Abstract
The COVID-19 pandemic is a very contagious respiratory illness with has affected millions of individuals worldwide. In addition to the well-known symptoms of any respiratory virus, COVID-19 can present with anosmia (failure to smell) and dysgeusia (distortion of the sense of taste). It appears to be a genetic link to the biological mechanisms underlying COVID-19-related anosmia and dysgeusia. Significant locus in the vicinity of the UGT2A1 and UGT2A2 genes are currently considered as the main culprit of the symptoms. However, more studies are needed to delineate a clear pathophysiology.
Communicated by Ramaswamy H. Sarma
Introduction
The COVID-19 pandemic is a very contagious respiratory illness with effects that have been undesirable to global health, society, and economy (Hendaus & Jomha, Citation2021).
The world health organization (WHO) has reported 340,543,962 confirmed cases of COVID-19, including 5,570,163 deaths as of January 21, 2022. In addition, more than 9.5 Billion vaccines have been given (WHO, 2022). In addition to the well-known symptoms of any respiratory virus, COVID-19 can present with anosmia (failure to smell) and dysgeusia (distortion of the sense of taste) (Marinosci et al., Citation2020).
Dysgeusia is a sensory estate of losing taste discernment (Mutiawati et al., 2021), while anosmia (failure to smell) is a severe condition of hyposmia (a decreased capability of smelling) . It reflects a part of olfactory dysfunction where an individual cannot detect odor or cannot properly smell. This condition has a prevalence of around 15 of every 1000 people in the United States (prior to the COVID-19 pandemic) (Huynh et al., Citation2020). However, the prevalence of smelling and tasting dysfunction in COVID-19 patients can reach to 33.5 and 35.6%, respectively (Galluzzi et al., Citation2021), and the two symptoms have affected approximately 1.6 million Americans for longer than six months the initial infection with SARS-Cov-2 (Khan et al., Citation2022).
The loss of vital senses has been linked with changes in appetite and quality of life. Moreover, it can decrease the ability to detect harmful smoke or gases, aggravate worries about personal hygiene and can decrease social interaction (Arora et al., Citation2008; Croy et al., Citation2014).
Current data has shown a possible association between a genetic risk factor and anosmia/dysgeusia post- COVID-19 infection. Shelton et al (Shelton et al., Citation2022) reported a genome-wide significant locus in the vicinity of the UGT2A1 and UGT2A2 genes in COVID-19 patients with anosmia/dysgeusia. The investigators conducted a multi-ancestry genome-wide association study on 69,841 person infected with SARS-CoV-2. Approximately 70% of them reported anosmia or dysgeusia, and genetic investigation resulted in pinpointing locus UGT2A1 and UGT2A2 to be linked to COVID-19-related anosmia/dysgeusia.
Uridine diphosphate glucuronosyltransferases (UGTs)
Several medications and non-drug xenobiotics, such as dietary, environmental, dietary and industrial chemicals, are non-polar, lipophilic compounds. The lipophilic characteristic of those compounds hampers their proper excretion from the body. Hence, they require metabolism to be changed from lipophilic estate to hydrophilic and then excretion (Rowland et al., Citation2013). Glucuronidation catalyzed by uridine diphosphate glucuronosyltransferases (UGTs) is one of the most important xenobiotic metabolism mechanisms. UGTs can generate the conjugation of glucuronic acid to hydrophilic substances for excretion, such as bile acids and hormones (Kiang et al., Citation2005). The UGT membrane proteins are categorized into two major groups UGT1 and UGT2 and the latter is partitioned into UGT2A and UGT2B. The descendants of UGT1 family are encoded by a unique gene located on chromosome 2q37.1, while the UGT2 family members are encoded by genes located () on chromosome 4q13.2, 1200 kbp (Fujiwara et al., Citation2016; Tourancheau et al., Citation2016).
