Abstract
Anthracnose, caused by hemibiotrophic Colletotrichum spp., is a destructive disease of legumes and many other crops worldwide. Colletotrichum spp. constitute one of the top 10 phytopathogenic fungi, infecting ∼3,000 plant species, attacking food and forage legume crops at all growth stages; including seed, seedlings, young, and mature plants; with consequent significant yield reductions. Presently, cultural practices and substantial use of synthetic fungicides are the most prevalent approaches for anthracnose management. In addition, there has been a strong focus toward developing advanced breeding lines and cultivars with improved anthracnose resistance. This has involved traditional breeding resulting in a wide range of anthracnose resistance resources being identified, particularly using advanced techniques within the common bean, soybean, lentil, mungbean, blackgram, and lupins. For instance, quantitative trait loci (QTLs) for resistance have been identified, enabling marker-assisted resistance breeding. More recently, molecular approaches; including genomics, transcriptomics, proteomics, and metabolomics; have been utilized to understand the pathogenesis and defense mechanisms involved in the Colletotrichum-legume interaction. Genetic manipulation through omics offers scope to better protect legumes from anthracnose by improving the efficiency of breeding programs. This review focuses on key pathogens (viz., C. truncatum, C. lentis, C. lupini, and C. lindemuthianum) causing anthracnose in legumes, their biology, and epidemiology, the disease management levers embracing progress with host resistance, genetic and breeding approaches, and highlights critical knowledge gaps in conventional and molecular breeding programs. We conclude that the ongoing progress toward developing breeding lines/cultivars/donors with improved resistance in legume plant responses against anthracnose using omics approaches offers novel insights into legume-anthracnose pathogen interactions and ensures more sustainable and effective disease management strategies for the future.
I. Introduction
Legumes belong to the family Fabaceae (or Leguminosae) whose ripe and unripe seeds as well as pods play an important role in human nutrition due to their nutritional and health benefits since ancient times (Giugliano et al., Citation2006; Reckling et al., Citation2016; Clemente and Olias, Citation2017). Legumes are safe for consumption, being present in the diet of millions of individuals worldwide, also grown for livestock forage and fodder, and as a green manure to improve soil health (Tivoli et al., Citation2006). They play a key role in crop rotation, offering cropping system diversification, while also providing many ecosystem services including atmospheric nitrogen fixation, enhancing colonization by arbuscular mycorrhizal fungi, and boosting water supply and nutrient uptake in host plants (Murphy-Bokern et al., Citation2017). In addition, soil structure improvement and mobilization of phosphorus are key ecosystem benefits (Kamh et al., Citation1999). Worldwide, beans (Phaseolus vulgaris L.), blackgram or urdbean (Vigna mungo (L.) Hepper), broad bean or faba bean (Vicia faba L.), chickpea (Cicer arietinum L.), cowpea (Vigna unguiculata (L.) Walp.), lentil (Lens culinaris Medik), white lupin (Lupinus albus L.), narrow-leafed lupin or blue lupin (Lupinus angustifolius L.), yellow lupin (Lupinus luteus L.), mungbean (Vigna radiata (L.) R. Wilczek), pea (Pisum sativum L.), peanut (Arachis hypogaea L.), pigeon pea (Cajanus cajan L.), and soybean (Glycine max (L.) Merr.) are grown as major food legumes depending upon the requirements and suitable conditions for their cultivation (Pagano and Miransari, Citation2016; Pandey et al., Citation2018; Alkemade et al., Citation2023). India is the major legume producer (6.3 MT ha, 4.5 MT), followed by Myanmar, Russia, China, Canada, Turkey, and the United States (Pandey et al., Citation2018). Forage legumes, such as clovers (Trifolium spp.), and annual medic and perennial alfalfa (Medicago spp.), are of critical importance for animal production globally, particularly in temperate and Mediterranean-type climates (Jacob et al., Citation2016), whereas Stylosanthes spp. are important in tropical-type environments (Chakraborty, Citation2004). Together with other forage legume genera/species, these contribute a major proportion of feed for ruminants in agriculture, frequently underpinning many and varied animal grazing enterprises. For example, some 29 m ha of annual Trifolium spp. has been planted in Australia alone (Hill and Donald, Citation1998). Likewise, the annual Medicago spp. play an important agronomic role in dryland farming regions worldwide (Walsh et al., Citation2001) where they are often an integral component of cropping systems (Piano and Francis, Citation1992). Finally, Stylosanthes species are among the most versatile, widely adopted, and productive tropical pasture legumes commercially used in a range of agricultural systems in many countries with a tropical or sub-tropical climate (Chakraborty, Citation2004).
Pests and diseases are the major biotic stresses limiting the production of legumes worldwide, and among them, anthracnose is becoming a prevalent threat in major crop legume growing countries (Dias et al., Citation2016; Nataraj et al., Citation2020; Pandey et al., Citation2021a; Lima et al., Citation2023). For example, under favorable conditions, this disease can cause from 60 to 100% yield loss in-field crop legume production (Hartman et al., Citation1999; da Silva et al., Citation2020; Chakraborty et al., Citation2022; Kaur et al., Citation2023). Anticipated climate changes are likely to foster more severe diseases. For example, in Germany and Switzerland, C. lupini threatens lupin production with a current yield loss of >70% (Alkemade et al., Citation2021a), which is predicted to reach up to 100% from future anticipated climate change. Despite efforts to reduce the risks of anthracnose epidemic development, there have been frequent failures, such as in mid 1990s in Europe where very high yield losses were observed and resulted in a rapid decline of the lupin cultivation area (Talhinhas et al., Citation2016).
Important forage legume species, for example, clovers (Shivas, Citation1989; Jacob et al., Citation2016), annual and perennial Medicago spp. (Lamprecht and Knox-Davies, Citation1984; Tivoli et al., Citation2006; Jacob et al., Citation2016), and Stylosanthes spp. (Irwin and Cameron, Citation1978; Chakraborty, Citation2004), can be severely attacked by one or more Colletotrichum spp. Examples of the latter include C. trifolii on clovers and annual and perennial Medicago spp. (Mackie et al., Citation2003; Tivoli et al., Citation2006), and species, such as C. gloeosporioides on Stylosanthes spp. (Irwin and Cameron, Citation1978; Lenné, Citation1994; Chakraborty, Citation2004). Losses from anthracnose are well-defined for crop legumes but the impact of the disease on the productivity and sustainability of forage legumes and animal grazing enterprises remain largely undefined due to a lack of established quantitative links between disease severity and sustainable animal production (Chakraborty et al., Citation1996). The latter is perhaps a consequence of the insidious impact of anthracnose on forage legumes, resulting in a gradual decline of forage productivity leading to poor ground cover (Chakraborty, Citation2004). An exception among forage legumes is Stylosanthes spp., whereby foliar infection results from 40 to 100% yield losses in tropical forage legumes (Lenné, Citation1986; Kelemu et al., Citation1999).
Current research indicates that anthracnose in legumes has complex etiology (Perseguini et al., Citation2016; Dias et al., Citation2018; Luo and Jiang, Citation2022), with C. truncatum and C. lindemuthianum the most prevalent species affecting food crop legume production (Sharma et al., Citation2011). The genus, C. comprises a large clade of class Sordariomycetes with more than 200 accepted species. Based on the broad host range and molecular phylogenetics, the genus can be further classified into 14 species complexes and single species (Marin-Felix et al., Citation2017). Colletotrichum species are one of the top 10 phytopathogenic fungi infecting ∼3,000 plant species, resulting in significant yield reductions of food crops (Cannon et al., Citation2012; da Silva et al., Citation2020). Worldwide, several Colletotrichum species complexes, such as C. acutatum, C. orbiculare, C. truncatum, C. destructivum, C. orchidearum, C. dematium, C. spaethianum, C. magnum, C. gloeosporioides, C. chlorophyti, and C. coccodes infect legumes (Weir et al., Citation2012; Damm et al., Citation2014, Citation2019). This genus has a hemibiotrophic lifestyle and can be manipulated in the laboratory, making it a valuable model pathogen for physiological, biochemical, and genetic studies (Perfect et al., Citation1999).
In addition to C. truncatum, several other species of Colletotrichum cause anthracnose in food crop legumes. These include the following: C. lupini on lupin (Nirenberg et al., Citation2002; Rychel-Bielska et al., Citation2020), C. lentis on lentil (Damm et al., Citation2014; Buchwaldt et al., Citation2018), C. lindemuthianum on beans and blackgram (Lima Castro et al., Citation2017) and C. sojae (Damm et al., Citation2019), C. chlorophyti (Yang et al., Citation2012), C. gloeosporioides (Mahmodi et al., Citation2013), C. coccodes (Riccioni et al., Citation1998), C. cliviae Yang (Barbieri et al., Citation2017), C. plurivorum (Damm et al., Citation2019), C. incanum (Yang et al., Citation2014), C. musicola (Boufleur et al., Citation2020), C. destructivum (Manandhar, Citation1986; Damm et al., Citation2014) and C. brevisporum (Shi et al., Citation2021b) on soybean. These species also infect other food crops (). The disease is common under high humidity and temperature with frequent rainfall (Yang and Hartman, Citation2015), and affects crops at all the physiological stages including pods and seed, seedlings, young and mature plants. The necrotrophic lifestyle of the pathogen can cause complete defoliation of seedlings and mature plants (Yang et al., Citation2015), and results in yield reductions up to 100% under high humid conditions and temperature ∼30 °C that commonly occur in countries, such as India, China, Myanmar, Turkey, and USA.
Table 1. Colletotrichum species complexesTable Footnote* associated with anthracnose of legumes.
Mycologists now benefit in the era of molecular biology and no longer need to solely rely only on morphological features-based identification of Colletotrichum species characteristics that are highly influenced by environmental conditions (Jayawardena et al., Citation2016). A polyphasic approach is currently used to identify species in this genus using morpho-cultural features and multiple locus phylogenetic analyses of DNA sequences (Liu et al., Citation2016). Several species within Colletotrichum exhibit high genetic variability but the mechanisms behind such variability remain unclear (da Silva et al., Citation2020). Further, genetic studies of Colletotrichum spp. have offered unique insights toward improvements in plant disease prevention and management strategies in various legumes (Ureña-Padilla et al., Citation2002; Ciampi-Guillardi et al., Citation2014; Pandey et al., Citation2021a).
At present, most studies of anthracnose on food legumes concentrate on C. truncatum, C. lentis, C. lupini, and C. lindemuthianum, with little information regarding other opportunistic Colletotrichum spp. infecting them, which likely poses challenges for its management under field conditions (Ureña-Padilla et al., Citation2002; Pandey et al., Citation2018). It also raises questions as to which other complex species of Colletotrichum interact with these major four species thereby increasing disease severity. To prevent and manage the disease, it is crucial to accurately identify the causal agents. In legume anthracnose, multiple Colletotrichum species may differentially affect disease management where they respond differently to different management methods. This is perhaps one of the reasons why studies on the use of synthetic fungicides in managing anthracnose in legumes have shown contradictory efficacy (Dias et al., Citation2016; Poti et al., Citation2020).
Currently, anthracnose in legumes is primarily mitigated through non-genetic approaches, such as cultural practices along with the use of synthetic fungicides and natural agents. Nevertheless, locating and deploying genetic resistance has been keenly sought by legume breeders. Considerable progress has been made in developing anthracnose resistant varieties/cultivars in legumes using traditional and omics approaches. While, to date, genetic resistance has been searched for and utilized against a specific Colletotrichum species, such resistance is not effective when anthracnose is a disease complex caused by multiple Colletotrichum species. This highlights an urgent need for critical analysis and reappraisal of legume anthracnose literature. In this review, we provide a comprehensive overview of anthracnose in legumes caused by C. truncatum, C. lentis, C. lupini, and C. lindemuthianum, focusing on the pathogen’s epidemiology, pathogenicity, host resistance mechanisms, and genetics and breeding progress using genetic and omics approaches toward developing breeding lines/cultivars with improved resistance to better manage legume anthracnose diseases.
II. Current status of anthracnose in legumes
The pathogen, Colletotrichum (sexual stage: Glomerella) is one of the most significant members of the kingdom fungi in the division Ascomycota (Order: Glomerellales, Class: Sordariomycetes). Despite its asexual morphology, for which the genus is named, molecular phylogenetics place the species in the Ascomycota (Cai et al., Citation2009). Colletotrichum species in legumes remain undefined in terms of the number and type of species exhibiting pathogenicity. Worldwide, many countries have reported at least one Colletotrichum species allied with one or more of the major legumes, such as soybean, peanut, chickpea, common bean, pea, pigeon pea, lupins, lentil, blackgram, and mungbean. More than 15 European countries have already reported lupin anthracnose (Fischer et al., Citation2015), at least eight European countries have reported anthracnose in lentils (Buchwaldt et al., Citation2004), and C. lindemuthianum on common bean has been reported in several African countries (Adebitan and Olufajo, Citation1998). Countries like USA, China, India, Taiwan, and Brazil have greatest Colletotrichum species diversity, followed by Mediterranean countries, Canada, Myanmar, and other south Asian countries (da Silva et al., Citation2020). Historically, C. truncatum and C. lindemuthianum are considered worldwide prevalent species of anthracnose in legumes causing 30–100% yield loss in cowpea (Enyiukwu et al., Citation2014; Falade and Borisade, Citation2018), common bean (Adam-Blondon et al., Citation1994), mungbean (Pandey et al., Citation2021a), chickpea (Mahmodi et al., Citation2013), soybean (Sharma et al., Citation2011; Yang and Hartman, Citation2015), and blackgram (Aggarwal et al., Citation2019).
The main Colletotrichum species affecting lentil production was formerly considered as C. truncatum, which has a wide host range (Altaf et al., Citation2018). However, in view of the recent clear differentiation of C. lentis from C. truncatum (Damm et al., Citation2014; Buchwaldt et al., Citation2018), many plant species may be non-hosts of C. lentis. Therefore, while C. lentis appears to have a limited host range in the Fabaceae family, since 1987 it has seriously affected lentil cultivation in Canada (Morrall, Citation1988; Altaf et al., Citation2018). In association with extensive lentil cultivation, it was also confirmed in China (Xu et al., Citation2017), Bulgaria (Kaiser et al., Citation1998), and the United States (Venette, Citation1994). Other than lentils, C. lentis causes anthracnose in faba bean, pea, chickpea (Gossen et al., Citation2009), and in common vetch (Vicia sativa L.) in China (Xu et al., Citation2017). Although this species does not currently infect lupins, dry bean (Phaseolus vulgaris L.), or alfalfa (Gossen et al., Citation2009), this could change in the future with it becoming pathogenic across multiple legumes species, perhaps a consequence of expected environmental changes associated with future climates.
Colletotrichum boninense and C. gigasporum have been isolated from soybean, although isolations were made either from asymptomatic plant tissues or with no evidence of pathogenicity (da Silva et al., Citation2020). In contrast, the pathogenicity of C. gloeosporioides, C. dematium, C. truncatum, and C. magnum has been demonstrated in soybean (da Silva et al., Citation2020). Anthracnose caused by C. destructivum and C. truncatum is one of the most prevalent disease complexes of soybean in Argentina, the world’s third largest soybean producer (Ramos et al., Citation2010, Citation2013). However, since both species were only identified by conidial features, it remains unclear whether C. destructivum was truly associated with the disease (Ramos et al., Citation2013). Despite this, the literature reports show that soybean anthracnose is caused by multiple Colletotrichum species whereas anthracnose on lentil, lupins, bean, mungbean, blackgram, and cowpea is caused by a single species (Boufleur et al., Citation2022). Despite the importance of an accurate identification of the causal agent in effective disease management (Chen et al., Citation2018), reliable pathogen diagnosis techniques for the disease complexes are currently lacking, and addressing this needs to be prioritized in future research.
The distribution of C. truncatum, C. gloeosporioides, C. lindemuthianum, C. lupini, and the more recently described C. lentis, shows a noteworthy correlation with both relative humidity and atmospheric temperature (Shi et al., Citation2022). This is not surprising as these factors strongly influence pathogen infection, survival, and spread. Reports of anthracnose caused by Colletotrichum species in legumes and other crops on a global scale are presented in .
III. Disease symptoms
Colletotrichum-caused disease symptoms can be observed on legume crops at all growth stages. They include pre- and post-emergence damping-off that consists of seed rotting, seedling wilting, as well as dark, depressed, and irregular patches on petioles, stems, and pods in advanced growth stages leading to premature defoliation of plants (Pandey et al., Citation2021a; Sharma et al., Citation2011; Yang et al., Citation2015). Yellow to brown lesions appear on the infected seeds, which may result in poor germination. Small, dark brown to black lesions can be seen on diseased cotyledons. Infected hypocotyls develop rust-colored specks that enlarge longitudinally, resulting in sunken lesions. Infected cotyledons senesce prematurely, resulting in stunted growth. Species, such as C. coccodes, C. incanum, C. gloeosporioides, C. lindemuthianum, C. truncatum, C. musicola, and C. plurivorum cause such symptoms (Riccioni et al., Citation1998; Mahmodi et al., Citation2013; Dias et al., Citation2018; Boufleur et al., Citation2020). Necrotic spots caused by C. truncatum on stems are hexagonal (e.g., on soybean, Yang et al., Citation2014 and mungbean, Pandey et al., Citation2018) while rounded to asymmetrical grayish spots surrounded with dark margins are often caused by C. sojae (Damm et al., Citation2019). In contrast, C. chlorophyti causes intra- and inter-veinal necrotic spots bounded by slight chlorosis (Yang et al., Citation2012). On blackgram, C. truncatum produces circular, black, sunken spots with dark center and bright red orange margins on leaves and pods, which later become horseshoe shaped in appearance. In severe infections, the affected parts wither. Symptoms on leaves, pods, and stem caused by Colletotrichum species on legumes are shown in .
IV. Disease cycle
The disease cycle of C. lindemuthianum, C. lentis, C. lupini, and C. truncatum has been well-described in the literature (Adhikari et al., Citation2009; Yang and Hartman, Citation2015; Padder et al., Citation2017; Singh et al., Citation2022) while that of other Colletotrichum spp. associated with legume anthracnose is still poorly documented. These four species are soil- and seed-borne, survive in infected crop debris and on wild plants, and can produce virulent microsclerotia (Iamsupasit et al., Citation1993; Yang and Hartman, Citation2015; Padder et al., Citation2017; Singh et al., Citation2022). There is no evidence that weeds or alternative hosts play a role in the disease epidemiology. Infected seed is assumed to be the main source of primary inoculum allowing long-distance dissemination of these pathogens and global seed market and trade contribute to the introduction of aggressive pathogen isolates to new production areas (Chakraborty et al., Citation1996; Singh et al., Citation2022).
During the lifecycle, conidia germinate and form appressoria on the host plants after penetration of fungal hyphae on plant surfaces. All species, including C. truncatum, C. lentis, C. lupini, and C. lindemuthianum, infect and colonize leaf and/or stem surfaces in a similar manner (Khan, Citation1992; Cannon et al., Citation2012; Rao et al., Citation2020). The pathogen lives a hemibiotrophic lifecycle in the first stage of development whereby a primary hypha develops from the penetration peg. This is followed by biotrophic vesicles located between plasma membranes and cell walls. As the biotrophic phase ends, the necrotrophic phase begins with the development of secondary hyphae which colonize tissues intra- and inter-cellularly and kill the plant cells (Kavanashree et al., Citation2022). Sometimes, the pathogen also has an endophytic lifestyle (Bhadauria et al., Citation2013a) by colonizing internal plant tissues without showing any disease symptoms (Chen et al., Citation2006; Luo and Jiang, Citation2022). Necrotrophic stages of anthracnose produce acervuli containing conidia, a typical symptom of anthracnose. Secondary infection cycles follow, with conidia dispersed by splashing water that dissolves the mucilage covering them and aids in short-range dispersal (Kaiser et al., Citation1998). An example of C. truncatum lifecycle is presented in .
Epidemiological studies significantly contribute toward effective disease management and require an understanding of the relative status of each species complex in its development. Several species associated with legume anthracnose are known to undergo sexual reproduction, including C. sojae, C. gloeosporioides, C. plurivorum, and C. musicola (Ramos et al., Citation2013; Boufleur et al., Citation2020). For example, in soybean, previously Glomerella glycines (=C. sojae) was considered as the sexual morph of three Colletotrichum species, creating taxonomic confusion that was later resolved by Damm et al. (Citation2019). Similarly, Glomerella cingulata was considered as sexual morph of C. gloeosporioides, an anthracnose pathogen of mungbean (Chaudhari and Gohel, Citation2016) and forage legumes (Iamsupasit et al., Citation1993). However, some species like C. destructivum, C. incanum, and C. truncatum possess only the asexual stage (Cannon et al., Citation2012). Nonetheless, a few concerns regarding the epidemiology of pathogens still need to be addressed, as they directly affect disease management. For example, pathogens with a sexual stage in their lifecycle often have greater survival on alternate hosts. Genetic recombination plays an important role in Colletotrichum species variability and pathogen survival. While sexual ascospores can spread the disease over wide distances and can infect the plants in different way compared to conidia, most of the infection processes are generally similar (Cannon et al., Citation2012). Moreover, the infection process of C. lupini was recently visualized in Lupinus mutabilis (Guilengue et al., Citation2022), where it was found that the pathogen penetration occurred from melanized appressoria and then a switch to necrotrophy was observed after 3 days after pathogen inoculation. A recent study revealed that C. lupini reproduces clonally through four independent lineages (Alkemade et al., Citation2023). Differences in morphology and virulence patterns have been reported among the C. lupini isolates on Andrean lupin and white lupin between and within the clonal lineages. Isolates from lineage II contained a minichromosome, and while it was partially present in lineages III and IV it was not present in isolates belonging to lineage I (Alkemade et al., Citation2023). Variations in the presence of this minichromosome suggest it could have a possible role in host-pathogen interactions.
V. Variability of Colletotrichum isolates in legumes and causes of variability
Species of Colletotrichum are exceedingly variable in terms of toxin production and their morphological and genetic features (Hyde et al., Citation2009; Trabanco et al., Citation2015). There are various explanations for the high level of genetic variation in the genus Colletotrichum, including the occurrence of natural mutation (O’Connell et al., Citation1993; Bhadauria et al., Citation2013a) and heterokaryosis (O’Connell et al., Citation1993; Chacko et al., Citation1994; Souza-Paccola et al., Citation2003). In addition to natural mutation, asexual recombination, and heterokaryosis, the so-called parasexual cycle, has been proposed as more vital in causing genetic difference in imperfect fungi, viz., C. truncatum, C. lindemuthianum, and C. gloeosporioides (O’Connell et al., Citation1993; Bhadauria et al., Citation2013a). There is also evidence that in nature, heterokaryon incompatibility appears to inhibit parasexuality. In this section, we discuss the extent to which variability exists at different levels.
A. Morpho-cultural variability
Species of Colletotrichum that infect legumes are highly variable in their cultural (colony color, growth pattern) and morphological features, such as hyphal morphology; shape and size of conidia, and sporulation. For example, the presence of boat-shaped conidia can discriminate C. truncatum from other species of Colletotrichum but not between closely related/parallel species (Pandey et al., Citation2021a). The length and width of conidia and conidiophores are other morphological features that can be considered in the differentiation of species (da Silva et al., Citation2020). Another test to measure variability among isolates is the vegetative compatibility test (Liu et al., Citation2021). For instance, two genetic groups (I and II) have been identified within C. lupini based on their vegetative compatibility (Shivas et al., Citation1998). Historically, phenotypic variability was considered for the classification and nomenclature of species within the genus Colletotrichum. However, morphological features alone were inaccurate parameters in species discrimination as their growth was influenced by many factors, including the composition of culture media, intensity of light, and temperature (Cai et al., Citation2009; Liu et al., Citation2014, Citation2016). For instance, C. truncatum and C. gloeosporioides conidial length and width varied when both species were grown on different culture media (Mahmodi et al., Citation2013; Pandey et al., Citation2021a). Based on these morphological differences, host specialization has become more acceptable for species discrimination in Colletotrichum (Chongo et al., Citation2002; Gossen et al., Citation2009; Reveglia et al., Citation2023). The diagnostic value based morphological features, however, should not be overlooked. For instance, host specialization may not be a suitable diagnostic measure in cases where a Colletotrichum isolate can infect more than one host or when different species of the pathogen are involved as a disease complex.
