biochimie. 84(5–6):381–389. doi:10.1016/s0300-9084(02)01450-5.
- Jones J, Bouzar H, Somodi G, Stall R, Pernezny K, El-Morsy G, Scott, W. 1998. Evidence for the preemptive nature of tomato race 3 of Xanthomonas campestris pv. Vesicatoria in Florida Phytopathology. 88:33–38. doi:10.1094/PHYTO.1998.88.1.33.
- Jones JB, Pohronezny KL, Stall RE, Jones JP. 1986. Survival of Xanthomonas campestris pv. vesicatoria in Florida on tomato crop residue, weeds, seeds, and volunteer tomato plants. Phytopathology. 76(4):430–434. doi:10.1094/Phyto-76-430.
- Khanal S, Hind SR, Babadoost M. 2020. Occurrence of copper-resistant Xanthomonas perforans and X. gardneri in Illinois tomato fields. Plant Health Prog. 21(4):338–344. doi:10.1094/PHP-06-20-0048-RS.
- Kim SH, Olson TN, Peffer ND, Nikolaeva EV, Park S, Kang S. 2010. First Report of Bacterial Spot of Tomato Caused by Xanthomonas gardneri in Pennsylvania. Plant Dis. 94(5):638. doi:10.1094/PDIS-94-5-0638B.
- Klein JM, Stockwell VO, Minsavage GV, Vallad GE, Goss EM, Jones JB. 2020. Improved deferred antagonism technique for detecting antibiosis. Lett Appl Microbiol. 71(4):330–336. doi:10.1111/lam.13339.
- Kucharek T. 1994. Bacterial Spot of Tomato and Pepper. PP3. Gainesville: University of Florida Institute of Food and Agricultural Sciences.
- Leben C 1981. How plant-pathogenic bacteria survive. Plant Dis. 65:633–637.
- Lewis Ivey ML, Tusiime G, Miller SA. 2010. A polymerase chain reaction assay for the detection of Xanthomonas campestris pv. musacearum in banana. Plant Dis. 94(1):109–114.
- Louws FJ, Fulbright DW, Stephens CT, de Bruijn FJ. 1994. Specific genomic fingerprints of phytopathogenic Xanthomonas and Pseudomonas pathovars and strains generated with repetitive sequences and PCR. Appl Environ Microbiol. 60(7):2286–2295. doi:10.1128/aem.60.7.2286-2295.1994.
- Ma X 2015. Characterization and management of bacterial spot of processing tomato in Ohio. Ph.D. dissertation, The Ohio State University, Columbus.
- Ma X, Lewis Ivey ML, Miller SA. 2011. First Report of Xanthomonas gardneri Causing Bacterial Spot of Tomato in Ohio and Michigan. Plant Dis. 95(12):1584. doi:10.1094/PDIS-05-11-0448.
- Marco GM, Stall. 1983. Control of Bacterial Spot of Pepper Initiated by Strains of Xanthomonas campestris pv. vesicatoria That Differ in Sensitivity to Copper. Plant Dis. 67(7):779–781. doi:10.1094/PD-67-779
- Marutani-Hert M, Hert AP, Tudor-Nelson SM, Preston JF, Minsavage GV, Stall RE, Roberts PD, Timilsina S, Hurlbert JC, Jones JB. 2020. Characterization of three novel genetic loci encoding bacteriocins associated with Xanthomonas perforans. PLoS ONE. 15(5):e0233301. doi:10.1371/journal.pone.0233301.
- Mtui HD, Bennett MA, Maerere AP, Miller SA, Kleinhenz MD, Sibuga KP. 2010. Effect of seed treatments and mulch on seedborne bacterial pathogens and yield of tomato (Solanum lycopersicum Mill.) in Tanzania. J Anim Plant Sci. 8(3):1006–1015.
- Newberry E, Bhandari R, Kemble J, Sikora E, Potnis N. 2020. Genome-resolved metagenomics to study co-occurrence patterns and intraspecific heterogeneity among plant pathogen metapopulations. Environ Microbiol. 22(7):2693–2708. doi:10.1111/1462-2920.14989.
- Newberry EA, Bhandari R, Minsavage GV, Timilsina S, Jibrin MO, Kemble J, Sikora EJ, Jones JB, Potnis N 2019. Independent Evolution with the Gene Flux Originating from Multiple Xanthomonas Species Explains Genomic Heterogeneity in Xanthomonas perforans. Stabb EV, editor. Appl Environ Microbiol. 85(20). [accessed 2021 Dec 27]. https://doi.org/10.1128/AEM.00885-19
- Peterson GH. 1963. Survival of Xanthomonas vesicatoria in soil and diseased tomato plants. Phytopathology. 53:765–767.
- Potnis N. 2021. Harnessing Eco-Evolutionary dynamics of Xanthomonads on tomato and pepper to tackle new problems of an old disease. Annu Rev Phytopathol. 59(1):289–310. doi:10.1146/annurev-phyto-020620-101612.
- Potnis N, Krasileva K, Chow V, Almeida NF, Patil PB, Ryan RP, Sharlach M, Behlau F, Dow JM, Momol M, et al. 2011. Comparative genomics reveals diversity among xanthomonads infecting tomato and pepper. BMC Genom. 12(1):146. doi:10.1186/1471-2164-12-146.