Figure 1. The uridine diphosphate glucuronosyltransferases (UGT) membrane proteins are categorized into two major groups UGT1 and UGT2 and the latter is partitioned into UGT2A and UGT2B. The UGT2 family members are encoded by genes located on chromosome 4 and in the longer arm (q) at position 13.2, 1200 kbp (kilobase pairs).
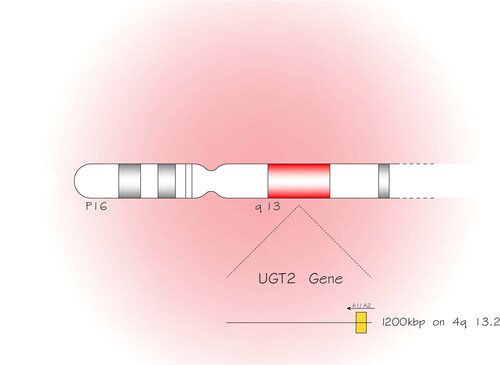
The diversity of UGT isoforms are linked to specific diseases . For example, deficiency of UGT1A1 (highly expressed in liver) can lead to inheritable unconjugated hyper-bilirubinemic diseases including neonatal jaundice (Bartlett & Gourley, Citation2011) . On the other hand, UGT2B15 and 2B17 are quite ubiquitous in the prostate gland and their polymorphism is highly linked to prostate cancer (Park et al., Citation2004; Park et al., Citation2006).
Anosmia and dysgeusia
Prolonged anosmia has been reported in 2006 due to severe acute respiratory syndrome (SARS), a very close relative to SARS-CoV-2 (Hwang, Citation2006). In addition, in 2008, researches have used a murine model to show that SARS-COV enters the brain primarily via the olfactory bulb causing anosmia in mice transgenic for human angiotensin-converting enzyme 2 (ACE-2) (Netland et al., Citation2008).
SARS-CoV-2 spike protein utilizes ACE-2 to enter the human cell (Hendaus, Citation2021) and the latter is found on the nasal mucosa, where it contributes in respiratory inflammatory illnesses and can be linked to asymptomatic infection and high transmissibility (Takabayashi et al., Citation2022).
The primary step of odor recognition occurs in the pseudostratified olfactory epithelium mostly comprised of olfactory sensory neurons surrounded by ancillary cells known as sustentacular cells. The olfactory neurons propel their axons to the olfactory bulb in the central nervous system (CNS) proposing a conceivable conduit for bugs to penetrate the CNS by escaping the blood brain barrier (Bryche et al, Citation2020). Odorant metabolizing enzymes (OMEs) are found in the olfactory epithelium where they play a crucial role in the process of smell. These enzymes catalyze the fast biotransformation of odorants resulting in either formation of a new odor or elimination of the primary smell. UGT2A1 and UGT2A2 are found in the olfactory epithelium, and are involved in getting rid of the odorants that go through the nasal cavity and attach to olfactory receptors. The process of glucuronidation of odorants leads to sub-optimal stimulation of the olfactory bulb, leading to the decrease perception of the brain and hence possible anosmia (Neiers et al., Citation2021). This phenomenon has been known since 1991, where Lazard et al (Lazard et al., Citation1991) suggested that glucuronic acid conjugation eliminates the capability of odorants to stimulate olfactory adenylyl cyclase, a key enzyme in transmembrane signaling . In addition, broad spectrum drug-detoxification enzymes can aggravate the situation supporting the role that olfactory UGT possesses in abolishing diverse odorant signals. Bryche et al investigated the effect of SARS-CoV-2 infection on the olfactory system in golden Syrian hamsters. The investigators reported enormous damage of the olfactory epithelium as early as 2 days after nasal administration of SARS-CoV-2, causing an enormous loss of cilia necessary for odor detection. These injuries were linked to infection of a many of sustentacular cells but not of olfactory neurons. Furthermore, the investigators did not observe any manifestation of the virus in the olfactory bulbs. However, substantial infiltration of immune cells in the olfactory epithelium and lamina propria of infected hamsters, causing desquamation of the olfactory epithelium (Bryche et al., Citation2020). Brann et al second the idea that SARs-CoV-2 affects cells not related to the neurons, but through trepidation of supporting cells. The investigators used a murine model exposing that olfactory mucosa demonstrates two key genes responsible for SARS-CoV-2 entry into the cell, ACE-2 and Transmembrane protease, serine 2 (TMPRSS2). The study showed a persistent demonstration of the ACE-2 in the olfactory bulb pericytes and epithelial sustentacular cells in the mouse. The authors concluded that SARS-CoV-2 infection of cells (other than neurons) can result in anosmia (Brann et al., 2020).