B. Variability at the molecular level
Since 1980, molecular markers have enabled the investigation of genetic variation at the molecular level along with molecular taxonomy and phylogeny. Genetic variability among isolates of C. truncatum, C. gloeosporioides, C. lindemuthianum, and other Colletotrichum species has been widely investigated using diverse molecular markers. These include the following: random amplified polymorphic DNA (RAPD, Adam-Blondon et al., Citation1994; Sant’Anna et al., Citation2010); restriction fragment length polymorphism (RFLP, Tullu et al., Citation2006); amplified fragment length polymorphism (Tullu et al., Citation2006); random amplified microsatellites (Rogério et al., Citation2019); inter simple sequence repeat (Mahmodi et al., Citation2014; Basandrai et al., Citation2016); simple-sequence repeats (Rogério et al., Citation2019); and nuclear internal transcribed spacers (Nirenberg et al., Citation2002; Ford et al., Citation2004; Padder et al., Citation2008; Fontenelle et al., Citation2017; Pandey et al., Citation2021a). Various molecular markers have allowed identification of high levels of genetic variability among isolates of C. truncatum and C. lentis from lentil (Ford et al., Citation2004; Xu et al., Citation2017), soybean (Rogério et al., Citation2017), blackgram (Basandrai et al., Citation2016), and other hosts (Vasconcelos et al., Citation1994; Sharma, Citation2009; Sant’Anna et al., Citation2010). This highlights the extensive genetic variation among Colletotrichum isolates worldwide despite genetically homogeneous regional populations and suggests a restricted geographical dispersal range of Colletotrichum species (Rogério et al., Citation2019). However, despite these molecular investigations, the molecular phylogeny and taxonomy of the genus Colletotrichum remain unclear.
C. Variability in toxin production
Colletotrichum species produce a highly variable range of host-specific toxins (García-Pajón and Collado, Citation2003), such as for isolates of C. truncatum on soybean (Masi et al., Citation2022). To date, more than 14 Colletotrichum species complexes have been identified (Bhunjun et al., Citation2021). Some of these cannot be discriminated from saprophytic or hemibiotrophic species like C. gloeosporioides, C. lindemuthianum, and C. truncatum based on morphological features, but they can be differentiated based on their unique pathogenicity (Chongo et al., Citation2002; Chakraborty et al., Citation2019) which is strictly dependent on the specific toxins (Masi et al., Citation2022). Despite this variability, there is no scientific consensus in classifying Colletotrichum species according to their pathotypes (Chongo et al., Citation2002; Banniza et al., Citation2018).
VI. Mechanisms of pathogenicity
A. Enzymatic reactions
The pathogenicity of Colletotrichum species in legumes has not been well-studied despite recent advances in omics technology (Rogério et al., Citation2019). However, research has shown that conidia germinate in both susceptible and resistant cultivars after successful infection, producing germ tubes, which build up in intercellular spaces in the leaf epidermal layer (Khan and Sinclair, Citation1991; Pandey et al., Citation2021a). In bean, the biotrophic stage of C. lindemuthianum develops into numerous cortical and epidermal cells, whereas in cowpea this stage is always limited to a single epidermal cell (O’Connell et al., Citation1985; Bailey et al., Citation1990). Pathogen penetration and movement in this process most likely occur through enzymatic action. Fungal phytopathogens also transude various cell wall degrading enzymes that permit them to successfully infect plants (O’Connell et al., 2012; Faisal Peeran et al., Citation2014). For example, Colletotrichum species produce cellulase enzymes that catalyze the host’s cell wall degradation (Anand et al., Citation2008; Faisal Peeran et al., Citation2014). While C. truncatum (Ramos et al., Citation2010), C. gloeosporioides (Faisal Peeran et al., Citation2014), and C. lindemuthianum (O’Connell et al., Citation1985; Sharma et al., Citation2007) have shown potential in vitro pectinolytic and cellulase activity, a significant variation in cellulase activity has been reported under field conditions (O’Connell et al., Citation1985; Ramos et al., Citation2010). Other enzymes produced by Colletotrichum species during pathogenesis include chitinase, pectinolytic enzyme, glucanase, protein kinases, and endo-polygalacturonases (Anand et al., Citation2008; Faisal Peeran et al., Citation2014). Considering the limited information available, no conclusion can be drawn regarding the role of enzymes in Colletotrichum pathogenicity.
B. Toxin production
Colletotrichum species produce low molecular weight compounds called phytotoxins that may cause histological and physiological alterations in legumes. For example, hemibiotrophic infection of Pisum sativum by C. truncatum caused significant histological and physiological changes in hosts (O’Connell et al., Citation1993). Phytotoxins produced by fungi may be host-specific (selective, non-toxic to other hosts) or non-specific (non-selective, toxic for a broader range of plant species). Hence, production of toxins is a key part of pathogenesis (Banniza et al., Citation2018). Toxins produced by the pathogen can have unexplainable effects on pathogenesis, elicitor production, and enzymatic reactions (Katoch et al., Citation2017). Therefore, it can be difficult to study the mechanisms of pathogenicity when these factors are interconnected (Amusa, Citation1994). Colletotrichum produces non-host-specific toxins, such as colletopyrone by C. nicotianae and colletotin by C. fuscum (Amusa, Citation1994). Likewise, C. truncatum produces meso- and D (−)-butane2,3-diol, colletruncoic acid, methyl ester, and 2-hydroxymethylhexa-2,4-dienol in soybean (García-Pajón and Collado, Citation2003). The presence of numerous other metabolites and non-host specific compounds in culture filtrates of C. lindemuthianum and C. truncatum have been reported (Amusa, Citation1994). In addition, while García-Pajón and Collado (Citation2003) also studied metabolites isolated from various Colletotrichum species, including C. gloeosporioides and C. truncatum, it is evident that their actual pathogenesis role in legumes remains unclear and needs resolution. Further, the role of toxins in anthracnose disease development remains poorly investigated. Parthasarathy et al. (Citation2015) showed that culture filtrates of C. gloeosporioides produce a non-host selective toxic metabolite that shows inhibitory effects on seed germination of various crops like maize, sorghum, tobacco, tomato, and chili. Recently, using the OSMAC–metabolomics approach, Reveglia et al. (Citation2023) studied secondary metabolites compounds produced by C. truncatum and C. trifolii that are accountable for phytotoxic activities in lentil, soybean, and red clover. Cultural filtrates produced colletopyrone, and higginsianin B, compounds reported to have phytotoxic and cytotoxic activities (Reveglia et al., Citation2023). Further, colletopyrone also contributed toward discriminating between fungal pathogens, being highly produced by C. truncatum (Reveglia et al., Citation2023). However, further analysis of the structure and function of the active component(s) of these toxins still needs to be investigated. Clearly, while Colletotrichum species can synthesize numerous toxins, their isolation, purification, and characterization need further research.
Various host-specific toxins are also produced by Colletotrichum species infecting legumes. For example, Liang et al. (Citation2021) identified a novel host-specific pathogenicity gene CgNPG1 of C. gloeosporioides. Likewise, CgDN3, a pathogenicity gene from C. gloeosporioides infecting tropical legumes, has been identified (Stephenson et al., Citation2000). Bhadauria et al. (Citation2015) identified ClToxB, a host-specific toxin in C. truncatum and C. lentis infecting lentil that is responsible for virulence differentiation, seemingly contributing quantitatively to virulence differences between C. lentis races 0 and 1 (Bhadauria et al., Citation2015). This indicates that these genes encode a novel pathogenicity determinant necessary to avert a hypersensitive reaction in a compatible host during the biotrophic phase of primary infection. However, no such studies have been conducted in relation to the interaction between C. truncatum and C. lindemuthianum with other legumes like chickpea, mungbean, blackgram, common bean and peanut, and this warrants future investigation.
VII. Impact of climate change on disease development
Disease outbreaks in legumes are influenced by multiple factors. The development of virulent strains within a diverse pathogen population, weather conditions, level of resistance in host plants against emerging aggressive strains of the pathogen, uniformity of cropping system, plant architecture, and limited antagonistic activities of microbial biocontrol agents can all play a crucial and inter-related role in disease outbreaks (Pangga et al., Citation2013). Under climate change, several previously reported minor diseases have become much more important worldwide, especially in Asian and European countries (Pande et al., Citation2010; Pathak et al., Citation2018; Pszczółkowska et al., Citation2019). South Asia and southern Africa are particularly vulnerable to the effects of climate change, resulting in more severe disease in grain legumes (Bahl, Citation2015). Various climatic conditions affect plant disease progress, including light, temperature maxima and minima, water availability, wind speed, and concentrations of atmospheric gases, viz., methane, ozone, and CO2 (Varanasi et al., Citation2016). These can have negative, neutral, or positive effects on disease progression, as each disease may respond differently to these agro-climatic variations (Elad and Pertot, Citation2014; Velásquez et al., Citation2018). As occurs with other crop diseases, anthracnose diseases of legumes seem similarly triggered by climate change (Thomas, Citation2010). For instance, climate change can affect anthracnose disease complex by replacing or predominating one species within the complex by another. In lentils, C. truncatum was previously considered as the main anthracnose pathogen but C. lentis has recently predominated disease epidemics in this crop (Buchwaldt et al., Citation2018).
Plant resistance traits may be affected in a positive or negative way by climate change. Elevated CO2 coupled with elevated temperatures and humidity increased anthracnose incidence in shrubby Stylosanthes in Australia (Pangga et al., Citation2004) and in chickpea in India (Sharma et al., Citation2011). Further, Sozen and Karadavut (Citation2018) reported anthracnose emergence and epidemic development, especially with heavy spring rains, led to huge production losses of chickpea in Turkey. In 2019, a field survey conducted by Girma et al. (Citation2022) to determine anthracnose epidemics in major common bean-growing areas of Ethiopia highlighted that anthracnose intensity was strongly influenced by regional zone and by altitude (i.e., by differences in rainfall, temperature, and humidity) emphasizing a need for climate change resilient agronomic practices. Climate change can alter host tolerance and/or resistance with consequent heightened risk of more serious epidemic development and greater crop losses. For example, in Australia, Thomas et al. (Citation2008) reported that Wanga, a resistant cv. of lupin, became susceptible to anthracnose when the temperature increased from 12 to 18 °C and again increased further at 26 °C. White lupin resistant Australian genotype P27174 was susceptible when cultivated in Switzerland, putatively due to difference in growing conditions (higher temperature in Switzerland, where lupin is sown as a spring crop as compared to Australia, where lupin is sown as a winter crop (Alkemade et al., Citation2021a). Similarly, mungbean genotype VI000203 B-Br found anthracnose-resistant in 2016 and 2017, became susceptible in 2018 possibly due to increased temperature (Pandey et al., Citation2021a). The anthracnose severity of mungbean and blackgram in India increased with an increase in temperature and relative humidity whereas rainfall had little effect (Kulkarni, Citation2019; Gupta, Citation2021; Pandey et al., Citation2021a). Likewise, increased soybean anthracnose severity was related to an increase in temperature and relative humidity in India (Nataraj et al., Citation2020), Brazil (Júnior et al., Citation2021), and Argentina (Dias et al., Citation2019). Similarly, elevated temperature and relative humidity significantly reduced lentil production in Canada (Buchwaldt et al., Citation2018), common bean production in Africa (Mukankusi et al., Citation2019), and cowpea production in Nigeria (Falade and Borisade, Citation2018), in each case a consequence of increased anthracnose severity. Climate change not only alters disease epidemics and associated host resistance responses, but fosters emergence of new pathotypes, ultimately leading to changes in disease management practices (Boadi and Owusu, Citation2019), as discussed further in the following section. Due to all these factors, it is difficult to speculate on future effects of climate change, particularly when the long-term datasets from the past needed to develop and test predictive models for the future are lacking (Lamichhane et al., Citation2015). However, there is growing interest in studies on the temperature requirements of Colletotrichum spp., as reflected by an increase in the number of papers published per year evaluating the effect of temperature on mycelial growth, conidial germination, conidial infection, and sporulation (Salotti et al., Citation2022).
VIII. Integrated management of legume anthracnose
As for all disease complexes, a single solution to a single problem, as used in the past, generally has not been an effective approach for sustainable anthracnose management. Several potential non-genetic approaches are used for legume anthracnose management, but these strategies individually are not anticipated to offer sustainable disease management in the future, for the reasons as discussed below. For effective anthracnose management in legumes, it needs to be based on the principles of integrated disease management (Barzman et al., Citation2015; Pandey et al., Citation2018) and agroecological crop protection (Deguine et al., Citation2023) by combing all available levers.
A. Cultural practices
This method aims to reduce or even eliminate the introduction of pathogen inoculum from nearby disease infected fields, minimize the rate of infection, and generate unfavorable conditions for disease spread and development (Mohammed, Citation2013). As the pathogen is seed-borne, hot water emersion treatment at 52–55 °C is efficient in reducing seed-borne anthracnose in mungbean, blackgram, and white lupin (Rana et al., Citation2016; Pandey et al., Citation2018; Alkemade et al., Citation2022a). Unfortunately, hot water treatment also reduces seed germination and viability limiting the possibility of its adoption for anthracnose management. Alkemade et al. (Citation2022a) reported that vinegar may also reduce white lupin anthracnose incidence under on-farm field conditions. Another cultural practice is soil solarization that, to some extent, can reduce the incidence of common bean anthracnose given the soilborne nature of the pathogen (Yousef, Citation2021). However, some Colletotrichum species, such as C. dematium, C. gloeosporioides, C. truncatum, and C. destructivum are likely to survive at temperatures higher than those achieved with soil solarization (Pandey et al., Citation2018; Salotti et al., Citation2022). However, soil solarization is not practical for large broadacre cropping situations. Other specific cultural practices used to manage legume anthracnose include crop rotation with a non-host crop, sowing of non-infected seeds, and reducing and/or eliminating sources of anthracnose from infected crop debris in which pathogen survives during winter and subsequently produce massive amounts of spores at the beginning of a new growing season (Mohammed, Citation2013). Failure to reduce/eliminate infected crop debris greatly increases the risk of serious anthracnose epidemics in nearby leguminous crops in the subsequent year.
B. Use of synthetic fungicides
Applications of synthetic fungicides are the most common approach for the management of fungal diseases in legumes (Pandey et al., Citation2018). Traditionally, broad-spectrum fungicides have been used in legumes as seed treatments and foliar sprays to manage diseases (Lamichhane et al., Citation2020). Both protectant and systemic fungicides have been included in chemical management. Systemic fungicides are applied before or during disease development, whereas protectant fungicides are applied before or during disease initiation. Currently, legume anthracnose is controlled by a mixture of fungicides designed to slow its progression. Fungicides commonly used as prophylactic treatments to control mungbean, blackgram, soybean, and beans anthracnose include methyl benzimidazole carbamate fungicides, such as carbendazim and thiophanate-methyl, that inhibit mitosis (β-tubulin assembly) in fungi, inhibiting their further growth and multiplication (Gupta et al., Citation2005; Mohammed Citation2013; Kale and Barhate, Citation2016; Kumar et al., Citation2020; Chatak and Banyal, Citation2021).
A mixture of fungicides is often used in-field as foliar sprays to slow the anthracnose progress in legumes, including beans (Mohammed, Citation2013; Sileshi et al., Citation2014; Ayana and Fininsa, Citation2018), mungbean (Chaudhari and Gohel, Citation2016; Jaiganesh et al., Citation2019; Kulkarni Citation2019; Misal et al., Citation2019), soybean (Kale and Barhate, Citation2016; Subedi et al., Citation2016), blackgram (Kumar et al., Citation2020; Chatak and Banyal, Citation2021), common bean (Amin et al., Citation2014), and chickpea (Benzohra et al., Citation2020). These include various combinations of fungicides like methyl benzimidazole carbamate, demethylation inhibitors, such as triazoles, dithiocarbamates, such as mancozeb, and quinone outside inhibitors, such as azoxystrobin. However, unfortunately multiple fungicide resistance in C. truncatum (Poti et al., Citation2020; Shi et al. Citation2021a), C. gloeosporioides (Han et al., Citation2018), and C. lindemuthianum (Lokya Naik and Anilkumar, Citation1991) populations appeared as early as 1991. In natural populations of Colletotrichum species, resistance to fungicides is controlled by a single, incompletely dominant gene present at a low level. Other limits of synthetic fungicides include their relatively high costs, toxicity to human health and the environment, and the need for their application at the right time (Mishra et al., Citation2018). Fortunately, fungicide application rates and frequency can be significantly reduced by using cultivar resistance or tolerance if available, especially where combined with accurate disease forecasting to ensure the best timing of application (Pandey et al., Citation2018).
C. Use of natural agents
Biological seed treatment is one of the sustainable levers to improve legumes productivity. A recent global meta-analysis showed that the yield gain potential due to biological seed treatment is higher in legumes compared to other field crops (Lamichhane et al., Citation2022). Combinations of different biological agents have shown the most promise in legume anthracnose management (Kumar et al., Citation2016). For example, seeds inoculated with Trichoderma species and Pseudomonas fluorescens reduced anthracnose incidence in-field by up to 80% in common bean (Amin et al., Citation2014; Sileshi et al., Citation2014) and blackgram (Chatak and Banyal, Citation2021). Similarly, biological control of three C. lindemuthianum races has been reported using P. chlororaphis with P. fluorescens (Bardas et al., Citation2009). Combined Rhizobium leguminosarum RPN5 + Bacillus sp. BPR7 + Pseudomonas sp. PPR8 has shown potential against a Colletotrichum sp. associated with anthracnose in common bean (Kumar et al., Citation2016). Foliar application of Trichoderma biocides also reduced anthracnose severity in soybean (Kale and Barhate, Citation2016), common bean (Padder et al., Citation2010; Amin et al., Citation2014), and cowpea (Adebanjo and Bankole, Citation2004) by up to 75%. The critical approaches needed for developing plant growth promoting rhizobacteria mixtures with improved biocontrol efficacy and stability under field conditions have been recently highlighted (Wang et al., Citation2021). Although Serratia marcescens has proved a promising against C. lindemuthianum, its validation under field conditions is required before recommendation (Papitha et al., Citation2020). Furthermore, aqueous extract of Lawsonia inermis L. (Kale and Barhate, Citation2016), Melia azedarach L. (Sharma et al., Citation2022), and Eucalyptus sp. (Chatak and Banyal, Citation2021) reduced anthracnose severity in soybean, bean, and blackgram up to 75–80%. Nevertheless, while low field efficacy and inconsistency have generally limited the adoption of microbial agents and natural products by legume growers, these constraints should be overcome in the future. Overall, next generation “fungicides” including those derived from active ingredients of plants offer significant potential toward more ecologically safe, efficient and minimal dose beneficial possibilities in combatting legume anthracnose diseases.
IX. Genetic approaches for anthracnose management
Reducing economic losses in legumes due to anthracnose through developing resistant cultivars offers the most cost-effective and efficient long term mitigation strategy (Boufleur et al., Citation2020). Several genetic approaches have been used to develop legume varieties resistant to anthracnose.
A. Search for genetic sources of resistance
Methods available for screening of legume germplasm against anthracnose include detached leaves, various glasshouse/greenhouse artificial inoculation methodologies, and evaluation under natural disease pressure. Disease screening can be particularly successful under field conditions with high disease pressure, or artificial inoculation with virulent pathogen isolates. Relative disease severities using these screening methods have been assessed using qualitative and quantitative disease rating scales (Pandey et al., Citation2021a).
The sources of genetic resistance identified against anthracnose in legumes are presented in . Anthracnose resistance in legumes has been identified mainly in cowpea, lentil, mungbean, lupins, blackgram, common bean, and soybean. However, research on sources of genetic resistance to anthracnose associated with chickpea, peanut, and pigeon pea has not yet been performed. Field/bush types of cowpeas have various levels of resistance to anthracnose compared to the vegetable/trailing types that are highly susceptible (Pradhan et al., Citation2018). Anthracnose resistant sources in cowpea were mainly from Nigeria and India and were also resistant against multiple foliar diseases, including Cercospora leaf spot, rust, bacterial pustule, and target spot (Williams, Citation1977). Cowpea genotype, IAR7/180-4-5 was also resistant against scab, and bacterial blight (Adebitan and Olufajo, Citation1998).
Table 2. Resistant genotypes of legumes against anthracnose.Table Footnote*)
Sources of anthracnose resistance in mungbean and blackgram were mostly identified from India (). In contrast, no breeding programs for varieties resistance to anthracnose were developed in other blackgram producing countries, such as Pakistan or Myanmar, despite large yield losses reported in these countries from Colletotrichum species. In such situations, the deployment of resistant varieties would greatly reduce production costs and allow reduced reliance on synthetic fungicides (Talhinhas et al., Citation2016). Therefore, the genetic diversity of mungbean presents significant opportunities for identifying anthracnose resistance sources within breeding programs. In the case of lentils, most screening programs have been conducted in Canada against specific and multiple races of Colletotrichum. Although India is the second largest lentil producer, its breeding program has not focused on anthracnose resistance. Perhaps this is due to anthracnose being a disease of relatively minor concern in India (Roy et al., Citation2022).
Few studies have been undertaken to identify sources of resistance to soybean. By inoculating 16 soybean accessions with C. truncatum isolates in Brazil, Dias et al. (Citation2019) found that while some soybean accessions had a high level of resistance in stems these same lines were highly susceptible to cotyledon infection. They hypothesized that stems and cotyledons likely had distinctly different genetic resistances with independent mechanisms of resistance. While different searches for sources of resistance in soybean against C. truncatum have been conducted (Costa et al., Citation2009; Nagaraj et al., Citation2014; Yang and Hartman, Citation2015; Dias et al., Citation2019), there has been no research to date on sources of resistance against other Colletotrichum species associated with soybean anthracnose. In contrast, many studies have been carried out to identify race specific sources of anthracnose resistance in common bean worldwide (). These genotypes represent significant potential donors of resistance genes in marker-assisted selection programs for transferring anthracnose resistance gene(s) into agronomically desirable susceptible genotypes.
Lupins offer great potential as an alternative protein source to soybeans since they have a similar protein content and better digestibility (Lucas et al., Citation2015). In Australia, New Zealand, Chile, and Germany, breeding efforts for lupins anthracnose resistance have been primarily conducted in the field (Cowling et al., Citation2000; Adhikari et al., Citation2009; Baer et al., Citation2009; Jacob et al., Citation2017). These efforts have identified anthracnose resistant genotypes, namely Ethiopian landraces P27174 and P27175 (Cowling et al., Citation2000; Adhikari et al., Citation2009). These two Ethiopian landraces form a distinct genetic group within white lupin (Raman et al., Citation2014). An F3-derived single-plant selection of a cross between an anthracnose-resistant landrace P27175 from Ethiopia and a well-adapted but highly susceptible Western Australian breeding line 89B10A-14 has led to the development of anthracnose resistant commercial white lupin cultivars. While studying anthracnose resistance in white lupin, Alkemade et al. (Citation2021a) found that under controlled conditions, stem inoculation-based disease phenotyping is a time-effective and appropriate method to identify field-relevant resistance. Further identification of resistance sources and their genetic components can now be achieved using this approach. However, blue lupin cultivars, such as Tanjil and Mandelup with strong anthracnose resistance under Australian conditions (Yang et al., Citation2008) proved susceptible in Germany (Fischer et al., Citation2015). Expression of plant resistance can vary widely across different inoculation methods, from environmental variation, and from difference in C. lupini strain-specific virulence (Rychel-Bielska et al., Citation2020). Further, variations in disease response in lupins and other legumes depend on several factors, including the source of pathogen isolation and virulence on different genotypes, and/or the nature of host resistance itself including whether it is governed by single or multiple genes.
B. Inheritance studies and genetic mapping
Studies on anthracnose inheritance have mainly focused on cowpea and common bean with much lower emphasis on lentil, blackgram, soybean, lupin and there have not been any studies on mungbean, chickpea, peanut, or pigeon pea. In general, anthracnose resistance in legumes is not governed by gene-for-gene hypothesis of qualitative/vertical resistance (Flor, Citation1971) where host resistance specificity is determined by the respective host R gene and pathogen avr gene interactions. Furthermore, anthracnose resistance in legumes has not been associated with a hypersensitive response. Rather, legume anthracnose is considered a complex quantitative trait controlled by multiple gene interactions that are highly influenced by environmental factors (Pradhan et al., Citation2018).
1. Cowpea, blackgram, and soybean
In cowpea, only one study showed that resistance is either dominant or polygenic in nature (Pradhan et al., Citation2018). A RAPD marker, OPAO2, and two ISSR markers (UBC810 and UBC811) co-segregated with anthracnose resistance genes in cowpea (Pradhan et al., Citation2018). In blackgram, resistance was controlled by a single dominant gene which was non-allelic (Kaushal and Singh, Citation1988; Bindra et al., Citation2016). Resistance to soybean anthracnose was governed by two major genes that interact in a complementary way (Nataraj et al., Citation2020). Transgenic soybean plants expressing the NmDef02 defensin gene were reported as having enhanced resistance against C. truncatum (Soto et al., Citation2020).