- Potnis N, Timilsina S, Strayer A, Shantharaj D, Barak JD, Paret ML, Vallad GE, Jones JB. 2015. Bacterial spot of tomato and pepper: diverse X anthomonas species with a wide variety of virulence factors posing a worldwide challenge. Mol Plant Pathol. 16(9):907–920. doi:10.1111/mpp.12244.
- Quezado-Duval AM, Leite RP, Truffi D, Camargo LEA. 2004. Outbreaks of Bacterial Spot Caused by Xanthomonas gardneri on Processing Tomato in Central-West Brazil. Plant Dis. 88(2):157–161. doi:10.1094/PDIS.2004.88.2.157.
- Rajashekara G, Glover DA, Krepps M, Splitter GA. 2005. Temporal analysis of pathogenic events in virulent and avirulent Brucella melitensis infections. Cell Microbiol. 7:1459–1473. doi:10.1111/j.1462-5822.2005.00570.x
- Sahin F 1997. Detection, identification and characterization of strains of Xanthomonascampestris pv. vesicatoria by traditional and molecular methods, and resistance in Capsicum species to Xanthomonas campestris pv. vesicatoria pepper race 6. Ph.D. dissertation, The Ohio State University, Columbus.
- Sahin F, Abbasi PA, Ivey MLL, Zhang J, Miller SA. 2003. Diversity Among Strains of Xanthomonas campestris pv. vitians from Lettuce. Phytopathology®. 93(1):64–70. doi:10.1094/PHYTO.2003.93.1.64.
- Schaad NW, Jones JB, Chun W Laboratory Guide for the Identification of Plant Pathogenic Bacteria. American Phytopathological Society.
- Srivastava V, Deblais L, Kathayat D, Rotondo F, Helmy YA, Miller SA, Rajashekara G. 2021. Novel small molecule growth inhibitors of Xanthomonas spp. causing bacterial spot of tomato. Phytopathology. 111(6):940–953. doi:10.1094/PHYTO-08-20-0341-R.
- Strayer-Scherer A, Liao Y, Abrahamian P, Timilsina S, Paret M, Momol T, Jones J, Vallad G 2019. Integrated management of bacterial spot on tomato in Florida. [accessed 2019 May 11]. doi:10.32473/edis-pp353-2019.
- Strayer AL, Jeyaprakash A, Minsavage GV, Timilsina S, Vallad GE, Jones JB, Paret ML. 2016. A Multiplex Real-Time PCR Assay Differentiates Four Xanthomonas Species Associated with Bacterial Spot of Tomato. Plant Dis. 100(8):1660–1668. doi:10.1094/PDIS-09-15-1085-RE.
- Thayer PL, Stall RE. 1962. A survey of Xanthomonas vesicatoria resistance to streptomycin. Bull – Univ Fla, Agric Exp Stn. 1523:163–165.
- Timilsina S, Jibrin MO, Potnis N, Minsavage GV, Kebede M, Schwartz A, Bart R, Staskawicz B, Boyer C, Vallad GE, et al. 2015. Multilocus sequence analysis of xanthomonads causing bacterial spot of tomato and pepper plants reveals strains generated by recombination among species and recent global spread of Xanthomonas gardneri. Appl Environ Microbiol. 81(4):1520–1529. doi:10.1128/AEM.03000-14.
- Timilsina S, Pereira-Martin JA, Minsavage GV, Iruegas-Bocardo F, Abrahamian P, Potnis N, Kolaczkowski B, Vallad GE, Goss EM, Jones JB. 2019. multiple recombination events drive the current genetic structure of Xanthomonas perforans in Florida. Front Microbiol. 10:448. doi:10.3389/fmicb.2019.00448
- Tudor SM 1999. Molecular characterization of bacteriocin-like activity in tomato race-three strains of Xanthomonas campestris pv. vesicatoria. Ph.D. dissertation, Univ. of Florida, Gainesville.
- Tudor-Nelson SM, Minsavage GV, Stall RE, Jones JB. 2003. Bacteriocin-Like substances from tomato race 3 Strains of Xanthomonas campestris pv. vesicatoria. Phytopathology. 93(11):1415–1421. doi:10.1094/PHYTO.2003.93.11.1415.
- USDA. 2016. Bacterial spot of tomato. Fact sheet. Available at https://nifa.usda.gov/sites/default/files/resource/Tomato_Spot_Fact_Sheet.pdf
- Vidaver AK. 1983. Bacteriocins: the lure and the reality. Plant Dis. 67(5):471–475. doi:10.1094/PD-67-471.
- Vidaver AK. 1989. Gram positive bacteria. In: Schaad NW, editor. Laboratory Guide for Identification of Plant Pathogenic Bacteria. St Paul (MN): The American Phytopathological Society; p. 12–16.
- Wang J, Woo M, Yan C. 2017. Spot plating assay for the determination of survival and plating efficiency of Escherichia coli in sub-MIC levels of antibiotics. JEMI Methods. 1:26–29.
- Zaccardelli M, Campanile F, Villecco D, Parisi M. 2011. Infections of bacterial spot on processing tomato in southern Italy. Acta Hort. 914:71–73. doi:10.17660/ActaHortic.2011.914.10