ACE-2 receptors have been also reported in the salivary glands and taste buds (Xu et al., Citation2020). possibly resulting in salivary dysfunction causing dysgeusia. Moreover, the literature has shown that SARS-CoV RNA can be detected in saliva prior to respiratory cells (Liu et al., Citation2011). Olfactory and gustatory have common associations. A damage of the olfactory epithelium might result in damage to the gustatory process. A plausible reported mechanism states that direct damage to nonneuronal cells in the olfactory epithelium due to SARS-CoV-2 replication can harm the same way the ACE2-expressing cells of the taste buds and peripheral taste neurosensory chemoreceptors (Finsterer & Stollberger, Citation2020).
Conclusion
It appears to be a genetic link to the biological mechanisms underlying COVID-19-related anosmia and dysgeusia. More studies are needed to delineate a clear pathophysiology.
For the meantime, commonly used precautions employed since the beginning of the COVID-19 pandemic are recommended.
Acknowledgments
Open Access funding provided by the Qatar National Library.
Funding
The author(s) reported there is no funding associated with the work featured in this article.
References
- Arora, N. K., Hesse, B. W., Rimer, B. K., Viswanath, K., Clayman, M. L., & Croyle, R. T. (2008). Frustrated and confused: the American public rates its cancer-related information-seeking experiences. Journal of General Internal Medicine, 23(3), 223–228. https://doi.org/10.1007/s11606-007-0406-y
- Bartlett, M. G., & Gourley, G. R. (2011). Assessment of UGT polymorphisms and neonatal jaundice. Seminars in Perinatology, 35(3), 127–133. https://doi.org/10.1053/j.semperi.2011.02.006
- Brann, D. H., Tsukahara, T., Weinreb, C., Lipovsek, M., Van den Berge, K., Gong, B., Chance, R., Macaulay, I. C., Chou, H.-J., Fletcher, R. B., Das, D., Street, K., de Bezieux, H. R., Choi, Y.-G., Risso, D., Dudoit, S., Purdom, E., Mill, J., Hachem, R. A., … Datta, S. R. (2020). Non-neuronal expression of SARS-CoV-2 entry genes in the olfactory system suggests mechanisms underlying COVID-19-associated anosmia. Science Advances, 6(31), eabc5801. https://doi.org/10.1126/sciadv.abc5801
- Bryche, B., St Albin, A., Murri, S., Lacôte, S., Pulido, C., Ar Gouilh, M., Lesellier, S., Servat, A., Wasniewski, M., Picard-Meyer, E., Monchatre-Leroy, E., Volmer, R., Rampin, O., Le Goffic, R., Marianneau, P., & Meunier, N. (2020). Massive transient damage of the olfactory epithelium associated with infection of sustentacular cells by SARS-CoV-2 in golden Syrian hamsters. Brain, Behavior, and Immunity, 89, 579–586. https://doi.org/10.1016/j.bbi.2020.06.032
- Croy, I., Nordin, S., & Hummel, T. (2014). Olfactory disorders and quality of life-an updated review. Chemical Senses, 39(3), 185–194. https://doi.org/10.1093/chemse/bjt072
- Finsterer, J., & Stollberger, C. (2020). Causes of hypogeusia/hyposmia in SARS-CoV2 infected patients. Journal of Medical Virology, 92(10), 1793–1794. https://doi.org/10.1002/jmv.25903