2. Common bean and lentil
In lentil, resistance to race 1 and race 0 of C. lentis was polygenic or oligogenic in nature (Bhadauria et al., Citation2017a). In common bean, most of the genes resistant to anthracnose are dominant except the Co-8 gene which is recessive (Kelly and Vallejo, Citation2004; Gonçalves-Vidigal et al., Citation2020). Over 20 anthracnose-independent resistance genes with multiple loci belonging to either the Mesoamerican or Andean gene pools have been identified in common bean (Vidigal Filho et al., Citation2020). The genes/QTLs and/or markers associated with anthracnose resistance in lentil and common bean have been summarized in . A few partially dominant genes (1-3) with an additive gene action conditioning resistance to anthracnose were also reported (Nkalubo, Citation2006). In a resistant cultivar JaloEEP558, an additional gene (KTR2/3) in the Co-x locus confers anthracnose resistance (Richard et al., Citation2021) as this gene encodes a truncated and chimeric CRINKLY4 kinase (CR4) located within a CRINKLY kinase cluster and its expression in leaves occurs following pathogen infection (Richard et al., Citation2018).
Table 3. Anthracnose related QTLs/markers in common bean and lentil.
Several single nucleotide polymorphisms (SNPs) associated with anthracnose resistance to races 3, 87, and 503 were located on Pv04 that encode leucine-rich repeat (LRR) and have typical NB-ARC domains (Banoo et al., Citation2020). A gene, Phvul.004G023900 encodes a methyltransferase for quantitative resistance against race 503 of pathogen on Chromosome (Chr) Pv04. Another SNP for resistance to race 503 was found on Chr Pv09 within gene model Phvul.009G169600, which encodes a zinc finger protein (Banoo et al., Citation2020). Quantitative resistance loci (QRLs) linked with race 73 were reported on linkage group Pv08 overlapping with the Co-4 gene. Additionally, the SNP within the gene Phvul.011G202300, which encodes a LRR within the NB-ARC domain, is also associated with resistance to race 73. For race 2047, different genomic regions on linkage groups Pv03, Pv09, and Pv11 were found to be linked with resistance in common bean (Banoo et al., Citation2020).
In the Andean Diversity Panel (ADP), major QTLs for anthracnose resistance were discovered on linkage groups Pv01 (races 65, 73, and 3481), Pv02 (races 39 and 55), and Pv04 (races 7, 109) and minor QTLs on Pv10 (race 7) and Pv11 (race 7) (Zuiderveen, Citation2015). Resistance on linkage group Pv01 to aforesaid races was associated with SNP ss715645251 within the gene Phvul.001G243800, which encodes for a LRR receptor-like protein kinase. On linkage group Pv02, resistance was significantly associated with SNP ss715648451 within gene Phvul.002G328300 which encodes a mitogen-activated protein kinase (MAPK). On linkage group Pv04, resistance was associated with SNPs ss715642306 (within gene Phvul.004G005800) and ss715649432 (within gene Phvul.004G006300), respectively. Both genes encode for cytochrome P450. The minor QTLs on Pv10 (race 7) for moderate resistance were linked to SNP ss715648754 within gene Phvul.010G025500, and on linkage group Pv11 (race 7) resistance was linked to SNP ss715645476 within gene Phvul.011G021500 (Zuiderveen, Citation2015). More recently, using recombinant inbred lines derived from Ruda × AND277 crossing, Lima et al. (Citation2023) mapped Co-14 allele in the cultivar AND277 using markers ss715645251 and BARCPVSSR01356 and reported two resistant genes, namely Phvul.001G243800 and Phvul.001G243900 within Co-14. Therefore, the linkage between Co-14 allele and markers ss715645351 and BARCPVSSR01356 will be essential for plant breeding programs to enable resistance genes to be transferred to elite cultivars via marker-assisted selection. Identifying and functionally analyzing candidate resistance genes in this region will help to develop accurate markers for anthracnose resistance, allowing for more efficient marker-assisted selection (Garzón et al., Citation2007; Ferreira et al., Citation2013; Vieira et al., Citation2018).
SNPs or SSRs (simple-sequence repeat) markers were discovered using genome wide association studies (GWAS) on 10 common bean chromosomes associated with anthracnose resistance (Perseguini et al., Citation2016; Zuiderveen et al., Citation2016). Using NBS (nucleotide-binding site)-SSR markers, Wu et al. (Citation2017) identified nine disease resistance loci for anthracnose, of which NSSR73, NSSR24, and NSSR265 were located at new Chr regions for anthracnose resistance. In addition, two markers NSSR271 and NSSR281 located on Chr 11, and NSSR24 on Chr 2 linked with resistance against anthracnose. Among these associated markers, in previous studies, NSSR65, NSSR8, NSSR234, NSSR117, NSSR281, and NSSR271 discovered for anthracnose resistance may be located at the same regions of the Chr (Campa et al., Citation2014; González et al., Citation2015; Perseguini et al., Citation2016; Zuiderveen et al., Citation2016).
In a meta-QTL (MQTL) investigation, information is assembled from numerous studies, and the confidence intervals are narrowed and better correlated with the results of individual studies. In several crops, MQTL analyses have been conducted for several traits, such as yield-related traits. In common bean, Shafi et al. (Citation2022) identified 11 MQTLs located in Chr 06, and 10 hotspots QTLs each including many QTLs from a specific study on Chr 07 against anthracnose. The same study identified 1,251 genes, including multiple R genes, such as those encoding for protein kinases and NBS-LRR domain-containing proteins, and various other defense related genes (Shafi et al., Citation2022). These MQTLs, hotspot QTLs, and potential candidate genes can be useful in marker-assisted breeding programs of common bean, in gene mapping, and in cloning of anthracnose resistance genomic regions.
3. Lupins
Inheritance studies involving lupin have been mainly conducted in Europe and Australia. Resistance is controlled by polygenic factors in a recombinant inbred line population of between P27174 and the susceptible cultivar Kiev Mutant (Phan et al., Citation2007; Yang et al., Citation2010). A similar case was reported for yellow lupin (Adhikari et al., Citation2011), while anthracnose resistance in blue lupin is governed by single dominant genes LanrBo, Lanr1, and AnMan (Yang et al., Citation2004, Citation2008; Fischer et al., Citation2015). The development of resistant cultivars was facilitated through marker-assisted selection using AnManM1 [later improved with Restriction-site Associated DNA (RAD) markers that are tightly linked to Larn1] (Yang et al., Citation2008). This AnManM1 marker is now being used for marker-assisted selection in the Australian lupin breeding program. Further, another anthracnose resistance gene, Llur, in yellow lupin has been reported (Haase and Ruge-Wehling, Citation2019). Based on linkage groups ALB10 (LG2), ALB02 (LG4), and ALB04 (LG17), QTL mapping of the recombinant inbred line population between P27174 and Kiev Mutant revealed three QTLs, antr04/05_1, antr04/05_2, and antr05_3, associated with resistance to anthracnose, which together accounted for 49% of the phenotypic variation observed in Western Australia in 2004 and 2005 (Phan et al., Citation2007; Yang et al., Citation2010).
With greater precision, using many SNP markers, Książkiewicz et al. (Citation2017) identified the same QTLs from genotyping-by-sequencing (Elshire et al., Citation2011). Even though white lupin and blue lupin share high synteny, anthracnose resistance QTLs in white lupin did not match with those in blue lupin (Książkiewicz et al., Citation2017). In addition to facilitating QTL mapping, genotyping-by-sequencing provided many markers that could be used for genomic selection. This is a method for constructing a statistical model of phenotypic and genotypic data of representative populations to envisage breeding ethos for a trait (polygenic) found in genetic resources/inbred lines (Heffner et al., Citation2009). According to a genomic prediction model, based on recombinant inbred line populations used to identify major QTLs, anthracnose resistance was predicted with a probability of 0.56, after considering variation not explained by the QTLs (Rychel-Bielska et al., Citation2020).
In Chile, by mapping major QTLs in syntenic regions, Lichtin et al. (Citation2020) found that alleles from the wild parent (CGNA’s Core 98 × AluProt-CGNA), explained phenotypic variation of 75% for anthracnose resistance. The marker sca82470 was highly linked with anthracnose resistance in yellow lupin and produced a hit of 90.9% identity on pseudo-Chr 11, 3,376,319–4,744,591 bp, where anthracnose resistance gene Lanr1 was localized. Accessions selected based on these QTLs failed to show enhanced resistance under either controlled or field conditions (Alkemade et al., Citation2021b). A high-throughput phenotypic system for field-relevant anthracnose resistance, as well as the availability of a reference genome for white lupin, allows in-depth genomic studies. A GWAS study with 181 white lupin accessions revealed two SNPs (Lalb_Chr05_2957940 and Lalb_Chr05_2957601) linked with resistance to anthracnose on SNP Lalb_Chr05_g021616 encoding a RING zinc-finger E3 ubiquitin ligase (Alkemade et al., Citation2022b). These genes can be tagged with allele sequences and PCR-markers in marker-assisted selection programs.
X. Omics approaches for anthracnose management
Many potential omics approaches can help unravel the molecular mechanisms of legume responses to Colletotrichum species thereby improving the detection and diagnosis of the causal agents (). The genome in each Colletotrichum species contains a unique set of genes from which numerous fungal phenotypes with varying virulence can be elucidated genetically by population genomic studies of a large number of loci (Sarrocco et al., Citation2020). Fungal pathogen genes involved in host-specific interactions can be identified by utilizing techniques, such as GWAS, QTL mapping, and genome scans for selective sweeps and selection signatures (Plissonneau et al., Citation2017).
Figure 3. Schematic explanation of omics approaches used in future projects in the improvement of resistance/tolerant to legume anthracnose (PPI: protein–protein interaction; DIGE: differential gel electrophoresis; GC-MS: gas chromatography-mass spectrometry).
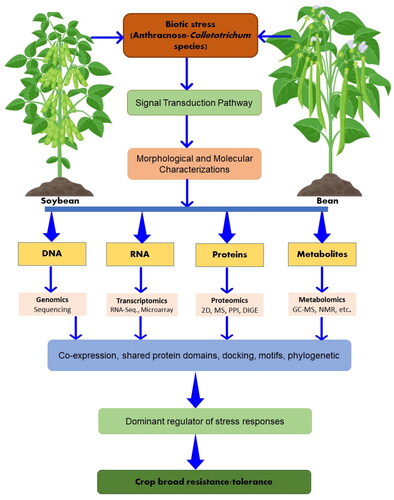
A transcriptome is composed of all the coding and non-coding RNAs, whereas a proteome is composed of the proteins derived from a genome (Pandey et al., Citation2021b). Conversely, the metabolome is all the metabolites existing in the plant/microbe system. Through transcriptomic and genomics methods, it is not possible to study the defense systems of leguminous plants against anthracnose pathogens. This is because these defense systems not only involve the expression of multiple genes but also the accumulation of post-translational modifications or metabolites affecting the expression of the final gene products. However, data from genomes and transcriptomes can be used in conjunction with proteomics and metabolomics to gain insight into gene expressions by proteins and metabolites. Even though these approaches are complex, they can be used to understand how plants respond to fungal pathogens and also to associated beneficial microbes. In addition, metagenomics approaches enable a deeper understanding of the microorganisms associated with plants, potentially opening up the way for new possibilities for large-scale legume production.
A. Genomics studies of hosts and pathogens
To date, draft genomes of Colletotrichum species causing soybean anthracnose (Rogério et al., Citation2020) have been sequenced. These include the following species: C. plurivorum (IMI 507127), C. truncatum (IMI 507125), C. sojae (IMI 507126), and C. musicola (IMI 507128). A draft genome of two isolates of C. lindemuthianum infecting common bean with a genome size of 97.4 Mbp is also available (Queiroz et al., Citation2017). Furthermore, the genome of two other C. truncatum strains, viz. TYU (Taxus cuspidate) and MTCC 3114 (Capsicum annuum), are available in the GenBank database (Gan et al., Citation2017; Rao and Nandineni, Citation2017; Rogério et al., Citation2020). Concerning C. gloeosporioides, a draft genome is available from the hosts Chinese fir (QFRH00000000, PRJNA471237, and SAMN09205517; Huang et al., Citation2019) and avocado (Alkan et al., Citation2013), with genome size 61.9 and 53.2 Mbp, respectively. The genome of C. lentis is 56.10 Mb in size and consists of 10 cores and two minichromosomes (Bhadauria et al., Citation2019). Besides, the full genome sequence of C. lupini is 63.41 Mb in size (Baroncelli et al., Citation2021). The genome assembly data of Colletotrichum species causing anthracnose in legumes is provided in . With the availability of genome sequences, numerous studies can be conducted, including comparative genomic analyses that characterize effector repertoires, which likely play a role in host-pathogen interactions and directly influence several disease management strategies (de Queiroz et al., Citation2017).
Table 4. Summary of Colletotrichum spp. pathogenic to legumes and other crop that have whole genome sequences.
A significant number of legume species have sequenced genomes available in GenBank database, and here we summarize a few of these resources based on their genome sizes. Whole genome sequences are available for mungbean (Kang et al., Citation2014; Ha et al., Citation2021; ∼431 Mb), blackgram (Jegadeesan et al., Citation2021, ∼475 Mb), soybean (Schmutz et al., Citation2010, 1,115 Mb), chickpea (Varshney et al., Citation2013, ∼738 Mb), pigeon pea (Varshney et al., Citation2011; ∼605.78 Mb), lentil (Arumuganathan and Earle, Citation1991; ∼4 Gbp), peanut (Bertioli et al., Citation2019; ∼2.54 Gb), narrow-leafed lupin (Hane et al., Citation2017; ∼609 Mb), white lupin (Hufnagel et al., Citation2020, ∼451 mm), and cowpea (Lonardi et al., Citation2019; ∼519 Mb). In addition, pan genome sequences are also available for narrow-leafed lupin (Garg et al., Citation2022, ∼975 Mb, Xu et al., Citation2020, ∼559 Mb), white lupin (Hufnagel et al., Citation2021; ∼14.9 Gb), and 26 wild and cultivated soybeans (Liu et al., Citation2020; ranging ∼992.3–1059.8 Mb). The genome sequences of these legumes are useful in unraveling the plant defense system against Colletotrichum species. It effectively identifies candidate genes accountable for the virulence of Colletotrichum species and allows targeting of potential genes in legumes that confer resistance. The available genomes of both legumes and Colletotrichum species will together be useful for legume breeders in developing new cultivars with durable anthracnose resistance through either alteration in the pathogen’s virulence genes or through selection of defense-related genes in the host crop.
Despite the lack of breeding programs on anthracnose resistance in mungbean, blackgram, soybean, cowpea, peanut, pigeon pea, or chickpea, the available genomic resources should still support development of future breeding programs. However, the availability of the whole genome sequences of Colletotrichum species affecting legumes will not only be very useful for developing diagnostic tools for an early and accurate detection of Colletotrichum species (Klosterman et al., Citation2016), but they will also enable new insights into pathogenesis processes at the molecular level. Together, they will contribute to the development of more efficient and innovative approaches to combat the pathogen in the legume–anthracnose pathosystem along with enlightening our knowledge of host–fungal interactions.
B. Transcriptomics studies
Multi-omics methods allow the discovery of resistant genes and analysis of molecular defense mechanisms for legume diseases including anthracnose. Plant pathogen interactions can be studied by RNA sequencing, allowing the recognition of genes and pathways involved in various phases of plant defense (Ranathunge et al., Citation2009). For instance, an integrated analysis of metabolomics and transcriptomics revealed the regulation of primary metabolism in soybean crops in response to anthracnose (Zhu et al., Citation2022). The R genes, RPP13, PTI1, RGA2, ULP2B, RPS6, and PR genes, such as PR14 (lipid transfer proteins) and CHI (chitinase), provide enhanced resistance in cultivar ZC-2 than cultivar ZC-3 (Zhechun No. 3) to C. truncatum (Zhu et al., Citation2022). The gene RGA2 also confers partial resistance to common bean anthracnose (López et al., Citation2003). Among these genes, RPS6 provides resistance in Arabidopsis by regulating the MAPK signaling pathway (Takagi et al., Citation2020). However, the role of the ULP2B gene, which was differentially expressed in resistant soybean cultivar ZC-2, remains still unclear that needs future investigation.
Other factors enhancing resistance in soybean cultivar ZC-2 against anthracnose include transcription factors (TFs), such as WRKY and bHLH, signal transduction mediated through auxin (AUX), jasmonic acid (JA), MAPK, and Ca2+ signaling, and strong terpenoid metabolism at the right time (Zhu et al., Citation2022). JA, MAPK, AUX, and Ca2+ signaling have been identified as plant defense response regulators (Aldon et al., Citation2018; Fan et al., Citation2020). In a resistant soybean mutant where pods are challenged with C. truncatum, an overexpression of Ca2+ fluctuation and plant hormone signaling genes was also reported (Boufleur et al., Citation2022; Zhu et al., Citation2022). Further, there was extensive cross-talk between these signaling pathways, including examples of MAPK-WRKY (Wang et al., Citation2018), MAPK and JA signaling (Liu et al., Citation2011), AUX and JA signaling (Kazan and Manners, Citation2009; Naseem et al., Citation2015), as well as Ca2+ and JA and signaling (Lv et al., Citation2019). These signaling pathways interact synergistically to mediate plant defense responses. The cross-talk between WRKY TFs and AUX signaling as well as Ca2+ signaling and WRKY TFs may occur during the interaction of soybean–C. truncatum, although the underlying mechanism is not yet clear.
In particular, a plant’s response to pathogen infection is regulated by WRKY TFs by modulating the expression of camalexin and resistance-associated gene synthesis (Jiang et al., Citation2017). Thus, several WRKY TFs contribute to the positive regulation of the expression of presumed defense genes during infection by pathogens as reported in case of soybean. For example, Boufleur et al. (Citation2022) identified seven WRKY TFs overexpressed only in the more resistant soybean genotypes Gm1-1080; Gm2-1059; two of them, WRKY23 and WRKY12, were time-specific. The WRKY12 encoding gene has been found down- and upregulated in more resistant and susceptible soybean genotypes, respectively, at the same time interval following C. truncatum infection (Zhu et al., Citation2022). In soybean, the biosynthesis of glyceollin (phytoalexin) is positively regulated by GmNAC42, a defensive metabolite that is involved in systemic acquired resistance (Jahan et al., Citation2019). Further, overexpression of GmNAC42 in soybean pods has been observed following C. truncatum infection (Zhu et al., Citation2022).
In a susceptible bean genotype, four WRKY TFs were upregulated and the pattern of expression increased over the time during C. lindemuthianum infection (Padder et al., Citation2016). A few WRKY TFs have been associated with negative regulation of defense signaling thereby increasing the hosts’ susceptibility toward anthracnose (Kim et al., Citation2006; Pandey and Somssich, Citation2009), corroborating the findings by Padder et al. (Citation2016). Tissue specific expression of WRKY TFs has been reported in common bean, and while these are dispersed across all chromosomes of bean the expression of most of these was found in roots, with few in leaves, over different time courses (Wang et al., Citation2016). Taken together, the up-regulation of WRKY TFs may account for the host’s susceptibility.
The importance of many signaling hormones, such as JA, indole acetic acid (IAA), ethylene, and salicylic acid (SA), in disease resistance activation is well-studied (Verma et al., Citation2016). Zhu et al. (Citation2022) found that in a more resistant soybean genotype both JA and IAA hormones responded more strongly and were associated with enhanced resistance of a more susceptible cultivar to C. truncatum following its treatment with these hormones (Zhu et al., Citation2022). Similarly, up-regulation of the AP2 and ethylene responsive TFs were reported in an anthracnose susceptible common bean genotype (Padder et al., Citation2016). This suggests that TFs play a key role in JA and SA mediated pathways that contribute to acquired resistance, as shown by this suppression of resistance due to pattern-triggered immunity in more susceptible bean genotypes. Other TFs, such as bZIPs and kinase defense genes, have shown potential response toward increased anthracnose resistance in common bean (Padder et al., Citation2016).
The role of more than 50 NBS genes in disease resistance in legumes has been identified (Sekhwal et al., Citation2015). Among 171 NBS genes reported in common bean, 67 genes showed differential expression levels for anthracnose between resistant and susceptible genotypes. More upregulated (48) than downregulated genes (19) were found in susceptible common bean genotype Jingdou as compared with the resistant genotype Hongyundou (Wu et al., Citation2017). While gene Phvul.010G054400 normally shows higher expression in a resistant cultivar, gene expression sharply declined following infection by pathogen race 81. In contrast, there was no change in expression in the susceptible genotype, confirming that this gene may act as a negative regulator of anthracnose resistance during host-pathogen interaction, thus enhancing the host’s susceptibility. These studies have contributed significant new understanding of common bean NBS genes, especially in relation to diseases.
Padder et al. (Citation2016) identified 3,250 DEGs with and between the near isogenic lines of common bean, with more DEGs upregulated in resistant lines during necrotrophic phase than biotrophic phase. The genes that help in the regulation of anthracnose in common bean include those encoding peroxidase, lipoxygenases, and PR proteins. In another study, PvPR1, NPR1, FLS2, and β-GLUC genes overexpressed in a resistant common bean genotype after inoculation with race 65 of C. lindemuthianum, suggesting that these genes have a crucial role in the common bean defense system against this pathogen (da Silva et al., Citation2021). Another regulator gene, pacCl which encodes the TF PacC of C. lindemuthianum, also showed a differential response in biotrophic as compared with the necrotrophic phase (Soares et al., Citation2014).
In lentil, a complex interaction between effector genes and resistance has been found for the development of anthracnose disease (Bhadauria et al., Citation2017b). Overall, 26 resistance genes have been identified in this host including dirigent (a resistance response protein) and a suppressor of npr1-1, constitutive 1 (NBS-LRR) following infection by a C. lentis virulent isolate of race 0 (Bhadauria et al., Citation2017b). Several anthracnose resistance genes in lentil are also antagonistic defense signaling pathway markers, including PR5 (SA), PR1, and PR4 (JA) (Bhadauria et al., Citation2013a). In anthracnose resistance, these genes can be positive or negative regulators of plant immunity. Cross-talk between signaling pathways permits plants to activate defense responses common to tackling diverse groups of pathogens, including biotrophic and necrotrophic pathogens. A resistance gene encoding a CC-NBS-LRR R protein was differentially expressed in a partial resistance lentil genotype, CDC Robin race 1 during infection. This gene is involved in host resistance after invasion (Bhadauria et al., Citation2013a). By analyzing ESTs, Bhadauria et al. (Citation2013b) discovered two effectors, CtToxB and CtNUDIX, and showed that CtToxB amplifies cell death signals to accommodate colonization of C. lentis whereas induction of CtNUDIX results in abrupt cell death (Bhadauria et al., Citation2015). Defense response against anthracnose in narrow-leafed lupin was linked with upregulation of CC-NBS-LRR, TIR-NBS, and NBS-LRR genes, and 10 pathogenesis-related proteins, glucan endo-1,3-beta-glucosidases, lipid transfer proteins, genes from ROS pathway and glycine-rich cell wall proteins (Książkiewicz et al., Citation2022).
The pathogenicity mechanism in C. lupini infecting white lupin has been identified by using transcriptomic and proteomic approaches (Dubrulle et al., Citation2020). That study revealed 897 genes in white lupin that were differentially expressed within 24–84 h following C. lupini inoculation. Of these, 520 genes had putative roles in coding for “pathogenicity factors” like effectors, enzyme-biosynthetic genes, and transporters. In addition, more than 300 putative pathogenesis-related proteins were identified by mass spectrometry (Dubrulle et al., Citation2020). Despite such progress in pathogenicity characterization, little is known about the exact molecular pathway and this constitutes an area needing further research (Dubrulle et al., Citation2020).