- Fujiwara, R., Yokoi, T., & Nakajima, M. (2016). Structure and Protein-Protein Interactions of Human UDP-Glucuronosyltransferases. Frontiers in Pharmacology, 7, 388 https://doi.org/10.3389/fphar.2016.00388
- Galluzzi, F., Rossi, V., Bosetti, C., & Garavello, W. (2021). Risk Factors for Olfactory and Gustatory Dysfunctions in Patients with SARS-CoV-2 Infection. Neuroepidemiology, 55(2), 154–161. https://doi.org/10.1159/00051488
- Hendaus, M. A. (2021). Letter to the Editor: COVID-19 utilization of ACE-2 receptor to enter the host cell. Journal of Biomolecular Structure & Dynamics, 1–2. https://doi.org/10.1080/07391102.2021.1917174
- Hendaus, M. A., & Jomha, F. A. (2021). Covid-19 induced superimposed bacterial infection. Journal of Biomolecular Structure & Dynamics, 39(11), 4185–4191. https://doi.org/10.1080/07391102.2020.1772110
- Huynh, P. P., Ishii, L. E., & Ishii, M. (2020). What Is Anosmia? JAMA, 324(2), 206 https://doi.org/10.1001/jama.2020.10966
- Hwang, C. S. (2006). Olfactory neuropathy in severe acute respiratory syndrome: report of A case. Acta Neurologica Taiwanica, 15(1), 26–28.
- Khan, A. M., Kallogjeri, D., & Piccirillo, J. F. (2022). Growing public health concern of COVID-19 chronic olfactory dysfunction. JAMA Otolaryngol Head & Neck Surgery, 148(1), 81–82. https://doi.org/10.1001/jamaoto.2021.3379
- Kiang, T. K., Ensom, M. H., & Chang, T. K. (2005). UDP-glucuronosyltransferases and clinical drug-drug interactions. Pharmacology & Therapeutics, 106(1), 97–132. https://doi.org/10.1016/j.pharmthera.2004.10.013
- Lazard, D., Zupko, K., Poria, Y., Nef, P., Lazarovits, J., Horn, S., Khen, M., & Lancet, D. (1991). Odorant signal termination by olfactory UDP glucuronosyl transferase. Nature, 349(6312), 790–793. https://doi.org/10.1038/349790a0
- Liu, L., Wei, Q., Alvarez, X., Wang, H., Du, Y., Zhu, H., Jiang, H., Zhou, J., Lam, P., Zhang, L., Lackner, A., Qin, C., & Chen, Z. (2011). Epithelial cells lining salivary gland ducts are early target cells of severe acute respiratory syndrome coronavirus infection in the upper respiratory tracts of rhesus macaques. Journal of Virology, 85(8), 4025–4030. https://doi.org/10.1128/JVI.02292-10
- Marinosci, A., Landis, B. N., & Calmy, A. (2020). Possible link between anosmia and COVID-19: sniffing out the truth. European Archives of Oto-Rhino-Laryngology : official Journal of the European Federation of Oto-Rhino-Laryngological Societies (EUFOS) : affiliated with the German Society for Oto-Rhino-Laryngology - Head and Neck Surgery, 277(7), 2149–2150. https://doi.org/10.1007/s00405-020-05966-0
- Mutiawati, E., Fahriani, M., Mamada, S. S., Fajar, J. K., Frediansyah, A., Maliga, H. A., Ilmawan, M., Emran, T. B., Ophinni, Y., Ichsan, I., Musadir, N., Rabaan, A. A., Dhama, K., Syahrul, S., Nainu, F., & Harapan, H. (2021). Anosmia and dysgeusia in SARS-CoV-2 infection: incidence and effects on COVID-19 severity and mortality, and the possible pathobiology mechanisms - a systematic review and meta-analysis. F1000Research, 10, 40. https://doi.org/10.12688/f1000research.28393.1
- Neiers, F., Jarriault, D., Menetrier, F., Briand, L., & Heydel, J. M. (2021). The odorant metabolizing enzyme UGT2A1: Immunolocalization and impact of the modulation of its activity on the olfactory response. PloS One, 16(3), e0249029 https://doi.org/10.1371/journal.pone.0249029
- Netland, J., Meyerholz, D. K., Moore, S., Cassell, M., & Perlman, S. (2008). Severe acute respiratory syndrome coronavirus infection causes neuronal death in the absence of encephalitis in mice transgenic for human ACE2. Journal of Virology, 82(15), 7264–7275. https://doi.org/10.1128/JVI.00737-08
- Park, J., Chen, L., Ratnashinge, L., Sellers, T. A., Tanner, J. P., Lee, J. H., Dossett, N., Lang, N., Kadlubar, F. F., Ambrosone, C. B., Zachariah, B., Heysek, R. V., Patterson, S., & Pow-Sang, J. (2006). Deletion polymorphism of UDP-glucuronosyltransferase 2B17 and risk of prostate cancer in African American and Caucasian men. Cancer Epidemiology, Biomarkers & Prevention: A Publication of the American Association for Cancer Research, 15(8), 1473–1478. https://doi.org/10.1158/1055-9965.EPI-06-0141
- Park, J., Chen, L., Shade, K., Lazarus, P., Seigne, J., Patterson, S., Helal, M., & Pow-Sang, J. (2004). Asp85tyr polymorphism in the udp-glucuronosyltransferase (UGT) 2B15 gene and the risk of prostate cancer. The Journal of Urology, 171(6 Pt 1), 2484–2488. https://doi.org/10.1097/01.ju.0000117748.44313.43
- Rowland, A., Miners, J. O., & Mackenzie, P. I. (2013). The UDP-glucuronosyltransferases: their role in drug metabolism and detoxification. The International Journal of Biochemistry & Cell Biology, 45(6), 1121–1132. https://doi.org/10.1016/j.biocel.2013.02.019
- Shelton, J. F., Shastri, A. J., & Fletez-Brant, K. (2022). The UGT2A1/UGT2A2 locus is associated with COVID-19-related loss of smell or taste. Nature Genetics, https://doi.org/10.1038/s41588-021-00986-w
- Takabayashi, T., Yoshida, K., Imoto, Y., Schleimer, R. P., & Fujieda, S. (2022). Regulation of the expression of SARS-CoV-2 receptor angiotensin-converting enzyme 2 in nasal mucosa. American Journal of Rhinology & Allergy, 36(1), 115–122. https://doi.org/10.1177/19458924211027798
- Tourancheau, A., Margaillan, G., Rouleau, M., Gilbert, I., Villeneuve, L., Lévesque, E., Droit, A., & Guillemette, C. (2016). Unravelling the transcriptomic landscape of the major phase II UDP-glucuronosyltransferase drug metabolizing pathway using targeted RNA sequencing. The Pharmacogenomics Journal, 16(1), 60–70. https://doi.org/10.1038/tpj.2015.20
- WHO Coronavirus (COVID-19) Dashboard (2022). Available from WHO Coronavirus (COVID-19) Dashboard | WHO Coronavirus (COVID-19) Dashboard With Vaccination Data Accessed January 23,
- Xu, H., Zhong, L., Deng, J., Peng, J., Dan, H., Zeng, X., Li, T., & Chen, Q. (2020). High expression of ACE2 receptor of 2019-nCoV on the epithelial cells of oral mucosa. International Journal of Oral Science, 12(1), 8. https://doi.org/10.1038/s41368-020-0074-x