C. Metabolomics and proteomics studies
While several studies characterized a subset of metabolites in response to anthracnose, comprehensive metabolomic studies that are applied to disease and pest resistance are still lacking. Pathways involving flavonoid, phenylpropanoid, amino acid, isoflavonoid, terpenoid metabolism, and carbohydrate were adjusted in response to soybean infection by C. truncatum (Zhu et al., Citation2022). The soybean resistance mechanism after infection with C. truncatum might be influenced by flavonoids, phenylpropanoids, and isoflavonoids where, at all-time points, cultivars ZC-2 and ZC3 were highly enriched in the “flavonoid biosynthesis,” “phenylpropanoid biosynthesis,” and “isoflavonoid biosynthesis” pathways. Additionally, other studies demonstrated that these pathways increase host resistance to anthracnose (Wang et al., Citation2016, Citation2020; Chakraborty et al., Citation2019; Botero et al., Citation2021; Jiang et al., Citation2021). While these roles were likely determined by amino acid and carbohydrate metabolism during soybean–C. truncatum interaction, more focused studies are needed to clarify their specific roles. Numerous studies have investigated the phenylpropanoid pathway and biosynthesis of isoflavonoids. The latter contains numerous defense-related compounds, strongly indicating that flavonoid-related compounds are prominently involved in the defense mechanisms of narrow-leafed lupin × C. lupini (Muth et al., Citation2009; Wojakowska et al., Citation2013, Citation2015), soybean × C. gloeosporioides (Lee et al., Citation2013), and Stylosanthes × C. gloeosporioides (Jiang et al., Citation2021), with several examples demonstrating their potential to increase disease resistance levels by genetic transformation (Lozovaya et al., Citation2005). A TF called RAP2-7 regulates the biosynthesis of quinolizidine alkaloids in narrow-leafed lupin (Czepiel et al., Citation2021). The flavor of soybean products is derived from glycitein that exhibited antibacterial and fungicidal effects in studies with C. gloeosporioides (Lee et al., Citation2013). Taken together, all these studies highlight how secondary metabolites act as defense agents in legumes against anthracnose.
To date, only a single study has provided proteomic data on the legume-Colletotrichum pathosystem. By using two-dimensional electrophoresis and mass spectrometry, infected common beans were found to accumulate 17 proteins that were related to the following cellular processes: photosynthesis, antioxidants, carbon metabolism, defense, genetics, protein folding, stress response, and biosynthesis of phenylpropanoid and flavonoid compounds (Borges et al., Citation2015). The accumulation of PR1 protein and antioxidant proteins in infected plants confirms the findings of transcriptomic studies. In addition, chalcone isomerase, an enzyme involved in generating cell wall compounds, was over-accumulated at the beginning of the necrotrophic phase (>48 h post-inoculation). Results at the transcript level are similar to those reported in the previous study of Oblessuc et al. (Citation2012). A combination of transcriptomic and comparative proteomic approach to legume resistance in the future will allow a much better understanding of how protein activity is regulated in response to biotic stress, which will be essential for identifying resistance proteins. Consequently, metabolomics will play an increasingly important role in legume breeding programs in the coming years, as a tool for selection and validation to increase the breeding efficiency and shorten the time needed to develop new varieties.
XI. Conclusion and future remarks
Anthracnose is a very destructive disease of legumes worldwide. Unlike many other legume diseases, developing genetic resistance or determining the underlying mechanisms of host resistance to anthracnose are both challenging and complex as disease resistance in legumes does not follow the gene-for-gene interaction model. Except for a few legumes like the common bean, lentil, lupins, blackgram, and soybean, in the majority of anthracnose-affected legumes, no major resistance gene and QTLs have been identified and there is no race specificity among the pathogen populations. Further, many factors, such as plant type, plant physiological maturity, growth habit, and pod load affect the host response to anthracnose. Sources of genetic resistance to anthracnose have been identified worldwide within the common bean, cowpea, mungbean, blackgram, soybean, lentil, and lupins, and have been exploited in classical breeding programs. However, there remains much scope and need for further investigation into genetic resistance to anthracnose in chickpea, peanut, and pigeon pea. The limited success of traditional breeding for improved anthracnose resistance is due to many factors, including various Colletotrichum species disease complexes, the polygenic nature of the resistance, the complex interactions between expression of resistance with other physiological and morphological features, the pathogen variability, the difficulties in maintaining resistance through successive generations of backcrossing, the lack of sufficient correlation between glasshouse and field screenings, the inability to directly identify and select for resistance genes, as well as the confounding effects of other foliar diseases during phenotypic screening.
From a taxonomic, biological, and ecological perspective, it is crucial to know the exact identities and diversity of different pathogens within an anthracnose complex and the diversity within each individual pathogen population. Accurate pathogen identity has major and direct implications for disease resistance breeding programs as well as the management of diseases by cultural and chemical approaches. This equally helps distinguishing between emerging and established fungal populations associated with legumes, to detect, limit the spread of, and effectively manage new Colletotrichum species. C. truncatum, C. lindemuthianum, and C. lentis are the most prevalent species infecting the majority of legumes. This review highlights at least 11 Colletotrichum lineages associated with soybean, with C. truncatum and C. orchidearum species complex having the broadest distribution worldwide and the greatest impact. Most of the available literature on soybean, mungbean, and blackgram anthracnose until now has been limited to C. truncatum, on common bean to C. lindemuthianum, on lentil to C. lentis, and on lupins to C. lupini. Considering all the Colletotrichum species that cause anthracnose, there remains a significant paucity of knowledge about critical aspects of their epidemiology, worldwide distribution, identification, control measures, fungicide efficiency, and genetic resistance. There remains a critical need for robust genomic sampling to fully understand relationships within and between taxa in the genus Colletotrichum, as well as to distinguish species-within-species complexes. While genome data are now available for several Colletotrichum species associated with anthracnose in legumes, there remains a need for phylogenomic studies of the genus to be conducted. Through population genomics and comparative genomics, we can identify genes that contribute to pathogenicity, virulence (or aggression), host specialization, fungicide resistance, and adaptability to different environments, with increased precision, enabling us to gain a deeper understanding of all aspects of Colletotrichum species dynamics, including within the various agroecosystems where anthracnose-susceptible legumes are deployed.
Among the available mitigation approaches, resistance breeding is the most effective, inexpensive, farmer friendly, and ecologically sound approach. Improved crop resistance is vital for sustainable legume production, and can be achieved through many different approaches ranging from traditional breeding to omics interventions. Recently, new sources of anthracnose resistance have been identified within legumes, which have been utilized extensively for identifying resistance QTLs and developing cultivars with improved resistance. The preliminary results from these studies have been encouraging, suggesting the possibility of developing commercially acceptable cultivars with good levels of anthracnose resistance. However, there is a need to further validate and refine these identified genetic bases to enable their wider more effective use in marker-assisted breeding of legumes. To improve breeding efficiency, a more detailed understanding of host-pathogen interactions, particularly of the operation and efficiencies of resistance mechanisms at genetic and molecular levels by implementing the omics interventions, is required.
Although the development of genomic resources in legumes against anthracnose has been relatively slow compared to other food crops, such as vegetables, oilseeds, and cereals, the extensive applicability of marker assisted selection has already been established in common bean, lentil, and soybean, and to a much lesser extent in cowpea, lupins, and pea, but they are still in an early infancy stage for most other legumes prone to anthracnose. Nevertheless, it is encouraging that potential research progress has already been made in engendering sufficient genomic resources in all the major legumes prone to anthracnose. The recent progress of phenotyping at larger scale, sequencing of the whole genome, genetic analysis, and expression of metabolites and proteins, will be helpful in further deciphering legumes-Colletotrichum interactions and recognizing potential resistance elements in economically important legume crops prone to anthracnose.
In recent years, the CRISPR/Cas approach has been used to enhance disease resistance among different crops through genome editing of hosts, a broad range of related necrotrophic fungi, and particularly C. truncatum (Mishra et al., Citation2021). However, to the best of our knowledge, CRISPR/Cas has not been used in legumes against anthracnose by legume breeders. This approach can be utilized to engender a wide range of genetic diversity for legume breeding that can more easily enable plant breeders to develop advanced cultivars resistant to anthracnose. However, the potential application of these new genome editing tools including CRISPR/Cas in legumes may be impeded in some instances by a lack of an efficient plant regeneration protocol from callus culture. As the world faces synchronized challenges of climate change and rapidly increasing population, there is an urgent need to use a range of breeding and biotechnological tools to develop climate-resilient cultivars. To this aim, this review has made a first attempt to convey current knowledge gaps and has highlighted critical research needs based on previous and ongoing efforts in the study of anthracnose resistance in legumes across various disciplines, especially fields of genomics, transcriptomics, metabolomics, proteomics, and genome editing.
Disclosure statement
No potential conflict of interest was reported by the author(s).
Additional information
Funding
References
- Adam-Blondon, A. F., Sévignac, M., Bannerot, H., and Dron, M. 1994. SCAR, RAPD and RFLP markers linked to a dominant gene (Are) conferring resistance to anthracnose in common bean. Theor. Appl. Genet. 88: 865–870. doi:10.1007/BF01253998
- Adebanjo, A., and Bankole, S. A. 2004. Evaluation of some fungi and bacteria for biocontrol of anthracnose disease of cowpea. J. Basic Microbiol. 44: 3–9. doi:10.1002/jobm.200310310
- Adebitan, S. A., and Olufajo, O. O. 1998. Field evaluation of cowpea (Vigna unguiculata) varieties for grain and fodder production and for multiple disease resistance in Nigeria. Indian J. Agric. Sci. 68: 152–154.
- Adhikari, K. N., Buirchell, B. J., Thomas, G. J., Sweetingham, M. W., and Yang, H. 2009. Identification of anthracnose resistance in Lupinus albus L. and its transfer from landraces to modern cultivars. Crop Pasture Sci. 60: 472–479. doi:10.1071/CP08092
- Adhikari, K. N., Thomas, G., Buirchell, B. J., and Sweetingham, M. W. 2011. Identification of anthracnose resistance in yellow lupin (Lupinus luteus L.) and its incorporation into breeding lines. Plant Breed 130: 660–664. doi:10.1111/j.1439-0523.2011.01880.x
- Aggarwal, S. K., Mali, B. L., Rajput, L. S., and Choudhary, M. 2017. Epidemiology of anthracnose of black gram caused by Colletotrichum lindemuthianum. Int. J. Agric. Sci. 9: 975–3710.
- Aggarwal, S. K., Mali, B. L., Trivedi, A., Bunker, R. N., Rajput, L. S., Kumar, S., and Tripathi, A. 2019. Host plant resistance in different black gram cultivars against anthracnose. Int. J. Curr. Microbiol. Appl. Sci. 8: 571–575. doi:10.20546/ijcmas.2019.803.069
- Aldon, D., Mbengue, M., Mazars, C., and Galaud, J. P. 2018. Calcium signalling in plant biotic interactions. Int. J. Mol. Sci. 19: 665. doi:10.3390/ijms19030665
- Alkan, N., Meng, X., Friedlander, G., Reuveni, E., Sukno, S., Sherman, A., Thon, M., Fluhr, R., and Prusky, D. 2013. Global aspects of pacC regulation of pathogenicity genes in Colletotrichum gloeosporioides as revealed by transcriptome analysis. Mol. Plant. Microbe Interact. 26: 1345–1358. doi:10.1094/MPMI-03-13-0080-R
- Alkemade, J. A., Arncken, C., Hirschvogel, C., Messmer, M. M., Leska, A., Voegele, R. T., Finckh, M. R., Kölliker, R., Groot, S. P. C., and Hohmann, P. 2022a. The potential of alternative seed treatments to control anthracnose disease in white lupin. Crop Prot. 158: 106009. doi:10.1016/j.cropro.2022.106009
- Alkemade, J. A., Baroncelli, R., Messmer, M. M., and Hohmann, P. 2023. Attack of the clones: population genetics reveals clonality of Colletotrichum lupini, the causal agent of lupin anthracnose. Mol. Plant Pathol. 24: 616–627. doi:10.1111/mpp.13332
- Alkemade, J. A., Messmer, M. M., Arncken, C., Leska, A., Annicchiarico, P., Nazzicari, N., Książkiewicz, M., Voegele, R. T., Finckh, M. R., and Hohmann, P. 2021a. A high-throughput phenotyping tool to identify field-relevant anthracnose resistance in white lupin. Plant Dis. 105: 1719–1727. doi:10.1094/PDIS-07-20-1531-RE
- Alkemade, J. A., Messmer, M. M., Voegele, R. T., Finckh, M. R., and Hohmann, P. 2021b. Genetic diversity of Colletotrichum lupini and its virulence on white and Andean lupin. Sci. Rep. 11: 13547. doi:10.1038/s41598-021-92953-y
- Alkemade, J. A., Nazzicari, N., Messmer, M. M., Annicchiarico, P., Ferrari, B., Voegele, R. T., Finckh, M. R., Arncken, C., and Hohmann, P. 2022b. Genome-wide association study reveals white lupin candidate gene involved in anthracnose resistance. Theor. Appl. Genet. 135: 1011–1024. doi:10.1007/s00122-021-04014-7
- Almeida, C. P. D., Carvalho Paulino, J. F. D., Barbosa, C. C. F., Moraes Cunha Goncalves, G. D., Fritsche-Neto, R., Carbonell, S. A. M., Chiorato, A. F., and Benchimol-Reis, L. L. 2021. Genome-wide association mapping reveals race-specific SNP markers associated with anthracnose resistance in carioca common beans. PLOS One 16: e0251745. doi:10.1371/journal.pone.0251745
- Altaf, R., Naz, F., Rauf, C. A., and Shah, M. K. N. 2018. The scenario of lentil anthracnose in the Punjab, Pakistan. Pak. J. Bot. 50: 407–413.
- Alzate-Marin, A. L., Baía, G. S., Paula, T. J. D., Carvalho, G. A. D., Barros, E. G. D., and Moreira, M. A. 1997. Inheritance of anthracnose resistance in common bean differential cultivar AB 136. Plant Dis. 81: 996–998. doi:10.1094/PDIS.1997.81.9.996
- Alzate-Marin, A. L., Baia, G. S., Paula, T. J., De Souza, K. A., De Barros, E. G., and Moreira, M. A. 2001. Inheritance of anthracnose resistance in the common bean differential cultivar G 2333 and identification of a new molecular marker linked to the Co-42 gene. J. Phytopathol. 149: 259–264. doi:10.1046/j.1439-0434.2001.00612.x
- Alzate-Marin, A. L., Souza, K. A. D., Morais Silva, M. G. D., Oliveira, E. J. D., Moreira, M. A., and Barros, E. G. De. 2007. Genetic characterization of anthracnose resistance genes Co-43 and Co-9 in common bean cultivar tlalnepantla 64 (PI 207262). Euphytica 154: 1–8. doi:10.1007/s10681-006-9253-x
- Amin, M., Fitsum, S., Thangavel, S., and Negeri, M. 2014. Field management of anthracnose (Colletotrichum lindemuthianum) in common bean through fungicides and bioagents. Adv. Crop Sci. Technol. 2: 1–8.
- Amusa, N. A. 1994. Production, partial purification and bioassay of toxic metabolites of three plant pathogenic species of Colletotrichum in Nigeria. Mycopathologia 128: 161–166. doi:10.1007/BF01138478
- Amusa, N. A., Ikotun, T., and Osikanlu, Y. O. K. 1994. Screening cowpea and soybean cultivars for resistance to anthracnose and brown blotch diseases using phytotoxin metabolites. Afr. Crop Sci. J. 2: 221–224.
- Anand, T., Bhaskaran, R., Raguchander, T., Karthikeyan, G., Rajesh, M., and Senthilraja, G. 2008. Production of cell wall degrading enzymes and toxins by Colletotrichum capsici and Alternaria alternata causing fruit rot of chillies. J. Plant Prot. Res. 48: 437–451. doi:10.2478/v10045-008-0053-2
- Anitha, K., Kumar, G. S., Abraham, B., and Chakrabarty, S. K. 2020. Interception of Colletotrichum lindemuthianum (Sacc. & Magn.) Bri. & Cav. on sunflower seed from Argentina, a new host record. Ann. Plant. Prot. Sci. 28: 190. doi:10.5958/0974-0163.2020.00050.6
- Ankur, J., Shashi, T., Sunil, Z., and Sobita, S. 2012. First report of anthracnose disease on groundnut caused by Colletotrichum dematium from Allahabad (Uttar Pradesh) in India. Int. J. Agric. Sci. 8: 465–467.
- Arumuganathan, K., and Earle, E. D. 1991. Nuclear DNA content of some important plant species. Plant Mol. Biol. Rep. 9: 208–218. doi:10.1007/BF02672069
- Awa, O. C., Samuel, O., Oworu, O. O., and Sosanya, O. 2012. First report of fruit anthracnose in mango caused by Colletotrichum gloeosporioides in Southwestern Nigeria. Int. J. Sci. Technol. Res. 1: 30–34.
- Ayana, G., and Fininsa, C. 2018. Progresses in diseases management research in lowland food legumes of Ethiopia: A review. Ethiop. J. Crop Sci. 6: 329–341.
- Baer, E., von Baer, I., and von Riegel, R. 2009. Pecosa-Baer: a new cultivar of new cultivar cultivar of white lupin with determined bushy growth habit, sweet grain and high protein content. Chil. J. Agric. Res. 69: 577–580.
- Bahl, P. N. 2015. Climate change and pulses: approaches to combat its impact. Agric. Res. 4: 103–108. doi:10.1007/s40003-015-0163-9
- Bailey, J. A., Nash, C., O’Connell, R. J., and Skipp, R. A. 1990. Infection process and host specificity of a Colletotrichum species causing anthracnose disease of cowpea, Vigna unguiculata. Mycol. Res. 94: 810–814. doi:10.1016/S0953-7562(09)81382-8
- Banniza, S., Warale, R., Menat, J., Cohen-Skali, A., Armstrong-Cho, C., and Bhadauria, V. 2018. The long path to understanding the host–pathogen interactions of Colletotrichum lentis on lentil. Can. J. Plant Pathol. 40: 199–209. doi:10.1080/07060661.2018.1451391
- Banoo, A., Nabi, A., Rasool, R. S., Mahiya-Farooq, Shah, M. D., Ahmad, M., Sofi, P. A., Aasiya-Nabi, Itoo, H., Sharma, P. N., and Padder, B. A. 2020. North-Western Himalayan common beans: population structure and mapping of quantitative anthracnose resistance through genome wide association study. Front. Plant Sci. 11: 571618. doi:10.3389/fpls.2020.571618
- Barbieri, M. C. G., Ciampi-Guillardi, M., Moraes, S. R. G., Bonaldo, S. M., Rogério, F., Linhares, R. R., and Massola, N. S. 2017. First report of Colletotrichum cliviae causing anthracnose on soybean in Brazil. Plant Dis. 101: 1677–1677. doi:10.1094/PDIS-07-16-0963-PDN
- Bardas, G. A., Lagopodi, A. L., Kadoglidou, K., and Tzavella-Klonari, K. 2009. Biological control of three Colletotrichum lindemuthianum races using Pseudomonas chlororaphis PCL1391 and Pseudomonas fluorescens WCS365. Biol. Control 49: 139–145. doi:10.1016/j.biocontrol.2009.01.012
- Barilli, E., Moral, J., Aznar-Fernández, T., and Rubiales, D. 2020. Resistance to anthracnose (Colletotrichum lentis, race 0) in Lens spp. germplasm. Agronomy 10: 1799. doi:10.3390/agronomy10111799
- Baroncelli, R., Pensec, F., Da Lio, D., Boufleur, T., Vicente, I., Sarrocco, S., Picot, A., Baraldi, E., Sukno, S., Thon, M., and Le Floch, G. 2021. Complete genome sequence of the plant-pathogenic fungus Colletotrichum lupini. Mol. Plant. Microbe Interact. 34: 1461–1464. doi:10.1094/MPMI-07-21-0173-A
- Barzman, M., Bàrberi, P., Birch, A. N. E., Boonekamp, P., Dachbrodt-Saaydeh, S., Graf, B., Hommel, B., Jensen, J. E., Kiss, J., Kudsk, P., Lamichhane, J. R., Messéan, A., Moonen, A. C., Ratnadass, A., Ricci, P., Sarah, J. L., and Sattin, M. 2015. Eight principles of integrated pest management. Agron. Sustain. Dev. 35: 1199–1215. doi:10.1007/s13593-015-0327-9
- Basandrai, A. K., Gartan, S. L., Basandrai, D., and Kalia, V. 1999. Blackgram (Phaseolus mungo) germplasm evaluation against different diseases. Indian J. Agric. Sci. 69: 506–508.
- Basandrai, A. K., Sharma, V., Katoch, A., Basandrai, D., and Sharma, P. N. 2016. Genetic diversity of Colletotrichum truncatum infecting urdbean (Vigna mungo) in Himanchal Pradesh. Indian Phytopath. 69: 386–390.
- Basandrai, D., Basandrai, A. K., Singh, I., and Kalia, V. 2003. Multiple disease resistance against anthracnose, cercospora leaf spot, powdery mildew and mungbean yellow mosaic virus in blackgram (Vigna mungo). J. Mycol. Plant Pathol. 33: 56–58.
- Bawa, P. K., Halliday, J., Kapoor, K., and Banniza, S. 2022. Identification of candidate genes associated with resistance against race 0 of Colletotrichum lentis in Lens ervoides. Sci. Rep. 12: 18447. doi:10.1038/s41598-022-23175-z
- Bele, L., Kouamé, D. K., and Atta, H. D. 2018. Sensitivity of Colletotrichum species responsible for banana anthracnose disease to some fungicides used in postharvest treatments in Côte d’Ivoire. Int. J. Environ. Agric. Biotechnol. 3: 537–542. doi:10.22161/ijeab/3.2.30
- Bellé, C., Ramos, R. F., Moccellin, R., and Farias, C. R. J. de. 2020. Detection of Colletotrichum coccodes causing leaf anthracnose on Pisum sativum in southern Brazil. J. Plant Pathol. 102: 255–255. doi:10.1007/s42161-019-00392-6
- Benzohra, I. E., Bendahmane, B. S., Benkada, M. Y., Mégateli, M., and Belaidi, H. 2020. Use of three synthetic fungicides to reduce the incidence of ascochyta blight (Ascochyta rabiei) in chickpea (Cicer arietinum L.): a susceptible cultivars case. Indian J. Agric. Res. 54: 459–464.
- Bertioli, D. J., Jenkins, J., Clevenger, J., Dudchenko, O., Gao, D., Seijo, G., Leal-Bertioli, S. C. M., Ren, L., Farmer, A. D., Pandey, M. K., Samoluk, S. S., Abernathy, B., Agarwal, G., Ballén-Taborda, C., Cameron, C., Campbell, J., Chavarro, C., Chitikineni, A., Chu, Y., Dash, S., El Baidouri, M., Guo, B., Huang, W., Kim, K. D., Korani, W., Lanciano, S., Lui, C. G., Mirouze, M., Moretzsohn, M. C., Pham, M., Shin, J. H., Shirasawa, K., Sinharoy, S., Sreedasyam, A., Weeks, N. T., Zhang, X., Zheng, Z., Sun, Z., Froenicke, L., Aiden, E. L., Michelmore, R., Varshney, R. K., Holbrook, C. C., Cannon, E. K. S., Scheffler, B. E., Grimwood, J., Ozias-Akins, P., Cannon, S. B., Jackson, S. A., and Schmutz, J. 2019. The genome sequence of segmental allotetraploid peanut (Arachis hypogaea). Nat. Genet. 51: 877–884. doi:10.1038/s41588-019-0405-z
- Bhadauria, V., Banniza, S., Vandenberg, A., Selvaraj, G., and Wei, Y. 2013a. Overexpression of a novel biotrophy-specific Colletotrichum truncatum effector, CtNUDIX, in hemibiotrophic fungal phytopathogens causes incompatibility with their host plants. Eukaryot. Cell. 12: 2–11. doi:10.1128/EC.00192-12
- Bhadauria, V., Bett, K. E., Zhou, T., Vandenberg, A., Wei, Y., and Banniza, S. 2013b. Identification of Lens culinaris defense genes responsive to the anthracnose pathogen Colletotrichum truncatum. BMC Genet. 14: 9. doi:10.1186/1471-2156-14-31
- Bhadauria, V., MacLachlan, R., Pozniak, C., and Banniza, S. 2015. Candidate effectors contribute to race differentiation and virulence of the lentil anthracnose pathogen Colletotrichum lentis. BMC Genomics 16: 21. doi:10.1186/s12864-015-1836-2
- Bhadauria, V., MacLachlan, R., Pozniak, C., Cohen-Skalie, A., Li, L., Halliday, J., and Banniza, S. 2019. Genetic map-guided genome assembly reveals a virulence-governing minichromosome in the lentil anthracnose pathogen Colletotrichum lentis. New Phytol. 221: 431–445. doi:10.1111/nph.15369
- Bhadauria, V., Ramsay, L., Bett, K. E., and Banniza, S. 2017a. QTL mapping reveals genetic determinants of fungal disease resistance in the wild Lentil species Lens ervoides. Sci. Rep. 7: 3231. doi:10.1038/s41598-017-03463-9
- Bhadauria, V., Vijayan, P., Wei, Y., and Banniza, S. 2017b. Transcriptome analysis reveals a complex interplay between resistance and effector genes during the compatible lentil-Colletotrichum lentis interaction. Sci. Rep. 7: 13. doi:10.1038/srep42338
- Bhatt, P., Singh, K. P., and Aravind, T. 2022. Screening of soybean varieties under natural epiphytotic conditions against anthracnose/pod blight (Colletotrichum truncatum (Schw.) Andrus and Moore). Indian Phytopathol. 75: 1185–1189. doi:10.1007/s42360-022-00541-5
- Bhattacharjee, D., and Bhattacharjee, J. 2019. Identifying of mungbean resistance genotypes against mungbean yellow mosaic virus, anthracnose and cercospora diseases under natural condition in Tripura. Int. J. Curr. Microbiol. Appl. Sci. 8: 74–80. doi:10.20546/ijcmas.2019.809.011
- Bhunjun, C. S., Phukhamsakda, C., Jayawardena, R. S., Jeewon, R., Promputtha, I., and Hyde, K. D. 2021. Investigating species boundaries in Colletotrichum. Fungal Divers. 107: 107–127. doi:10.1007/s13225-021-00471-z
- Bindra, S., Mittal, R. K., Sood, V. K., and Sharma, P. N. 2016. Inheritance of resistance in urdbean (Vigna mungo) to anthracnose caused by Colletotrichum truncatum. Indian Phytopathol. 69: 311–313.
- Boadi, S. A., and Owusu, K. 2019. Impact of climate change and variability on hydropower in Ghana. Afr. Geogr. Rev. 38: 19–31.
- Borges, L. L., Santana, F. A., Castro, I., Arruda, K. M. A., Ramos, H. J. O., and Barros, E. G. 2015. Two-dimensional electrophoresis-based proteomic analysis of Phaseolus vulgaris in response to Colletotrichum lindemuthianum. J. Plant Pathol. 97: 249–257.
- Botero, L., Vizcaíno, S., Quiñones, W., Echeverri, F., Gil, J., and Durango, D. 2021. Increased accumulation of isoflavonoids in common bean (Phaseolus vulgaris L.) tissues treated with 1-oxo-indane-4-carboxylic acid derivatives. Biotechnol. Rep. 29: e00601.
- Boufleur, T. R., Castro, R. R. L., Rogério, F., Ciampi-Guillardi, M., Baroncelli, R., and Massola Júnior, N. S. 2020. First report of Colletotrichum musicola causing soybean anthracnose in Brazil. Plant Dis. 104: 1858. doi:10.1094/PDIS-12-19-2627-PDN
- Boufleur, T. R., Massola Júnior, N. S., Becerra, S., Baraldi, E., Bibiano, L. B. J., Sukno, S. A., Thon, M. R., and Baroncelli, R. 2022. Comparative transcriptomic provides novel insights into the soybean response to Colletotrichum truncatum infection. Front. Plant Sci. 13: 1046418. doi:10.3389/fpls.2022.1046418
- Buchwaldt, L., Anderson, K. L., Morrall, R. A. A., Gossen, B. D., and Bernier, C. C. 2004. Identification of lentil germ plasm resistant to Colletotrichum truncatum and characterization of two pathogen races. Phytopathology 94: 236–243. doi:10.1094/PHYTO.2004.94.3.236
- Buchwaldt, L., Dzananovic, E., and Durkin, J. 2018. Lentil anthracnose: epidemiology, fungicide decision support system, resistance and pathogen races. Can. J. Plant Pathol. 40: 189–198. doi:10.1080/07060661.2018.1441185
- Buchwaldt, L., Shaikh, R., Adam, J., Tullu, A., and Slinkard, A. E. 2013. Recessive and dominant genes confer resistance to Colletotrichum truncatum in cultivated lentil. Can. J. Plant Pathol. 35: 222–231. doi:10.1080/07060661.2013.768296
- Cai, L., Hyde, K. D., Taylor, P., Weir, B. S., Waller, J. M., Abang, M. M., Zhang, J. Z., Yang, Y. L., Phoulivong, S., and Liu, Z. Y. 2009. A polyphasic approach for studying Colletotrichum. Fungal Divers. 39: 183–204.
- Campa, A., Giraldez, R., and Ferreira, J. J. 2009. Genetic dissection of the resistance to nine anthracnose races in the common bean differential cultivars MDRK and TU. Theor. Appl. Genet. 119: 1–11. doi:10.1007/s00122-009-1011-8
- Campa, A., Giraldez, R., and Ferreira, J. J. 2011. Genetic analysis of the resistance to eight anthracnose races in the common bean differential cultivar kaboon. Phytopathology 101: 757–764. doi:10.1094/PHYTO-11-10-0296
- Campa, A., Rodríguez-Suárez, C., Giraldez, R., and Ferreira, J. J. 2014. Genetic analysis of the response to eleven Colletotrichum lindemuthianum races in a RIL population of common bean (Phaseolus vulgaris L.). BMC Plant Biol. 14: 12. doi:10.1186/1471-2229-14-115
- Cannon, P. F., Damm, U., Johnston, P. R., and Weir, B. S. 2012. Colletotrichum current status and future directions. Stud. Mycol. 73: 181–213. doi:10.3114/sim0014
- Chacko, R. J., Weidemann, G. J., TeBeest, D. O., and Correll, J. C. 1994. The use of vegetative compatibility and heterokaryosis to determine potential asexual gene exchange in Colletotrichum gloeosporioides. Biol. Control 4: 382–389. doi:10.1006/bcon.1994.1048
- Chakraborty, N., Mukherjee, K., Sarkar, A., and Acharya, K. 2019. Interaction between bean and Colletotrichum gloeosporioides: understanding through a biochemical approach. Plants 8: 345. doi:10.3390/plants8090345
- Chakraborty, N., Sarkar, A., Dasgupta, A., Paul, A., Mukherjee, K., and Acharya, K. 2022. In planta validation of nitric oxide mediated defense responses in common bean against Colletotrichum gloeosporioides infection. Indian Phytopathol. 75: 15–24. doi:10.1007/s42360-021-00425-0
- Chakraborty, S. 2004. High-yielding anthracnose-resistant Stylosanthes for agricultural systems. Aust. Cent. Int. Agric. Res. 1: 124.
- Chakraborty, S., Thomas, M. R., and Ellis, N. 1996. A multivariate analysis of pathogenic variation in Colletotrichum gloeosporioides infecting the tropical pasture legume, Stylosanthes scabra. Phytopathology 86: 283–289. doi:10.1094/Phyto-86-283
- Chatak, S., and Banyal, D. K. 2021. Evaluation of IDM components for the management of urdbean anthracnose caused by Colletotrichum truncatum (Schwein) Andrus and Moore. Himachal J. Agric. Res. 46: 156–161.
- Chaudhari, K. A., and Gohel, N. M. 2016. Management of anthracnose disease of mungbean through new fungicidal formulations. J. Pure Appl. Microbiol. 10: 691–696.
- Chen, L. S., Chu, C., Liu, C. D., Chen, R. S., and Tsay, J. G. 2006. PCR-based detection and differentiation of anthracnose pathogens, Colletotrichum gloeosporioides and C. truncatum, from vegetable soybean in Taiwan. J. Phytopathol. 154: 654–662. doi:10.1111/j.1439-0434.2006.01163.x
- Chen, M., Wu, J., Wang, L., Mantri, N., Zhang, X., Zhu, Z., and Wang, S. 2017. Mapping and genetic structure analysis of the anthracnose resistance locus Co-1HY in the common bean (Phaseolus vulgaris L.). PLOS One 12: e0169954. doi:10.1371/journal.pone.0169954
- Chen, S., Wang, Y., Schnabel, G., Peng, C. A., Lagishetty, S., Smith, K., Luo, C., and Yuan, H. 2018. Inherent resistance to 14a-demethylation inhibitor fungicides in Colletotrichum truncatum is likely linked to CYP51A and/or CYP51B gene variants. Phytopathology 108: 1263–1275. doi:10.1094/PHYTO-02-18-0054-R
- Chongo, G., Gossen, B. D., and Bernier, C. C. 2002. Infection by Colletotrichum truncatum in resistant and susceptible lentil genotypes. Can. J. Plant Pathol. 24: 81–85. doi:10.1080/07060660109506977
- Ciampi-Guillardi, M., Baldauf, C., Souza, A. P., Silva-Junior, G. J., and Amorim, L. 2014. Recent introduction and recombination in Colletotrichum acutatum populations associated with citrus postbloom fruit drop epidemics in São Paulo, Brazil. Phytopathology 104: 769–778. doi:10.1094/PHYTO-06-13-0165-R
- Clemente, A., and Olias, R. 2017. Beneficial effects of legumes in gut health. Curr. Opin. Food Sci. 14: 32–36. doi:10.1016/j.cofs.2017.01.005
- Coimbra-Gonçalves, G. K., Gonçalves-Vidigal, M. C., Coelho, R. T., Valentini, G., Filho, P. S. V., Lacanallo, G. F., Sousa, L. L., and Elias, H. T. 2016. Characterization and mapping of anthracnose resistance gene in mesoamerican common bean cultivar Crioulo 159. Crop Sci. 56: 2904–2915. doi:10.2135/cropsci2015.10.0651
- Costa Lara Fioreze, A. C. D., Grigolo, S., Piva, C. A. G., and Sartori, L. 2018. Common bean landraces as potential sources of resistance to anthracnose. Pesqui. Agropecu. Trop. 48: 126–133. doi:10.1590/1983-40632018v4851251
- Costa, I. F. D. da., Balardin, R. S., Medeiros, L. A. M., Lenz, G., Gulart, C. A., Zemolin, C. R., and Silva, T. M. B. 2009. Reao de germoplasma comercial de soja a Colletotrichum truncatum. Trop. Plant Pathol. 34: 47–50. doi:10.1590/S1982-56762009000100009
- Cowling, W. A., Buirchell, B. J., Sweetingham, M. W., Yang, H., Thomas, G., Luckett, D. J., Brown, A. G. P., and Hamblin, J. 2000. Anthracnose resistance in lupins–an innovative Australian research effort 1996–1998. In: Lupin, An Ancient Crop for the New Millennium: Proceedings of the 9th International Lupin Conference, Klink/Muritz, Germany, 20-24 June, 1999. International Lupin Association: Klink; Muritz, pp 60–62.
- Czepiel, K., Krajewski, P., Wilczura, P., Bielecka, P., Święcicki, W., and Kroc, M. 2021. Expression profiles of alkaloid-related genes across the organs of narrow-leafed lupin (Lupinus angustifolius L.) and in response to anthracnose infection. Int. J. Mol. Sci. 22: 1–22.
- da Silva, C. M., Costa, L. C., Porto, A. C. M., Lima, A. A., Chalfun-Junior, A., Souza, E. A. D., and Pereira, W. A. 2021. Differential gene expression in common bean during interaction with race 65 of Colletotrichum lindemuthianum. Trop. Plant Pathol. 46: 518–527. doi:10.1007/s40858-021-00447-z
- da Silva, L. L., Moreno, H. L. A., Correia, H. L. N., Santana, M. F., and Queiroz, M. V. de. 2020. Colletotrichum: species complexes, lifestyle, and peculiarities of some sources of genetic variability. Appl. Microbiol. Biotechnol. 104: 1891–1904. doi:10.1007/s00253-020-10363-y
- Damm, U., O’Connell, R. J., Groenewald, J. Z., and Crous, P. W. 2014. The Colletotrichum destructivum species complex–hemibiotrophic pathogens of forage and field crops. Stud. Mycol. 79: 49–84. doi:10.1016/j.simyco.2014.09.003
- Damm, U., Sato, T., Alizadeh, A., Groenewald, J. Z., and Crous, P. W. 2019. The Colletotrichum dracaenophilum. Stud. Mycol. 92: 1–46. doi:10.1016/j.simyco.2018.04.001
- Damm, U., Woudenberg, J. H. C., Cannon, P. F., and Crous, P. W. 2009. Colletotrichum species with curved conidia from herbaceous hosts. Fungal Divers. 39: 45–87.
- David, P., Sévignac, M., Thareau, V., Catillon, Y., Kami, J., Gepts, P., Langin, T., and Geffroy, V. 2008. BAC end sequences corresponding to the B4 resistance gene cluster in common bean: a resource for markers and synteny analyses. Mol. Genet. Genomics 280: 521–533. doi:10.1007/s00438-008-0384-8
- Deguine, J. P., Aubertot, J. N., Bellon, S., Côte, F., Lauri, P. E., and Lescourret, F. 2023. Agroecological crop protection for sustainable agriculture. Adv. Agron. 178: 1–59.
- Dias, M. D., Dias-Neto, J. J., Santos, M. D. M., Formento, A. N., Bizerra, L. V. A. S., Fonseca, M. E. N., Boiteux, L. S., and Café-Filho, A. C. 2019. Current status of soybean anthracnose associated with Colletotrichum truncatum in Brazil and Argentina. Plants 8: 459. doi:10.3390/plants8110459
- Dias, M. D., Fonseca, M. E. N., Dias-Neto, J. J., Santos, M. D. M., Pandolfo, G. M., Boiteux, L. S., and Café-Filho, A. C. 2018. Biology, pathogenicity, and haplotype analyses of Colletotrichum cliviae: a novel soybean anthracnose agent in warm tropical areas. Trop. Plant Pathol. 43: 439–451. doi:10.1007/s40858-018-0249-6
- Dias, M. D., Pinheiro, V. F., and Café-Filho, A. C. 2016. Impact of anthracnose on the yield of soybean subjected to chemical control in the north region of Brazil. Summa Phytopathol. 42: 18–23. doi:10.1590/0100-5405/2114
- Dubrulle, G., Picot, A., Madec, S., Corre, E., Pawtowski, A., Baroncelli, R., Zivy, M., Balliau, T., Floch, G. Le., and Pensec, F. 2020. Deciphering the infectious process of Colletotrichum lupini in lupin through transcriptomic and proteomic analysis. Microorganisms 8: 1621. doi:10.3390/microorganisms8101621
- Dwi, S., Sri, W., Edy, B. M. S., and Erman, M. 2014. Utilization of chitinolytic bacterial isolates to control anthracnose of cocoa leaf caused by Colletotrichum gloeosporioides. Afr. J. Biotechnol. 13: 1631–1637. doi:10.5897/AJB11.3687
- Elad, Y., and Pertot, I. 2014. Climate Change impacts on plant pathogens and plant diseases. J. Crop Improv. 28: 99–139. doi:10.1080/15427528.2014.865412
- Elshire, R. J., Glaubitz, J. C., Sun, Q., Poland, J. A., Kawamoto, K., Buckler, E. S., and Mitchell, S. E. 2011. A robust, simple genotyping-by-sequencing (GBS) approach for high diversity species. PLOS One 6: e19379. doi:10.1371/journal.pone.0019379
- Enyiukwu, D. N., Awurum, A. N., Ononuju, C. C., and Nwaneri, J. A. 2014. Biology and management strategies of cowpea anthracnose disease caused by Colletrotrichum species. Greener J. Biochem. Biotechnol. 1: 52–65. doi:10.15580/GJBB.2014.2.070414288
- Faisal Peeran, M., Kuppusami, P., and Thiruvengadam, R. 2014. Pathogenesis of Colletotrichum lindemuthianum the incitant of anthracnose disease in beans mediated by macerating enzymes. N Save Nat. Surviv. 9: 295–300.
- Falade, M. J., and Borisade, O. A. 2018. Toxicity of copper (1) oxide metalaxyl fungicide and selected plant extracts to Colletotrichium lindemuthianum (Sensu Lato) and management of cowpea anthracnose disease in Nigeria. J. Agric. Sci. Technol. 18: 1–11. doi:10.9734/AJAAR/2017/38158
- Fan, S., Chang, Y., Liu, G., Shang, S., Tian, L., and Shi, H. 2020. Molecular functional analysis of auxin/indole-3-acetic acid proteins (Aux/IAAs) in plant disease resistance in cassava. Physiol. Plant 168: 88–97. doi:10.1111/ppl.12970
- Ferreira, J. J., Campa, A., and Kelly, J. D. 2013. Organization of genes conferring resistance to anthracnose in common bean. In Translational Genomics for Crop Breeding: Biotic Stress, Ames, IA: John Wiley & Sons, Inc. Vol. 1, pp 151–181.
- Fiala, J. V., Tullu, A., Banniza, S., Séguin-Swartz, G., and Vandenberg, A. 2009. Interspecies transfer of resistance to anthracnose in lentil (Lens culinaris medic). Crop Sci. 49: 825–830. doi:10.2135/cropsci2008.05.0260
- Fischer, K., Dieterich, R., Nelson, M. N., Kamphuis, L. G., Singh, K. B., Rotter, B., Krezdorn, N., Winter, P., Wehling, P., and Ruge-Wehling, B. 2015. Characterization and mapping of LanrBo: a locus conferring anthracnose resistance in narrow-leafed lupin (Lupinus angustifolius L.). Theor. Appl. Genet. 128: 2121–2130. doi:10.1007/s00122-015-2572-3
- Flor, H. H. 1971. Current status of the gene-for-gene concept. Annu. Rev. Phytopathol. 9: 275–296. doi:10.1146/annurev.py.09.090171.001423
- Fontenelle, M. R., Santana, M. F., Cnossen, A., Bazzolli, D. M. S., Bromonschenkel, S. H., Araújo, E. F. de., and Queiroz, M. V. de. 2017. Differential expression of genes during the interaction between Colletotrichum lindemuthianum and Phaseolus vulgaris. Eur. J. Plant Pathol. 147: 653–670. doi:10.1007/s10658-016-1033-4
- Ford, R., Banniza, S., Photita, W., and Taylor, P. W. J. 2004. Morphological and molecular discrimination of Colletotrichum truncatum causing anthracnose on lentil in Canada. Austral. Plant Pathol. 33: 559–569. doi:10.1071/AP04058
- Freeman, S., Katan, T., and Shabi, E. 1996. Characterization of Colletotrichum gloeosporioides isolates from avocado and almond fruits with molecular and pathogenicity tests. Appl. Environ. Microbiol. 62: 1014–1020. doi:10.1128/aem.62.3.1014-1020.1996
- Gan, P., Narusaka, M., Tsushima, A., Narusaka, Y., Takano, Y., and Shirasu, K. 2017. Draft genome assembly of Colletotrichum chlorophyti, a pathogen of herbaceous plants. Genome Announc. 5: 01733-16. doi:10.1128/genomeA.01733-16
- Ganesh, S. K., Packiaraj, D., Geetha, S., Gnanamalar, R. P., Manivannan, N., Mahalingam, A., Narayanan, S. L., Satya, V. K., Kavitha, Z., and Ganesamurthy, K. 2022. VBN 3: a new high yielding multiple disease resistant cowpea variety. Electron. J. Plant Breed. 12: 1375–1379.
- García-Pajón, C. M., and Collado, I. G. 2003. Secondary metabolites isolated from Colletotrichum species. Nat. Prod. Rep. 20: 426–431. doi:10.1039/b302183c
- Garg, G., Kamphuis, L. G., Bayer, P. E., Kaur, P., Dudchenko, O., Taylor, C. M., Frick, K. M., Foley, R. C., Gao, L.-L., Aiden, E. L., Edwards, D., and Singh, K. B. 2022. A pan-genome and chromosome-length reference genome of narrow-leafed lupin (Lupinus angustifolius) reveals genomic diversity and insights into key industry and biological traits. Plant J. 111: 1252–1266. doi:10.1111/tpj.15885
- Garzón, L. N., Blair, M. W., and Ligarreto, G. A. 2007. Use of molecular marker assisted selection for resistance to anthracnose in common beans. Agron. Colomb. 25: 207–214.
- Geffroy, V., Sévignac, M., Billant, P., Dron, M., and Langin, T. 2008. Resistance to Colletotrichum lindemuthianum in Phaseolus vulgaris: a case study for mapping two independent genes. Theor. Appl. Genet. 116: 407–415. doi:10.1007/s00122-007-0678-y
- Geffroy, V., Sicard, D., de Oliveira, J. C., Sévignac, M., Cohen, S., Gepts, P., Neema, C., Langin, T., and Dron, M. 1999. Identification of an ancestral resistance gene cluster involved in the coevolution process between Phaseolus vulgaris and its fungal pathogen Colletotrichum lindemuthianum. Mol. Plant. Microbe Interact. 12: 774–784. doi:10.1094/MPMI.1999.12.9.774
- Gela, T. S., Banniza, S., and Vandenberg, A. 2020. Lack of effective resistance to the virulent race of Colletotrichum lentis in Lens culinaris Medikus subsp. culinaris. Plant Genet. Resour. 18: 81–87. doi:10.1017/S1479262120000027
- Gela, T. S., Koh, C. S., Caron, C. T., Chen, L. A., Vandenberg, A., and Bett, K. E. 2021b. QTL mapping of lentil anthracnose (Colletotrichum lentis) resistance from Lens ervoides accession IG 72815 in an interspecific RIL population. Euphytica 217: 1–11. doi:10.1007/s10681-021-02804-0
- Gela, T., Ramsay, L., Haile, T. A., Vandenberg, A., and Bett, K. 2021a. Identification of anthracnose race 1 resistance loci in lentil by integrating linkage mapping and genome-wide association study. Plant Genome 14: 20131. doi:10.1002/tpg2.20131
- Gilio, T. A. S., Hurtado-Gonzales, O. P., Gonçalves-Vidigal, M. C., Valentini, G., Ferreira Elias, J. C., Song, Q., and Pastor-Corrales, M. A. 2020. Fine mapping of an anthracnose-resistance locus in Andean common bean cultivar Amendoim Cavalo. PLOS One 15: e0239763. doi:10.1371/journal.pone.0239763
- Girma, F., Fininsa, C., Terefe, H., and Amsalu, B. 2022. Distribution of common bacterial blight and anthracnose diseases and factors influencing epidemic development in major common bean growing areas in Ethiopia. Acta Agric. Scand. Sect. B Soil Plant Sci. 72: 685–699. doi:10.1080/09064710.2022.2063168
- Giugliano, D., Ceriello, A., and Esposito, K. 2006. The Effects of diet on inflammation. emphasis on the metabolic syndrome. J. Am. Coll. Cardiol. 48: 677–685. doi:10.1016/j.jacc.2006.03.052
- Gonçalves-Vidigal, M. C., and Kelly, J. D. 2006. Inheritance of anthracnose resistance in the common bean cultivar Widusa. Euphytica 151: 411–419. doi:10.1007/s10681-006-9164-x
- Gonçalves-Vidigal, M. C., Cruz, A. S., Garcia, A., Kami, J., Filho, P. S. V., Sousa, L. L., McClean, P., Gepts, P., and Pastor-Corrales, M. A. 2011. Linkage mapping of the Phg-1 and Co-14 genes for resistance to angular leaf spot and anthracnose in the common bean cultivar AND 277. Theor. Appl. Genet. 122: 893–903. doi:10.1007/s00122-010-1496-1
- Gonçalves-Vidigal, M. C., Cruz, A. S., Lacanallo, G. F., Vidigal Filho, P. S., Sousa, L. L., Pacheco, C. M. N. A., McClean, P., Gepts, P., and Pastor-Corrales, M. A. 2013. Co-segregation analysis and mapping of the anthracnose Co-10 and angular leaf spot Phg-ON disease-resistance genes in the common bean cultivar Ouro Negro. Theor. Appl. Genet. 126: 2245–2255. doi:10.1007/s00122-013-2131-8
- Gonçalves-Vidigal, M. C., Gilio, T. A. S., Valentini, G., Vaz-Bisneta, M., Vidigal Filho, P. S., Song, Q., Oblessuc, P. R., and Melotto, M. 2020. New Andean source of resistance to anthracnose and angular leaf spot: fine mapping of disease-resistance genes in California Dark Red Kidney common bean cultivar. PLOS One 15: e0235215. doi:10.1371/journal.pone.0235215
- Gonçalves-Vidigal, M. C., Lacanallo, G. F., and Vidigal Filho, P. S. 2008. A new gene conferring resistance to anthracnose in Andean common bean (Phaseolus vulgaris L.) cultivar “Jalo Vermelho”. Plant Breed. 127: 592–596. doi:10.1111/j.1439-0523.2008.01530.x
- Gonçalves-Vidigal, M. C., Silva, C. R. da, Vidigal Filho, P. S., Gonela, A., and Kvitschal, M. V. 2007. Allelic relationships of anthracnose (Colletotrichum lindemuthianum) resistance in the common bean (Phaseolus vulgaris L.) cultivar Michelite and the proposal of a new anthracnose resistance gene, Co-11. Genet. Mol. Biol. 30: 589–593. doi:10.1590/S1415-47572007000400015
- Gonçalves-Vidigal, M., Pacheco, C., Vidigal Filho, P., Lacanallo, G., Sousa, L., and Martins, V. 2016. Genetic mapping of the anthracnose resistance gene Co-14 in the common bean cultivar Pitanga. Annu. Rep. Bean Improv. Coop. 59: 85–86.
- González, A. M., Yuste-Lisbona, F. J., Paula Rodiño, A., Ron, A. M., De, Capel, C., García-Alcázar, M., Lozano, R., and Santalla, M. 2015. Uncovering the genetic architecture of Colletotrichum lindemuthianum resistance through QTL mapping and epistatic interaction analysis in common bean. Front. Plant Sci. 6: 141. doi:10.3389/fpls.2015.00141
- Gossen, B. D., Anderson, K. L., and Buchwaldt, L. 2009. Host specificity of Colletotrichum truncatum from lentil. Can. J. Plant Pathol. 31: 65–73. doi:10.1080/07060660909507573
- Guilengue, N., Silva, M. C., Talhinhas, P., Neves-Martins, J., and Loureiro, A. 2022. Subcuticular–intracellular hemibiotrophy of Colletotrichum lupini in Lupinus mutabilis. Plants 11: 3028. doi:10.3390/plants11223028
- Gupta, C., Gupta, M., Gupta, S., and Salgotra, R. K. 2021. Screening of common bean (Phaseolus vulgaris L.) germplasm against Colletotrichum lindemuthianum inciting bean anthracnose. Res. J. Biotech. 17: 13–18. doi:10.25303/1701rjbt1318
- Gupta, O. 2021. Pulses–changing scenario of diseases and their management strategies. J. Food Legum. 34: 147–148.
- Gupta, S., Kalha, C. S., Vaid, A., and Rizvi, S. E. H. 2005. Integrated management of anthracnose of French bean caused by Colletotrichum lindemuthianum. J. Mycol. Plant Pathol. 35: 432–436.
- Ha, J., Satyawan, D., Jeong, H., Lee, E., Cho, K. H., Kim, M. Y., and Lee, S. H. 2021. A near-complete genome sequence of mungbean (Vigna radiata L.) provides key insights into the modern breeding program. Plant Genome 14: 20121. doi:10.1002/tpg2.20121
- Haase, F., and Ruge-Wehling, B. 2019. Transcriptome-based mapping of anthracnose resistance gene (Llur) in yellow lupin (Lupinus luteus). In: 12th Young Scientists Meeting 2019: 6th–8th November in Kleinmachnow. https://www.openagrar.de/receive/openagrar_mods_00052350.
- Han, Y., Zeng, X., Xiang, F., Zhang, Q., Guo, C., Chen, F., and Gu, Y. 2018. Carbendazim sensitivity in populations of Colletotrichum gloeosporioides complex infecting strawberry and yams in Hubei Province of China. J. Integr. Agric. 17: 1391–1400. doi:10.1016/S2095-3119(17)61854-9
- Hane, J. K., Ming, Y., Kamphuis, L. G., Nelson, M. N., Garg, G., Atkins, C. A., Bayer, P. E., Bravo, A., Bringans, S., Cannon, S., Edwards, D., Foley, R., Gao, L.-L., Harrison, M. J., Huang, W., Hurgobin, B., Li, S., Liu, C.-W., McGrath, A., Morahan, G., Murray, J., Weller, J., Jian, J., and Singh, K. B. 2017. A comprehensive draft genome sequence for lupin (Lupinus angustifolius), an emerging health food: insights into plant–microbe interactions and legume evolution. Plant Biotechnol. J. 15: 318–330. doi:10.1111/pbi.12615
- Hartman, G. L., Sinclair, J. B., and Rupe, J. C. 1999. Compendium of soybean diseases. St. Paul, MN: APS Press.
- Heffner, E. L., Sorrells, M. E., and Jannink, J. L. 2009. Genomic selection for crop improvement. Crop Sci. 49: 1–12. doi:10.2135/cropsci2008.08.0512
- Hegay, S., Geleta, M., Bryngelsson, T., Asanaliev, A., Garkava-Gustavsson, L., Persson Hovmalm, H., and Ortiz, R. 2014. Introducing host-plant resistance to anthracnose in Kyrgyz common bean through inoculation-based and marker-aided selection. Plant Breed. 133: 86–91. doi:10.1111/pbr.12121
- Hernández-Álvarez, A. J., Carrasco-Castilla, J., Dávila-Ortiz, G., Alaiz, M., Girón-Calle, J., Vioque-Peña, J., Jacinto-Hernández, C., and Jiménez-Martínez, C. 2013. Angiotensin-converting enzyme-inhibitory activity in protein hydrolysates from normal and anthracnose disease-damaged Phaseolus vulgaris seeds. J. Sci. Food Agric. 93: 961–966. doi:10.1002/jsfa.5841
- Hill, M. J., and Donald, G. E. 1998. Australian Temperate Pastures Database. CSIRO, CD ROM, National Pasture Improvement Coordinating Committee/CSIRO Division of Animal Production: Canberra.
- Huang, L., Kim, K. T., Yang, J. Y., Song, H., Choi, G., Jeon, J., Cheong, K., Ko, J., Xu, H., and Lee, Y. H. 2019. A high-quality draft genome sequence of Colletotrichum gloeosporioides sensu stricto SMCG1#C, a causal agent of anthracnose on Cunninghamia lanceolata in China. Mol. Plant. Microbe Interact. 32: 139–141. doi:10.1094/MPMI-05-18-0144-A
- Hufnagel, B., Marques, A., Soriano, A., Marquès, L., Divol, F., Doumas, P., Sallet, E., Mancinotti, D., Carrere, S., Marande, W., Arribat, S., Keller, J., Huneau, C., Blein, T., Aimé, D., Laguerre, M., Taylor, J., Schubert, V., Nelson, M., Geu-Flores, F., Crespi, M., Gallardo, K., Delaux, P.-M., Salse, J., Bergès, H., Guyot, R., Gouzy, J., and Péret, B. 2020. High-quality genome sequence of white lupin provides insight into soil exploration and seed quality. Nat. Commun. 11: 492. doi:10.1038/s41467-019-14197-9
- Hufnagel, B., Soriano, A., Taylor, J., Divol, F., Kroc, M., Sanders, H., Yeheyis, L., Nelson, M., and Péret, B. 2021. Pangenome of white lupin provides insights into the diversity of the species. Plant Biotechnol. J. 19: 2532–2543. doi:10.1111/pbi.13678
- Hyde, K. D., Cai, L., McKenzie, E. H. C., Yang, Y. L., Zhang, J. Z., and Prihastuti, H. 2009. Colletotrichum: a catalogue of confusion. Fungal Divers. 39: 1–17.
- Iamsupasit, N., Chakraborty, S., Cameron, D. F., and Adkins, S. W. 1993. Components of quantitative resistance to anthracnose (Colletotrichum gloeosporioides) in tetraploid accessions of the pasture legume Stylosanthes hamata. Aust. J. Exp. Agric. 33: 855–860. doi:10.1071/EA9930855
- Intan Sakinah, M. A., Suzianti, I. V., and Latiffah, Z. 2013. First report of Colletotrichum gloeosporioides causing anthracnose of banana (Musa spp.) in Malaysia. Plant Dis. 97: 991. doi:10.1094/PDIS-10-12-0985-PDN
- Irwin, J. A. G., and Cameron, D. F. 1978. Two diseases in Stylosanthes spp. caused by Colletotrichum gloeosporioides in Australia, and pathogenic specialization within one of the causal organisms. Aust. J. Agric. Res. 29: 305–317. doi:10.1071/AR9780305
- Iskandar Vijaya, S., Mohd Anuar, I. S., and Zakaria, L. 2015. Characterization and pathogenicity of Colletotrichum truncatum causing stem anthracnose of red-fleshed dragon fruit (Hylocereus polyrhizus) in Malaysia. J. Phytopathol. 163: 67–71. doi:10.1111/jph.12261
- Jacob, I., Feuerstein, U., Heinz, M., Schott, M., and Urbatzka, P. 2017. Evaluation of new breeding lines of white lupin with improved resistance to anthracnose. Euphytica 213: 236. doi:10.1007/s10681-017-2011-4
- Jacob, I., Hartmann, S., and Struck, C. 2016. Response of different fodder legume species to Colletotrichum trifolii. Crop Pasture Sci. 67: 1110–1115. doi:10.1071/CP16162
- Jahan, M. A., Harris, B., Lowery, M., Coburn, K., Infante, A. M., Percifield, R. J., Ammer, A. G., and Kovinich, N. 2019. The NAC family transcription factor GmNAC42-1 regulates biosynthesis of the anticancer and neuroprotective glyceollins in soybean. BMC Genomics 20: 149. doi:10.1186/s12864-019-5524-5
- Jaiganesh, V., Kiruthika, P., and Kannan, C. 2019. Integrated disease management of chilli anthracnose. J. Biopestic. 12: 126–133.
- Jayawardena, R. S., Hyde, K. D., Damm, U., Cai, L., Liu, M., Li, X. H., Zhang, W., Zhao, W. S., and Yan, J. Y. 2016. Notes on currently accepted species of Colletotrichum. Mycosphere 7: 1192–1260. doi:10.5943/mycosphere/si/2c/9
- Jegadeesan, S., Raizada, A., Dhanasekar, P., and Suprasanna, P. 2021. Draft genome sequence of the pulse crop blackgram [Vigna mungo (L.) Hepper] reveals potential R-genes. Sci. Rep. 11: 11247. doi:10.1038/s41598-021-90683-9
- Jiang, J., Ma, S., Ye, N., Jiang, M., Cao, J., and Zhang, J. 2017. WRKY transcription factors in plant responses to stresses. J. Integr. Plant Biol. 59: 86–101. doi:10.1111/jipb.12513
- Jiang, L., Wu, P., Yang, L., Liu, C., Guo, P., Wang, H., Wang, S., Xu, F., Zhuang, Q., Tong, X., Liu, P., and Luo, L. 2021. Transcriptomics and metabolomics reveal the induction of flavonoid biosynthesis pathway in the interaction of Stylosanthes-Colletotrichum gloeosporioides. Genomics 113: 2702–2716. doi:10.1016/j.ygeno.2021.06.004
- Júnior, M. B. S., Resende, M. L. V., Pozza, E. A., Cruz Machado, J., Resende, A. R. M., Cardoso, A. M. S., Silva Costa Guimarães, S., and Santos Botelho, D. M. dos. 2021. Effect of temperature on Colletotrichum truncatum growth, and evaluation of its inoculum potential in soybean seed germination. Eur. J. Plant Pathol. 160: 999–1004. doi:10.1007/s10658-021-02293-w
- Kaiser, W. J., Mihov, M., Muehlbauer, F. J., and Hannan, R. M. 1998. First report of anthracnose of lentil incited by Colletotrichum truncatum in Bulgaria. Plant Dis. 82: 128. doi:10.1094/PDIS.1998.82.1.128C
- Kale, S. L., and Barhate, B. G. 2016. Management of anthracnose in soybean caused by Colletotrichum truncatum. Int. J. Plant Prot. 9: 583–588. doi:10.15740/HAS/IJPP/9.2/583-588
- Kamh, M., Horst, W. J., Amer, F., Mostafa, H., and Maier, P. 1999. Mobilization of soil and fertilizer phosphate by cover crops. Plant Soil 211: 19–27. doi:10.1023/A:1004543716488
- Kang, Y. J., Kim, S. K., Kim, M. Y., Lestari, P., Kim, K. H., Ha, B.-K., Jun, T. H., Hwang, W. J., Lee, T., Lee, J., Shim, S., Yoon, M. Y., Jang, Y. E., Han, K. S., Taeprayoon, P., Yoon, N., Somta, P., Tanya, P., Kim, K. S., Gwag, J.-G., Moon, J.-K., Lee, Y.-H., Park, B.-S., Bombarely, A., Doyle, J. J., Jackson, S. A., Schafleitner, R., Srinives, P., Varshney, R. K., and Lee, S.-H. 2014. Genome sequence of mungbean and insights into evolution within Vigna species. Nat. Commun. 5: 5443. doi:10.1038/ncomms6443
- Katoch, A., Sharma, P., Padder, B. A., and Sharma, P. N. 2017. Population structure of Colletotrichum truncatum in Himachal Pradesh and Identification of broad-spectrum resistant sources in Capsicum. Agric. Res. 6: 296–303. doi:10.1007/s40003-017-0261-y
- Kaur, L., Singh, P., and Sirari, A. 2011. Biplot analysis for locating multiple disease resistance diversity in mungbean germplasm. Plant Dis. Res. 26: 55–60.
- Kaur, S., Gonçalves-Vidigal, M. C., Davidson, J., Mysore, K. S., and Pandey, A. K. 2023. Disease and pest resistance in legume crops. Front. Plant Sci. 14: 1166387. doi:10.3389/fpls.2023.1166387
- Kaushal, R. P., and Singh, B. M. 1988. Genetics of disease resistance in urdbean (Vigna mungo (L.) Hepper) to the leaf spots caused by Colletotrichum truncatum (Schw.) Andrus & Moore. Euphytica 37: 279–281. doi:10.1007/BF00015124
- Kavanashree, K., Jahagirdar, S., Priyanka, K., Uday, G., Kambrekar, D. N., Krishnaraj, P. U., Basavaraja, G. T., and Patil, M. S. 2022. Molecular variability of Colletotrichum spp. associated with anthracnose of soybean. Legum. Res. 45: 1048–1053.
- Kazan, K., and Manners, J. M. 2009. Linking development to defense: auxin in plant-pathogen interactions. Trends Plant Sci. 14: 373–382. doi:10.1016/j.tplants.2009.04.005
- Kelemu, S., Skinner, D. Z., Badel, J. L., Moreno, C. X., Rodríguez, M. X., Fernandes, C. D., Charchar, M. J., and Chakraborty, S. 1999. Genetic diversity in South American Colletotrichum gloeosporioides isolates from Stylosanthes guianensis, a tropical forage legume. Eur. J. Plant Pathol. 105: 261–272. doi:10.1023/A:1008764428437
- Kelly, J. D., and Vallejo, V. A. 2004. A comprehensive review of the major genes conditioning resistance to anthracnose in common bean. Hortscience 39: 1196–1207. doi:10.21273/HORTSCI.39.6.1196
- Khan, M. 1992. Pathogenicity of sclerotia- and nonsclerotia-forming isolates of Colletotrichum truncatum on soybean plants and roots. Phytopathology 82: 314. doi:10.1094/Phyto-82-314
- Khan, M., and Sinclair, J. B. 1991. Effect of soil temperature on infection of soybean roots by sclerotia-forming isolates of Colletotrichum truncatum. Plant Dis. 75: 1282–1285. doi:10.1094/PD-75-1282
- Kim, B., Cho, K., and Lee, Y. 1998. Anthracnose of Rumex crispus caused by Colletotrichum gloeosporioides. Korean J. Plant Pathol. 14: 358–360.
- Kim, K. C., Fan, B., and Chen, Z. 2006. Pathogen-induced Arabidopsis WRKY7 is a transcriptional represser and enhances plant susceptibility to Pseudomonas syringae. Plant Physiol. 142: 1180–1192. doi:10.1104/pp.106.082487
- Klosterman, S. J., Rollins, J. R., Sudarshana, M. R., and Vinatzer, B. A. 2016. Disease management in the genomics era-summaries of focus issue papers. Phytopathology 106: 1068–1070. doi:10.1094/PHYTO-07-16-0276-FI
- Książkiewicz, M., Nazzicari, N., Yang, H., Nelson, M. N., Renshaw, D., Rychel, S., Ferrari, B., Carelli, M., Tomaszewska, M., Stawiński, S., Naganowska, B., Wolko, B., and Annicchiarico, P. 2017. A high-density consensus linkage map of white lupin highlights synteny with narrow-leafed lupin and provides markers tagging key agronomic traits. Sci. Rep. 7: 15335. doi:10.1038/s41598-017-15625-w
- Książkiewicz, M., Rychel-Bielska, S., Plewiński, P., Bielski, W., Nuc, M., Kozak, B., Krajewski, P., and Jędryczka, M. 2022. A successful defense of the narrow-leafed lupin against anthracnose involves quick and orchestrated reprogramming of oxidation–reduction, photosynthesis and pathogenesis-related genes. Sci. Rep. 12: 8164. doi:10.1038/s41598-022-12257-7
- Kulkarni, S. 2019. Epidemiology of greengram (Vigna radiate) anthracnose in northern Karnataka. J. Pharmacog. Phytochem. 8: 434–437.
- Kulkarni, S. A. 2009. Epidemiology and integrated management of anthracnose of greengram. M.Sc. (Agri.) Thesis. UAS Dharwad, Karnataka, India.
- Kumar, A., Rawa, R., Roy, N., Ahamad, A., and Kumar, H. 2020. Evaluation of fungicides for management of anthracnose disease of black gram (Vigna mungo L.) in growing areas of district Jhansi of Bundelkhand region. J. Appl. Nat. Sci. 12: 110–114. doi:10.31018/jans.vi.2257
- Kumar, P., Pandey, P., Dubey, R. C., and Maheshwari, D. K. 2016. Bacteria consortium optimization improves nutrient uptake, nodulation, disease suppression and growth of the common bean (Phaseolus vulgaris) in both pot and field studies. Rhizosphere 2: 13–23. doi:10.1016/j.rhisph.2016.09.002
- Lacanallo, G. F., and Gonçalves-Vidigal, M. C. 2015. Mapping of an Andean gene for anthracnose resistance (Co-13) in common bean (Phaseolus vulgaris L.) Jalo Listras Pretas landrace. Aust. J. Crop Sci. 9: 394–400.
- Lamichhane, J. R., Barzman, M., Booij, K., Boonekamp, P., Desneux, N., Huber, L., Kudsk, P., Langrell, S. R. H., Ratnadass, A., Ricci, P., Sarah, J. L., and Messéan, A. 2015. Robust cropping systems to tackle pests under climate change. A review. Agron. Sustain. Dev. 35: 443–459. doi:10.1007/s13593-014-0275-9
- Lamichhane, J. R., Corrales, D. C., and Soltani, E. 2022. Biological seed treatments promote crop establishment and yield: a global meta-analysis. Agron. Sustain. Dev. 42: 45. doi:10.1007/s13593-022-00761-z
- Lamichhane, J. R., You, M. P., Laudinot, V., Barbetti, M. J., and Aubertot, J. N. 2020. Revisiting sustainability of fungiside seed treatments for field crops. Plant Dis. 104: 610–623. doi:10.1094/PDIS-06-19-1157-FE
- Lamprecht, P. S., and Knox-Davies, S. C. 1984. Preliminary survey of foliage diseases of annual Medicago spp. in South Africa. Phytophylactic 16: 177–183.
- Lee, J. H., Jeong, S. W., Cho, Y. A., Park, S., Kim, Y.-H., Bae, D. W., Chung, J. I., Kwak, Y.-S., Jeong, M.-J., Park, S.-C., Shim, J.-H., Jin, J. S., and Shin, S. C. 2013. Determination of the variations in levels of phenolic compounds in soybean (Glycine max Merr.) sprouts infected by anthracnose (Colletotrichum gloeosporioides). J. Sci. Food Agric. 93: 3081–3086. doi:10.1002/jsfa.6142
- Lenné, J. M. 1986. Recent advances in the understanding of anthracnose of Stylosanthes in tropical America. In: Proceedings of XV International Grasslands Congress, Kyoto, Japan.
- Lenné, J. M. 1994. Diseases of stylosanthes. In Diseases of Tropical Pasture Plants; Lenné, J. M. and Trutmann, P., Eds. CAB International: Kent, pp 21–42.
- Liang, C., Zhang, B., Zhou, Y., Yin, H., An, B., Lin, D., He, C., and Luo, H. 2021. CgNPG1 as a novel pathogenic gene of Colletotrichum gloeosporioides from Hevea brasiliensis in mycelial growth, conidiation, and the invasive structures development. Front. Microbiol. 12: 629387. doi:10.3389/fmicb.2021.629387
- Lichtin, N., Salvo-Garrido, H., Till, B., Caligari, P. D. S., Rupayan, A., Westermeyer, F., and Olivos, M. 2020. Genetic and comparative mapping of Lupinus luteus L. highlight syntenic regions with major orthologous genes controlling anthracnose resistance and flowering time. Sci. Rep. 10: 19174. doi:10.1038/s41598-020-76197-w
- Lima Castro, S. A. de, Gonçalves-Vidigal, M. C., Gilio, T. A. S., Lacanallo, G. F., Valentini, G., Silva Ramos Martins, V., Song, Q., Galván, M. Z., Hurtado-Gonzales, O. P., and Pastor-Corrales, M. A. 2017. Genetics and mapping of a new anthracnose resistance locus in Andean common bean Paloma. BMC Genomics 18: 12. doi:10.1186/s12864-017-3685-7
- Lima, L. R. L., Gonçalves‐Vidigal, M. C., Vaz Bisneta, M., Valentini, G., Vidigal Filho, P. S., Martins, V. d S. R., and de Souza, T. L. P. O. 2023. Genetic fine-mapping of anthracnose disease-resistance allele Co-14 present in the Andean common bean cultivar AND 277. Crop Sci. 63: 750–763. doi:10.1002/csc2.20905
- Liu, B., Pavel, J. A., Hausbeck, M. K., Feng, C., and Correll, J. C. 2021. Phylogenetic analysis, vegetative compatibility, virulence, and fungal filtrates of leaf curl pathogen Colletotrichum fioriniae from celery. Phytopathology 111: 751–760. doi:10.1094/PHYTO-04-20-0123-R
- Liu, F., Cai, L., Crous, P. W., and Damm, U. 2014. The Colletotrichum gigasporum species complex. Persoonia Mol. Phylogeny Evol. Fungi 33: 83–97. doi:10.3767/003158514X684447
- Liu, F., Wang, M., Damm, U., Crous, P. W., and Cai, L. 2016. Species boundaries in plant pathogenic fungi: a Colletotrichum case study. BMC Evol. Biol. 16: 1–14.
- Liu, J.-Z., Horstman, H. D., Braun, E., Graham, M. A., Zhang, C., Navarre, D., Qiu, W.-L., Lee, Y., Nettleton, D., Hill, J. H., and Whitham, S. A. 2011. Soybean homologs of MPK4 negatively regulate defense responses and positively regulate growth and development. Plant Physiol. 157: 1363–1378. doi:10.1104/pp.111.185686
- Liu, Y., Du, H., Li, P., Shen, Y., Peng, H., Liu, S., Zhou, G.-A., Zhang, H., Liu, Z., Shi, M., Huang, X., Li, Y., Zhang, M., Wang, Z., Zhu, B., Han, B., Liang, C., and Tian, Z. 2020. Pan-genome of wild and cultivated soybeans. Cell 182: 162–176.e13. doi:10.1016/j.cell.2020.05.023
- Lokya Naik, B. H., and Anilkumar, T. B. 1991. Conidial production and germination in carbendazim and thiophanate resistant strains of Colletotrichum lindemuthianum from cowpea. Zentralbl. Mikrobiol. 146: 463–465. doi:10.1016/S0232-4393(11)80232-5
- Lonardi, S., Muñoz-Amatriaín, M., Liang, Q., Shu, S., Wanamaker, S. I., Lo, S., Tanskanen, J., Schulman, A. H., Zhu, T., Luo, M.-C., Alhakami, H., Ounit, R., Hasan, A. M., Verdier, J., Roberts, P. A., Santos, J. R. P., Ndeve, A., Doležel, J., Vrána, J., Hokin, S. A., Farmer, A. D., Cannon, S. B., and Close, T. J. 2019. The genome of cowpea (Vigna unguiculata [L.] Walp.). Plant J. 98: 767–782. doi:10.1111/tpj.14349
- López, C. E., Acosta, I. F., Jara, C., Pedraza, F., Gaitán-Solís, E., Gallego, G., Beebe, S., and Tohme, J. 2003. Identifying resistance gene analogs associated with resistances to different pathogens in common bean. Phytopathology 93: 88–95. doi:10.1094/PHYTO.2003.93.1.88
- Lozovaya, V. V., Lygin, A. V., Zernova, O. V., and Widholm, J. M. 2005. Genetic engineering of plant root disease resistance by modification of the phenylpropanoid pathway. Plant Biosyst. 139: 20–23. doi:10.1080/11263500500055247
- Lucas, M. M., Stoddard, F. L., Annicchiarico, P., Frías, J., Martínez-Villaluenga, C., Sussmann, D., Duranti, M., Seger, A., Zander, P. M., and Pueyo, J. J. 2015. The future of lupin as a protein crop in Europe. Front. Plant Sci. 6: 705. doi:10.3389/fpls.2015.00705
- Luo, M., and Jiang, Y. 2022. First report of anthracnose caused by Colletotrichum karsti in lentil (Lablab purpureus). Crop Prot. 155: 105903. doi:10.1016/j.cropro.2021.105903
- Lv, T., Li, X., Fan, T., Luo, H., Xie, C., Zhou, Y., and Tian, C. E. 2019. The calmodulin-binding protein IQM1 interacts with CATALASE2 to affect pathogen defense. Plant Physiol. 181: 1314–1327. doi:10.1104/pp.19.01060
- Mackie, J. M., Musial, J. M., O'Neill, N. R., and Irwin, J. A. G. 2003. Pathogenic specialisation within Colletotrichum trifolii in Australia, and lucerne cultivar reactions to all known Australian pathotypes. Aust. J. Agric. Res. 54: 829–836. doi:10.1071/AR03079
- Mahiya-Farooq, Padder, B. A., Bhat, N. N., Shah, M. D., Shikari, A. B., Awale, H. E., and Kelly, J. D. 2019. Temporal expression of candidate genes at the Co-1 locus and their interaction with other defense related genes in common bean. Physiol. Mol. Plant Pathol 108: 101424. doi:10.1016/j.pmpp.2019.101424
- Mahmodi, F., Kadir, J. B., Nasehi, A., Puteh, A., and Soleimani, N. 2013. Occurrence of anthracnose caused by Colletotrichum truncatum on chickpea (Cicer arietinum) in Malaysia. Plant Dis. 97: 1507. doi:10.1094/PDIS-03-13-0231-PDN
- Mahmodi, F., Kadir, J. B., Puteh, A., Pourdad, S. S., Nasehi, A., and Soleimani, N. 2014. Genetic diversity and differentiation of Colletotrichum spp. isolates associated with leguminosae using multigene loci, RAPD and ISSR. Plant Pathol. J. 30: 10–24. doi:10.5423/PPJ.OA.05.2013.0054
- Manandhar, J. B. 1986. Colletotrichum destructivum, the anamorph of Glomerella glycines. Phytopathology 76: 282. doi:10.1094/Phyto-76-282
- Marak, T., Mahapatra, S., and Das, S. 2018. Stability analysis of disease reactions and yield of green gram [Vigna radiata (L.) Wilczck] against anthracnose caused by Colletotrichum truncatum. Legum. Res. 41: 919–924.
- Marcon, J. R. S., Gonçalves-Vidigal, M. C., Paulino, J. F. C., Vidigal Filho, P. S., and Coêlho, M. 2020. Genetic resistance of common bean cultivar beija flor to Colletotrichum lindemuthianum. Acta Sci. Agron. 43: e44910. doi:10.4025/actasciagron.v43i1.44910
- Marin-Felix, Y., Groenewald, J. Z., Cai, L., Chen, Q., Marincowitz, S., Barnes, I., Bensch, K., Braun, U., Camporesi, E., Damm, U., de Beer, Z. W., Dissanayake, A., Edwards, J., Giraldo, A., Hernández-Restrepo, M., Hyde, K. D., Jayawardena, R. S., Lombard, L., Luangsa-Ard, J., McTaggart, A. R., Rossman, A. Y., Sandoval-Denis, M., Shen, M., Shivas, R. G., Tan, Y. P., van der Linde, E. J., Wingfield, M. J., Wood, A. R., Zhang, J. Q., Zhang, Y., and Crous, P. W. 2017. Genera of phytopathogenic fungi: GOPHY 1. Stud. Mycol. 86: 99–216. doi:10.1016/j.simyco.2017.04.002
- Masi, M., Castaldi, S., Sautua, F., Pescitelli, G., Carmona, M. A., and Evidente, A. 2022. Truncatenolide, a bioactive disubstituted nonenolide produced by Colletotrichum truncatum, the causal agent of anthracnose of soybean in Argentina: fungal antagonism and SAR studies. J. Agric. Food Chem. 70: 9834–9844. doi:10.1021/acs.jafc.2c02502
- Melotto, M., Afanador, L., and Kelly, J. 1996. Development of a SCAR marker linked to the I gene in common bean. Genome 39: 1216–1219. doi:10.1139/g96-155
- Méndez-Vigo, B., Rodríguez-Suárez, C., Pañeda, A., Ferreira, J. J., and Giraldez, R. 2005. Molecular markers and allelic relationships of anthracnose resistance gene cluster B4 in common bean. Euphytica 141: 237–245. doi:10.1007/s10681-005-7075-x
- Meziadi, C., Richard, M. M. S., Derquennes, A., Thareau, V., Blanchet, S., Gratias, A., Pflieger, S., and Geffroy, V. 2016. Development of molecular markers linked to disease resistance genes in common bean based on whole genome sequence. Plant Sci. 242: 351–357. doi:10.1016/j.plantsci.2015.09.006
- Misal, D., Khaire, P., Misal, M., and Hingole, D. 2019. Integrated evaluation of fungicides, botanicals and bioagents against anthracnose of mungbean on natural field condition. IJSC 7: 1975–1978.
- Mishra, R. K., Bohra, A., Kamaal, N., Kumar, K., Gandhi, K., Sujayanand, G. K., Saabale, P. R., Satheesh Naik, S. J., Sarma, B. K., and Kumar, D. 2018. Utilization of biopesticides as sustainable solutions for management of pests in legume crops: achievements and prospects. Egypt. J. Biol. Pest Control 28: 1–11.
- Mishra, R., Mohanty, J. N., Mahanty, B., and Joshi, R. K. 2021. A single transcript CRISPR/Cas9 mediated mutagenesis of CaERF28 confers anthracnose resistance in chilli pepper (Capsicum annuum L.). Planta 254: 5. doi:10.1007/s00425-021-03660-x
- Mohammed, A. 2013. An overview of distribution, biology and the management of common bean anthracnose. J. Plant Pathol. Microbiol. 4: 1–6.
- Morrall, R. A. A. 1988. A new disease of lentil induced by Colletotrichum truncatum in Manitoba. Plant Dis. 72: 994. doi:10.1094/PD-72-0994D
- Mukankusi, C., Raatz, B., Nkalubo, S., Berhanu, F., Binagwa, P., Kilango, M., Williams, M., Enid, K., Chirwa, R., and Beebe, S. 2019. Genomics, genetics and breeding of common bean in Africa: a review of tropical legume project. Plant Breed. 138: 401–414. doi:10.1111/pbr.12573
- Murphy-Bokern, D., Stoddard, F. L., and Watson, C. A. 2017. Legumes in cropping systems. Boston: CABI Publishing.
- Muth, D., Kachlicki, P., Krajewski, P., Przystalski, M., and Stobiecki, M. 2009. Differential metabolic response of narrow leafed lupine (Lupinus angustifolius) leaves to infection with Colletotrichum lupini. Metabolomics 5: 354–362. doi:10.1007/s11306-009-0162-6
- Nagaraj, B. T., Shamarao, J., and Basavaraja, G. T. 2014. Identification of resistant sources in glass house and field evaluation of soybean genotypes to anthracnose caused by Colletotrichum truncatum (Schw.) Andrus and Moore. Bioscan 9: 1333–1336.
- Naseem, M., Srivastava, M., Tehseen, M., and Ahmed, N. 2015. Auxin crosstalk to plant immune networks: a plant-pathogen interaction perspective. Curr. Protein Pept. Sci. 16: 389–394. doi:10.2174/1389203716666150330124911
- Nataraj, V., Maranna, S., Kumawat, G., Gupta, S., Rajput, L. S., Kumar, S., Sharma, A. N., and Bhatia, V. S. 2020. Genetic inheritance and identification of germplasm sources for anthracnose resistance in soybean [Glycine max (L.) Merr.]. Genet. Resour. Crop Evol. 67: 1449–1456. doi:10.1007/s10722-020-00917-4
- Nirenberg, H. I., Feiler, U., and Hagedorn, G. 2002. Description of Colletotrichum lupini comb. nov. in modern terms. Mycologia 94: 307–320. doi:10.2307/3761809
- Nkalubo, S. 2006. Study of anthracnose (Colletotrichum lindemuthianum) resistance and its inheritance in Ugandan dry bean germplasm, p 167.
- Noor, N. M., and Zakaria, L. 2018. Identification and characterization of Colletotrichum spp. associated with chili anthracnose in peninsular Malaysia. Eur. J. Plant Pathol. 151: 961–973. doi:10.1007/s10658-018-1431-x
- O’Connell, R. J., Bailey, J. A., and Richmond, D. V. 1985. Cytology and physiology of infection of Phaseolus vulgaris by Colletotrichum lindemuthianum. Physiol. Plant Pathol. 27: 75–98. doi:10.1016/0048-4059(85)90058-X
- O’Connell, R. J., Thon, M. R., Hacquard, S., Amyotte, S. G., Kleemann, J., Torres, M. F., Damm, U., Buiate, E. A., Epstein, L., Alkan, N., Altmüller, J., Alvarado-Balderrama, L., Bauser, C. A., Becker, C., Birren, B. W., Chen, Z., Choi, J., Crouch, J. A., Duvick, J. P., Farman, M. A., Gan, P., Heiman, D., Henrissat, B., Howard, R. J., Kabbage, M., Koch, C., Kracher, B., Kubo, Y., Law, A. D., Lebrun, M.-H., Lee, Y.-H., Miyara, I., Moore, N., Neumann, U., Nordström, K., Panaccione, D. G., Panstruga, R., Place, M., Proctor, R. H., Prusky, D., Rech, G., Reinhardt, R., Rollins, J. A., Rounsley, S., Schardl, C. L., Schwartz, D. C., Shenoy, N., Shirasu, K., Sikhakolli, U. R., Stüber, K., Sukno, S. A., Sweigard, J. A., Takano, Y., Takahara, H., Trail, F., van der Does, H. C., Voll, L. M., Will, I., Young, S., Zeng, Q., Zhang, J., Zhou, S., Dickman, M. B., Schulze-Lefert, P., Ver Loren van Themaat, E., Ma, L.-J., and Vaillancourt, L. J. 2012. Lifestyle transitions in plant pathogenic Colletotrichum fungi deciphered by genome and transcriptome analyses. Nat. Genet. 44: 1060–1065. doi:10.1038/ng.2372
- O’Connell, R. J., Uronu, A. B., Waksman, G., Nash, C., Keon, J. P. R., and Bailey, J. A. 1993. Hemibiotrophic infection of Pisum sativum by Colletotrichum truncatum. Plant Pathol 42: 774–783. doi:10.1111/j.1365-3059.1993.tb01564.x
- Oblessuc, P. R., Borges, A., Chowdhury, B., Caldas, D. G. G., Tsai, S. M., Camargo, L. E. A., and Melotto, M. 2012. Dissecting Phaseolus vulgaris innate immune system against Colletotrichum lindemuthianum infection. PLOS One 7: e43161. doi:10.1371/journal.pone.0043161
- Oo, M. M., and Oh, S. K. 2020. First report of anthracnose of chili pepper fruit caused by Colletotrichum truncatum in Korea. Plant Dis. 104: 564. doi:10.1094/PDIS-09-19-1874-PDN
- Padder, B. A., Kamfwa, K., Awale, H. E., and Kelly, J. D. 2016. Transcriptome profiling of the Phaseolus vulgaris–Colletotrichum lindemuthianum pathosystem. PLOS One 11: e0165823. doi:10.1371/journal.pone.0165823
- Padder, B. A., Sharma, P. N., and Sharma, O. P. 2008. Ribosomal DNA analysis of Colletotrichum lindemuthianum virulences from Himachal Pradesh (India). Appl. Biol. 10: 6–10.
- Padder, B. A., Sharma, P. N., Awale, H. E., and Kelly, J. D. 2017. Colletotrichum lindemuthianum, the causal agent of bean anthracnose. J. Plant Pathol. 99: 317–330.
- Padder, B. A., Sharma, P. N., Kapil, R., Pathania, A., and Sharma, O. P. 2010. Evaluation of bioagents and biopesticides against Colletotrichum lindemuthianum and its integrated management in common bean. Not. Sci. Biol. 2: 72–76. doi:10.15835/nsb234772
- Pagano, M. C., and Miransari, M. 2016. Production Worldwide; Elsevier Inc.: Amsterdam.
- Palacıoğlu, G., Özer, G., Yeken, M. Z., Çiftçi, V., and Bayraktar, H. 2021. Resistance sources and reactions of common bean (Phaseolus vulgaris L.) cultivars in Turkey to anthracnose disease. Genet. Resour. Crop Evol. 68: 3373–3381. doi:10.1007/s10722-021-01195-4
- Pande, S., Desai, S., and Sharma, M. 2010. Impacts of climate change on rainfed crop diseases: current status and future research needs. Natl. Symp. Clim. Chang. Rainfed Agric, pp 18–20.
- Pandey, A. K., Basandrai, A. K., Basandrai, D., Boddepalli, V. N., Rathore, A., Adapala, G., and Nair, R. M. 2021a. Field-relevant new sources of resistance to anthracnose caused by Colletotrichum truncatum in a mungbean mini-core collection. Plant Dis. 105: 2001–2010.
- Pandey, A. K., Burlakoti, R. R., Kenyon, L., and Nair, R. M. 2018. Perspectives and challenges for sustainable management of fungal diseases of mungbean [Vigna radiata (L.) R. Wilczek var. radiata]: a review. Front. Environ. Sci. 6: 53. doi:10.3389/fenvs.2018.00053
- Pandey, A. K., Savani, A. K., and Singh, P. 2021b. The Early blight of tomato: omics interventions toward controlling disease spread and development. In Omics Technologies for Sustainable Agriculture and Global Food Security, Vol. 1; Kumar, A., Kumar, R., Shukla, P., and Pandey, M. K., Eds. Springer: Singapore, pp 85–108.
- Pandey, S. P., and Somssich, I. E. 2009. The role of WRKY transcription factors in plant immunity. Plant Physiol. 150: 1648–1655. doi:10.1104/pp.109.138990
- Pangga, I. B., Chakraborty, S., and Yates, D. 2004. Canopy size and induced resistance in Stylosanthes scabra determine anthracnose severity at high CO2. Phytopathology 94: 221–227. doi:10.1094/PHYTO.2004.94.3.221
- Pangga, I., Hanan, J., and Chakraborty, S. 2013. Climate change impacts on plant canopyarchitecture: implications for pest and pathogen management. Eur. J. Plant Pathol. 135: 595–610. doi:10.1007/s10658-012-0118-y
- Papitha, K., Sanjeevkumar, K., Balabaskar, P., and Kumar, S. 2020. Bioefficacy evaluation of serratia marcescens against anthracnose (Colletotrichum lindemuthianum (Sacc. & Magnus) Briosi & Cavara) disease in Dolichos bean. Plant Arch. 20: 493–496.
- Parthasarathy, S., Nagendran, K., Narayanan, P., Rajalakshmi, J., Thiribhuvanamala, G., and Prabakar, K. 2015. Novel insights into the phytotoxins production of Colletotrichum gloeosporioides causing anthracnose of mango. Trends Biosci. 8: 3924–3927.
- Pathak, R., Kumar Singh, S., Tak, A., and Gehlot, P. 2018. Impact of climate change on host, pathogen and plant disease adaptation regime: a review. Biosci. Biotech. Res. Asia 15: 529–540. doi:10.13005/bbra/2658
- Perfect, S. E., Hughes, H. B., O’Connell, R. J., and Green, J. R. 1999. Colletotrichum: a model genus for studies on pathology and fungal-plant interactions. Fungal Genet. Biol. 27: 186–198. doi:10.1006/fgbi.1999.1143
- Perseguini, J. M. K. C., Oblessuc, P. R., Rosa, J. R. B. F., Gomes, K. A., Chiorato, A. F., Carbonell, S. A. M., Garcia, A. A. F., Vianello, R. P., and Benchimol-Reis, L. L. 2016. Genome-wide association studies of anthracnose and angular leaf spot resistance in common bean (Phaseolus vulgaris L.). PLOS One 11: e0150506. doi:10.1371/journal.pone.0150506
- Phan, H. T. T., Ellwood, S. R., Adhikari, K., Nelson, M. N., and Oliver, R. P. 2007. The first genetic and comparative map of white lupin (Lupinus albus L.): identification of QTLs for anthracnose resistance and flowering time, and a locus for alkaloid content. DNA Res. 14: 59–70. doi:10.1093/dnares/dsm009
- Piano, E., and Francis, C. M. 1992. The annual species of Medicago in the Mediterranean region, ecogeography and related aspects of plant introduction and breeding. In Proceedings of the Xth International Conference of the EUCARPIA Medicago Spp. Group, pp 373–385.
- Plissonneau, C., Benevenuto, J., Mohd-Assaad, N., Fouché, S., Hartmann, F. E., and Croll, D. 2017. Using population and comparative genomics to understand the genetic basis of effector-driven fungal pathogen evolution. Front. Plant Sci. 8: 119. doi:10.3389/fpls.2017.00119
- Poti, T., Mahawan, K., Cheewangkoon, R., Arunothayanan, H., Akimitsu, K., and Nalumpang, S. 2020. Detection and molecular characterization of carbendazim-resistant Colletotrichum truncatum isolates causing anthracnose of soybean in Thailand. J. Phytopathol. 168: 267–278. doi:10.1111/jph.12888
- Pradhan, D., Mathew, D., Mathew, S. K., and Nazeem, P. A. 2018. Identifying the markers and tagging a leucine-rich repeat receptor-like kinase gene for resistance to anthracnose disease in vegetable cowpea [Vigna unguiculata (L.) Walp.]. J. Hortic. Sci. Biotechnol. 93: 225–231. doi:10.1080/14620316.2017.1362962
- Pszczółkowska, A., Okorski, A., Fordoński, G., Kotecki, A., Kozak, M., and Dzienis, G. 2019. Effect of weather conditions on yield and health status of faba bean seeds in Poland. Agronomy 10: 48. doi:10.3390/agronomy10010048
- Queiroz, C. B., Correia, H. L. N., Menicucci, R. P., Vidigal, P. M. P., and Queiroz, M. V. de. 2017. Draft genome sequences of two isolates of Colletotrichum lindemuthianum, the causal agent of anthracnose in common beans. Genome Announc. 5: 00214-17. doi:10.1128/genomeA.00214-17
- Raman, R., Cowley, R. B., Raman, H., and Luckett, D. J. 2014. Analyses using SSR and DArT molecular markers reveal that Ethiopian accessions of white lupin (Lupinus albus L.) represent a unique genepool. Open J. Genet. 4: 87–98. doi:10.4236/ojgen.2014.42012
- Ramos, A. M., Gally, M., García, M. C., and Levin, L. 2010. Producción de enzimas pectinolíticas por Colletotrichum truncatum, agente causal de antracnosis en soja. Rev. Iberoam. Micol. 27: 186–190. doi:10.1016/j.riam.2010.06.002
- Ramos, A. M., Tadic, L. F., Cinto, I., Carmona, M., and Gally, M. 2013. Molecular characterization of Colletotrichum species causing soybean anthracnose in Argentina. Mycotaxon 123: 457–465. doi:10.5248/123.457
- Rampersad, S. N., Perez-Brito, D., Torres-Calzada, C., Tapia-Tussell, R., and Carrington, C. V. F. 2013. Genetic structure and demographic history of Colletotrichum gloeosporioides sensu lato and C. truncatum isolates from Trinidad and Mexico. BMC Evol. Biol. 13: 1–17.
- Rana, D. S., Dass, A., Rajanna, G. A., and Kaur, R. 2016. Biotic and abiotic stress in pulses. Indian J. Agron. 61: 238–248.
- Ranathunge, N. P., Ford, R., and Taylor, P. W. J. 2009. Development and optimization of sequence-tagged microsatellite site markers to detect genetic diversity within Colletotrichum capsici, a causal agent of chilli pepper anthracnose disease. Mol. Ecol. Resour. 9: 1175–1179. doi:10.1111/j.1755-0998.2009.02608.x
- Rao, S. N., Bhattiprolu, S. L., Kumari, V. P., Gopal, A. V., and Kumar, P. A. 2020. Cross Infectivity studies of Colletotrichum spp., causing Anthracnose in different beans. Andhra Agric. J. 67: 70–75.
- Rao, S., and Nandineni, M. R. 2017. Genome sequencing and comparative genomics reveal a repertoire of putative pathogenicity genes in chilli anthracnose fungus Colletotrichum truncatum. PLOS One 12: e0183567. doi:10.1371/journal.pone.0183567
- Reckling, M., Bergkvist, G., Watson, C. A., Stoddard, F. L., Zander, P. M., Walker, R. L., Pristeri, A., Toncea, I., and Bachinger, J. 2016. Trade-offs between economic and environmental impacts of introducing legumes into cropping systems. Front. Plant Sci. 7: 669. doi:10.3389/fpls.2016.00669
- Reveglia, P., Agudo-Jurado, F. J., Barilli, E., Masi, M., Evidente, A., and Rubiales, D. 2023. Uncovering phytotoxic compounds produced by Colletotrichum spp. involved in legume diseases using an OSMAC–metabolomics approach. J. Fungi 9: 610. doi:10.3390/jof9060610
- Riccioni, L., Conca, G., and Hartman, G. L. 1998. First report of Colletotrichum coccodes on soybean in the United States. Plant Dis. 82: 959–959. doi:10.1094/PDIS.1998.82.8.959C
- Richard, M. M. S., Gratias, A., Alvarez Diaz, J. C., Thareau, V., Pflieger, S., Meziadi, C., Blanchet, S., Marande, W., Bitocchi, E., Papa, R., Miklas, P. N., and Geffroy, V. 2021. A common bean truncated CRINKLY4 kinase controls gene-for-gene resistance to the fungus Colletotrichum lindemuthianum. J. Exp. Bot. 72: 3569–3581. doi:10.1093/jxb/erab082
- Richard, M. M. S., Gratias, A., Thareau, V., Kim, K., Do, Balzergue, S., Joets, J., Jackson, S. A., and Geffroy, V. 2018. Genomic and epigenomic immunity in common bean: the unusual features of NB-LRR gene family. DNA Res. 25: 161–172. doi:10.1093/dnares/dsx046
- Riera, N., Ramirez-Villacis, D., Barriga-Medina, N., Alvarez-Santana, J., Herrera, K., Ruales, C., and Leon-Reyes, A. 2019. First report of banana anthracnose caused by Colletotrichum gloeosporioides in Ecuador. Plant Dis. 103: 763–763. doi:10.1094/PDIS-01-18-0069-PDN
- Rodríguez-Suárez, C., Ferreira, J. J., Campa, A., Pañeda, A., and Giraldez, R. 2008. Molecular mapping and intra-cluster recombination between anthracnose race-specific resistance genes in the common bean differential cultivars Mexico 222 and Widusa. Theor. Appl. Genet. 116: 807–814. doi:10.1007/s00122-008-0714-6
- Rogério, F., Boufleur, T. R., Ciampi-Guillardi, M., Sukno, S. A., Thon, M. R., Massola Júnior, N. S., and Baroncelli, R. 2020. Genome sequence resources of Colletotrichum truncatum, C. plurivorum, C. musicola, and C. sojae: four species pathogenic to soybean (Glycine max). Phytopathology 110: 1497–1499. doi:10.1094/PHYTO-03-20-0102-A
- Rogério, F., Ciampi-Guillardi, M., Barbieri, M. C. G., Bragança, C. A. D., Seixas, C. D. S., Almeida, A. M. R., and Massola, N. S. 2017. Phylogeny and variability of Colletotrichum truncatum associated with soybean anthracnose in Brazil. J. Appl. Microbiol. 122: 402–415. doi:10.1111/jam.13346
- Rogério, F., Gladieux, P., Massola, N. S., and Ciampi-Guillardi, M. 2019. Multiple introductions without admixture of Colletotrichum truncatum associated with soybean anthracnose in Brazil. Phytopathology 109: 681–689. doi:10.1094/PHYTO-08-18-0321-R
- Roopadevi, Jamadar, M. M., and Anusha, B. G. 2014. Survey for incidence and severity of green gram [Vigna radiata (L.) Wilczek] anthracnose caused by Colletotrichum truncatum (Schw.) Andrus and Moore. Trends Biosci. 7: 3941–3943.
- Roy, A., Sahu, P. K., Das, C., Bhattacharyya, S., Raina, A., and Mondal, S. 2022. Conventional and new-breeding technologies for improving disease resistance in lentil (Lens culinaris Medik). Front. Plant Sci. 13: 1001682. doi:10.3389/fpls.2022.1001682
- Rychel-Bielska, S., Nazzicari, N., Plewiński, P., Bielski, W., Annicchiarico, P., and Książkiewicz, M. 2020. Development of PCR-based markers and whole-genome selection model for anthracnose resistance in white lupin (Lupinus albus L.). J. Appl. Genet. 61: 531–545. doi:10.1007/s13353-020-00585-1
- Salotti, I., Ji, T., and Rossi, V. 2022. Temperature requirements of Colletotrichum spp. belonging to different clades. Front. Plant Sci. 13: 953760. doi:10.3389/fpls.2022.953760
- Sant’Anna, J. R., Miyamoto, C. T., Rosada, L. J., Franco, C. C. S., Kaneshima, E. N., and Castro-Prado, M. A. A. 2010. Genetic relatedness of Brazilian Colletotrichum truncatum isolates assessed by vegetative compatibility groups and RAPD analysis. Biol. Res. 43: 51–62.
- Santos Vieria, W. A. dos, Santos Nunes, A. dos, Veloso, J. S., Machado, A. R., Balbino, V. Q., Silva, A. C. da, Gomes, A. Â. M., Doyle, V. P., and Câmara, M. P. S. 2020. Colletotrichum truncatum causing anthracnose on papaya fruit (Carica papaya) in Brazil. Australas. Plant Dis. Notes 15: 1–3.
- Sarrocco, S., Herrera-Estrella, A., and Collinge, D. B. 2020. Plant disease management in the post-genomic era: from functional genomics to genome editing. Front. Microbiol. 11: 3389. doi:10.3389/fmicb.2020.00107
- Schmutz, J., Cannon, S. B., Schlueter, J., Ma, J., Mitros, T., Nelson, W., Hyten, D. L., Song, Q., Thelen, J. J., Cheng, J., Xu, D., Hellsten, U., May, G. D., Yu, Y., Sakurai, T., Umezawa, T., Bhattacharyya, M. K., Sandhu, D., Valliyodan, B., Lindquist, E., Peto, M., Grant, D., Shu, S., Goodstein, D., Barry, K., Futrell-Griggs, M., Abernathy, B., Du, J., Tian, Z., Zhu, L., Gill, N., Joshi, T., Libault, M., Sethuraman, A., Zhang, X.-C., Shinozaki, K., Nguyen, H. T., Wing, R. A., Cregan, P., Specht, J., Grimwood, J., Rokhsar, D., Stacey, G., Shoemaker, R. C., and Jackson, S. A. 2010. Genome sequence of the palaeopolyploid soybean. Nature 463: 178–183. doi:10.1038/nature08670
- Sekhwal, M. K., Li, P., Lam, I., Wang, X., Cloutier, S., and You, F. M. 2015. Disease resistance gene analogs (RGAs) in plants. Int. J. Mol. Sci. 16: 19248–19290. doi:10.3390/ijms160819248
- Shafi, S., Saini, D. K., Khan, M. A., Bawa, V., Choudhary, N., Dar, W. A., Pandey, A. K., Varshney, R. K., and Mir, R. R. 2022. Delineating meta-quantitative trait loci for anthracnose resistance in common bean (Phaseolus vulgaris L.). Front. Plant Sci. 13: 966339. doi:10.3389/fpls.2022.966339
- Shaikh, R., Diederichsen, A., Harrington, M., Adam, J., Conner, R. L., and Buchwaldt, L. 2013. New sources of resistance to Colletotrichum truncatum race Ct0 and Ct1 in Lens culinaris Medikus subsp. culinaris obtained by single plant selection in germplasm accessions. Genet. Resour. Crop Evol. 60: 193–201. doi:10.1007/s10722-012-9825-7
- Sharma, N., Thakur, R., Rana, D., Sharma, P., and Basandrai, A. K. 2022. Combating bean anthracnose through integrated disease management. Plant Dis. Res. 37: 15–22. doi:10.5958/2249-8788.2022.00003.8
- Sharma, P. N., Padder, B. A., Sharma, O. P., Pathania, A., and Sharma, P. 2007. Pathological and molecular diversity in Colletotrichum lindemuthianum (bean anthracnose) across Himachal Pradesh, a north-western Himalayan state of India. Austral. Plant Pathol. 36: 191–197. doi:10.1071/AP07013
- Sharma, R. 2009. Genetic differentiation of host limited forms of Colletotrichum truncatum from northwestern Himalayas. Arch. Phytopathol. Plant Prot. 42: 960–966. doi:10.1080/03235400701543848
- Sharma, S. K., Gupta, G. K., and Ramteke, R. 2011. Colletotrichum truncatum [(Schw.) Andrus and W.D.]. Soybean Res. 9: 31–52.
- Sharma, V., Basandrai, A. K., Basandrai, D., Gautam, N. K., and Sharma, P. N. 2014. Identification of stable sources of resistance against anthracnose (Colletotrichum truncatum) in urdbean (Vigna mungo). J. Food Legum. 27: 218–220.
- Shi, M., Xue, S. M., Zhang, M. Y., Li, S. P., Huang, B. Z., Huang, Q., Liu, Q. B., Liao, X. L., and Li, Y. Z. 2022. Colletotrichum truncatum—A new etiological anthracnose agent of sword bean (Canavalia gladiata) in Southwestern China. Pathogens 11: 1463. doi:10.3390/pathogens11121463
- Shi, N. N., Ruan, H. C., Jie, Y. L., Chen, F. R., and Du, Y. X. 2021a. Characterization, fungicide sensitivity and efficacy of Colletotrichum spp. from chili in Fujian, China. Crop Prot. 143: 105572. doi:10.1016/j.cropro.2021.105572
- Shi, X. C., Wang, S. Y., Duan, X. C., Gao, X., Zhu, X. Y., and Laborda, P. 2021b. First report of Colletotrichum brevisporum causing soybean anthracnose in China. Plant Dis. 105: 707. doi:10.1094/PDIS-09-20-1910-PDN
- Shiny, A. A., Mathew, D., Nazeem, P. A., Abida, P. S., Mathew, S. K., and Valsala, P. A. 2015. Identification and confirmation of trailing-type vegetable cowpea resistance to anthracnose. Trop. Plant Pathol. 40: 169–175. doi:10.1007/s40858-015-0032-x
- Shivas, R. G. 1989. Fungal and bacterial diseases of plants in Western Australia. J. R. Soc. West. Aust. 72: 1–62.
- Shivas, R. G., McClements, J. L., and Sweetingham, M. W. 1998. Vegetative compatibility amongst isolates of Colletotrichum causing lupin anthracnose. Austral. Plant Pathol. 27: 269–273. doi:10.1071/AP98032
- Sileshi, F., Mohammed, A., Selvaraj, T., and Negeri, M. 2014. Field management of anthracnose (Colletotrichum lindemuthianum) in common bean through foliar spray fungicides and seed treatment bioagents. Sci. Technol. Arts Res. J. 3: 19. doi:10.4314/star.v3i2.3
- Singh, S., Prasad, D., and Singh, V. P. 2022. Evaluation of fungicides and genotypes against anthracnose disease of mungbean caused by Colletotrichum lindemuthianum. Int. J. Bio-Resource Stress Manag. 13: 448–453. doi:10.23910/1.2022.2884
- Soares, M. A., Nogueira, G. B., Bazzolli, D. M. S., Araújo, E. F., Langin, T., and Queiroz, M. V. 2014. PacCl, a pH-responsive transcriptional regulator, is essential in the pathogenicity of Colletotrichum lindemuthianum, a causal agent of anthracnose in bean plants. Eur. J. Plant Pathol. 140: 769–785. doi:10.1007/s10658-014-0508-4
- Soto, N., Hernández, Y., Delgado, C., Rosabal, Y., Ortiz, R., Valencia, L., Borrás-Hidalgo, O., Pujol, M., and Enríquez, G. A. 2020. Field resistance to Phakopsora pachyrhizi and Colletotrichum truncatum of transgenic soybean expressing the NmDef02 plant defensin gene. Front. Plant Sci. 11: 562. doi:10.3389/fpls.2020.00562
- Sousa, L. L., Gonçalves, A. O., Gonçalves-Vidigal, M. C., Lacanallo, G. F., Fernandez, A. C., Awale, H., and Kelly, J. D. 2015. Genetic characterization and mapping of anthracnose resistance of common bean landrace cultivar corinthiano. Crop Sci. 55: 1900–1910. doi:10.2135/cropsci2014.09.0604
- Souza-Paccola, E. A., Fávaro, L. C. L., Casela, C. R., and Paccola-Meirelles, L. D. 2003. Genetic recombination in Colletotrichum sublineolum. J. Phytopathol. 151: 329–334. doi:10.1046/j.1439-0434.2003.00727.x
- Sozen, O., and Karadavut, U. 2018. Determination of genotype x environment interactions of some chickpea (Cicer arietinum L.) genotypes by using different stability methods. Tarim. Bilim. Derg. 24: 431–438. doi:10.15832/ankutbd.490930
- Stephenson, S. A., Hatfield, J., Rusu, A. G., Maclean, D. J., and Manners, J. M. 2000. CgDN3: an essential pathogenicity gene of Colletotrichum gloeosporioides necessary to avert a hypersensitive-like response in the host Stylosanthes guianensis. Mol. Plant. Microbe Interact. 13: 929–941. doi:10.1094/MPMI.2000.13.9.929
- Subedi, S., Gharti, D. B., Neupane, S., and Ghimire, T. 2016. Management of anthracnose in soybean using fungicide. J. Nep. Agric. Res. Counc. 1: 29–32. doi:10.3126/jnarc.v1i0.15721
- Takagi, R., Sakamoto, E., Kido, J. I., Inagaki, Y., Hiroshima, Y., Naruishi, K., and Yumoto, H. 2020. S100A9 increases IL-6 and RANKL expressions through MAPKs and STAT3 signaling pathways in osteocyte-like cells. Biomed Res. Int. 2020: 1–12. doi:10.1155/2020/7149408
- Talhinhas, P., Baroncelli, R., and Floch, G. Le. 2016. Anthracnose of lupins caused by Colletotrichum lupini: a recent disease and a successful worldwide pathogen. J. Plant Pathol. 98: 5–14.
- Tar’an, B., Buchwaldt, L., Tullu, A., Banniza, S., Warkentin, T. D., and Vandenberg, A. 2003. Using molecular markers to pyramid genes for resistance to ascochyta blight and anthracnose in lentil (Lens culinaris Medik). Euphytica 134: 223–230. doi:10.1023/B:EUPH.0000003913.39616.fd
- Thomas, G. J., Sweetingham, M. W., Yang, H. A., and Speijers, J. 2008. Effect of temperature on growth of Colletotrichum lupini and on anthracnose infection and resistance in lupins. Austral. Plant Pathol. 37: 35–39. doi:10.1071/AP07075
- Thomas, K. 2010. Impact of climate change on diseases of cool season grain legume crops. Clim. Chang. Manag. Cool Seas. Grain Legum. Crop. 9789048137: 99–113.
- Tivoli, B., Baranger, A., Sivasithamparam, K., and Barbetti, M. J. 2006. Annual Medicago: From a model crop challenged by a spectrum of necrotrophic pathogens to a model plant to explore the nature of disease resistance. Ann. Bot. 98: 1117–1128. doi:10.1093/aob/mcl132
- Trabanco, N., Campa, A., and Ferreira, J. J. 2015. Identification of a new chromosomal region involved in the genetic control of resistance to anthracnose in common bean. Plant Genome 8: 2014-10. doi:10.3835/plantgenome2014.10.0079
- Tullu, A., Buchwaldt, L., Lulsdorf, M., Banniza, S., Barlow, B., Slinkard, A. E., Sarker, A., Tar’an, B., Warkentin, T., and Vandenberg, A. 2006. Sources of resistance to anthracnose (Colletotrichum truncatum) in wild lens species. Genet. Resour. Crop Evol. 53: 111–119. doi:10.1007/s10722-004-1586-5
- Tullu, A., Buchwaldt, L., Warkentin, T., Taran, B., and Vandenberg, A. 2003. Genetics of resistance to anthracnose and identification of AFLP and RAPD markers linked to the resistance gene in PI 320937 germplasm of lentil (Lens culinaris Medikus). Theor. Appl. Genet. 106: 428–434. doi:10.1007/s00122-002-1042-x
- Ureña-Padilla, A. R., MacKenzie, S. J., Bowen, B. W., and Legard, D. E. 2002. Etiology and population genetics of Colletotrichum spp. causing crown and fruit rot of strawberry. Phytopathology 92: 1245–1252. doi:10.1094/PHYTO.2002.92.11.1245
- Vandenberg, A., Kiehn, F. A., Vera, C., Gaudiel, R., Buchwaldt, L., Dueck, S., Wahab, J., and Slinkard, A. E. 2002. CDC Robin lentil. Can. J. Plant Sci. 82: 111–112. doi:10.4141/P01-003
- Varanasi, A., Prasad, P. V. V., and Jugulam, M. 2016. Impact of climate change factors on weeds and herbicide efficacy. Adv. Agron. 135: 107–146.
- Varshney, R. K., Chen, W., Li, Y., Bharti, A. K., Saxena, R. K., Schlueter, J. A., Donoghue, M. T. A., Azam, S., Fan, G., Whaley, A. M., Farmer, A. D., Sheridan, J., Iwata, A., Tuteja, R., Penmetsa, R. V., Wu, W., Upadhyaya, H. D., Yang, S.-P., Shah, T., Saxena, K. B., Michael, T., McCombie, W. R., Yang, B., Zhang, G., Yang, H., Wang, J., Spillane, C., Cook, D. R., May, G. D., Xu, X., and Jackson, S. A. 2011. Draft genome sequence of pigeonpea (Cajanus cajan), an orphan legume crop of resource-poor farmers. Nat. Biotechnol. 30: 83–89. doi:10.1038/nbt.2022
- Varshney, R. K., Song, C., Saxena, R. K., Azam, S., Yu, S., Sharpe, A. G., Cannon, S., Baek, J., Rosen, B. D., Tar’an, B., Millan, T., Zhang, X., Ramsay, L. D., Iwata, A., Wang, Y., Nelson, W., Farmer, A. D., Gaur, P. M., Soderlund, C., Penmetsa, R. V., Xu, C., Bharti, A. K., He, W., Winter, P., Zhao, S., Hane, J. K., Carrasquilla-Garcia, N., Condie, J. A., Upadhyaya, H. D., Luo, M.-C., Thudi, M., Gowda, C. L. L., Singh, N. P., Lichtenzveig, J., Gali, K. K., Rubio, J., Nadarajan, N., Dolezel, J., Bansal, K. C., Xu, X., Edwards, D., Zhang, G., Kahl, G., Gil, J., Singh, K. B., Datta, S. K., Jackson, S. A., Wang, J., and Cook, D. R. 2013. Draft genome sequence of chickpea (Cicer arietinum) provides a resource for trait improvement. Nat. Biotechnol. 31: 240–246. doi:10.1038/nbt.2491
- Vasconcelos, M. J. V., Machado, M. A., Almeida, Á. M. R., Henning, A. A., Barros, E. G., and Moreira, M. A. 1994. Differentiation of Colletotrichum truncatum isolates by random amplified polymorphic DNA. Fitopatol. Bras. 19: 520–523.
- Vazin, M., Navabi, A., Pauls, P. K., McDonald, M. R., and Gillard, C. 2015. Characterization of anthracnose resistance in common bean. M.Sc. Thesis, University of Guelph, Ontario, Canada.
- Velásquez, A. C., Castroverde, C. D. M., and He, S. Y. 2018. Plant–pathogen warfare under changing climate conditions. Curr. Biol. 28: R619–R634. doi:10.1016/j.cub.2018.03.054
- Venette, J. R. 1994. First Report of lentil anthracnose (Colletotrichum truncatum) in the United States. Plant Dis. 78: 1216D. doi:10.1094/PD-78-1216D
- Verma, V., Ravindran, P., and Kumar, P. P. 2016. Plant hormone-mediated regulation of stress responses. BMC Plant Biol. 16: 86. doi:10.1186/s12870-016-0771-y
- Vidigal Filho, P. S., Gonçalves‐Vidigal, M. C., Vaz Bisneta, M., Souza, V. B., Gilio, T. A. S., Calvi, A. A., Lima, L. R. L., Pastor‐Corrales, M. A., and Melotto, M. 2020. Genome-wide association study of resistance to anthracnose and angular leaf spot in Brazilian Mesoamerican and Andean common bean cultivars. Crop Sci. 60: 2931–2950. doi:10.1002/csc2.20308
- Vieira, A. F., Almeida, L. C. S., Rodrigues, L. A., Costa, J. G. C., Melo, L. C., Pereira, H. S., Sanglard, D. A., and Souza, T. L. P. O. 2018. Selection of resistance sources to common bean anthracnose by field phenotyping and DNA marker-assisted screening. Genet. Mol. Res. 17: GMR18066. doi:10.4238/gmr18066
- Walsh, M. J., Delaney, R. H., Groose, R. W., and Krall, J. M. 2001. Performance of annual medic species (Medicago spp.) in southeastern Wyoming. Agron. J. 93: 1249–1256. doi:10.2134/agronj2001.1249
- Wang, H., Liu, R., You, M. P., Barbetti, M. J., and Chen, Y. 2021. Pathogen biocontrol using plant growth-promoting bacteria (PGPR): role of bacterial diversity. Microorganisms 9: 1988. doi:10.3390/microorganisms9091988
- Wang, N., Xia, E. H., and Gao, L. Z. 2016. Genome-wide analysis of WRKY family of transcription factors in common bean, Phaseolus vulgaris: chromosomal localization, structure, evolution and expression divergence. Plant Gene 5: 22–30. doi:10.1016/j.plgene.2015.11.003
- Wang, Q. H., Fan, K., Li, D. W., Han, C. M., Qu, Y. Y., Qi, Y. K., and Wu, X. Q. 2020. Identification, virulence and fungicide sensitivity of Colletotrichum gloeosporioides s.s. responsible for walnut anthracnose disease in China. Plant Dis. 104: 1358–1368. doi:10.1094/PDIS-12-19-2569-RE
- Wang, Y., Schuck, S., Wu, J., Yang, P., Döring, A. C., Zeier, J., and Tsuda, K. 2018. A mpk3/6-wrky33-ald1-pipecolic acid regulatory loop contributes to systemic acquired resistance. Plant Cell. 30: 2480–2494. doi:10.1105/tpc.18.00547
- Weir, B. S., Johnston, P. R., and Damm, U. 2012. The Colletotrichum gloeosporioides species complex. Stud. Mycol. 73: 115–180. doi:10.3114/sim0011
- Williams, R. J. 1977. The identification of multiple disease resistance in cowpea. Trop. Agric. Trinidad 54: 53–59.
- Wojakowska, A., Kułak, K., Jasiński, M., Kachlicki, P., Stawiński, S., and Stobiecki, M. 2015. Metabolic response of narrow leaf lupine (Lupinus angustifolius) plants to elicitation and infection with Colletotrichum lupini under field conditions. Acta Physiol. Plant 37: 1–12.
- Wojakowska, A., Muth, D., Narożna, D., Mądrzak, C., Stobiecki, M., and Kachlicki, P. 2013. Changes of phenolic secondary metabolite profiles in the reaction of narrow leaf lupin (Lupinus angustifolius) plants to infections with Colletotrichum lupini fungus or treatment with its toxin. Metabolomics 9: 575–589. doi:10.1007/s11306-012-0475-8
- Wu, J., Zhu, J., Wang, L., and Wang, S. 2017. Genome-wide association study identifies NBS-LRR-encoding genes related with anthracnose and common bacterial blight in the common bean. Front. Plant Sci. 8: 1398. doi:10.3389/fpls.2017.01398
- Xie, L., Zhang, J. Z., Wan, Y., and Hu, D. W. 2010. Identification of Colletotrichum spp. isolated from strawberry in Zhejiang Province and Shanghai City, China. J. Zhejiang Univ. Sci. B 11: 61–70. doi:10.1631/jzus.B0900174
- Xu, S., Christensen, M. J., and Li, Y. 2017. Pathogenicity and characterization of Colletotrichum lentis: A causal agent of anthracnose in common vetch (Vicia sativa). Eur. J. Plant Pathol. 149: 719–731. doi:10.1007/s10658-017-1221-x
- Xu, W., Zhang, Q., Yuan, W., Xu, F., Muhammad Aslam, M., and Miao, R. 2020. The genome evolution and low-phosphorus adaptation in white lupin. Nat. Commun. 11: 1069.
- Yang, H. C., and Hartman, G. L. 2015. Anthracnose. In Compendium of Soybean Diseases and Pests; Hartman, G. L., Rupe, J., and Sikora, E. J., Eds. American Phytopathological Society: St Paul, MN, pp 31–34.
- Yang, H. C., Haudenshield, J. S., and Hartman, G. L. 2012. First report of Colletotrichum chlorophyti causing soybean anthracnose. Plant Dis. 96: 1699. doi:10.1094/PDIS-06-12-0531-PDN
- Yang, H. C., Haudenshield, J. S., and Hartman, G. L. 2014. Colletotrichum incanum sp. Nov., a curved-conidial species causing soybean anthracnose in USA. Mycologia 106: 32–42. doi:10.3852/13-013
- Yang, H. C., Haudenshield, J. S., and Hartman, G. L. 2015. Multiplex real-time PCR detection and differentiation of Colletotrichum species infecting soybean. Plant Dis. 99: 1559–1568. doi:10.1094/PDIS-11-14-1189-RE
- Yang, H., Boersma, J. G., You, M., Buirchell, B. J., and Sweetingham, M. W. 2004. Development and implementation of a sequence-specific PCR marker linked to a gene conferring resistance to anthracnose disease in narrow-leafed lupin (Lupinus angustifolius L.). Mol. Breed. 14: 145–151. doi:10.1023/B:MOLB.0000038003.49638.97
- Yang, H., Lin, R., Renshaw, D., Li, C., Adhikari, K., Thomas, G., Buirchell, B., Sweetingham, M., and Yan, G. 2010. Development of sequence-specific PCR markers associated with a polygenic controlled trait for marker-assisted selection using a modified selective genotyping strategy: a case study on anthracnose disease resistance in white lupin (Lupinus albus L.). Mol. Breed. 25: 239–249. doi:10.1007/s11032-009-9325-4
- Yang, H., Renshaw, D., Thomas, G., Buirchell, B., and Sweetingham, M. 2008. A strategy to develop molecular markers applicable to a wide range of crosses for marker assisted selection in plant breeding: a case study on anthracnose disease resistance in lupin (Lupinus angustifolius L.). Mol. Breed. 21: 473–483. doi:10.1007/s11032-007-9146-2
- Young, R. A., and Kelly, J. D. 1996. RAPD markers flanking the Are gene for anthracnose resistance in common bean. J. Am. Soc. Hort. Sci. 121: 37–41. doi:10.21273/JASHS.121.1.37
- Young, R. A., and Kelly, J. D. 1997. RAPD markers linked to three major anthracnose resistance genes in common bean. Crop Sci. 37: 940–946. doi:10.2135/cropsci1997.0011183X003700030039x
- Young, R. A., Melotto, M., Nodari, R. O., and Kelly, J. D. 1998. Marker-assisted dissection of the oligogenic anthracnose resistance in the common bean cultivar, “G 2333”. Theor. Appl. Genet. 96: 87–94. doi:10.1007/s001220050713
- Yousef, S. 2021. Bean Anthracnose Control Using Different Beneficial Bacteria, pp 1–20.
- Yu, J., Wu, J., Guo, Z., Zhang, X., Xu, M., Yu, J., Liu, T., and Chi, Y. 2020. First report of peanut anthracnose caused by Colletotrichum truncatum in China. Plant Dis. 104: 1555. doi:10.1094/PDIS-08-19-1599-PDN
- Zhang, L., Song, L., Xu, X., Zou, X., Duan, K., and Gao, Q. 2020. Characterization and fungicide sensitivity of Colletotrichum species causing strawberry anthracnose in eastern China. Plant Dis. 104: 1960–1968. doi:10.1094/PDIS-10-19-2241-RE
- Zhao, Q., Chen, X., Ren, G. W., Wang, J., Liu, L., Qian, W. G., and Wang, J. 2021. First report of Colletotrichum chlorophyti causing peanut anthracnose in China. Plant Dis. 105: 226. doi:10.1094/PDIS-08-19-1605-PDN
- Zhu, L., Yang, Q., Yu, X., Fu, X., Jin, H., and Yuan, F. 2022. Transcriptomic and metabolomic analyses reveal a potential mechanism to improve soybean resistance to anthracnose. Front. Plant Sci. 13: 850829. doi:10.3389/fpls.2022.850829
- Zuiderveen, G. H. 2015. The Genetics of Anthracnose Resistance in Common Bean. East Lansing, MI: Michigan State University. p. 75.
- Zuiderveen, G. H., Padder, B. A., Kamfwa, K., Song, Q., and Kelly, J. D. 2016. Genome-Wide association study of anthracnose resistance in Andean beans (Phaseolus vulgaris). PLOS One 11: e0156391. doi:10.1371/journal.pone.0156391